Abstract
1. The permeability of human red cells to 36Cl- and to [35S]SO42- was studied in the presence of various monovalent anions.
2. A maximum decrease of anion permeability was found in a study of the steady-state exchange of 36Cl in a medium containing 120 mM salicylate. The exchange had a half-time of 3 hr at 0° C, a reduction of normal chloride permeability by a factor of 105. The activation energy of chloride exchange decreased from a value of 45 to 22 kcal/mole in the interval between 0 and 10° C. Simultaneous determination of the permeability to potassium and chloride proved that salicylate induced a reversal of the normal selectivity of red cells at 0° C (permeability coefficient PK of 3·5 × 10-9 cm/sec to be compared with a PCl of 2 × 10-9 cm/sec).
3. In contradistinction to the slow movement of 36Cl, the exchange of [14C]salicylate was completed within 4 min, when red cells were suspended at 0° C in the salicylate medium.
4. A study of the sulphate permeability at 38° C showed that the rate of steady-state exchange decreased, when chloride was replaced by lyotropic anions other than bromide. The sequence of the permeability decrease was: Cl- = Br- < I- < NO3 < SCN- < salicylate, the same sequence which previously has been shown to increase the permeability to sodium and potassium. The activation energies of sulphate exchange were 32 kcal/mole (chloride medium), and 38 kcal/mole (thiocyanate medium).
5. Sufficient data were obtained during the study to demonstrate