Abstract
1. An analysis has been made of the change in optical retradation of the membrane elicited by the application of voltage—clamp pulses in squid giant axons.
2. The retardation response consists of three separate voltage—dependent components. For freshly mounted axons, defined as being in state 1, hyperpolarizing pulses give a rapid increase in the light intensity measured with crossed polarizers which has been termed the fast phase. This is followed by a rather slow return towards the base line termed the rebound. On treatment of the axon with certain agents that include tetrodotoxin, high calcium and terbium, the rebound disappears and the fast phase slows down, increases in size, and has a new slow component added to it. This transition from state 1 to a second state, 2, appears to be irreversible.
3. In state 1, the time constant of the fast phase is 20-40 μsec at 13° C; it has a very large negative temperature coefficient (Q10 = Ca.⅛). The size of the retardation change is independent of temperature and varies as the square of the applied voltage, but the voltage—retardation curve is symmetrical about a point well beyond zero membrane potential, at an internal potential of around + 70 mV. In state 2, the time constant is about five times larger, and varies much less markedly with temperature; the apex of the voltage—retardation curve is shifted to + 200 mV.
4. The rebound has a time constant of the order of 20 msec at 13° C. A 10° rise in temperature more than halves the time constant and roughly doubles the amplitude of the rebound. The voltage dependence of the rebound differed from that of the fast phase.
5. The slow component of state 2 has a time constant of about 2 msec which does not change noticeably between 10 and 25° C. The size of this component seems to be linearly dependent on the applied voltage, rather than obeying a square law.
6. A tenfold increase in external calcium concentration had no discernible effect on the fast and slow phases, but reversibly reduced the amplitude of the rebound nearly to half.
7. In experiments on perfused axons, the retardation response was not measurably altered by any of the modifications made to the composition of the perfusing fluid.
8. There was some indication of the possible existence of a small current- or conductance-dependent component of the retardation response.
9. These phenomena seem likely to originate either from molecular relaxation processes analogous with the Kerr effect, or from changes in membrane thickness under the influence of the pressure exerted by the electric field. However, the specific molecules involved in the retardation response cannot yet be identified.
Full text
PDF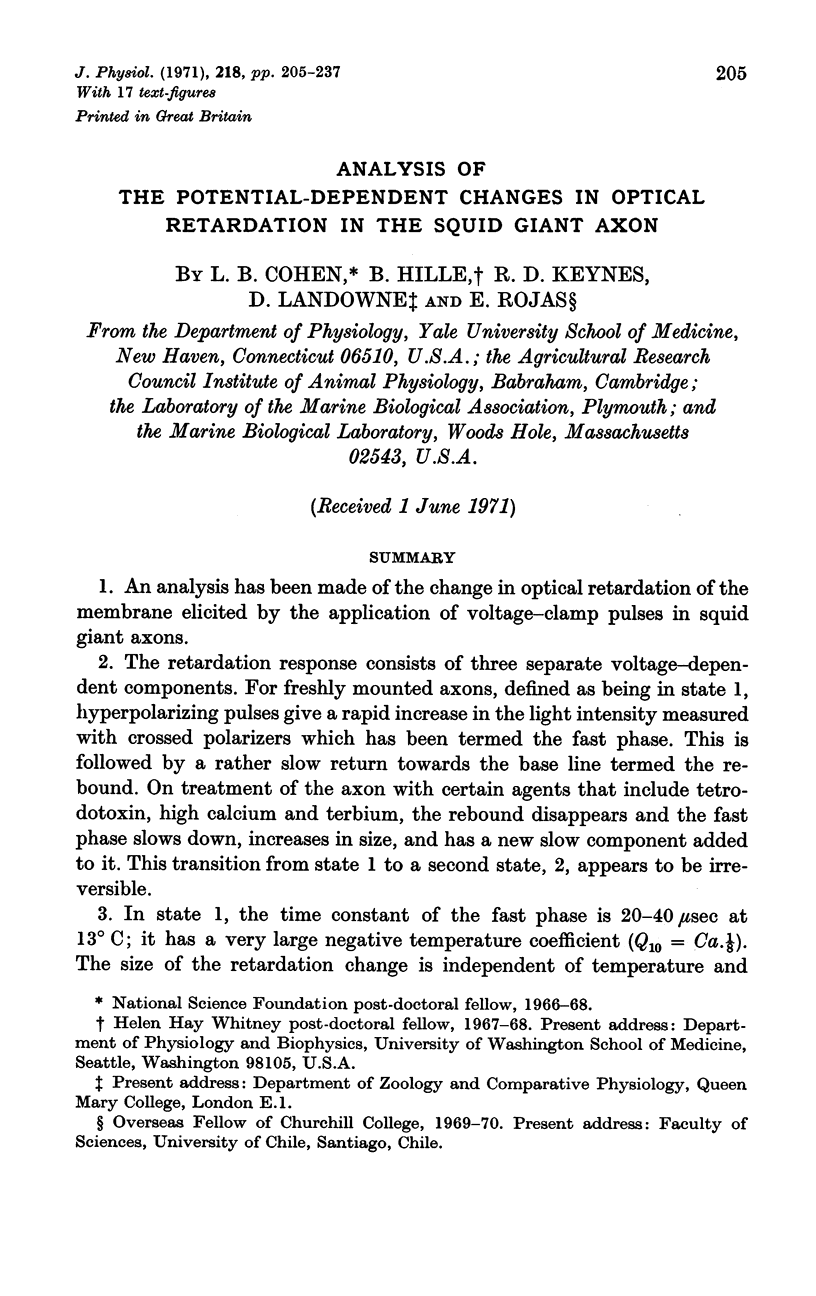
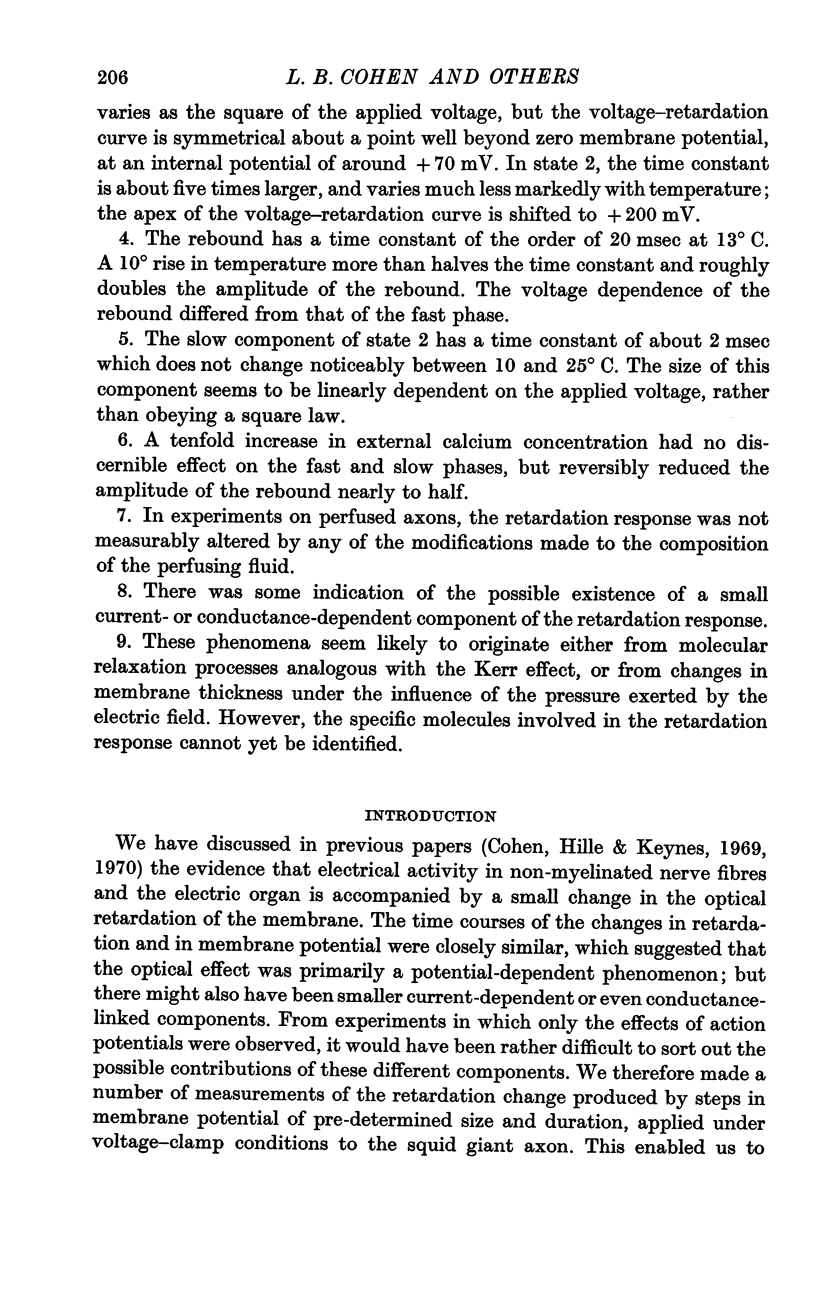
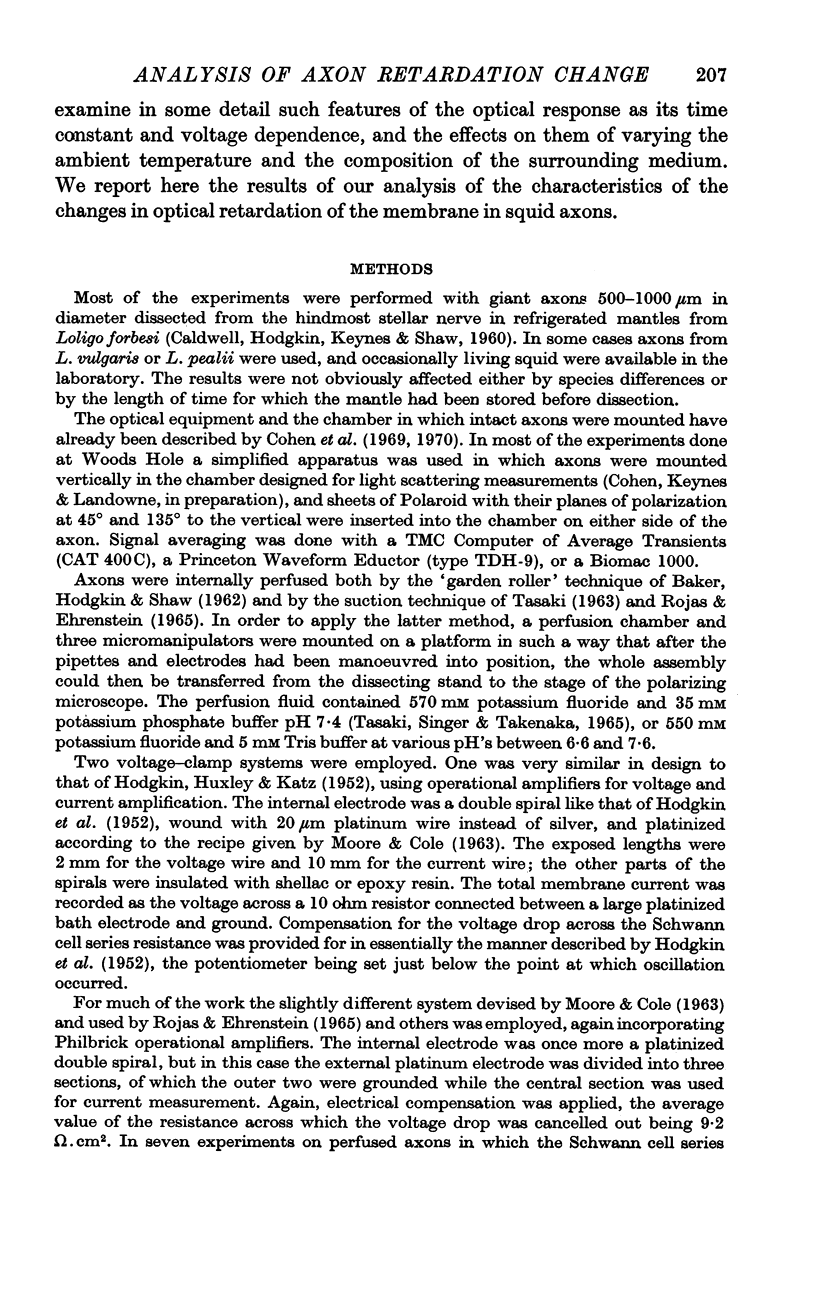
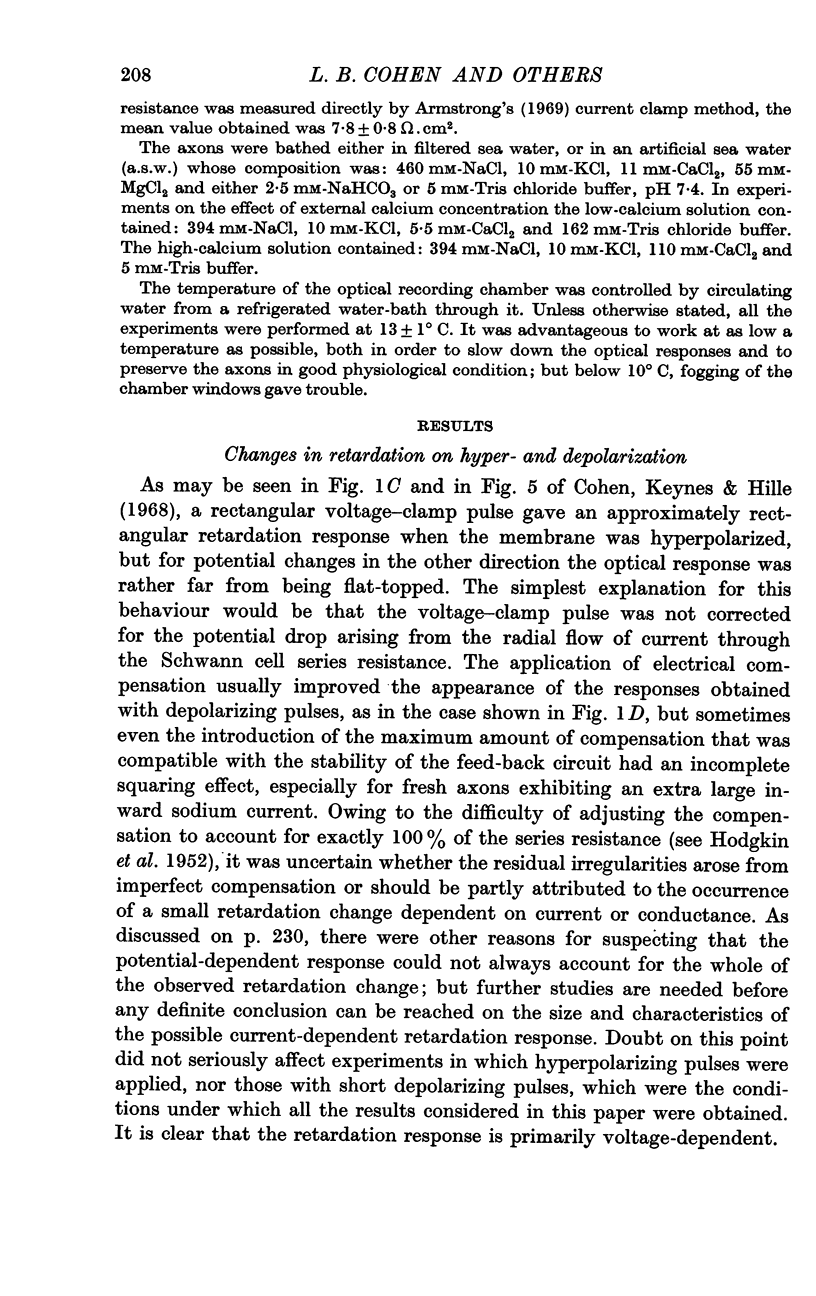
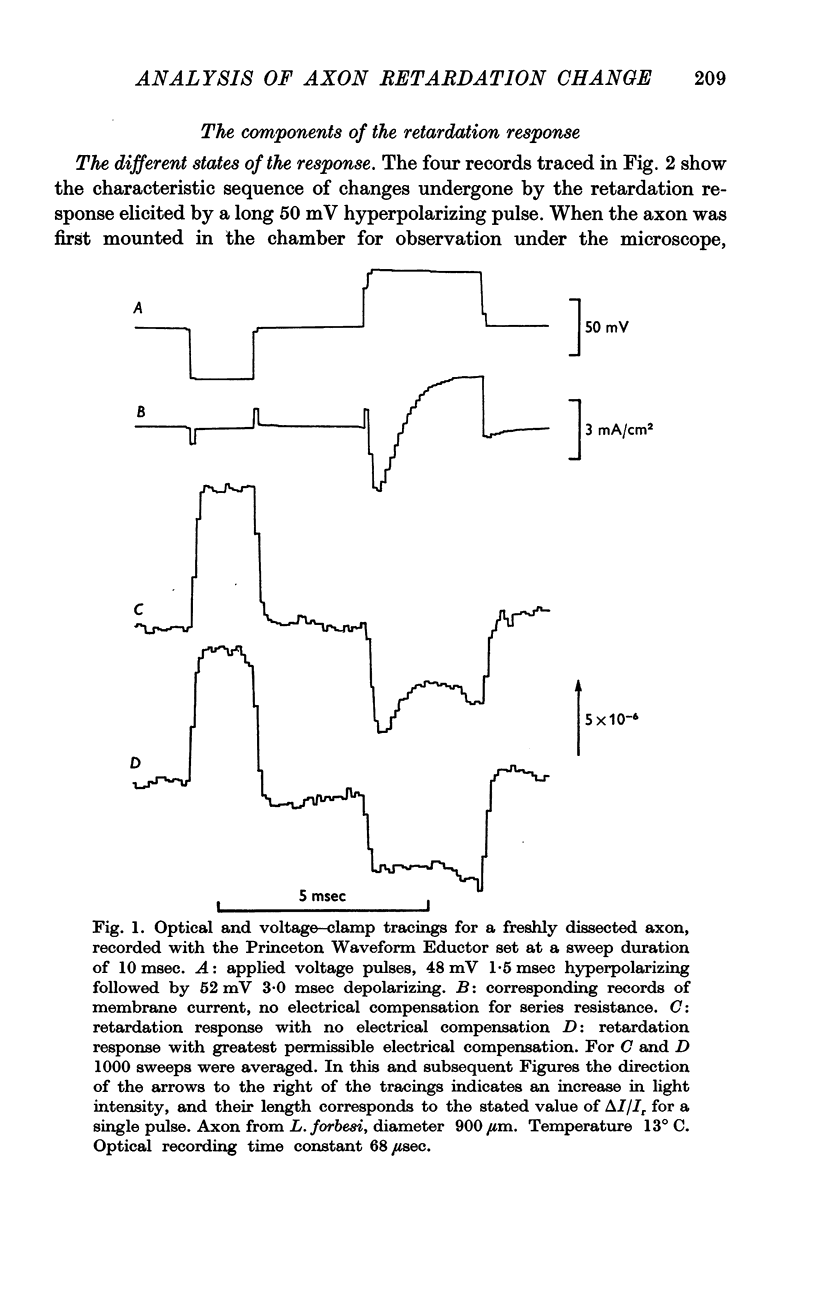
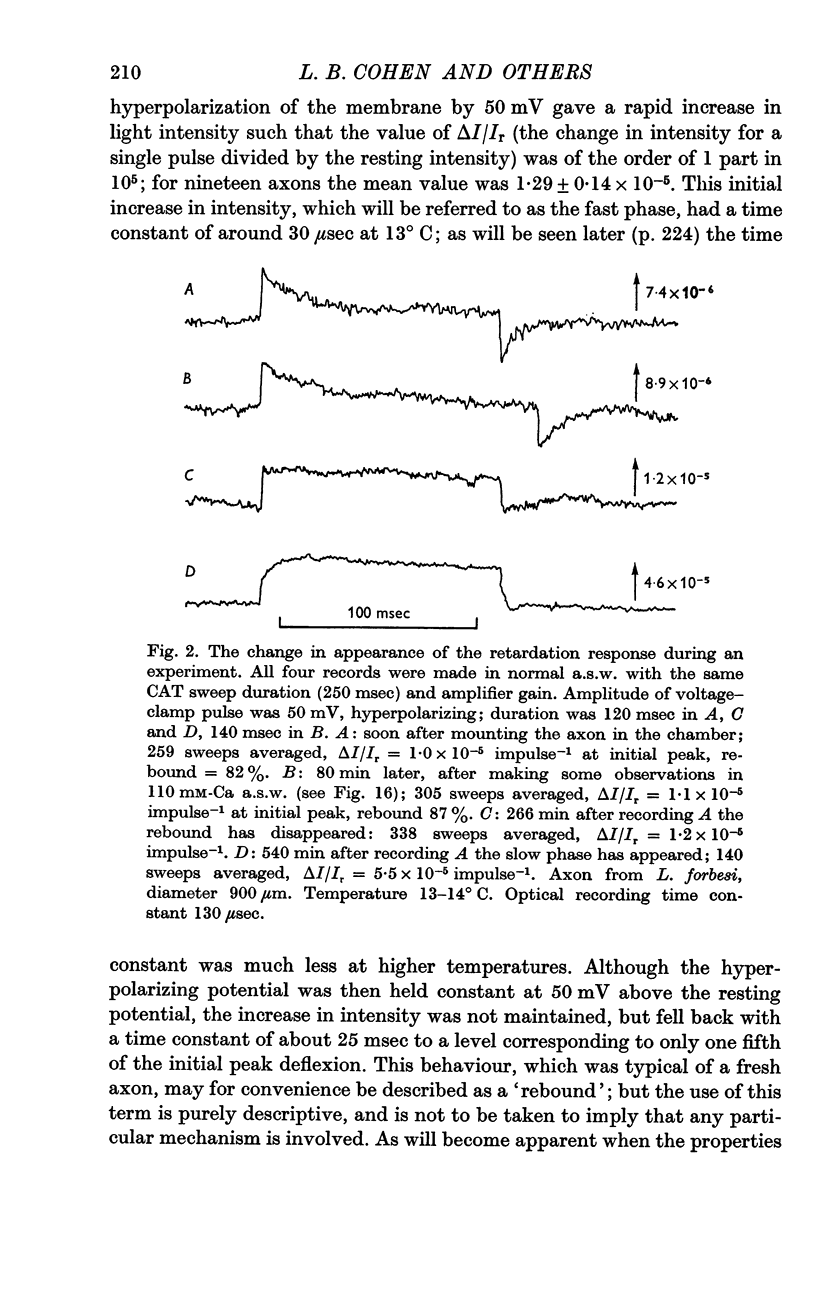
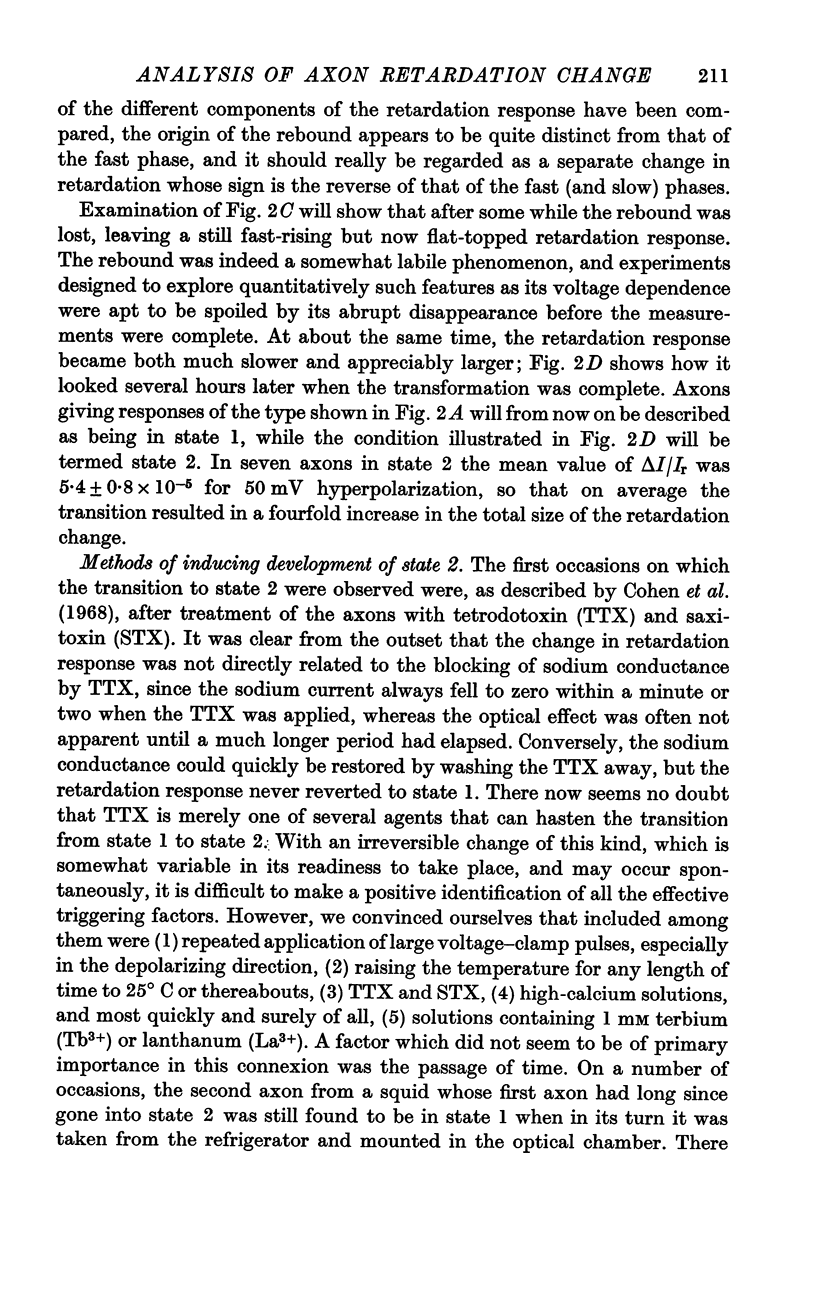
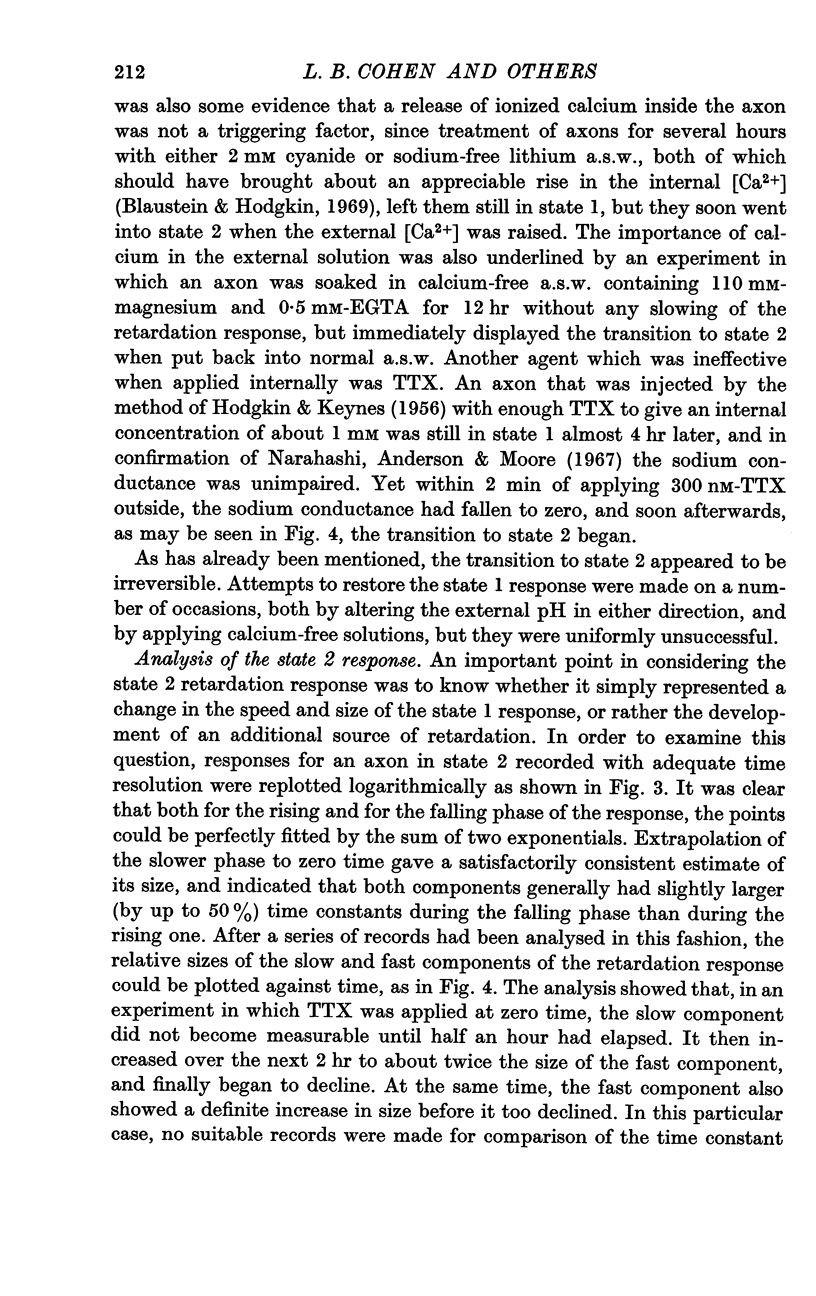
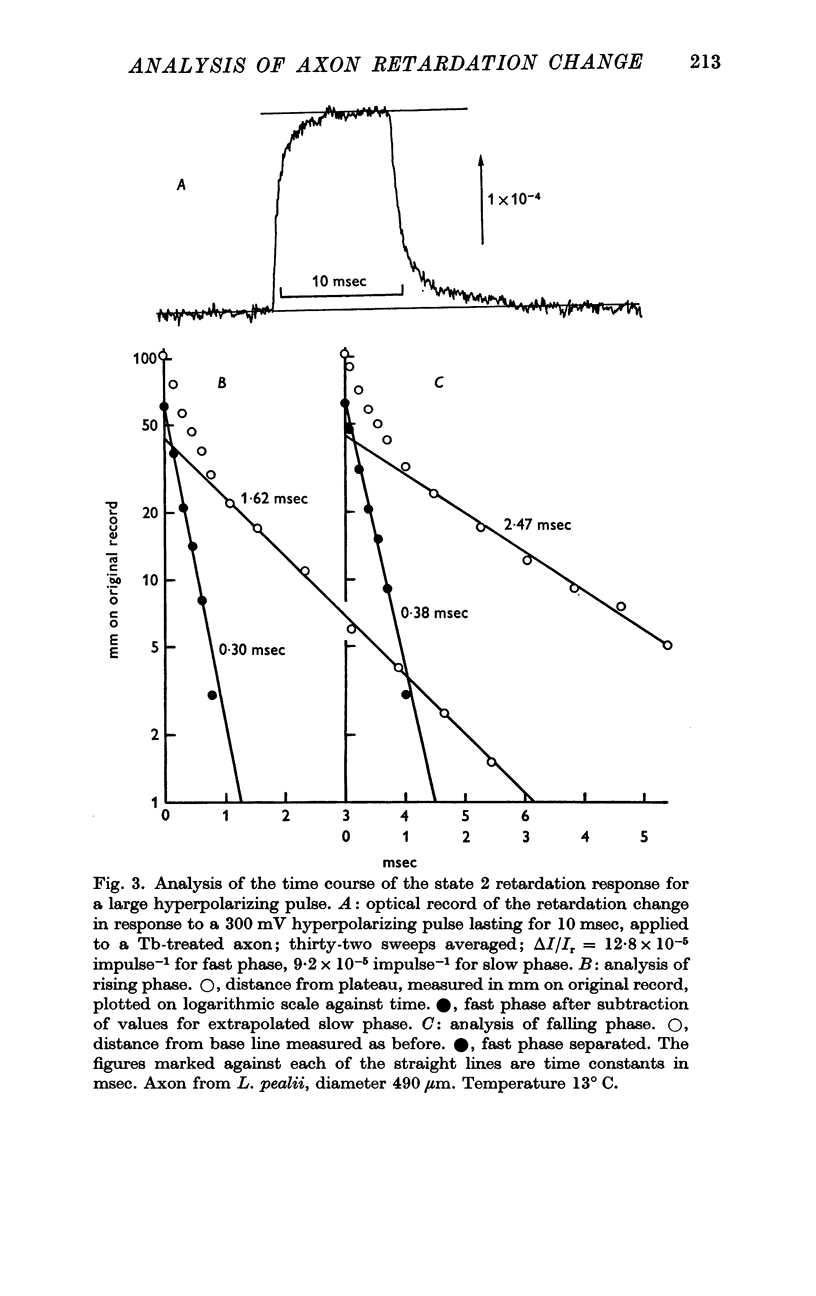
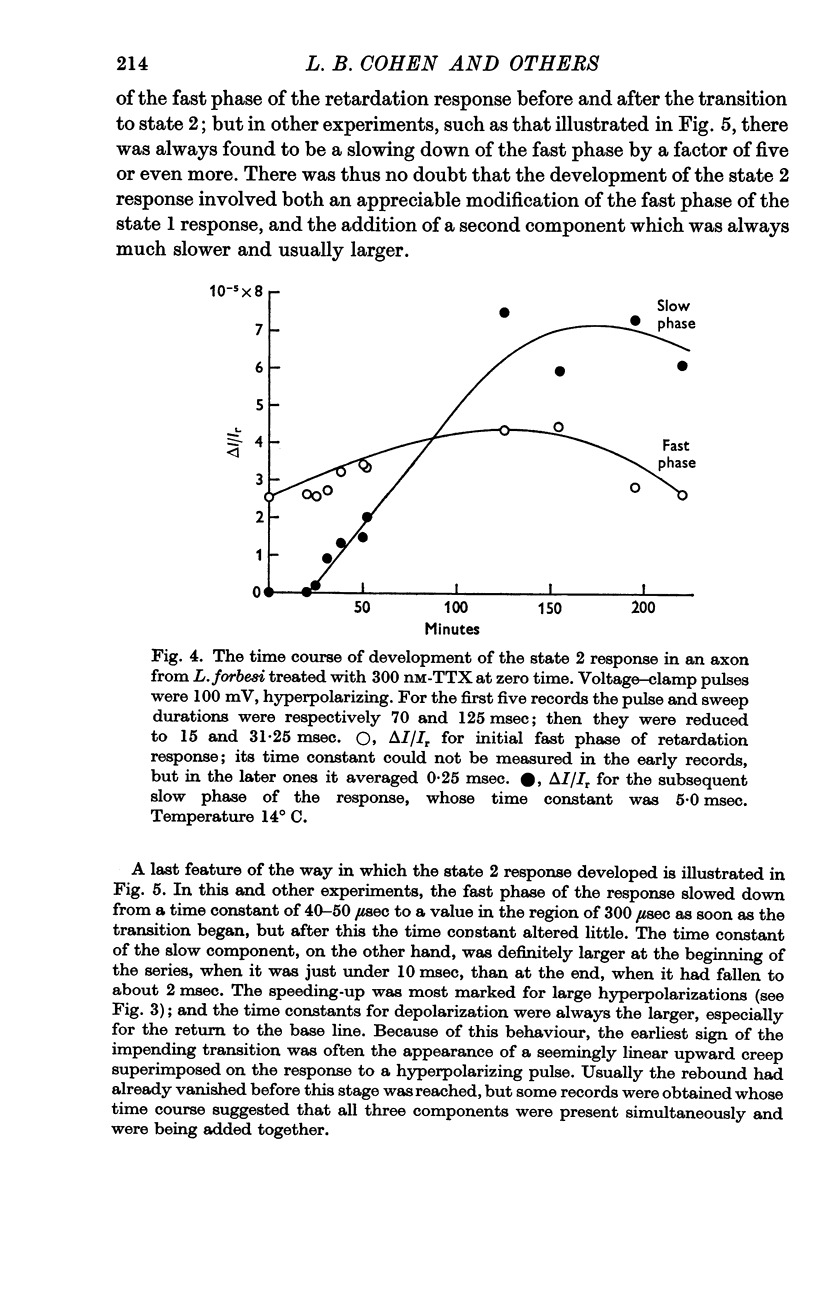
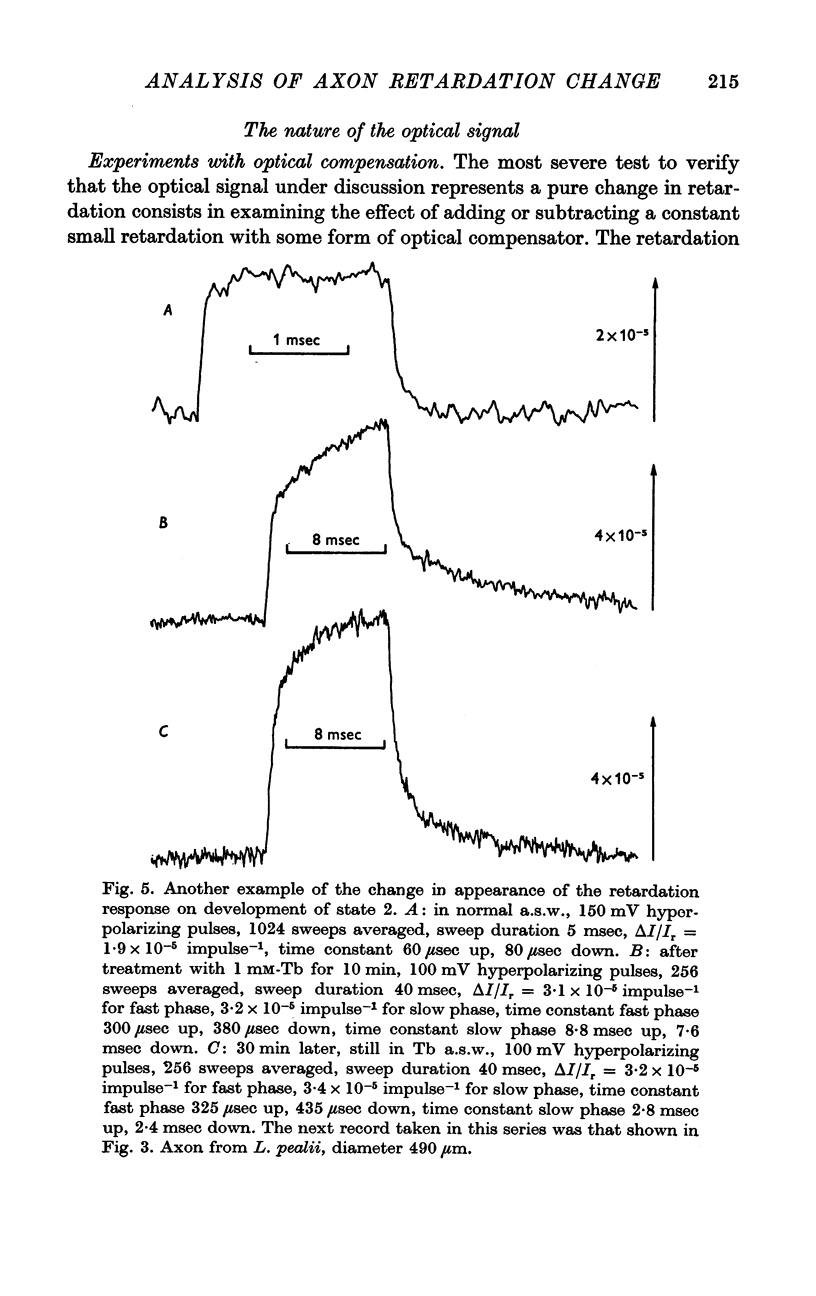
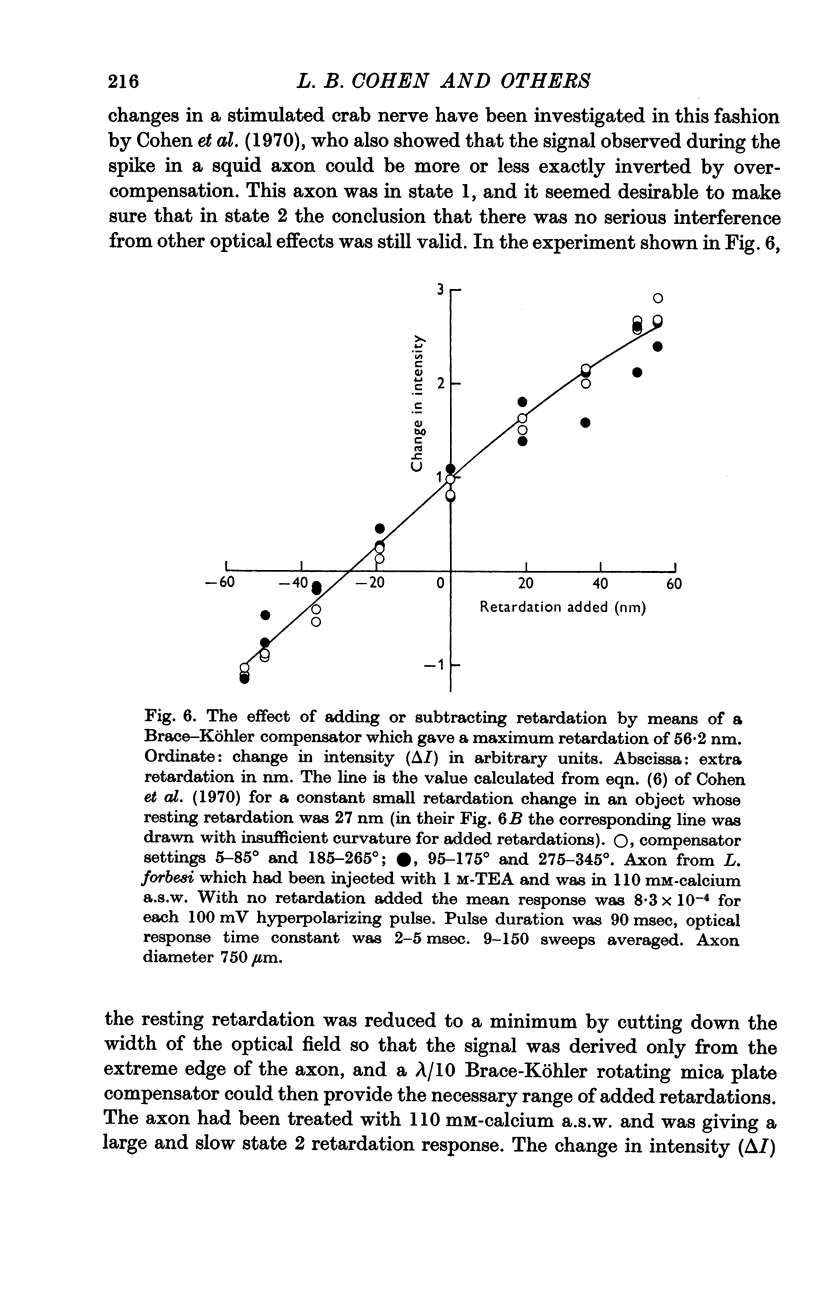
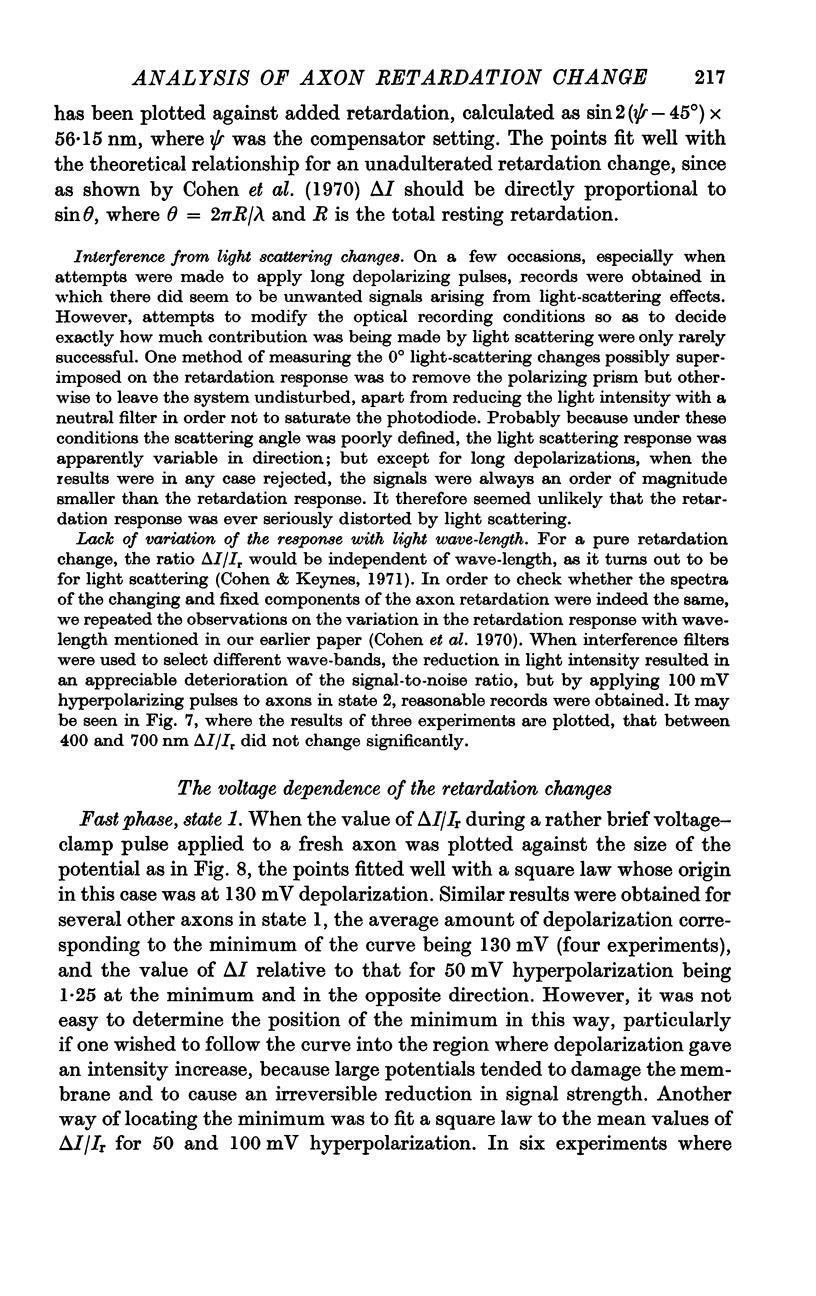
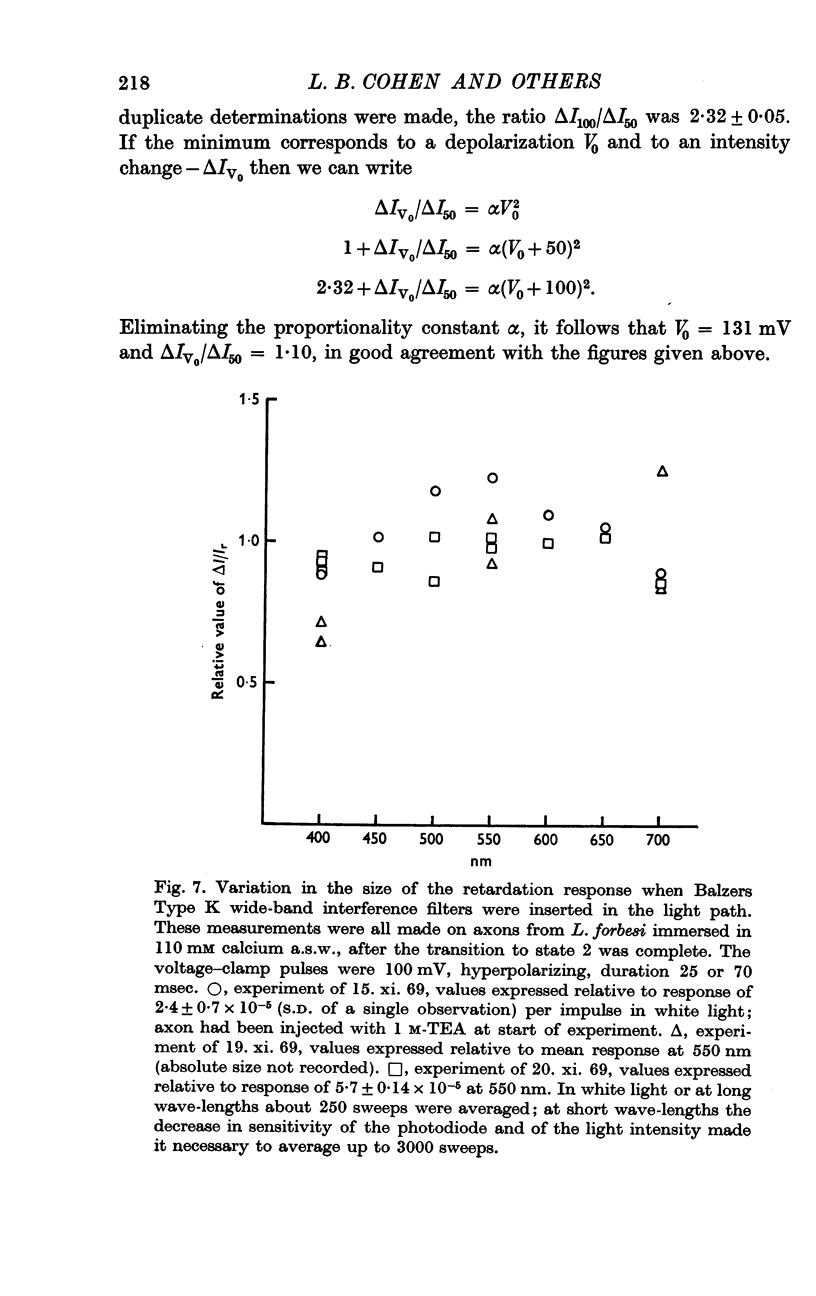
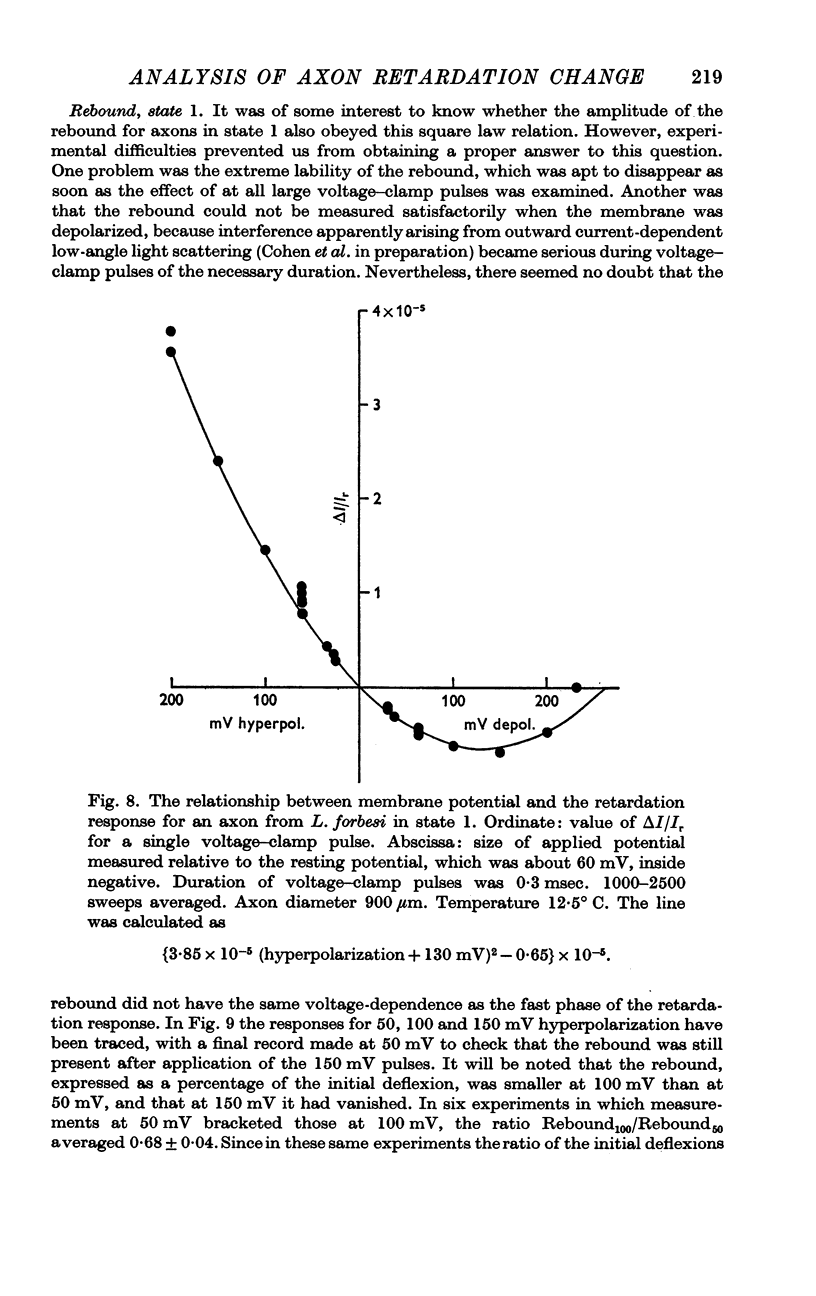
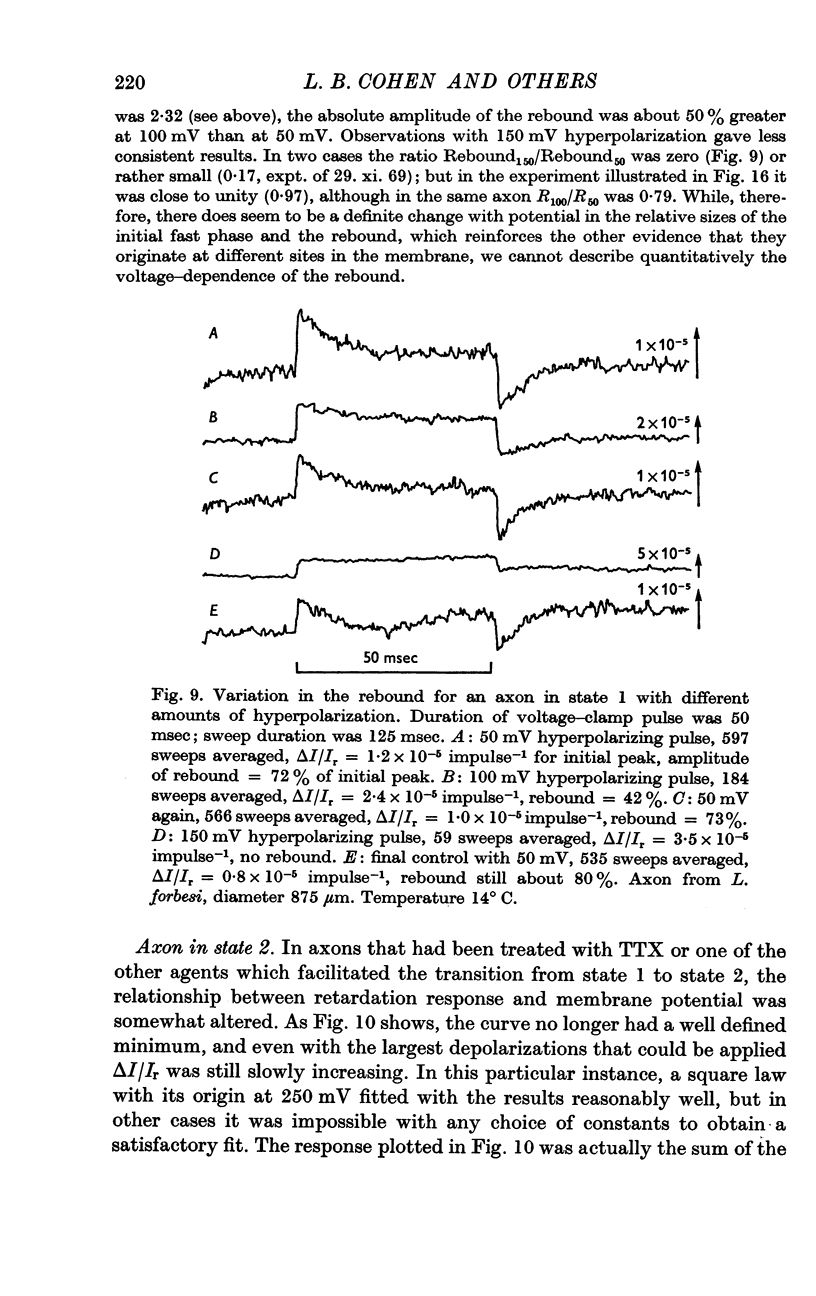
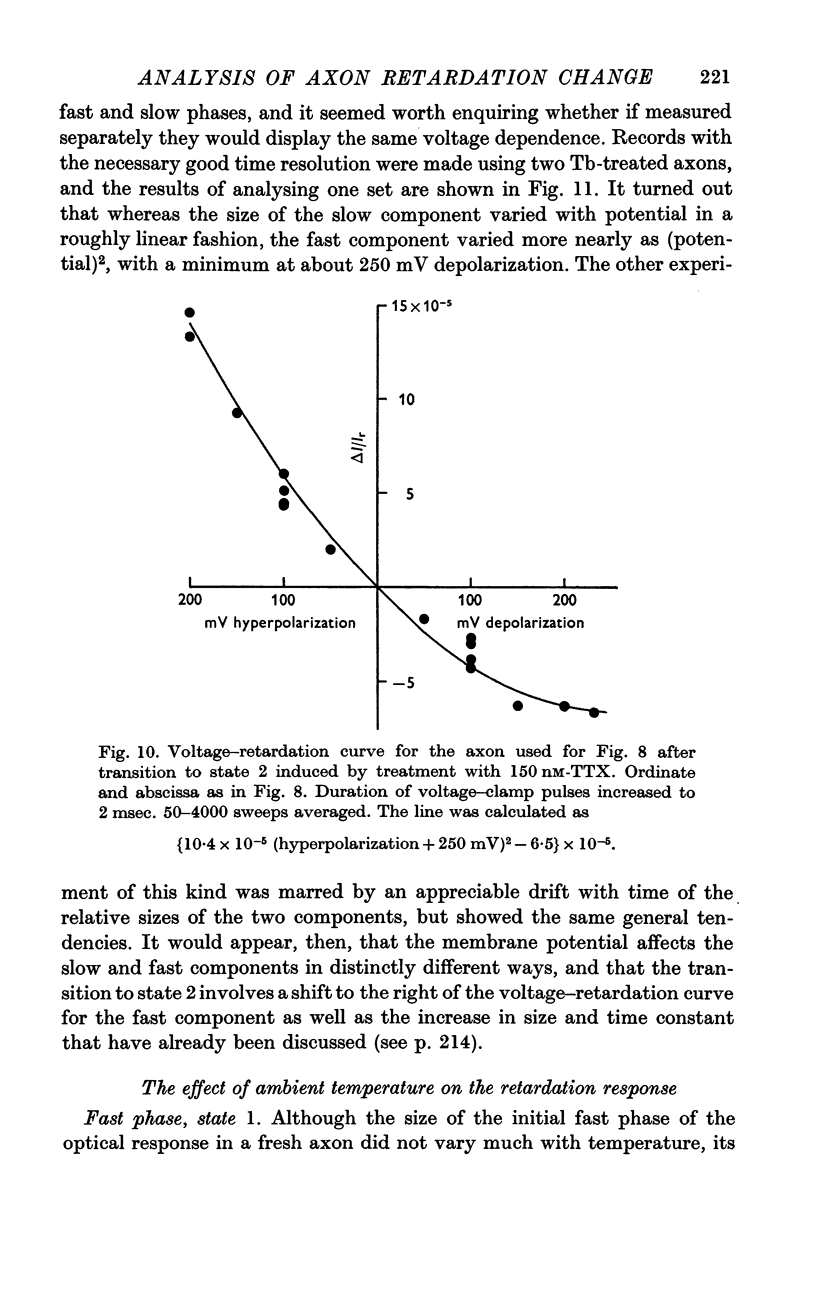
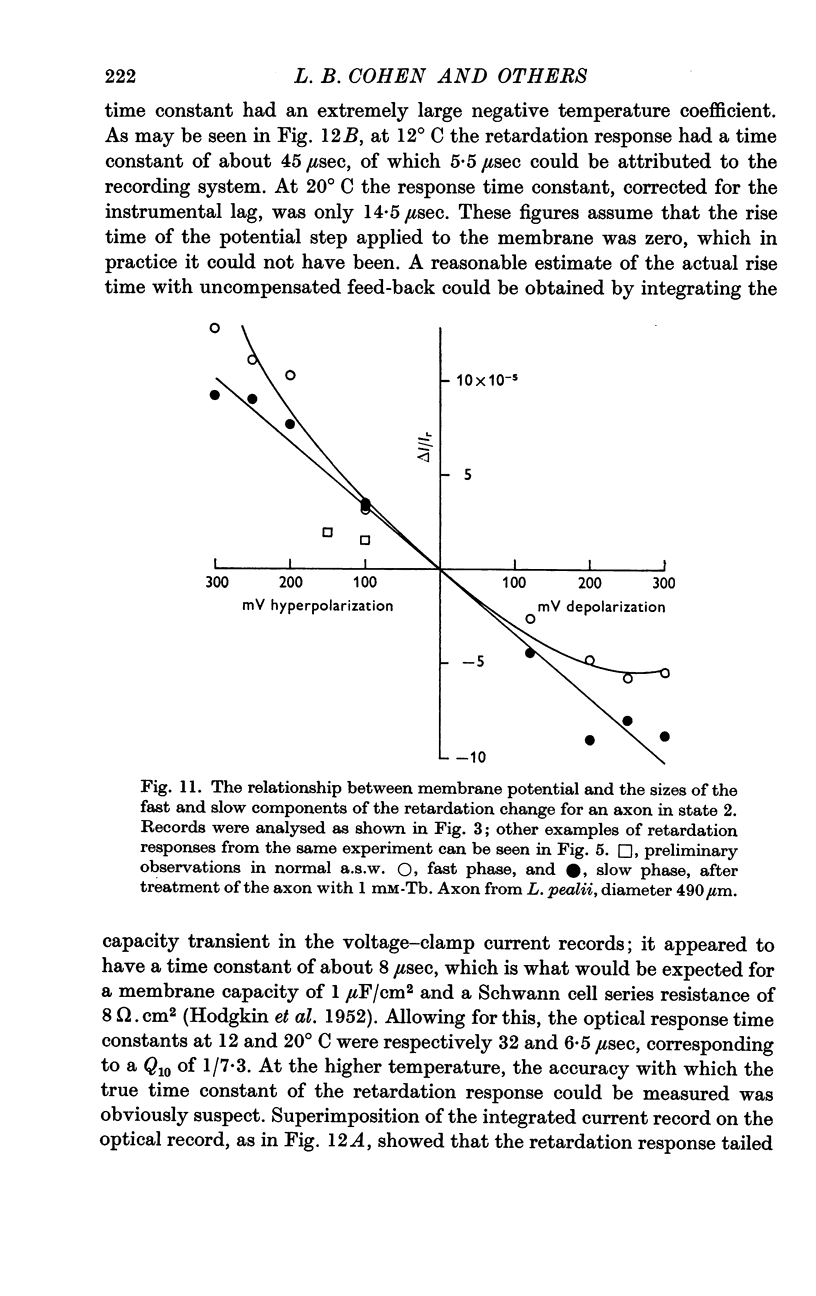
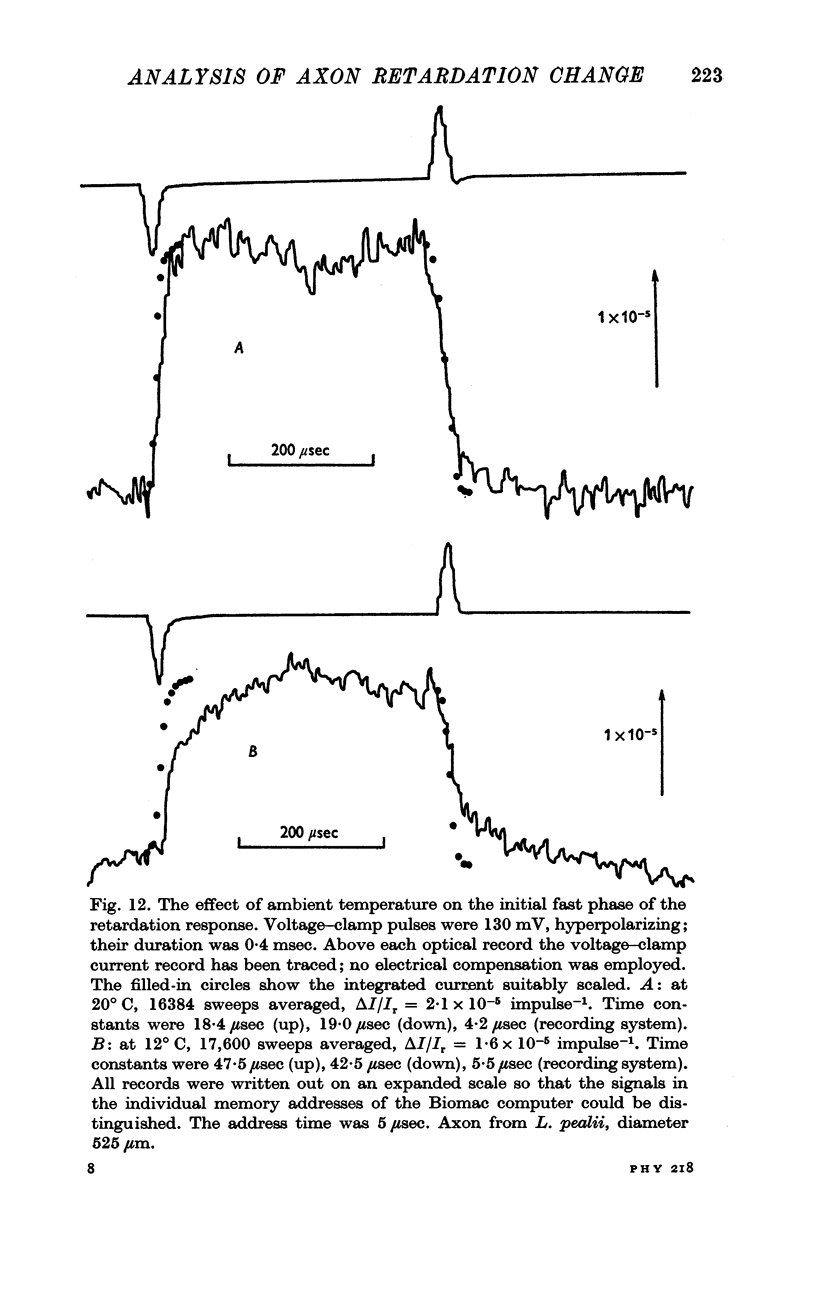
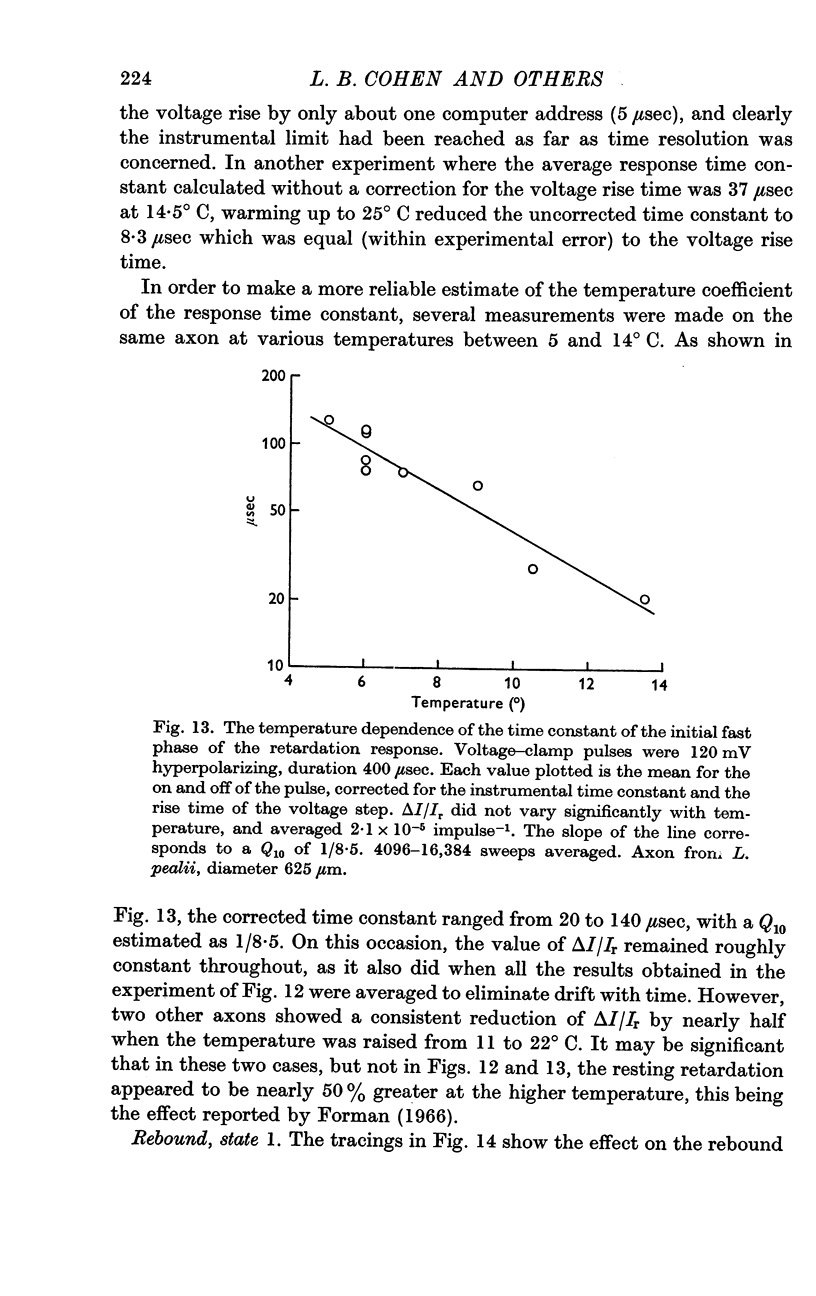
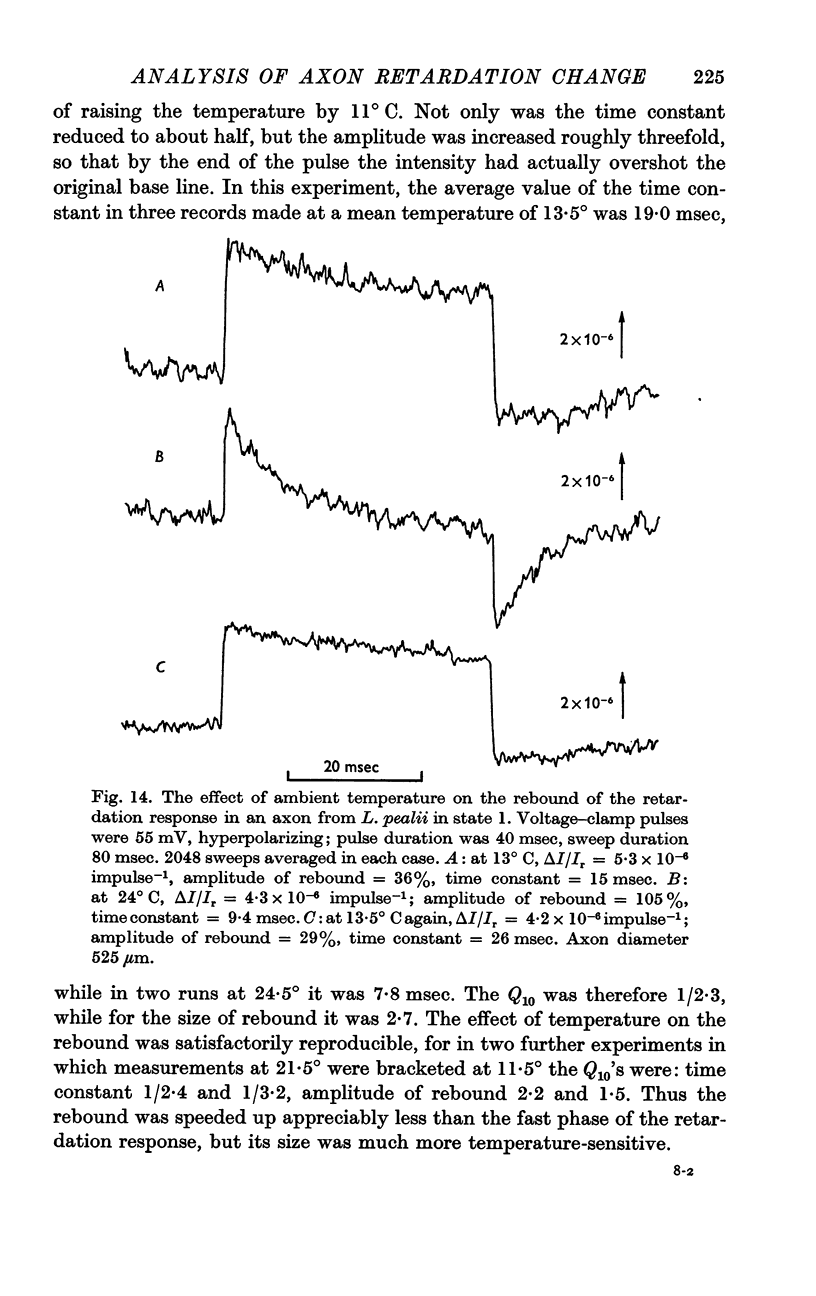