that when equilibrium has been obtained, there is a good agreement between the values of 36Cl (cell water)/36Cl (extracellular water) and of {[35S]SO4 (cell water)/[35S]SO4 (extracellular water)}½.
6. It is concluded that the anion-induced changes of permeability are due to binding of anions to fixed cationic charges in the red cell membrane.
Full text
PDF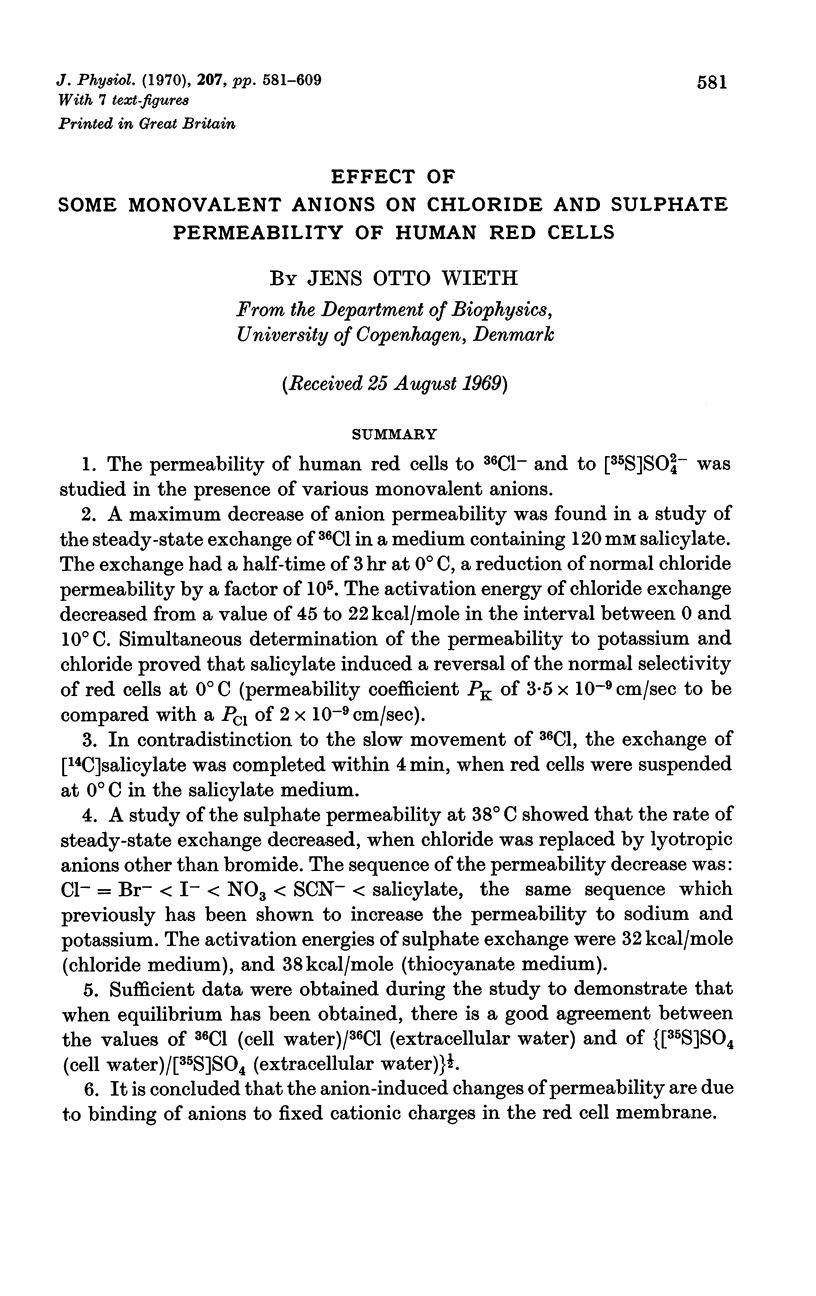