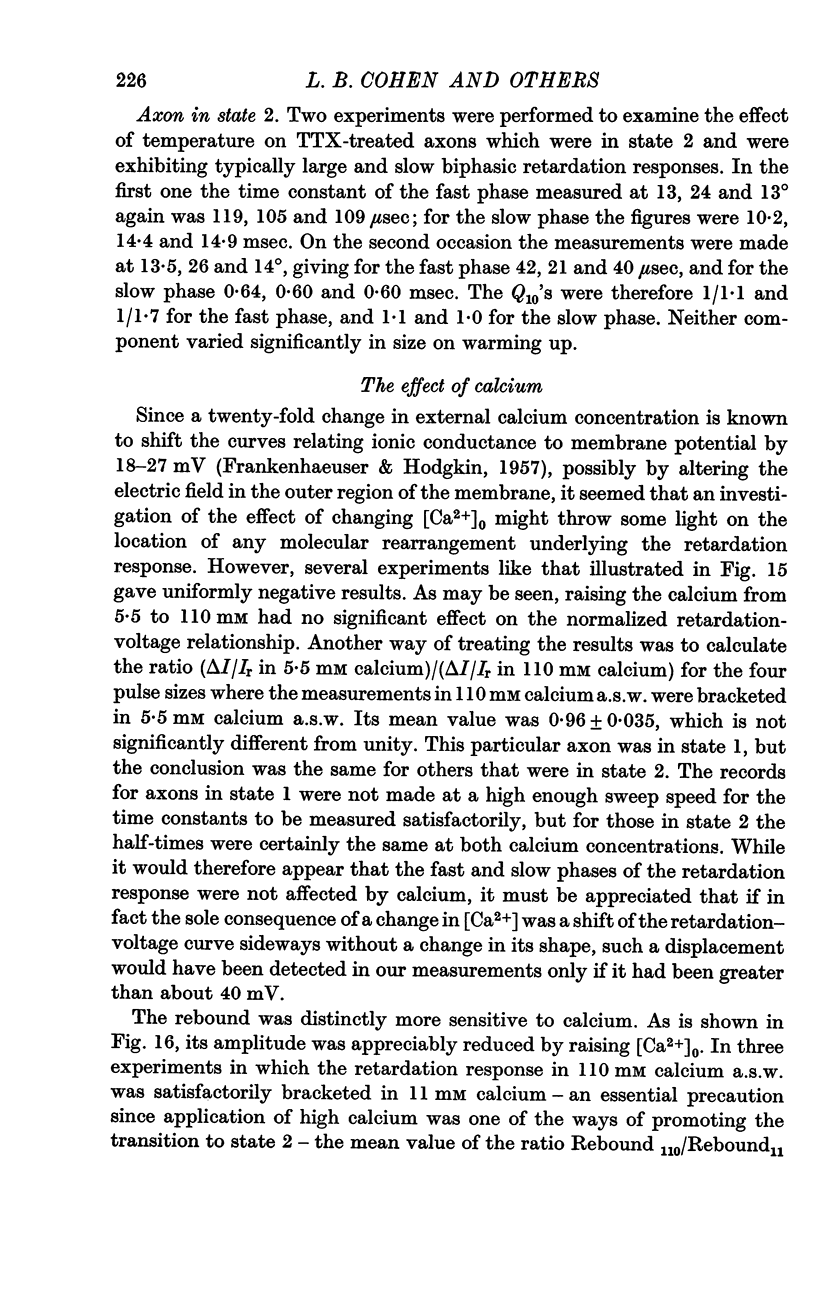
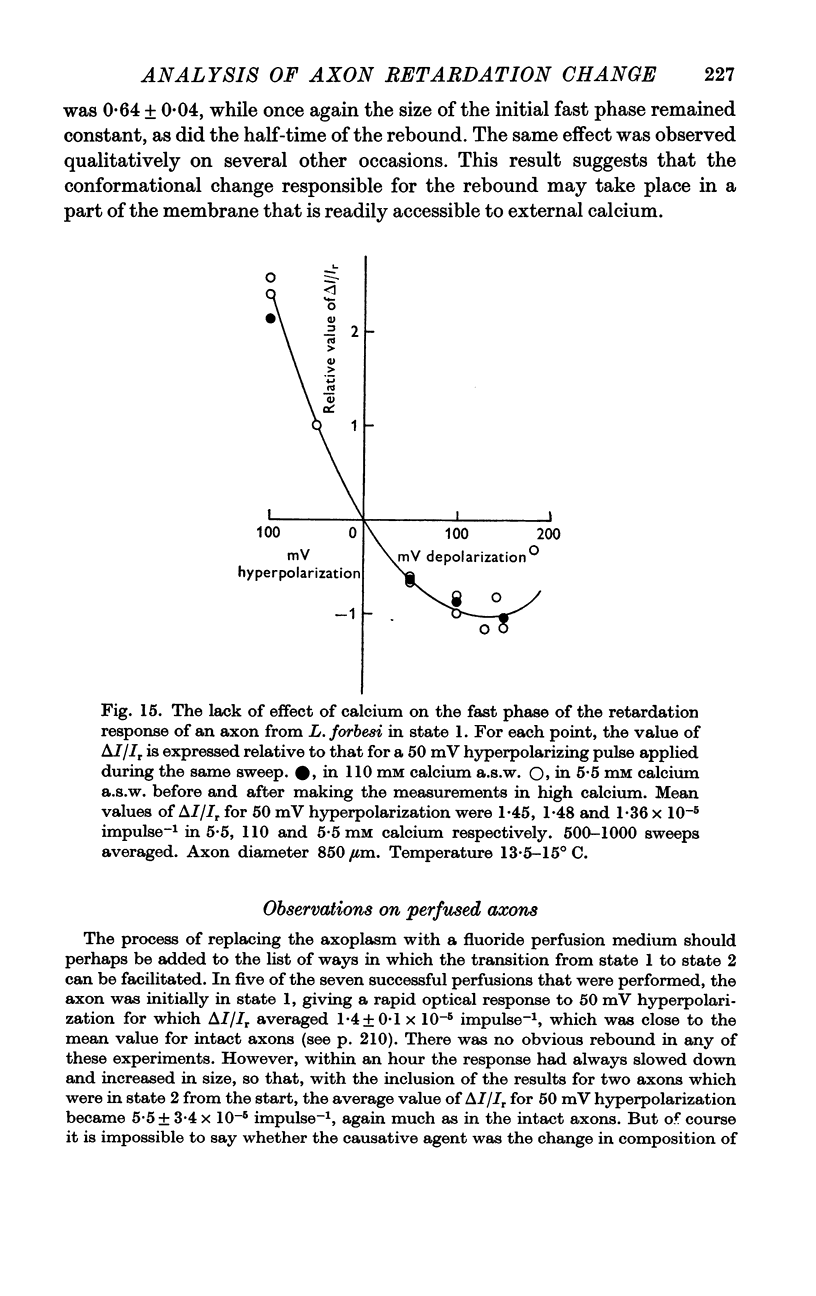
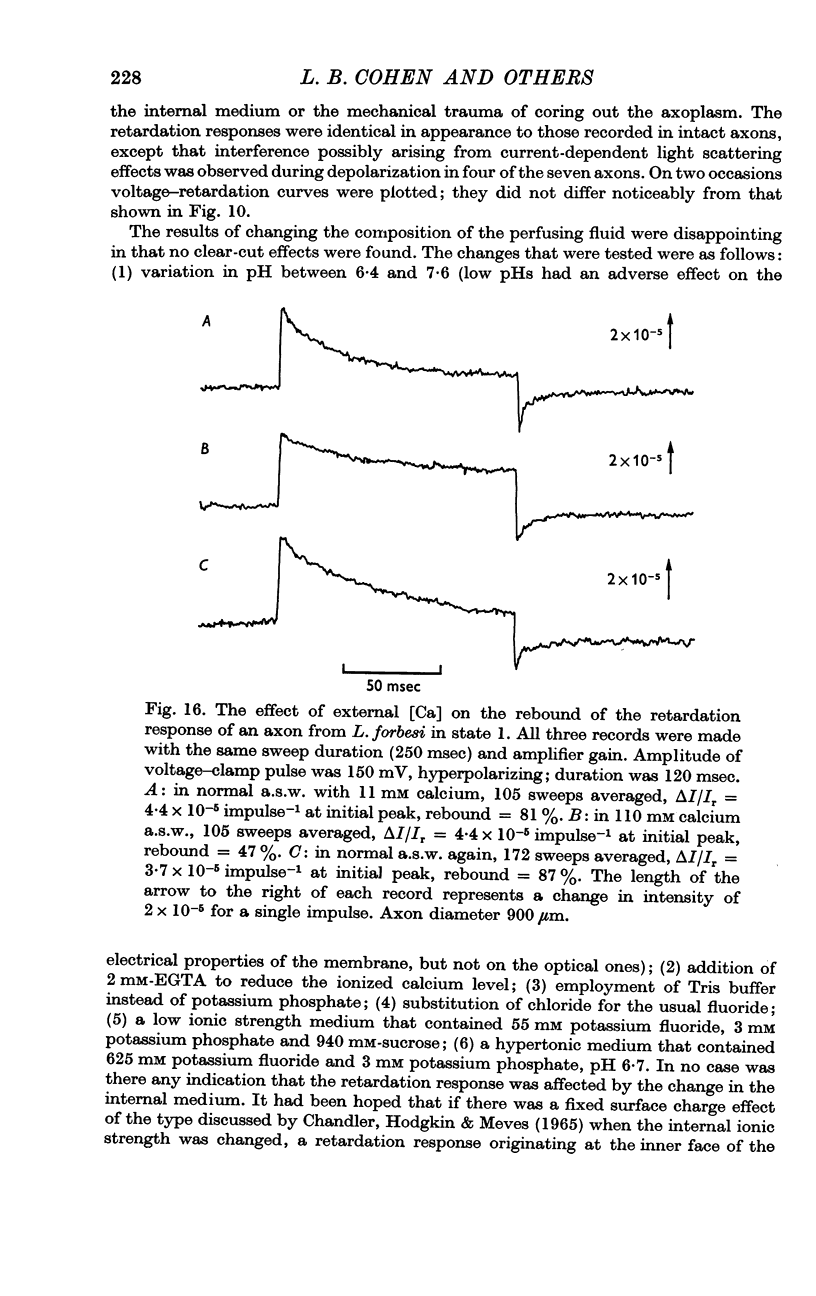
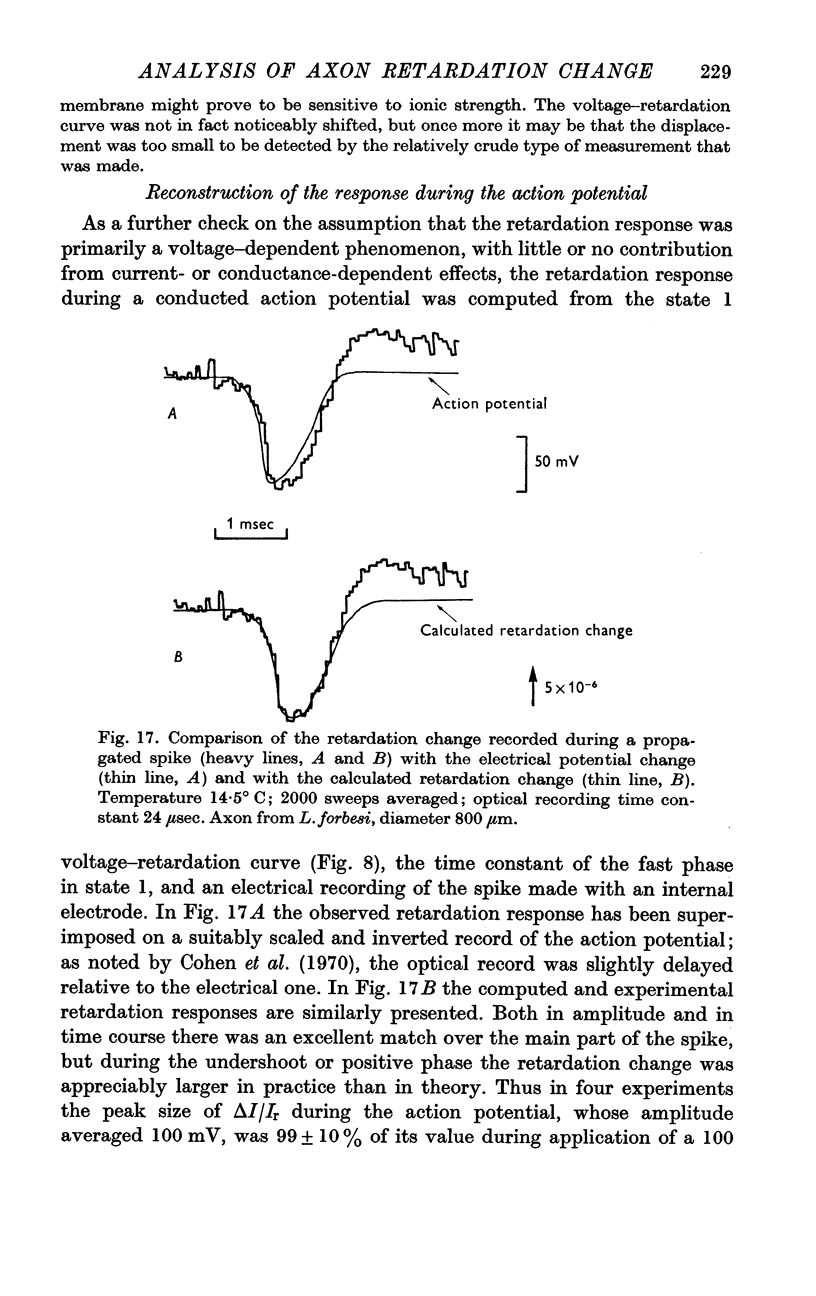
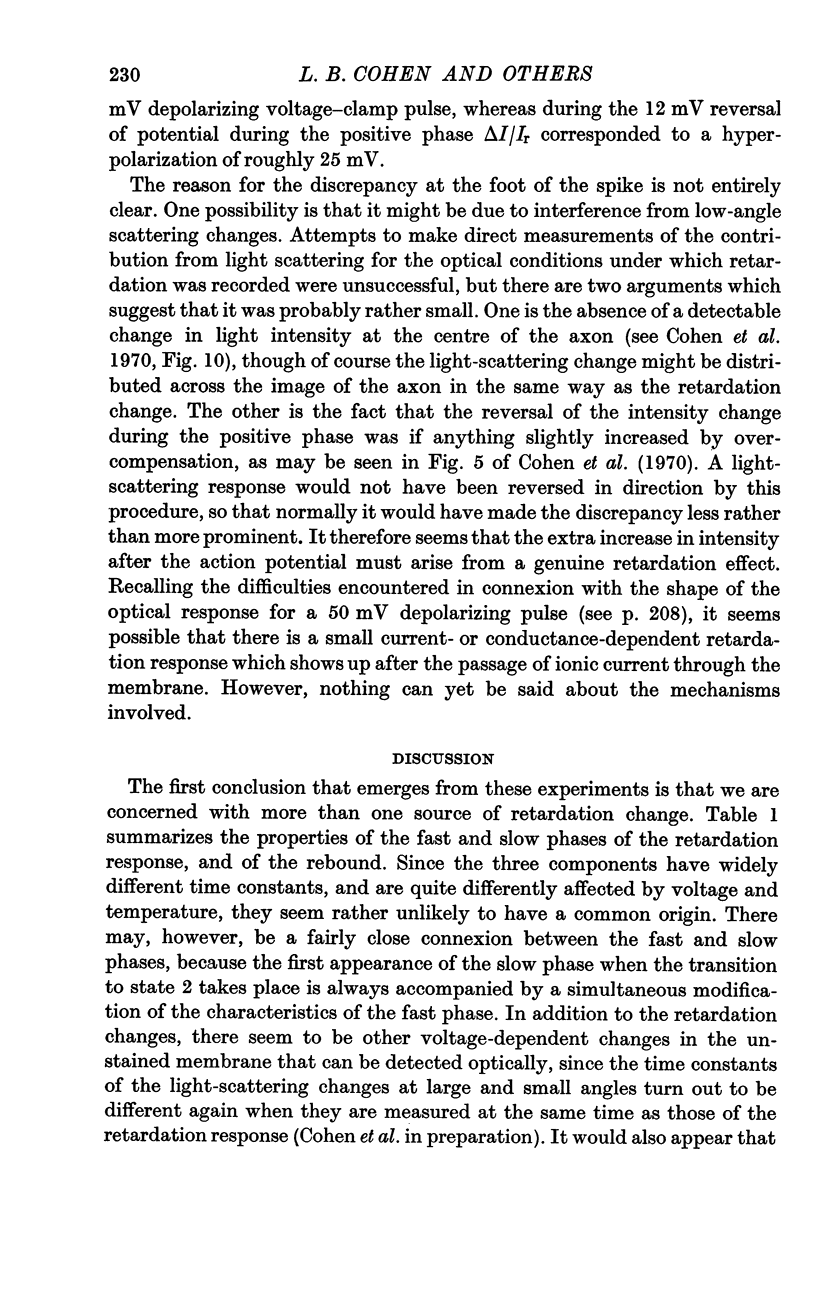
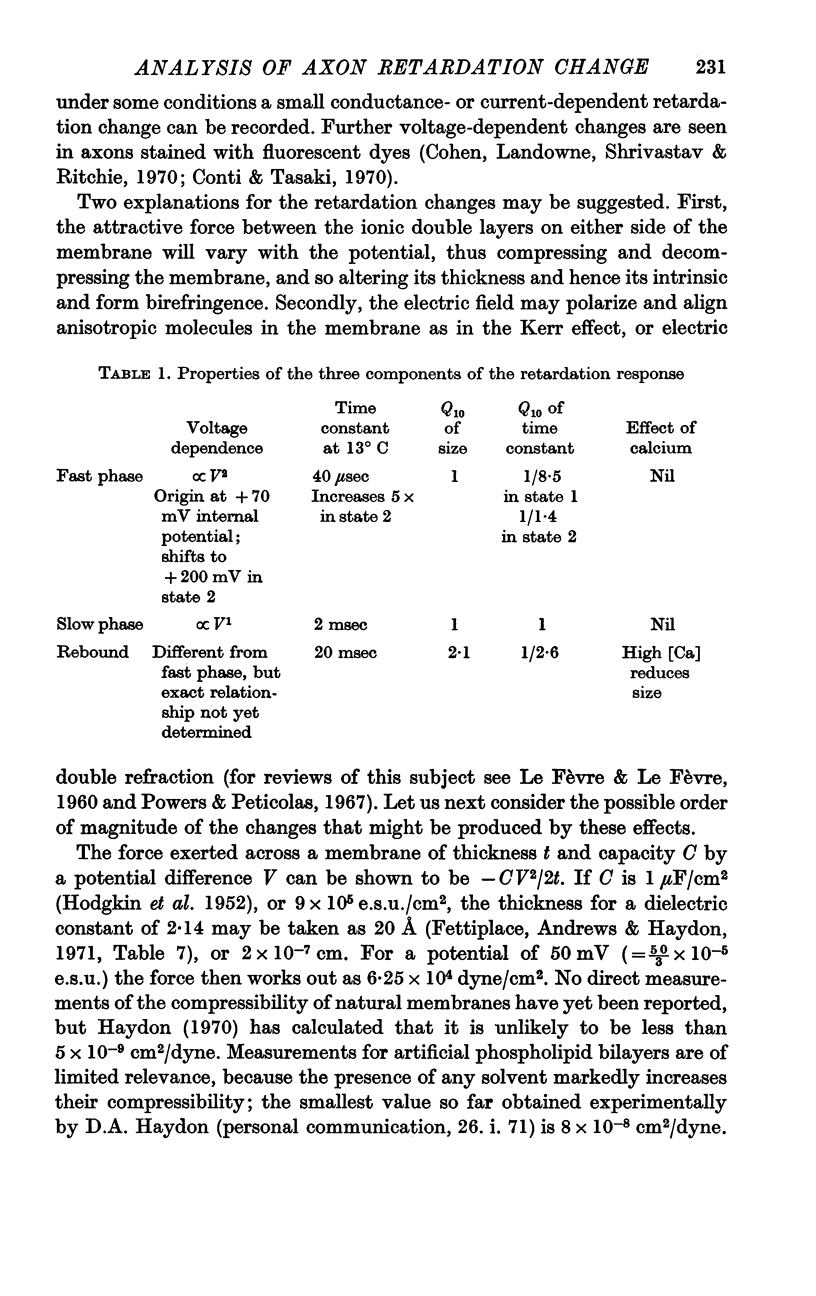
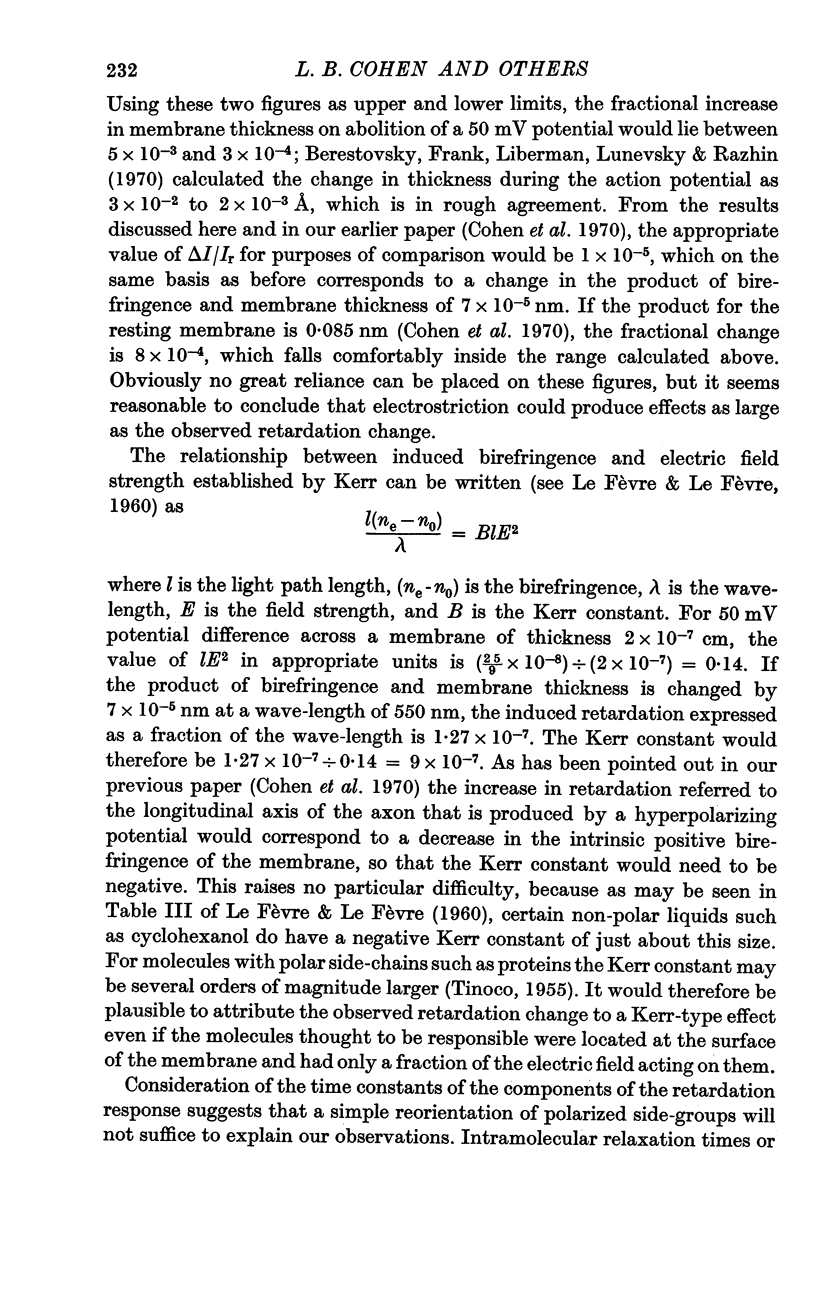
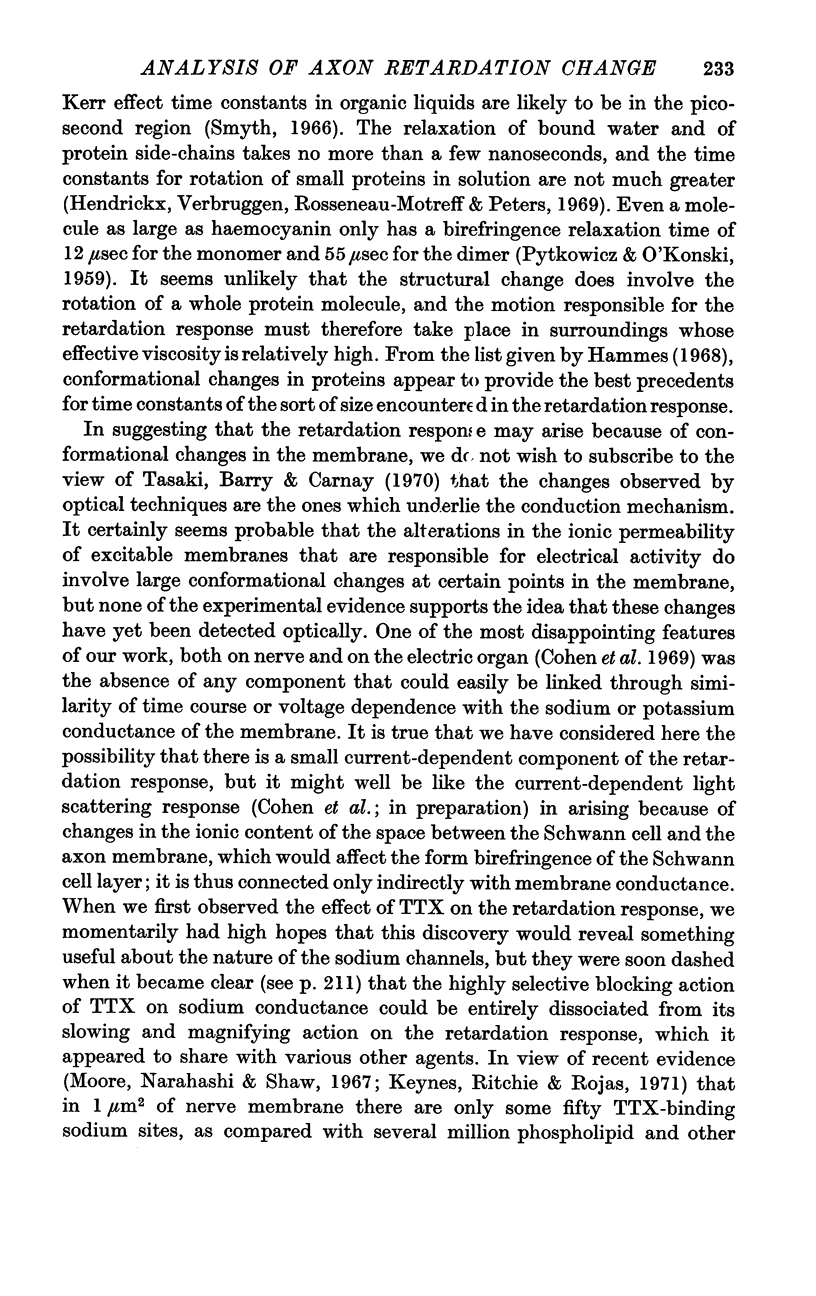
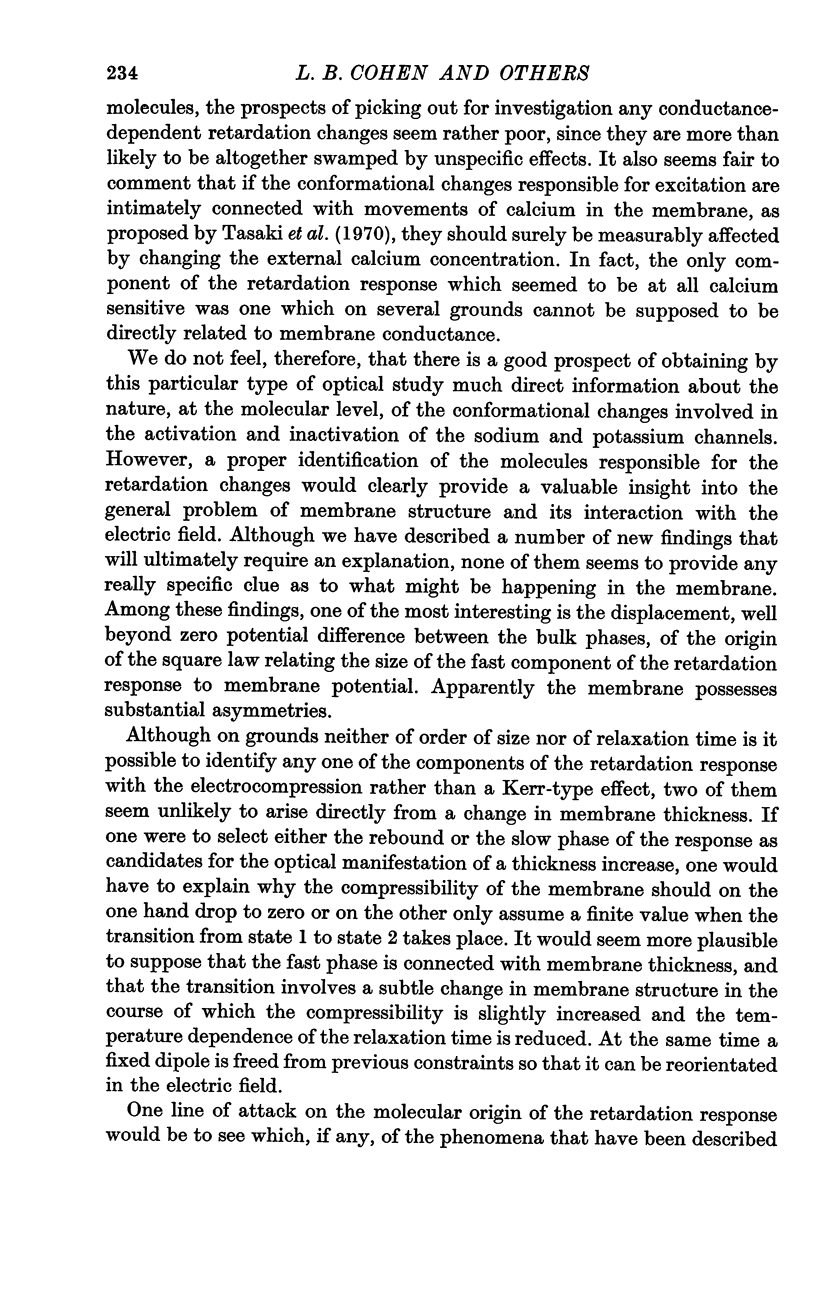
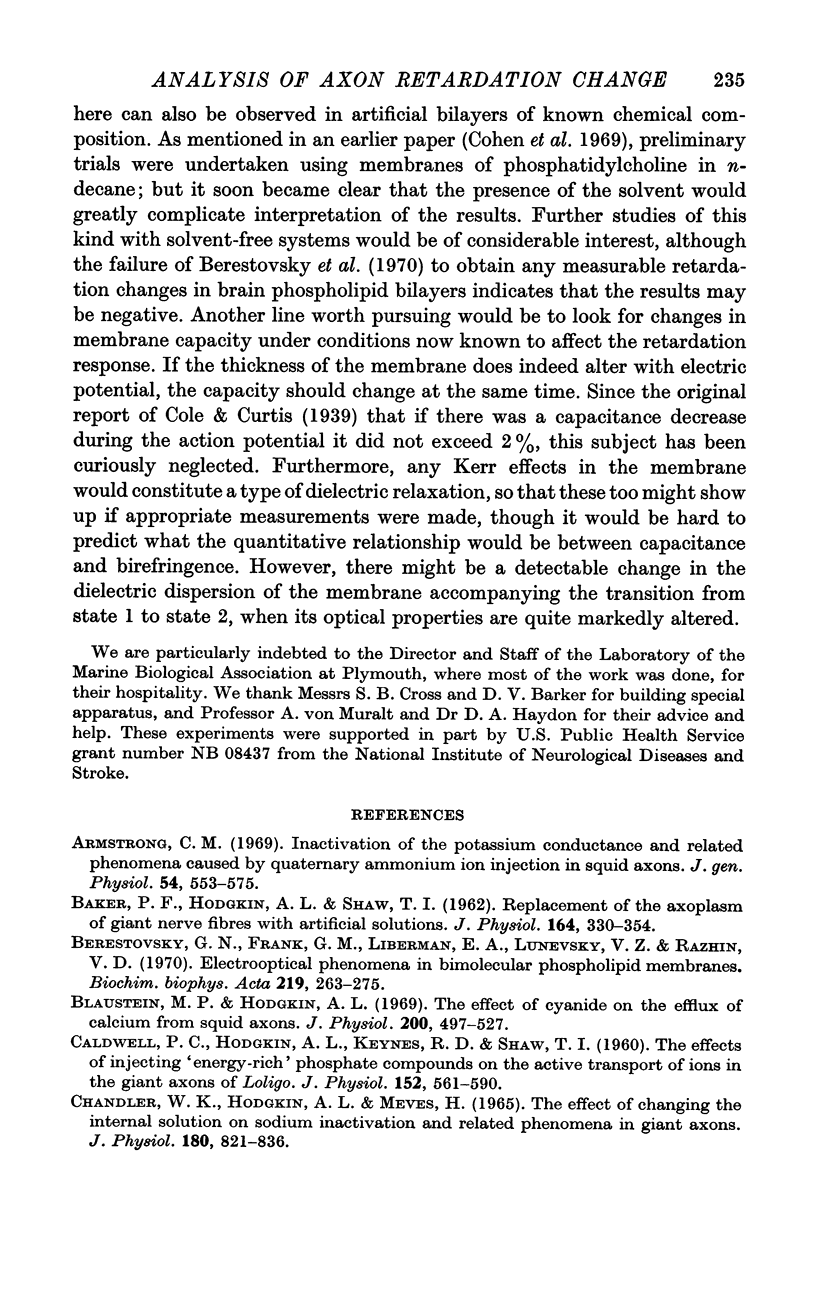
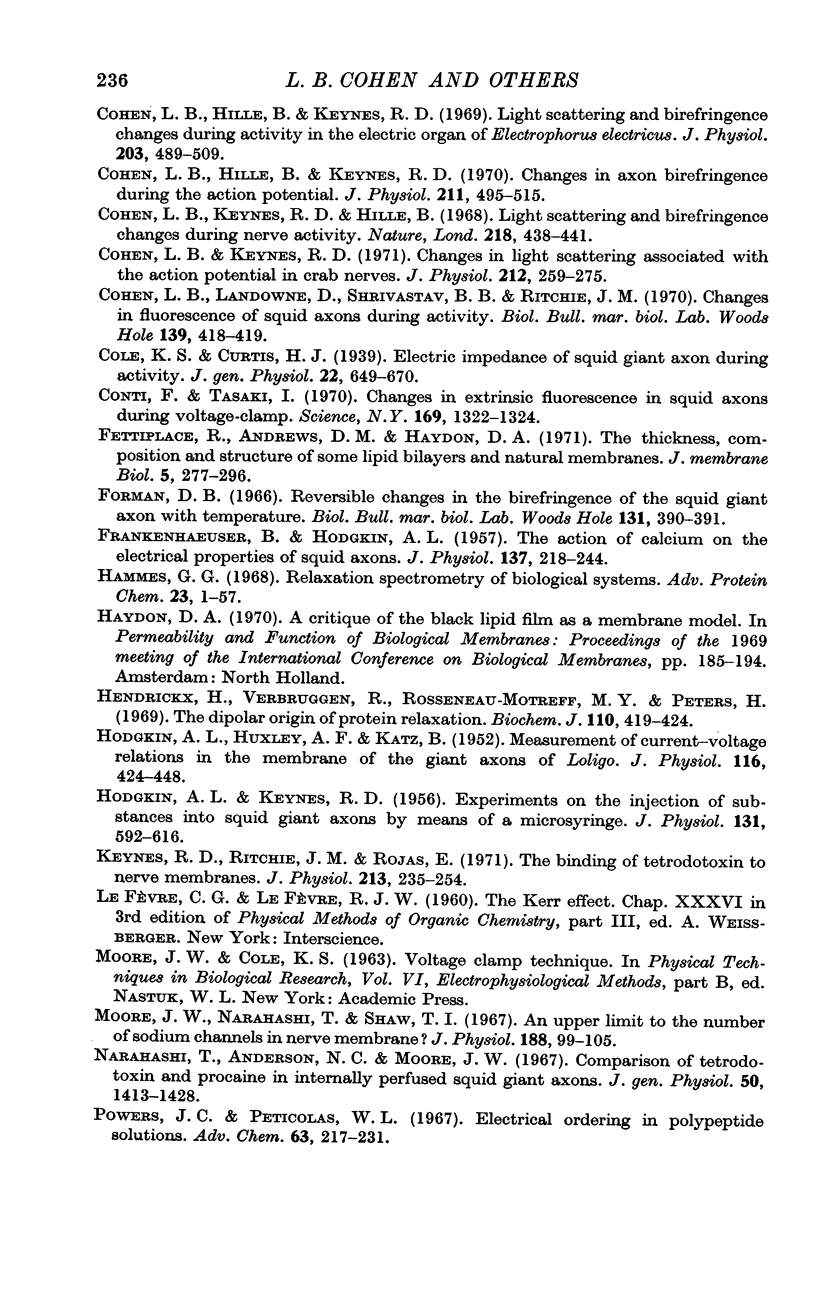
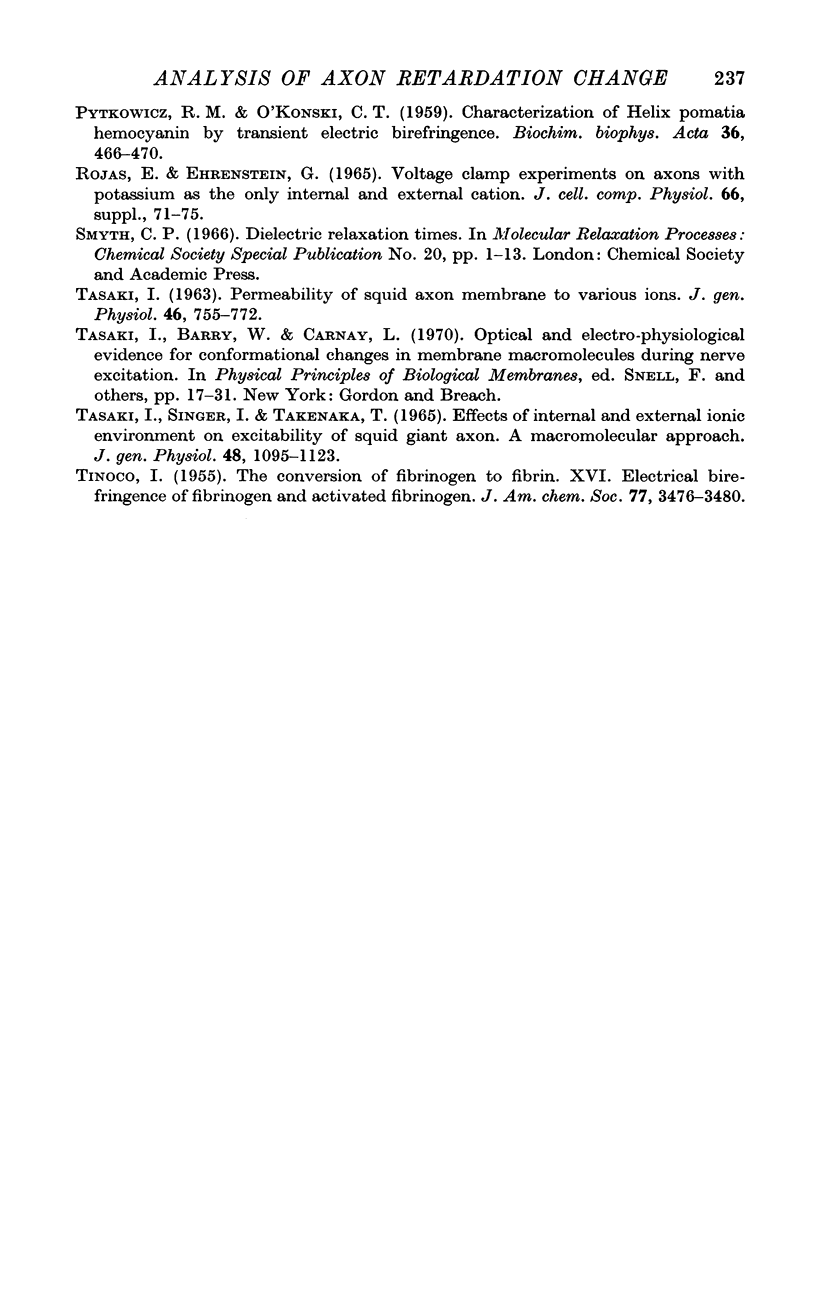
Selected References
These references are in PubMed. This may not be the complete list of references from this article.
- Armstrong C. M. Inactivation of the potassium conductance and related phenomena caused by quaternary ammonium ion injection in squid axons. J Gen Physiol. 1969 Nov;54(5):553–575. doi: 10.1085/jgp.54.5.553. [DOI] [PMC free article] [PubMed] [Google Scholar]
- BAKER P. F., HODGKIN A. L., SHAW T. I. Replacement of the axoplasm of giant nerve fibres with artificial solutions. J Physiol. 1962 Nov;164:330–354. doi: 10.1113/jphysiol.1962.sp007025. [DOI] [PMC free article] [PubMed] [Google Scholar]
- Berestovsky G. N., Frank G. M., Liberman E. A., Lunevsky V. Z., Razhin V. D. Electrooptical phenomena in bimolecular phospholipid membranes. Biochim Biophys Acta. 1970 Dec 1;219(2):263–275. doi: 10.1016/0005-2736(70)90205-1. [DOI] [PubMed] [Google Scholar]
- Blaustein M. P., Hodgkin A. L. The effect of cyanide on the efflux of calcium from squid axons. J Physiol. 1969 Feb;200(2):497–527. doi: 10.1113/jphysiol.1969.sp008704. [DOI] [PMC free article] [PubMed] [Google Scholar]
- CALDWELL P. C., HODGKIN A. L., KEYNES R. D., SHAW T. L. The effects of injecting 'energy-rich' phosphate compounds on the active transport of ions in the giant axons of Loligo. J Physiol. 1960 Jul;152:561–590. doi: 10.1113/jphysiol.1960.sp006509. [DOI] [PMC free article] [PubMed] [Google Scholar]
- Chandler W. K., Hodgkin A. L., Meves H. The effect of changing the internal solution on sodium inactivation and related phenomena in giant axons. J Physiol. 1965 Oct;180(4):821–836. doi: 10.1113/jphysiol.1965.sp007733. [DOI] [PMC free article] [PubMed] [Google Scholar]
- Cohen L. B., Hille B., Keynes R. D. Changes in axon birefringence during the action potential. J Physiol. 1970 Dec;211(2):495–515. doi: 10.1113/jphysiol.1970.sp009289. [DOI] [PMC free article] [PubMed] [Google Scholar]