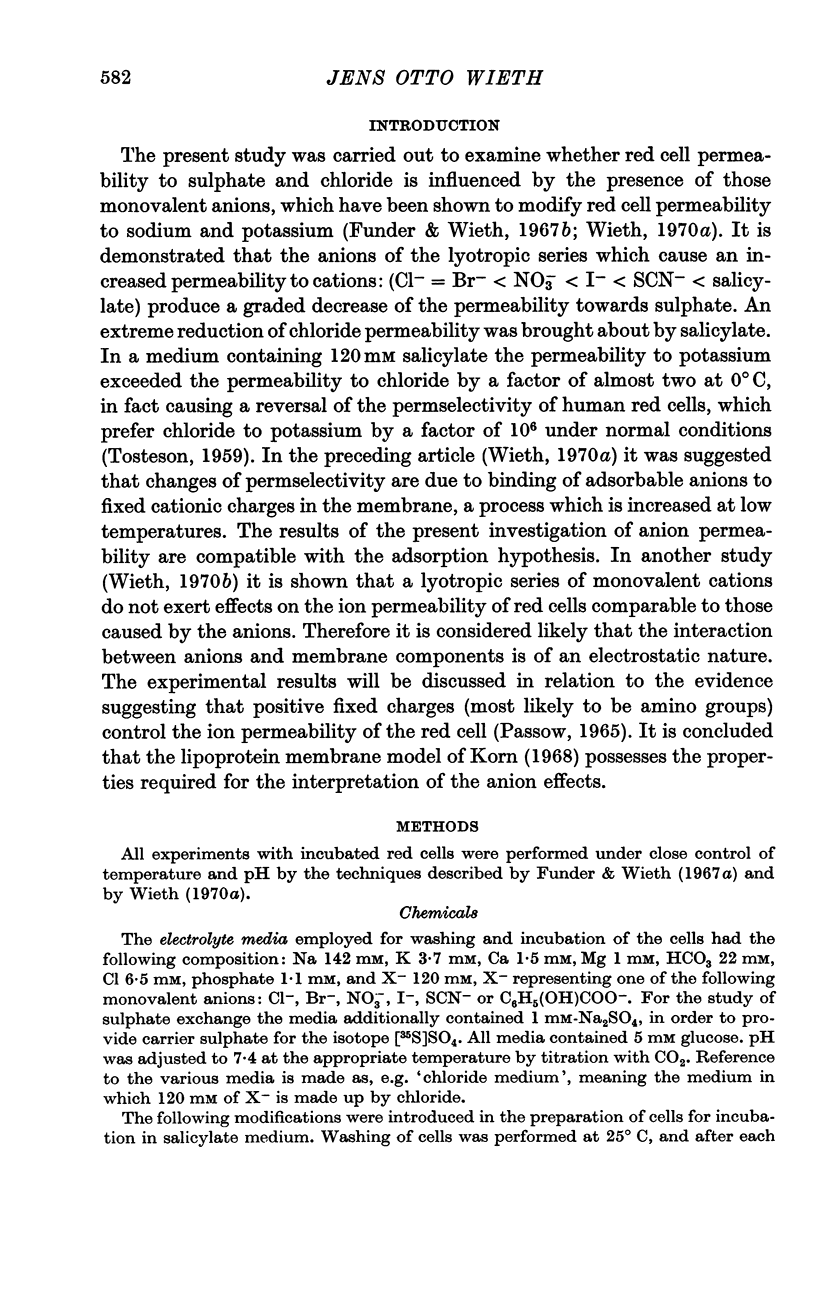
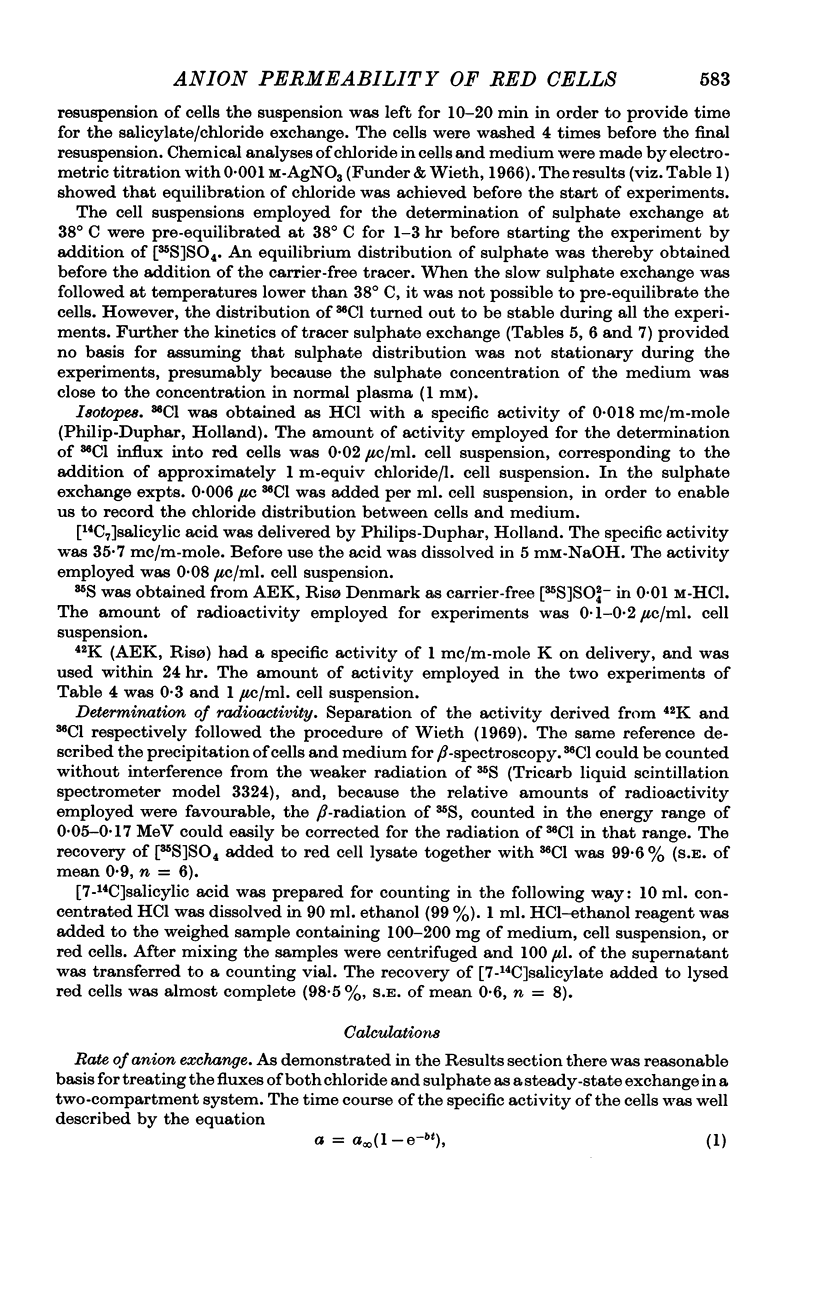
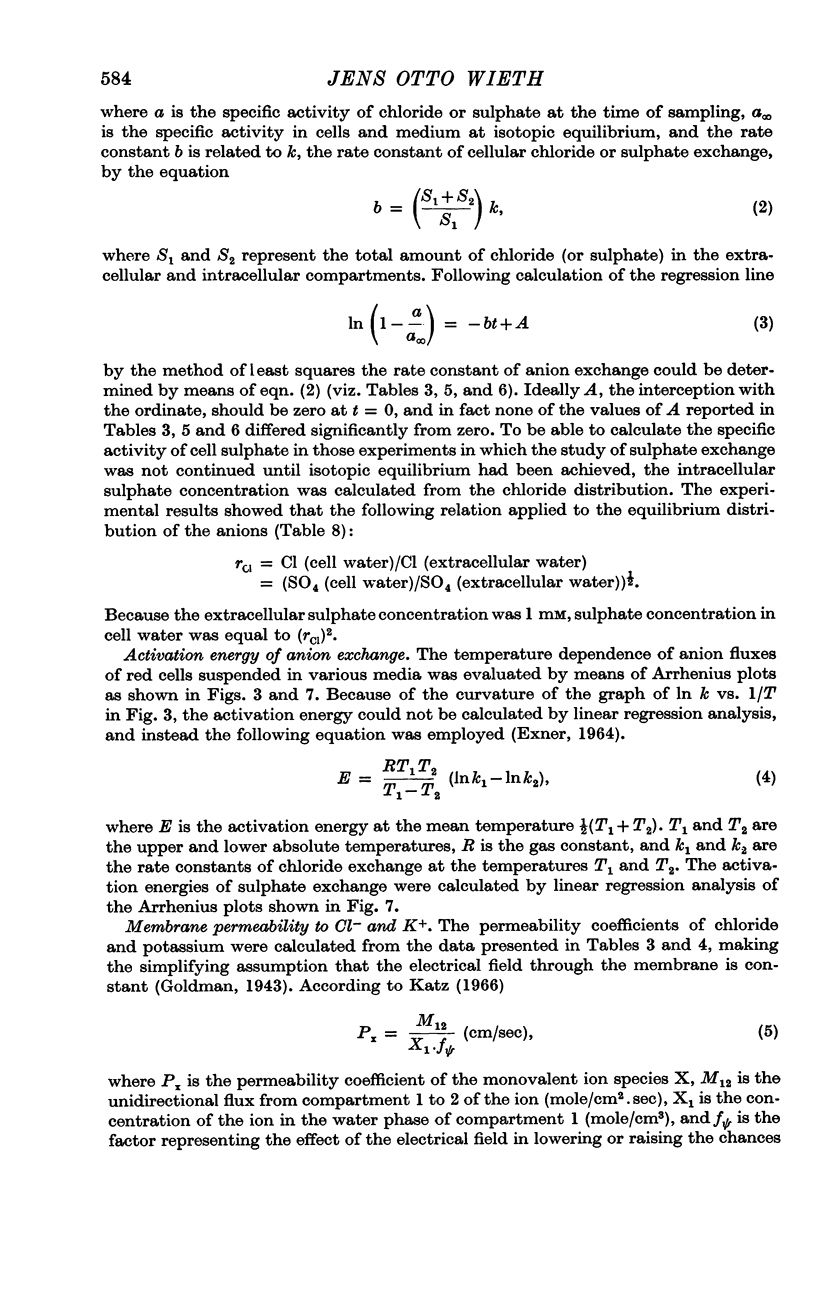
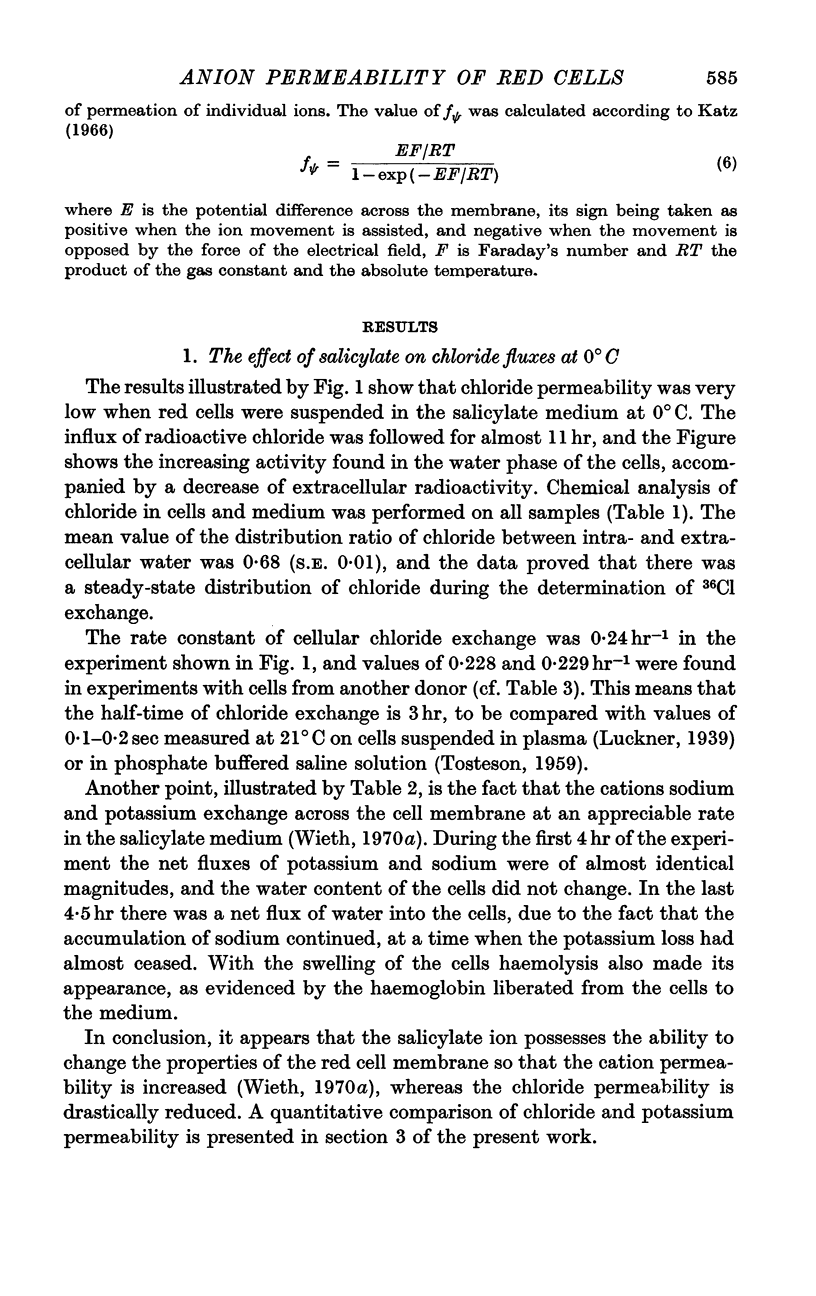
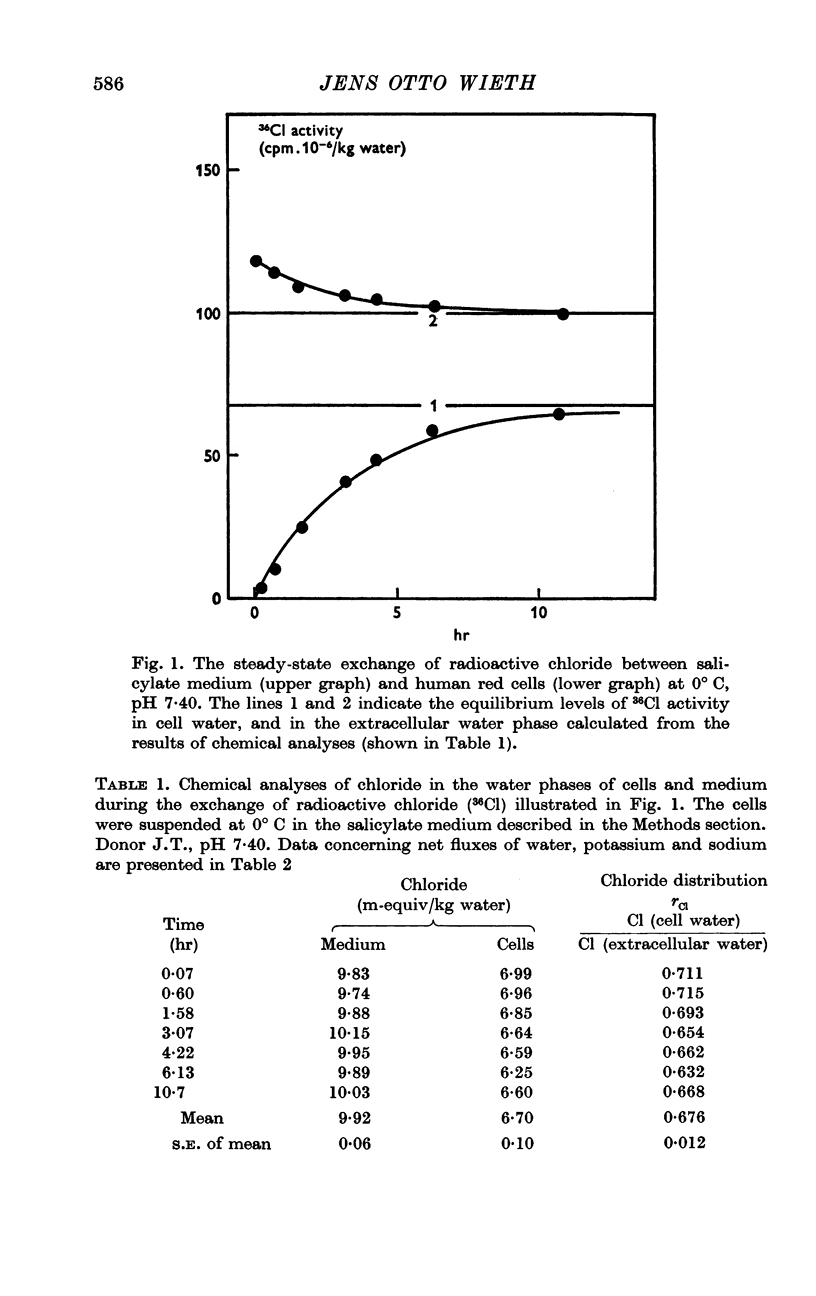
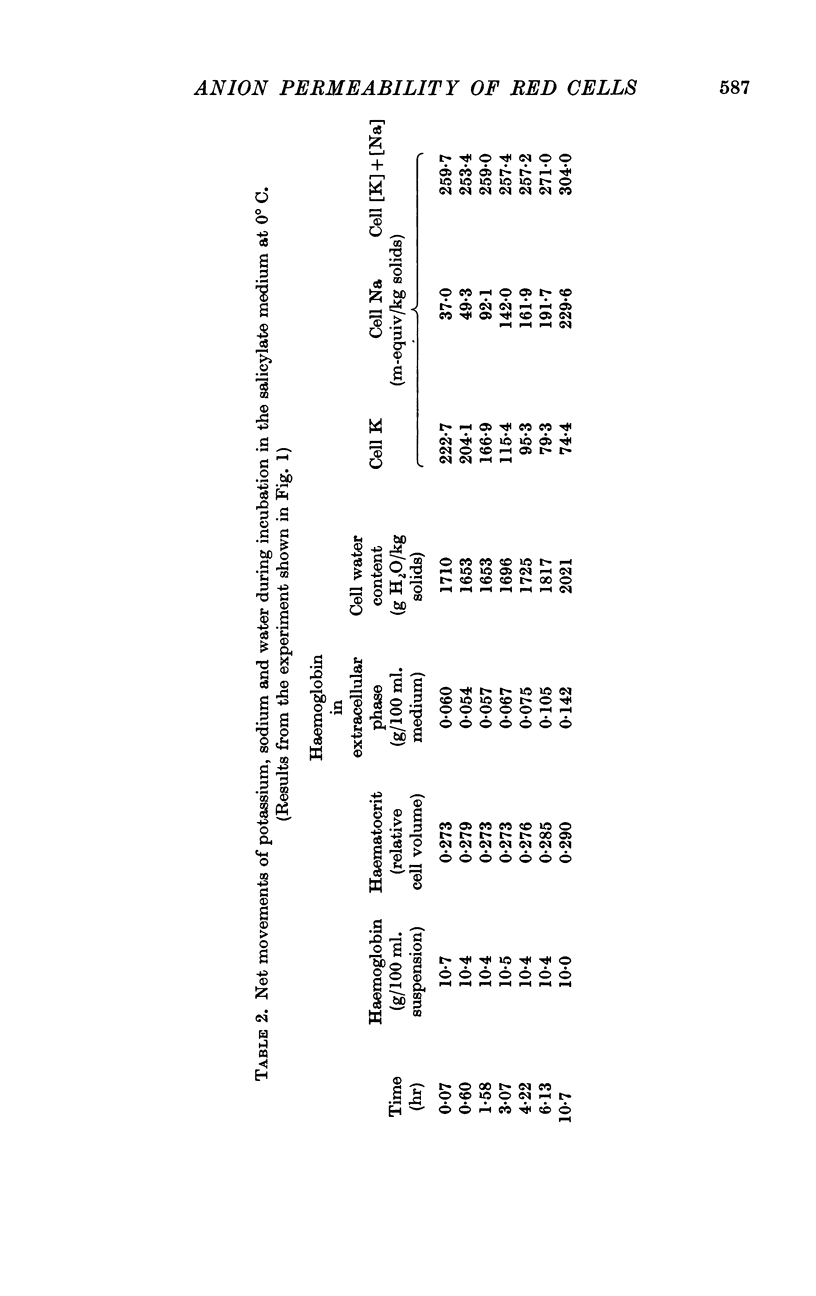
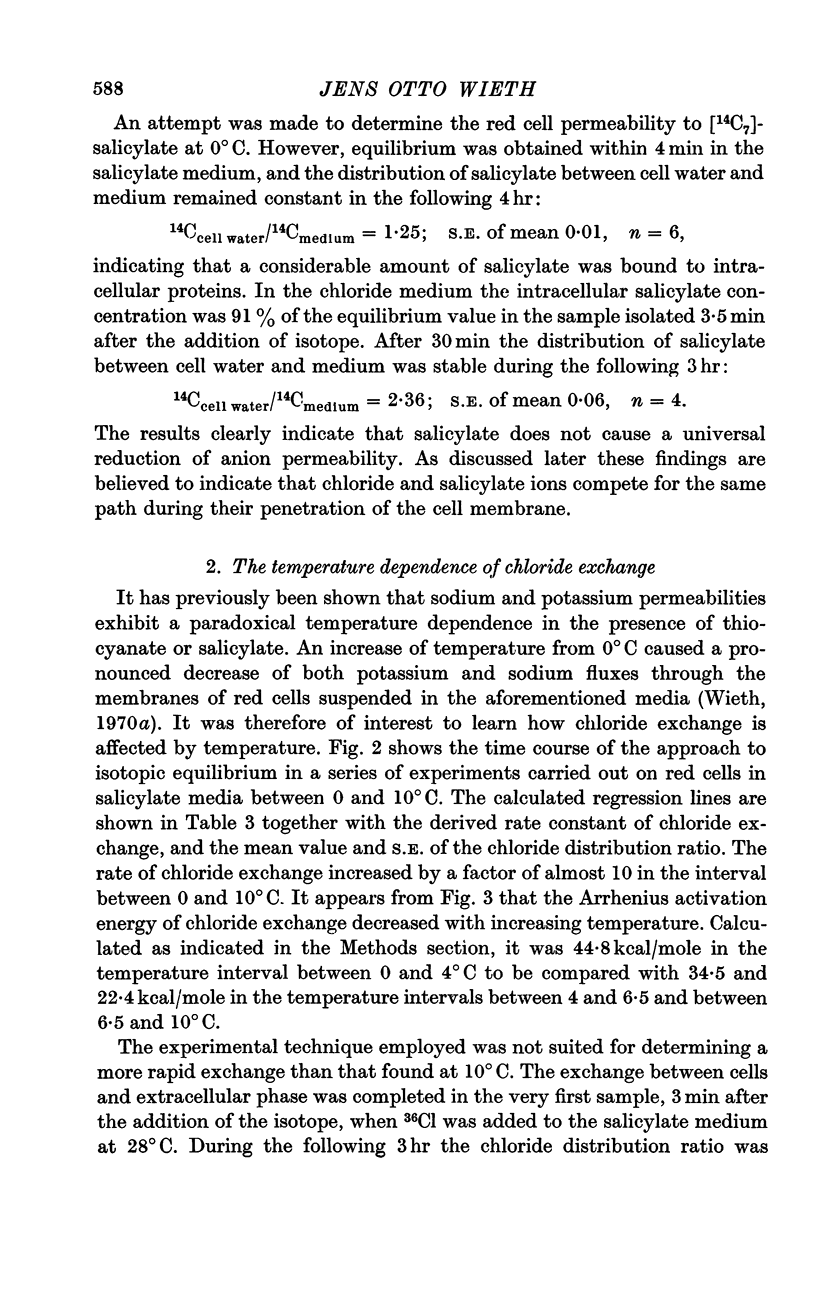
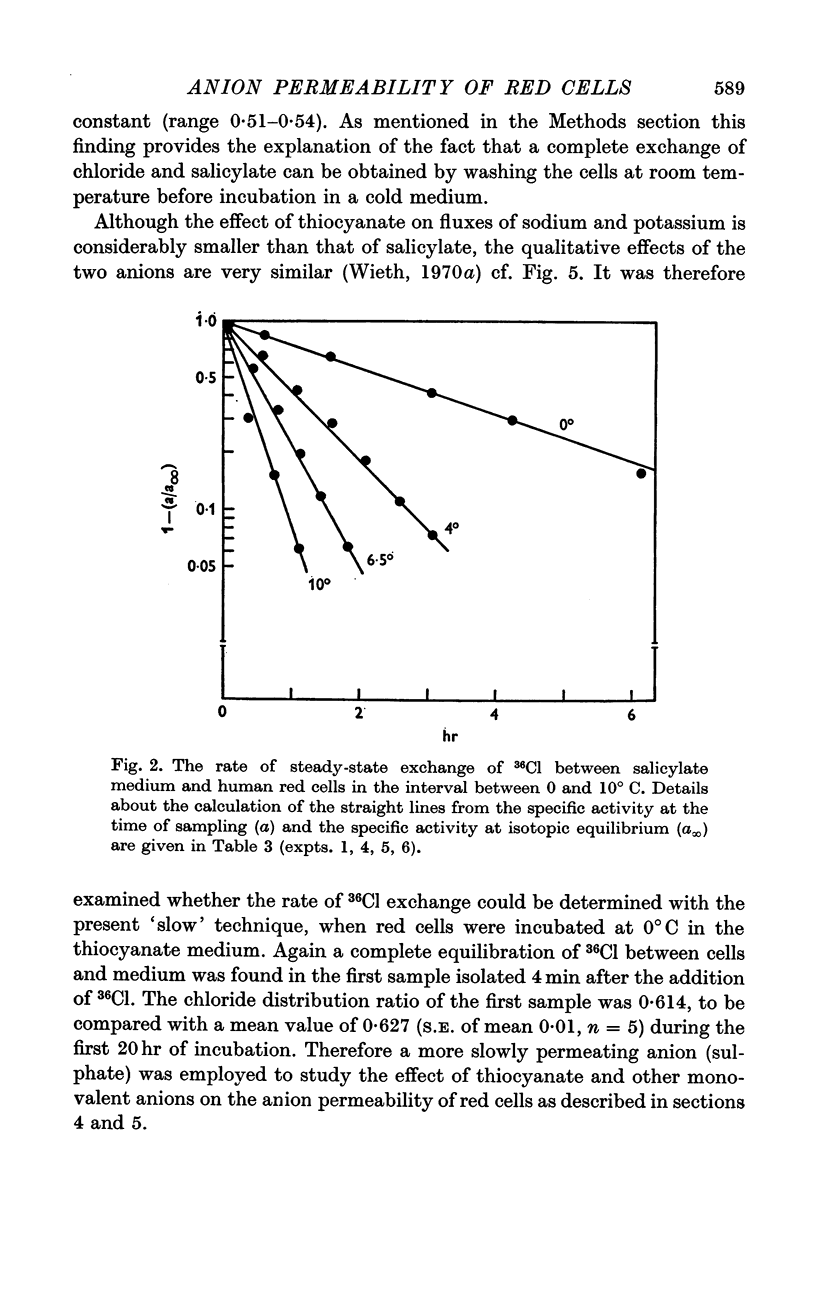
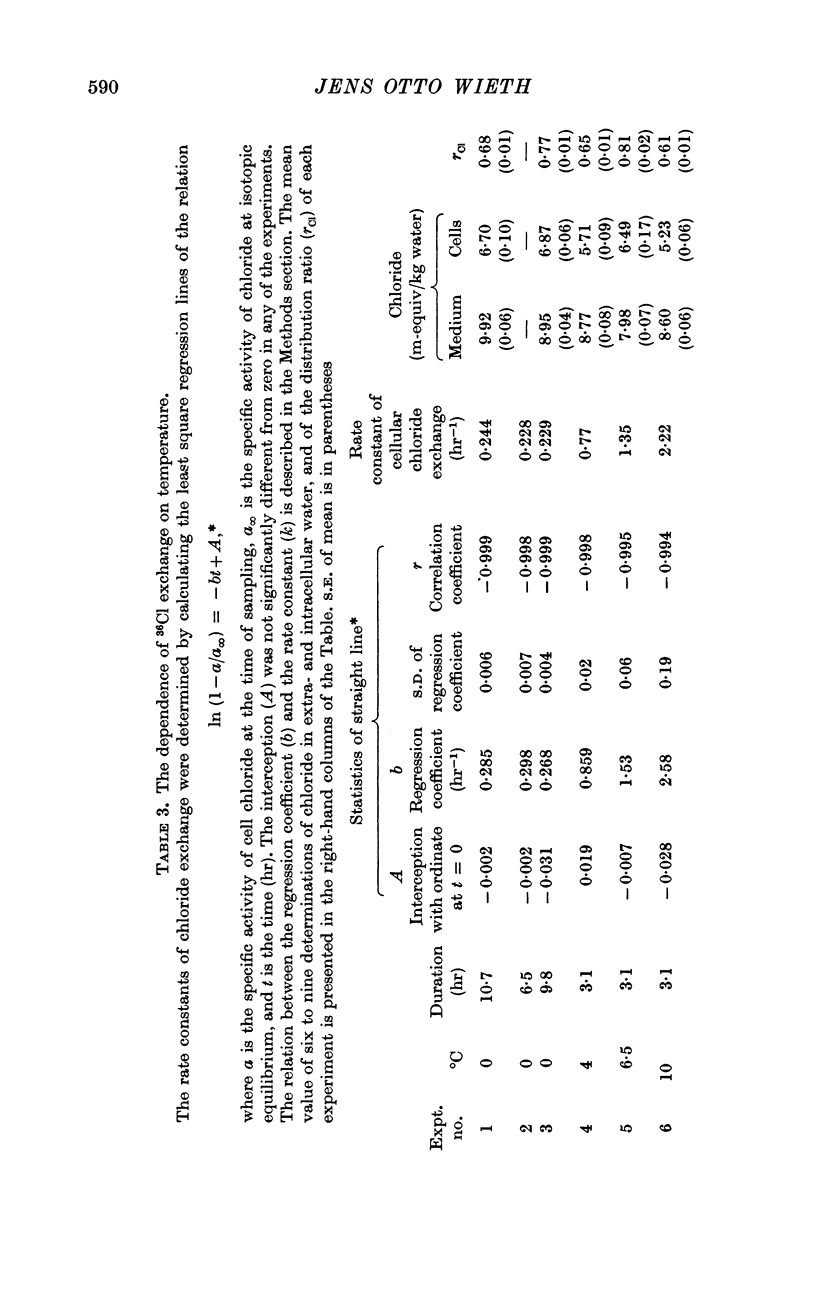