- Cohen L. B., Hille B., Keynes R. D. Light scattering and birefringence changes during activity in the electric organ of electrophorus electricus. J Physiol. 1969 Aug;203(2):489–509. doi: 10.1113/jphysiol.1969.sp008876. [DOI] [PMC free article] [PubMed] [Google Scholar]
- Cohen L. B., Keynes R. D. Changes in light scattering associated with the action potential in crab nerves. J Physiol. 1971 Jan;212(1):259–275. doi: 10.1113/jphysiol.1971.sp009321. [DOI] [PMC free article] [PubMed] [Google Scholar]
- Cohen L. B., Keynes R. D., Hille B. Light scattering and birefringence changes during nerve activity. Nature. 1968 May 4;218(5140):438–441. doi: 10.1038/218438a0. [DOI] [PubMed] [Google Scholar]
- Cole K. S., Curtis H. J. ELECTRIC IMPEDANCE OF THE SQUID GIANT AXON DURING ACTIVITY. J Gen Physiol. 1939 May 20;22(5):649–670. doi: 10.1085/jgp.22.5.649. [DOI] [PMC free article] [PubMed] [Google Scholar]
- Conti F., Tasaki I. Changes in extrinsic fluorescence in squid axons during voltage-clamp. Science. 1970 Sep 25;169(3952):1322–1324. doi: 10.1126/science.169.3952.1322. [DOI] [PubMed] [Google Scholar]