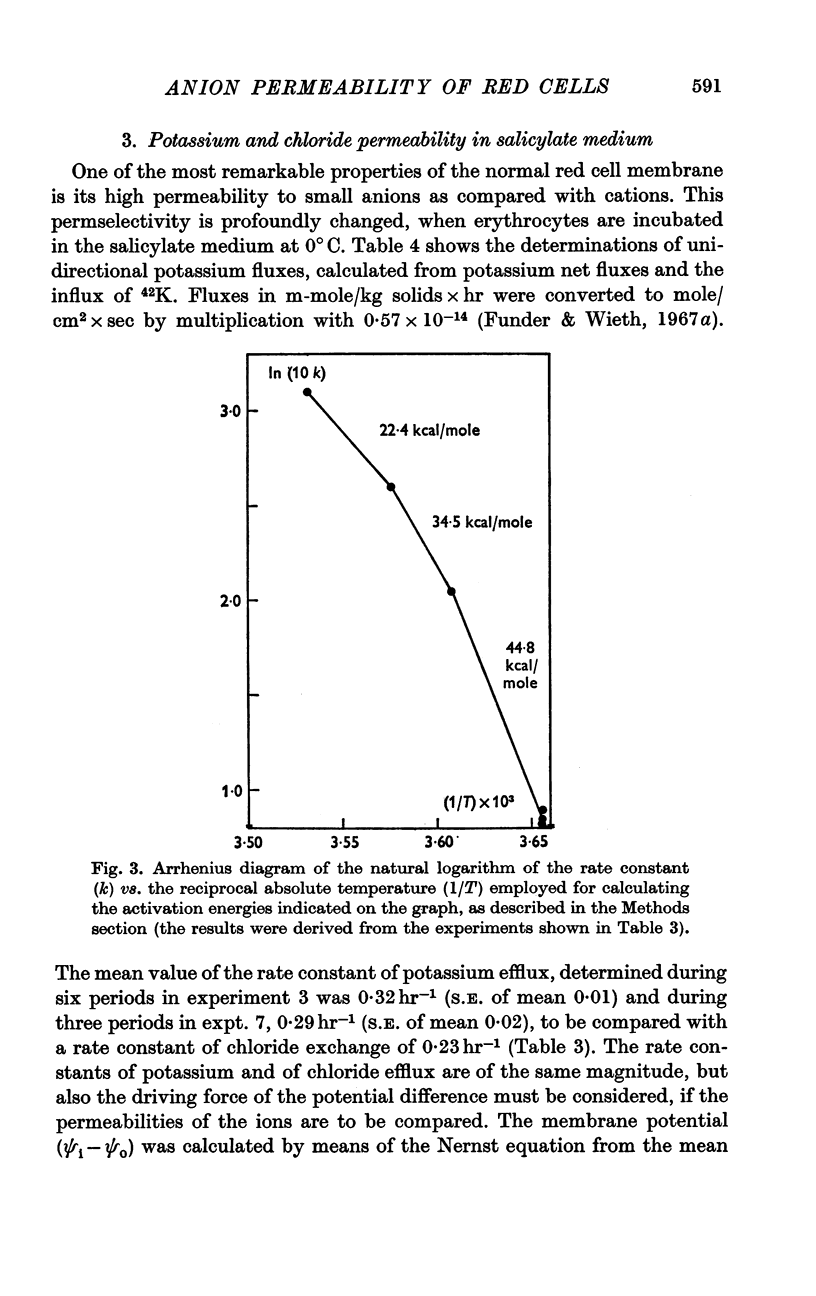
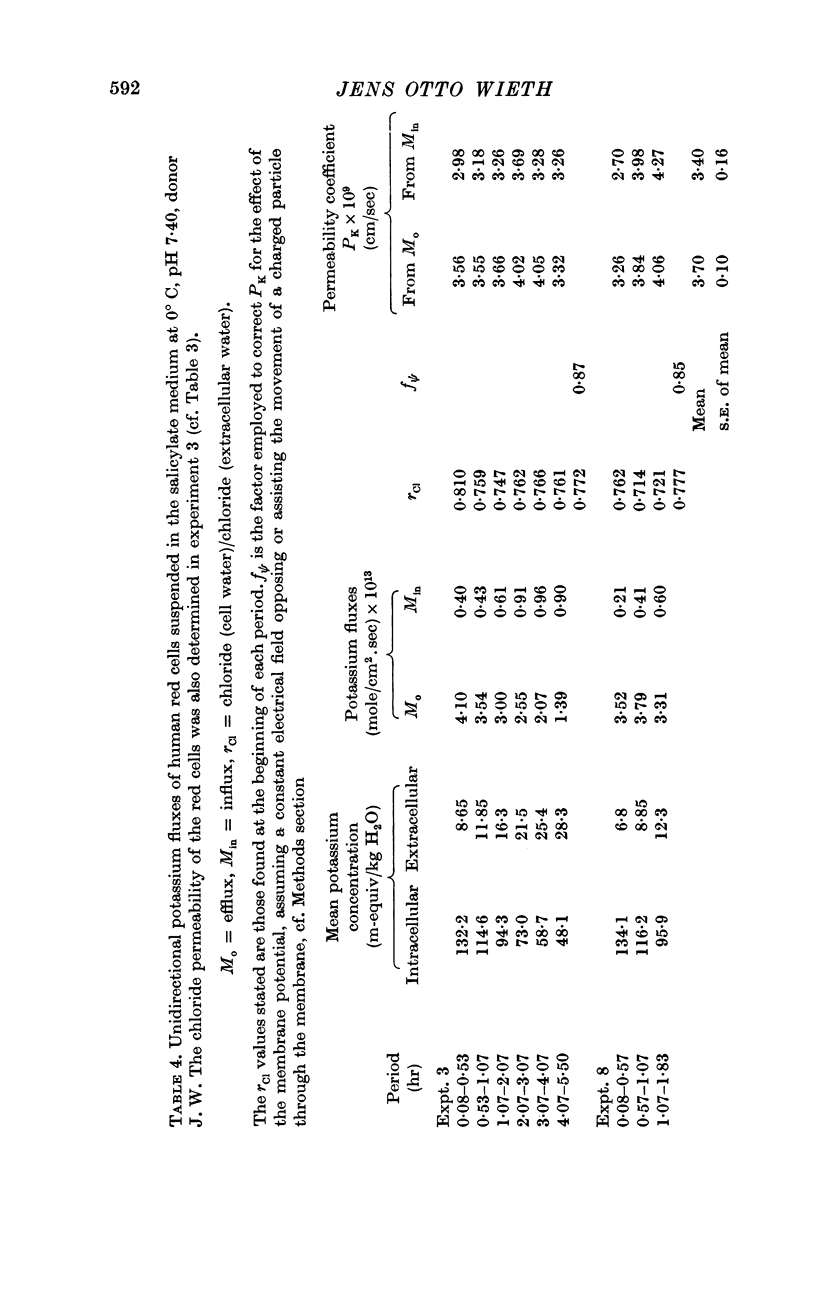
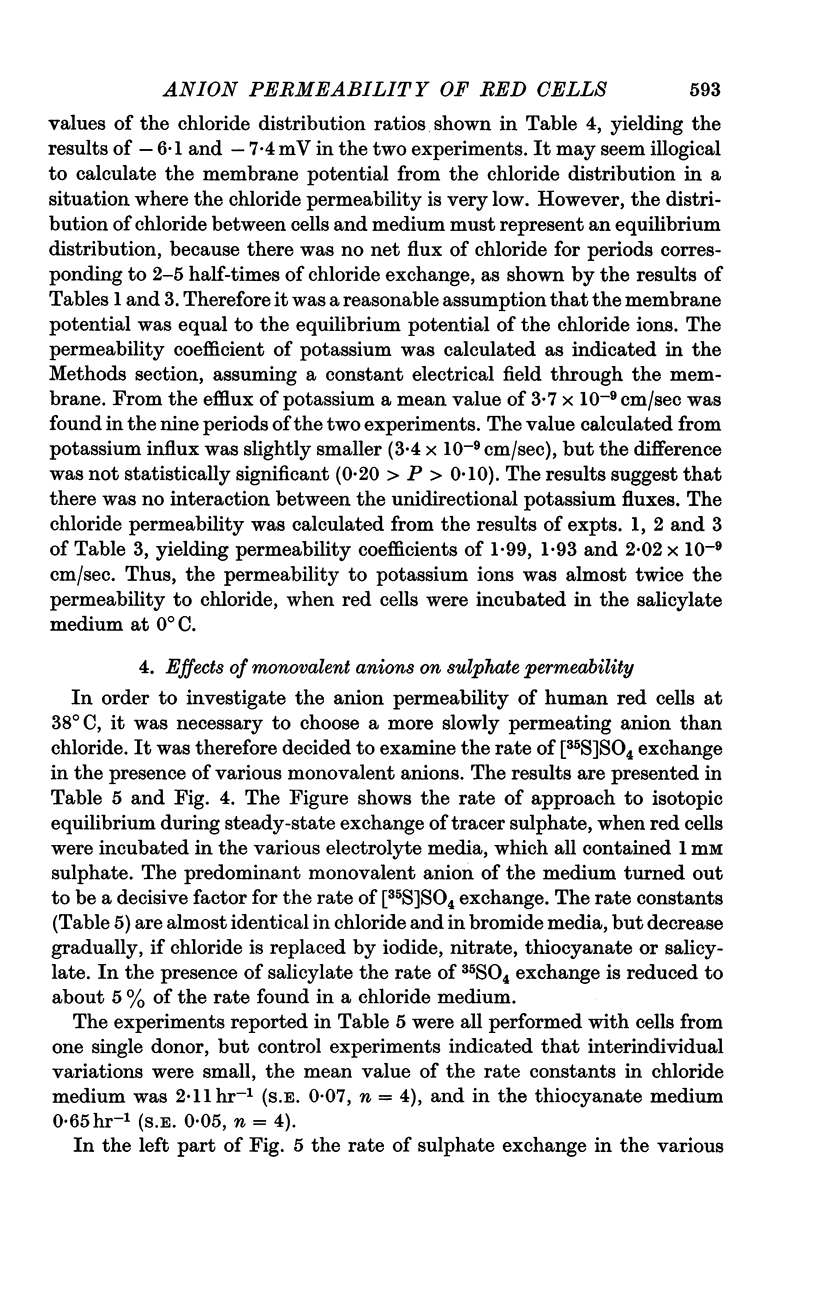
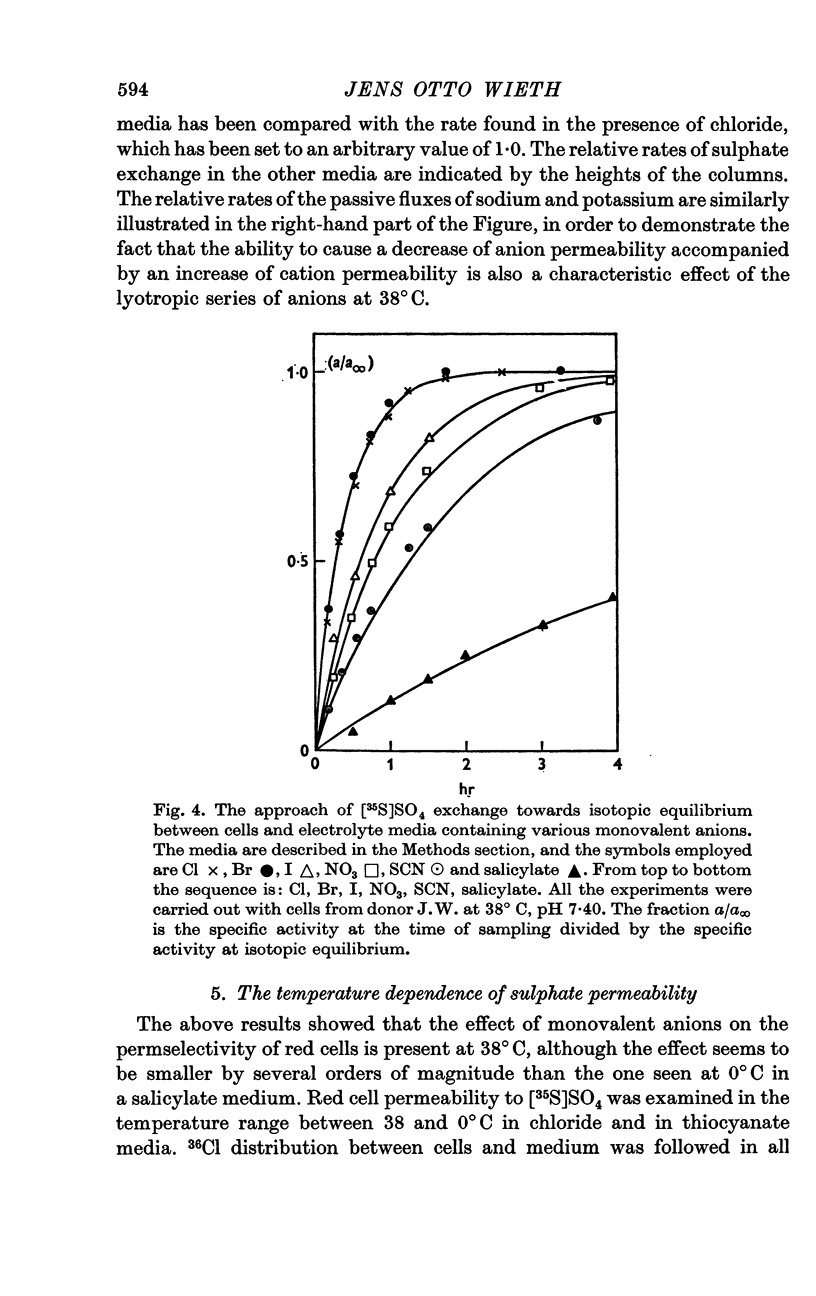
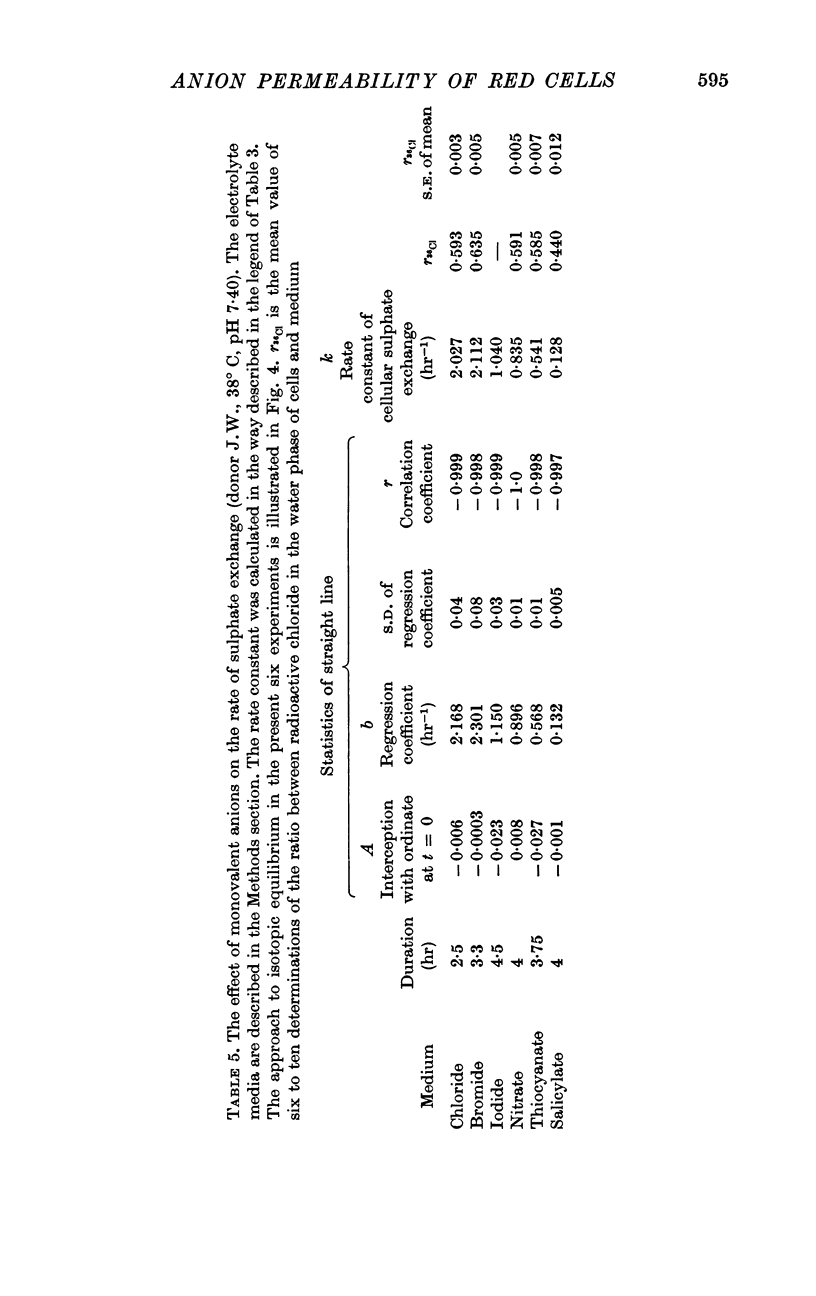
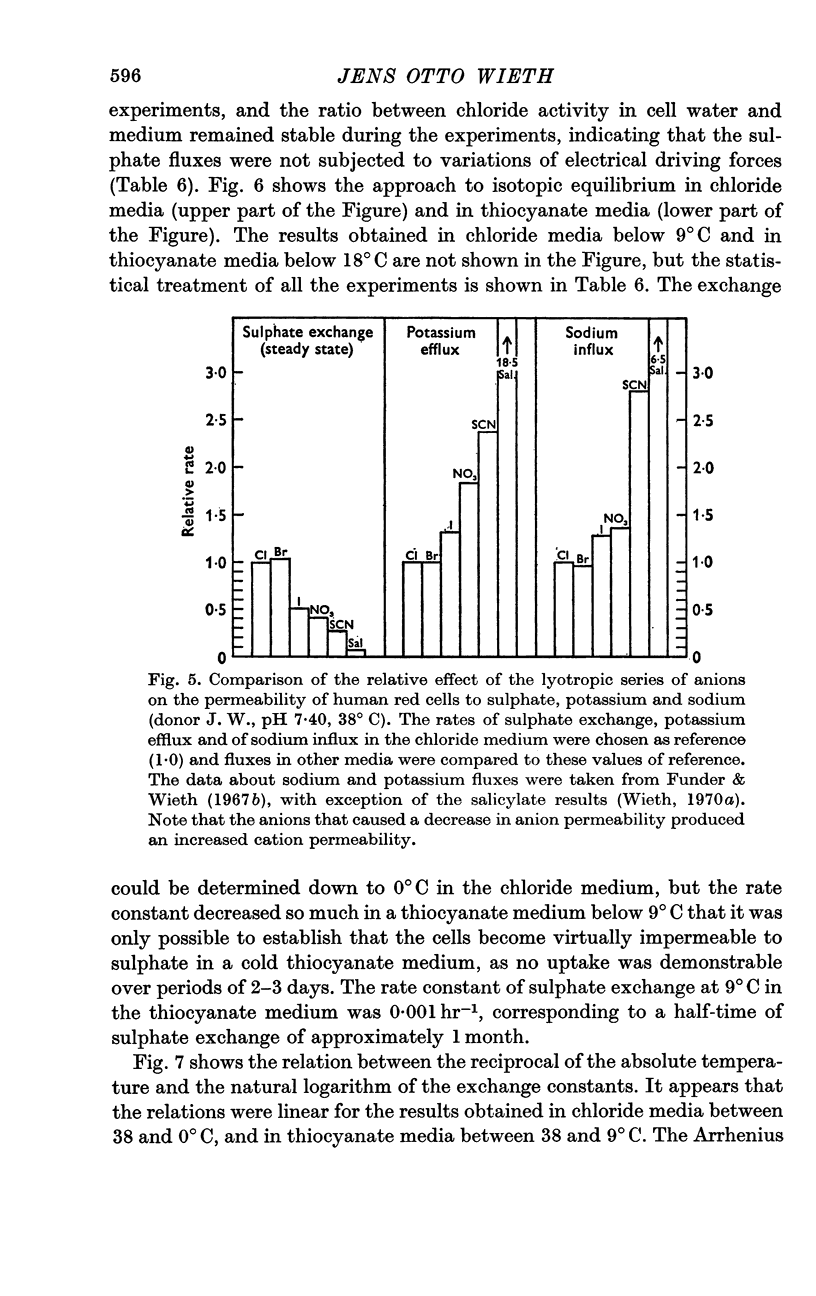
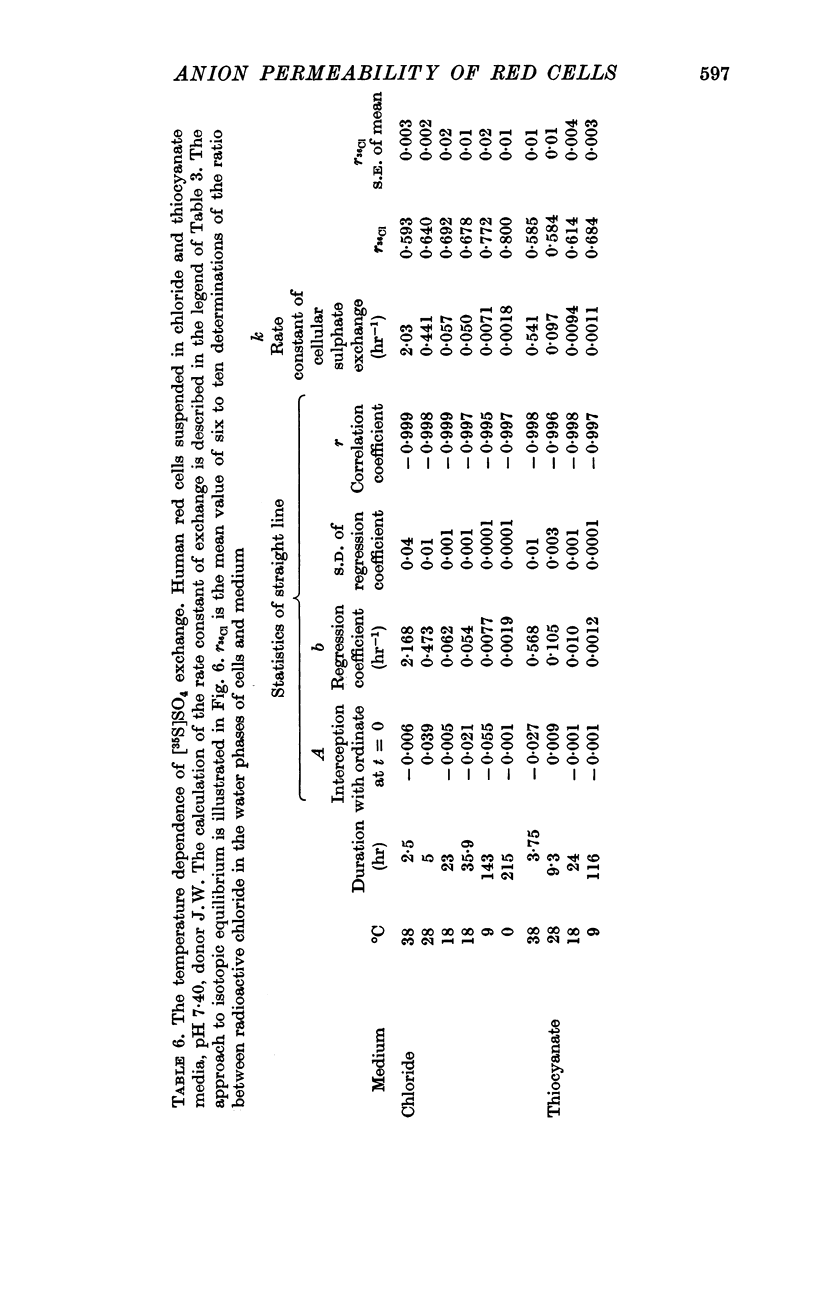
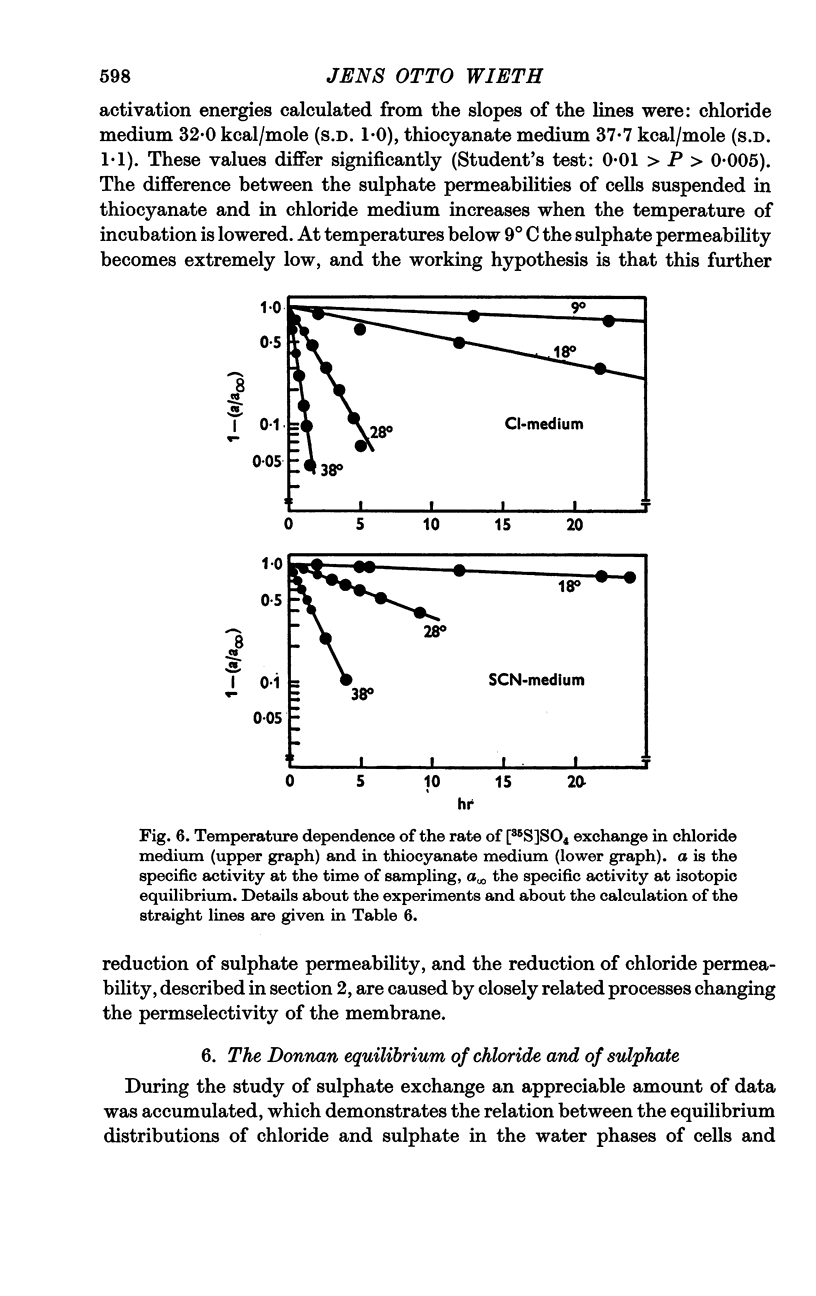
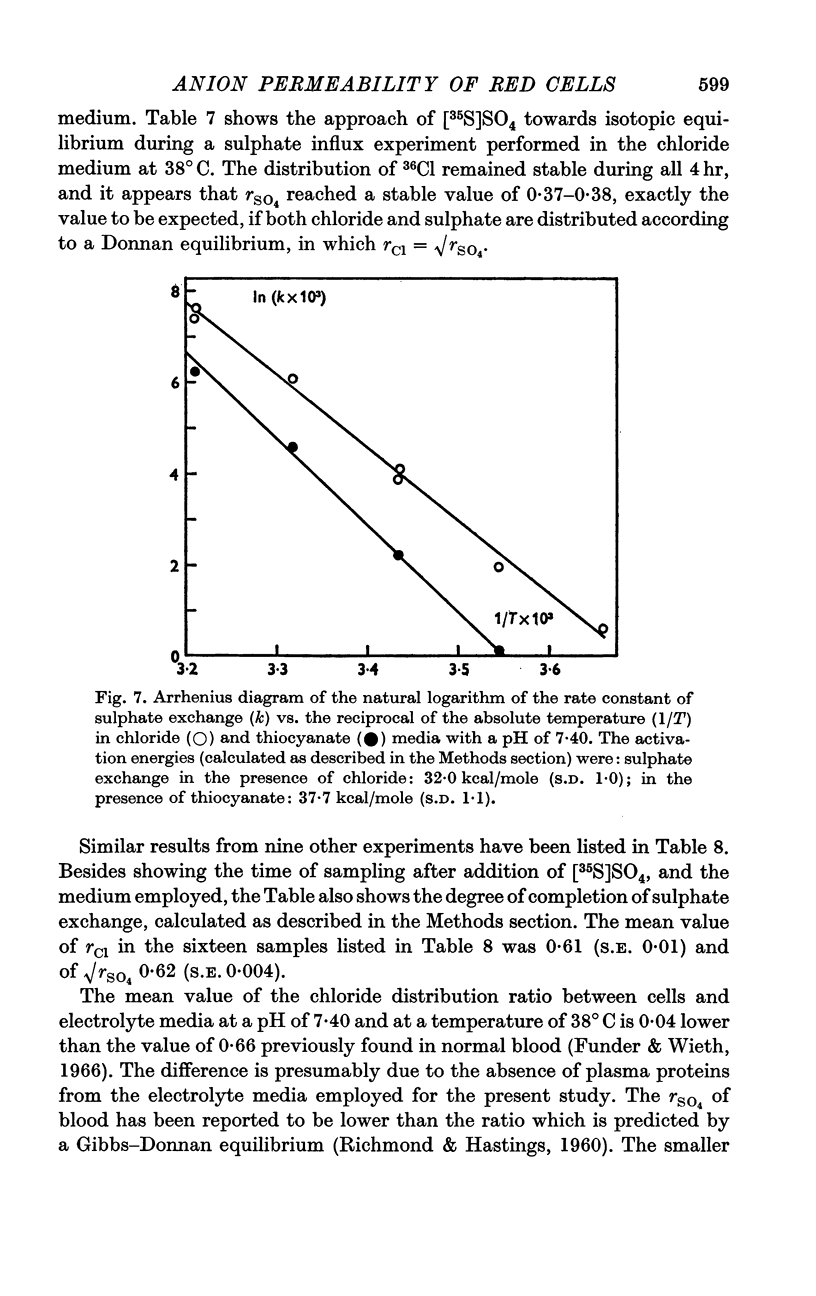