- FRANKENHAEUSER B., HODGKIN A. L. The action of calcium on the electrical properties of squid axons. J Physiol. 1957 Jul 11;137(2):218–244. doi: 10.1113/jphysiol.1957.sp005808. [DOI] [PMC free article] [PubMed] [Google Scholar]
- HODGKIN A. L., HUXLEY A. F., KATZ B. Measurement of current-voltage relations in the membrane of the giant axon of Loligo. J Physiol. 1952 Apr;116(4):424–448. doi: 10.1113/jphysiol.1952.sp004716. [DOI] [PMC free article] [PubMed] [Google Scholar]
- HODGKIN A. L., KEYNES R. D. Experiments on the injection of substances into squid giant axons by means of a microsyringe. J Physiol. 1956 Mar 28;131(3):592–616. doi: 10.1113/jphysiol.1956.sp005485. [DOI] [PMC free article] [PubMed] [Google Scholar]
- Hammes G. G. Relaxation spectrometry of biological systems. Adv Protein Chem. 1968;23:1–57. doi: 10.1016/s0065-3233(08)60399-x. [DOI] [PubMed] [Google Scholar]
- Hendrickx H., Verbruggen R., Rosseneu-Motreff M. Y., Blaton V., Peeters H. The dipolar origin of protein relaxation. Biochem J. 1968 Dec;110(3):419–424. doi: 10.1042/bj1100419. [DOI] [PMC free article] [PubMed] [Google Scholar]
- Keynes R. D., Ritchie J. M., Rojas E. The binding of tetrodotoxin to nerve membranes. J Physiol. 1971 Feb;213(1):235–254. doi: 10.1113/jphysiol.1971.sp009379. [DOI] [PMC free article] [PubMed] [Google Scholar]
- Moore J. W., Narahashi T., Shaw T. I. An upper limit to the number of sodium channels in nerve membrane? J Physiol. 1967 Jan;188(1):99–105. doi: 10.1113/jphysiol.1967.sp008126. [DOI] [PMC free article] [PubMed] [Google Scholar]
- Narahashi T., Anderson N. C., Moore J. W. Comparison of tetrodotoxin and procaine in internally perfused squid giant axons. J Gen Physiol. 1967 May;50(5):1413–1428. doi: 10.1085/jgp.50.5.1413. [DOI] [PMC free article] [PubMed] [Google Scholar]
- PYTKOWICZ R. M., O'KONSKI C. T. Characterization of Helix Pomatia hemocyanin by transient electric birefringence. Biochim Biophys Acta. 1959 Dec;36:466–470. doi: 10.1016/0006-3002(59)90187-8. [DOI] [PubMed] [Google Scholar]
- TASAKI I. Permeability of squid axon membrane to various ions. J Gen Physiol. 1963 Mar;46:755–772. doi: 10.1085/jgp.46.4.755. [DOI] [PMC free article] [PubMed] [Google Scholar]
- Tasaki I., Singer I., Takenaka T. Effects of internal and external ionic environment on excitability of squid giant axon. A macromolecular approach. J Gen Physiol. 1965 Jul;48(6):1095–1123. doi: 10.1085/jgp.48.6.1095. [DOI] [PMC free article] [PubMed] [Google Scholar]