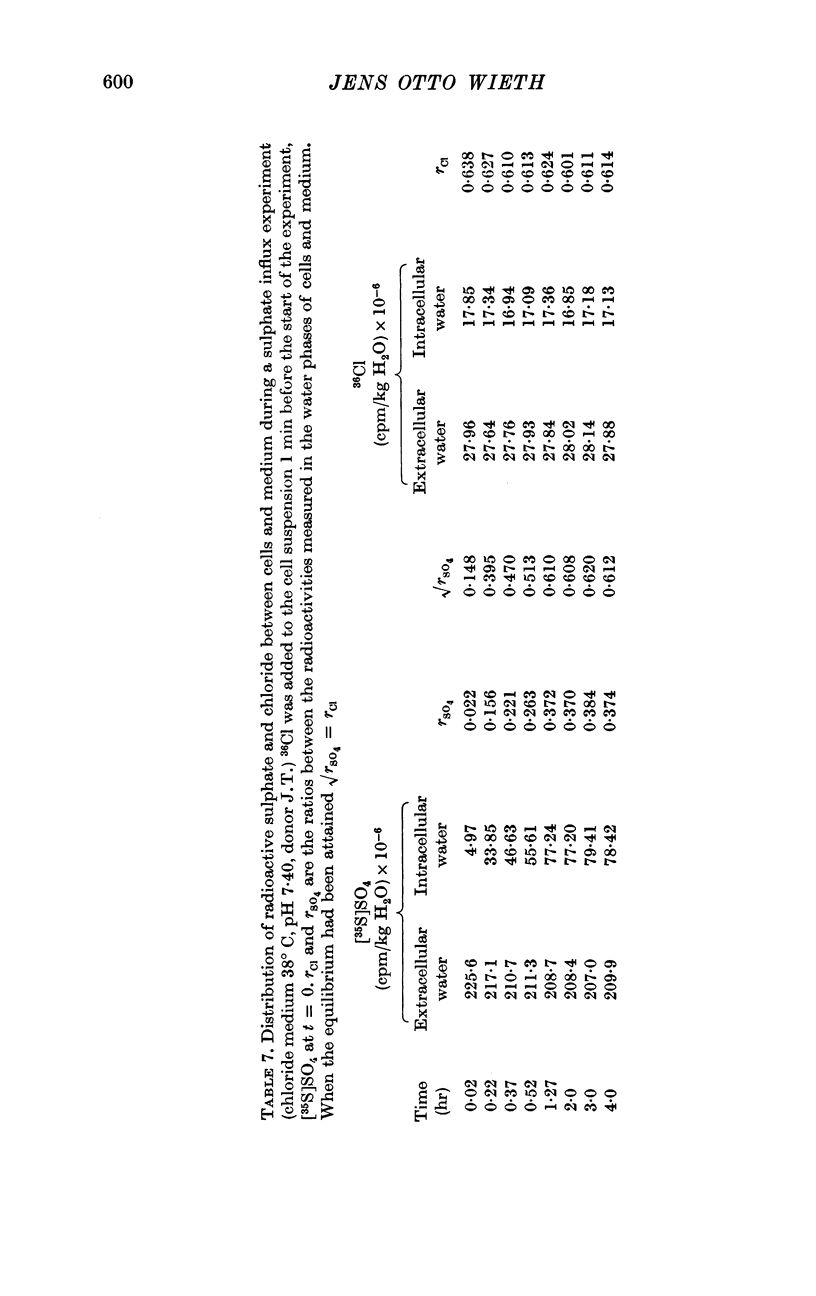
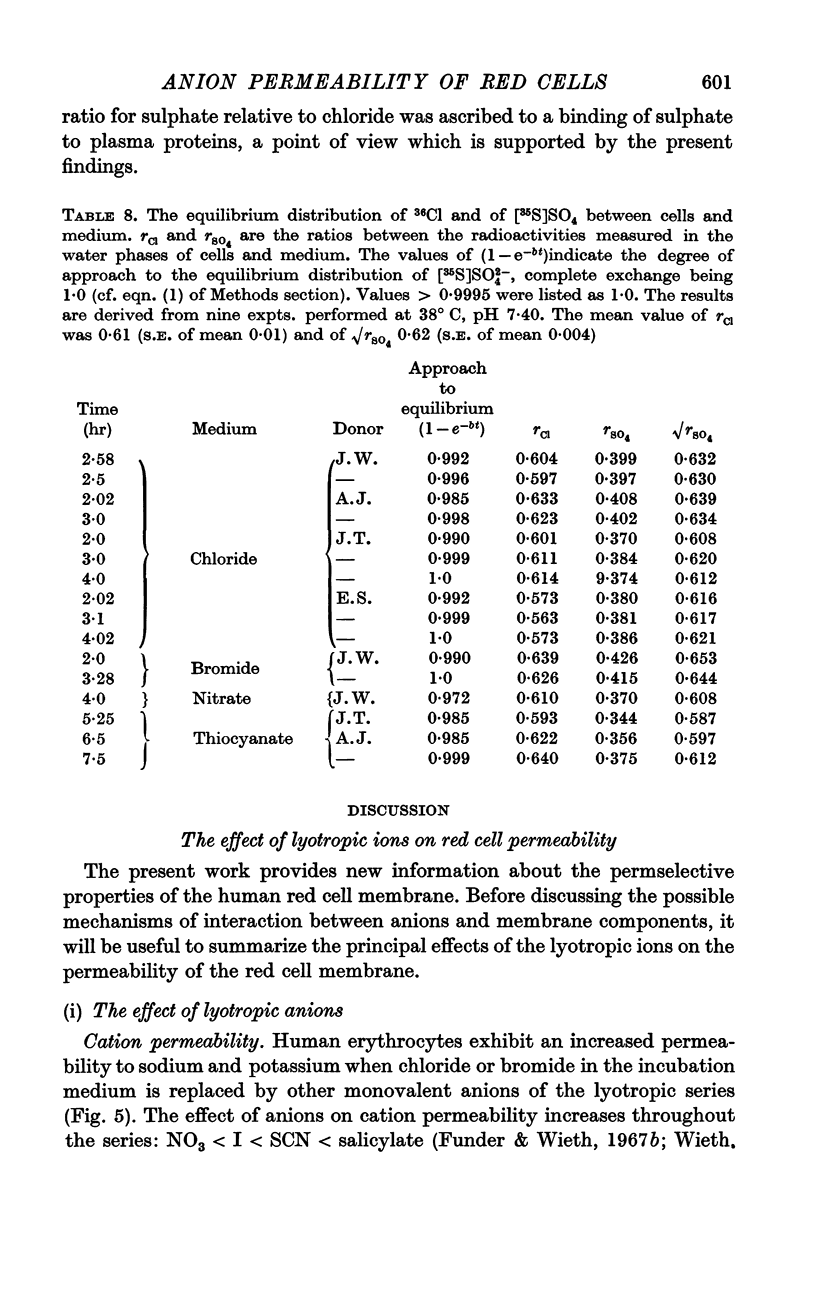
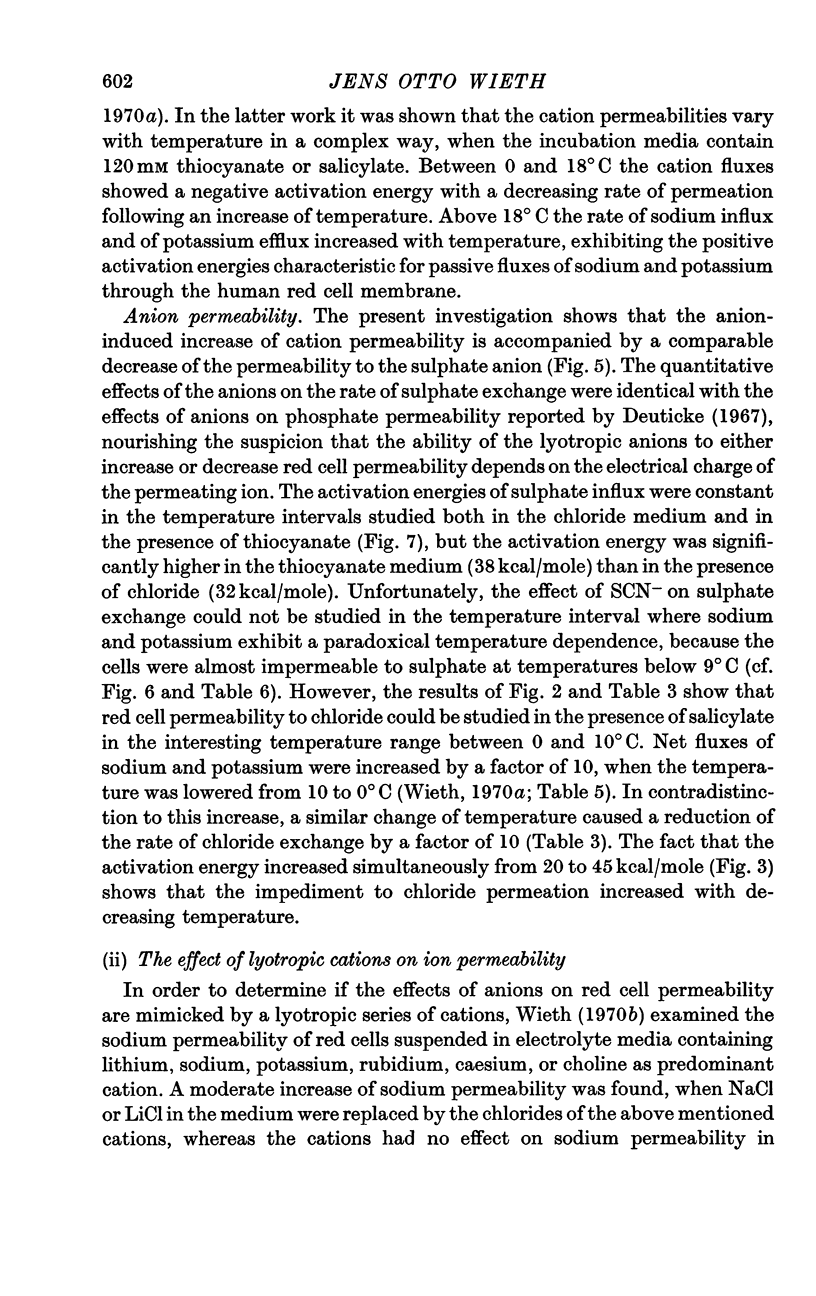
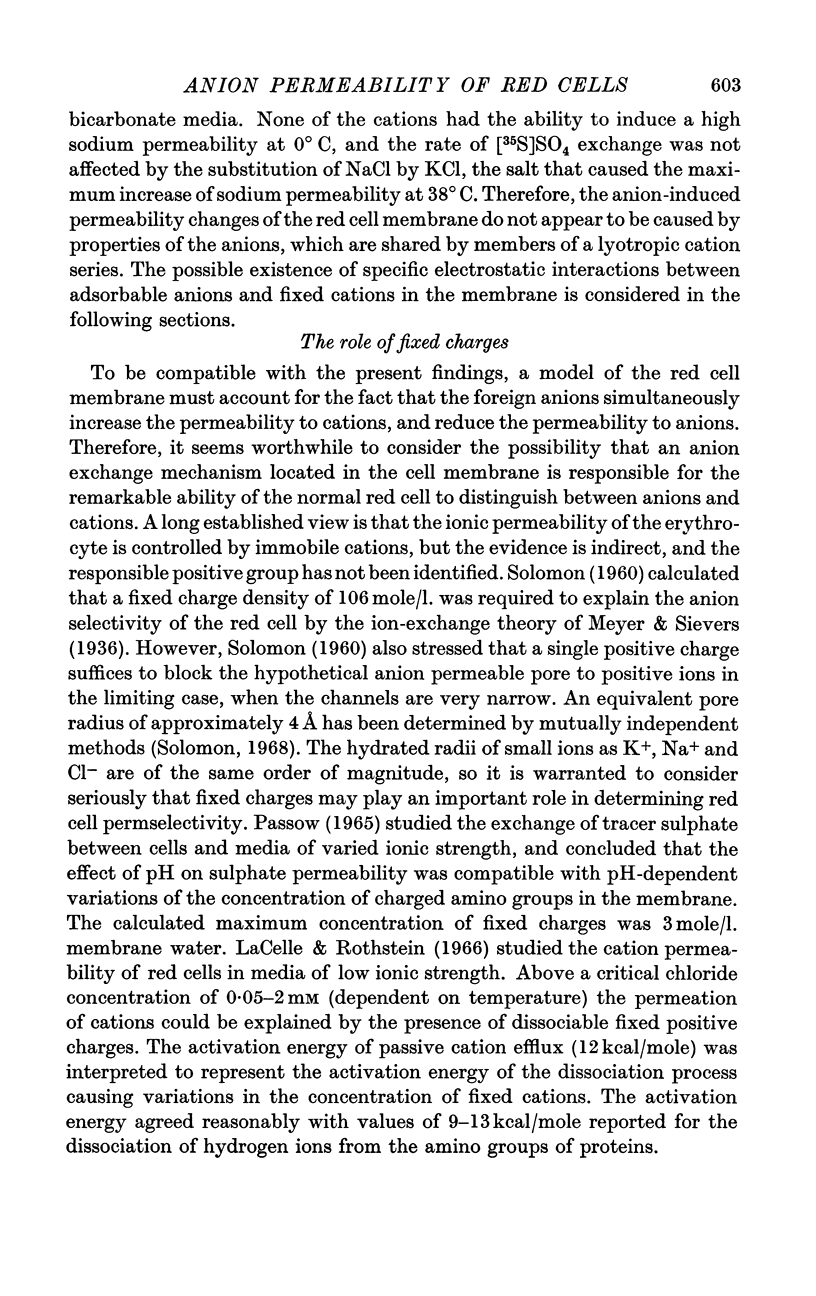
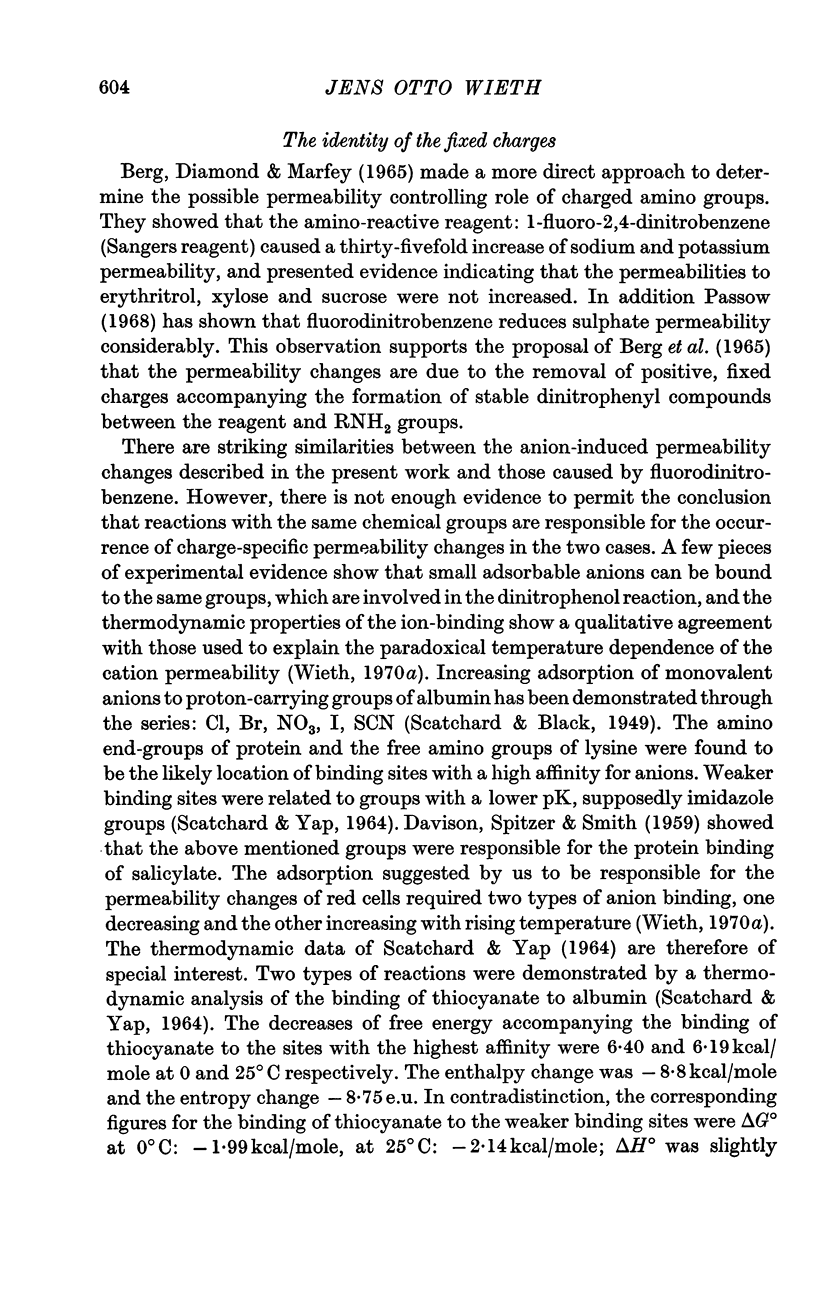
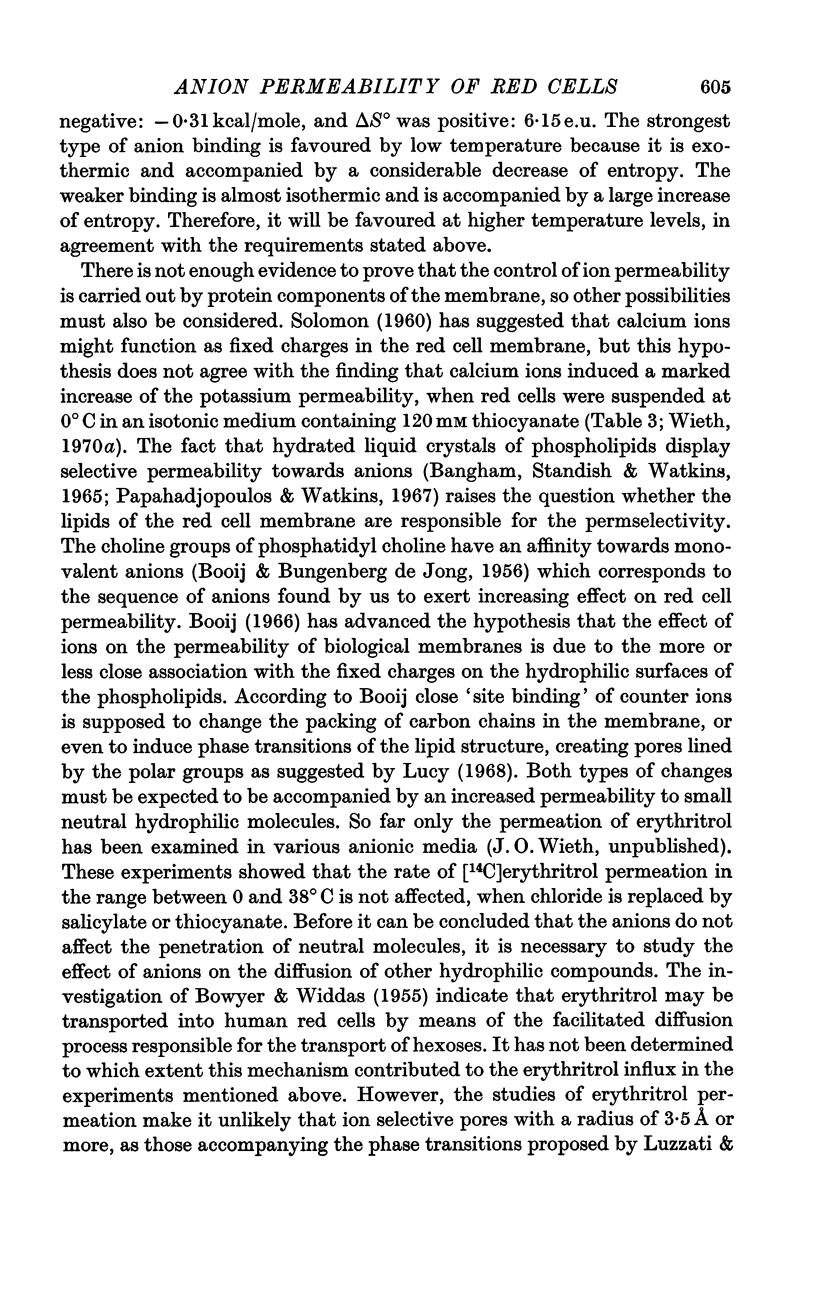
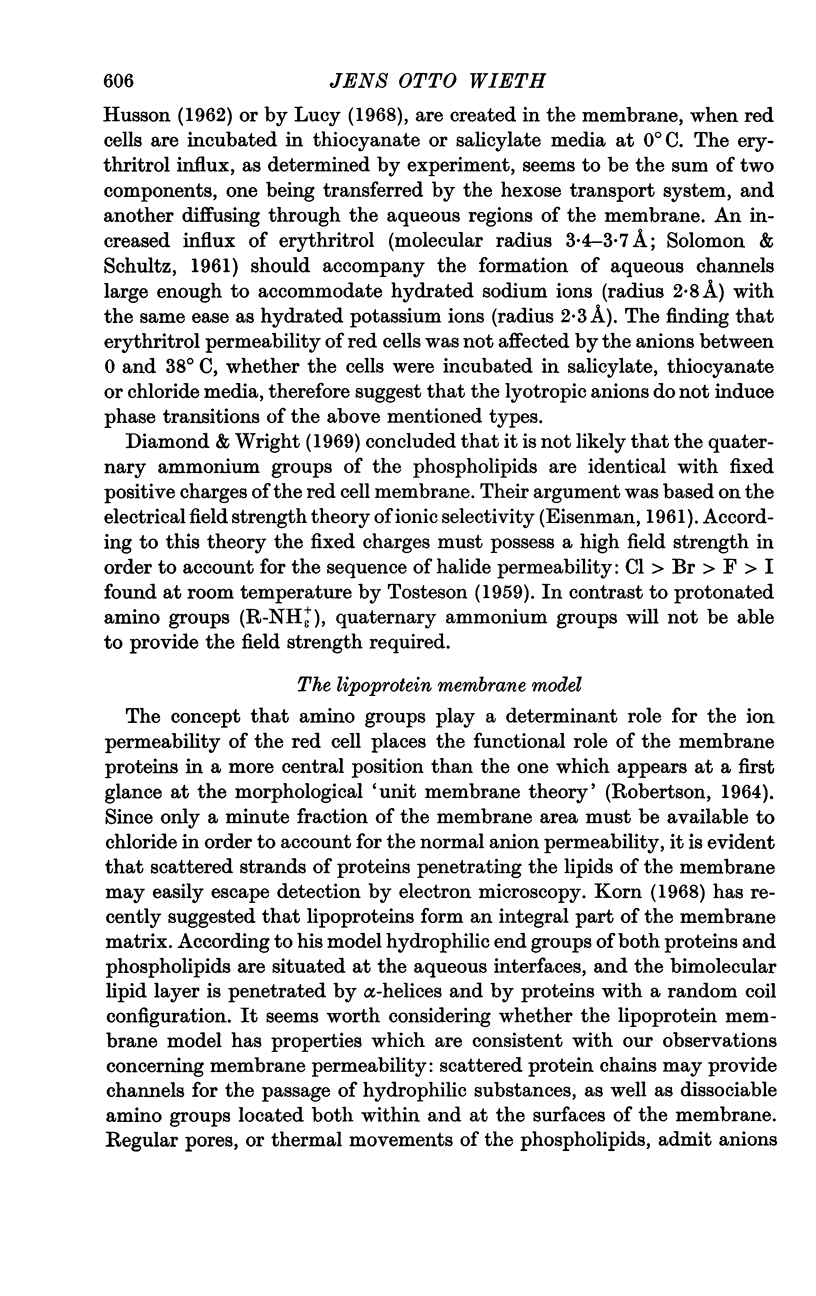
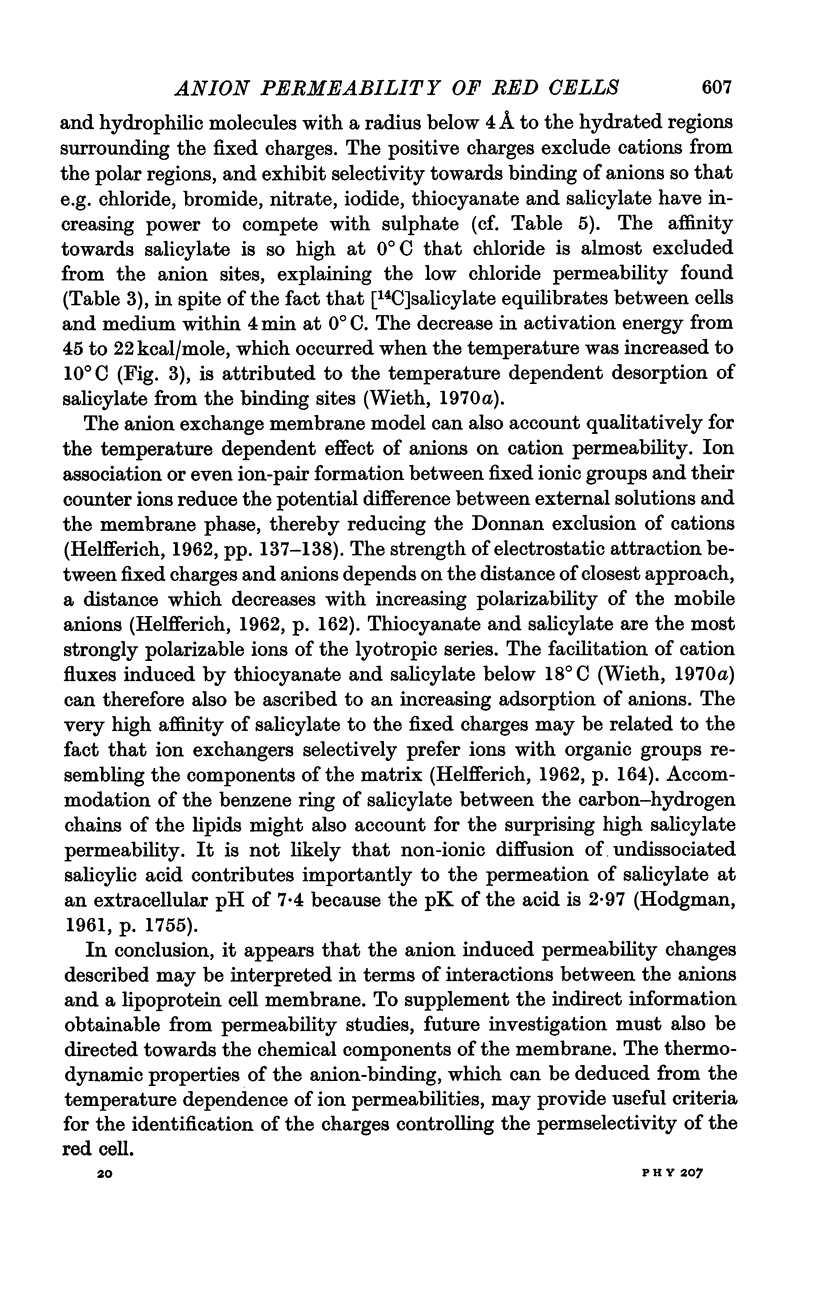
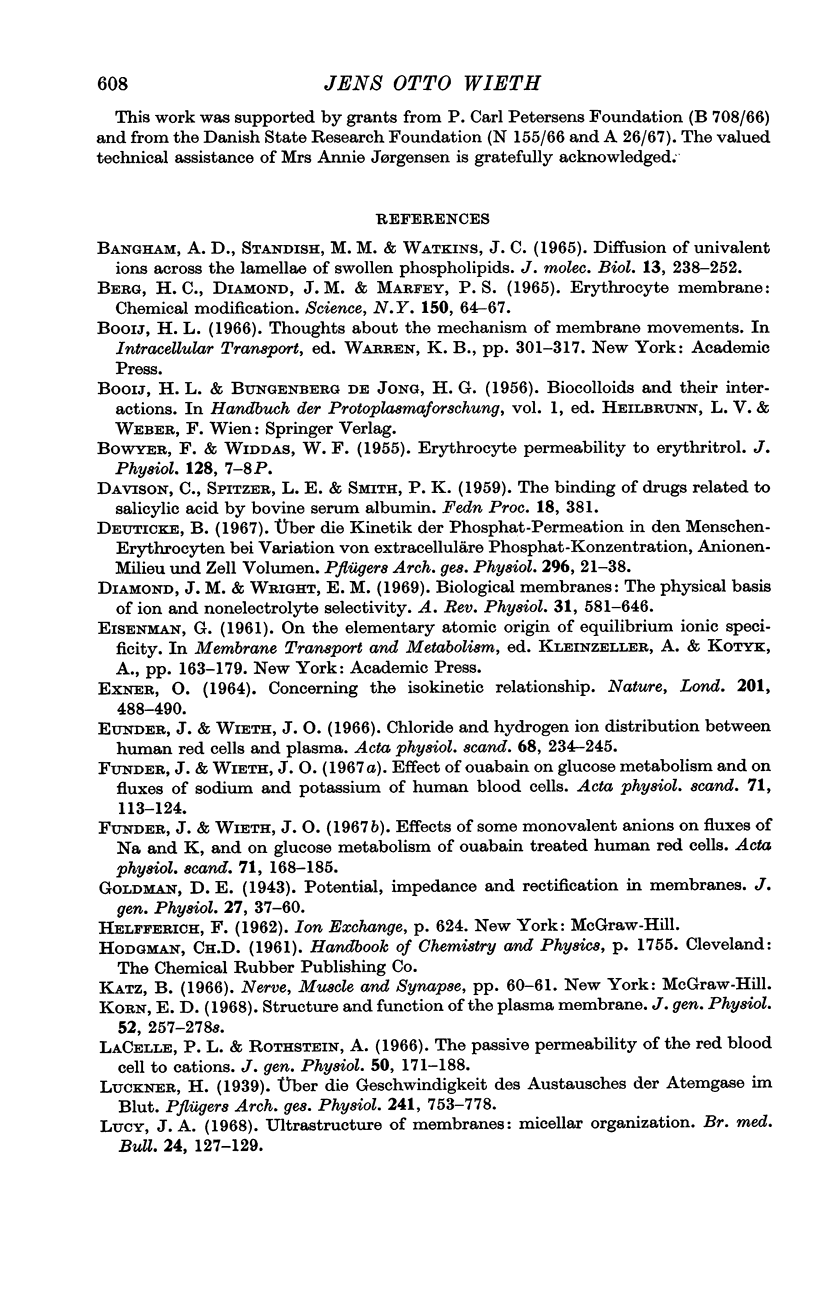
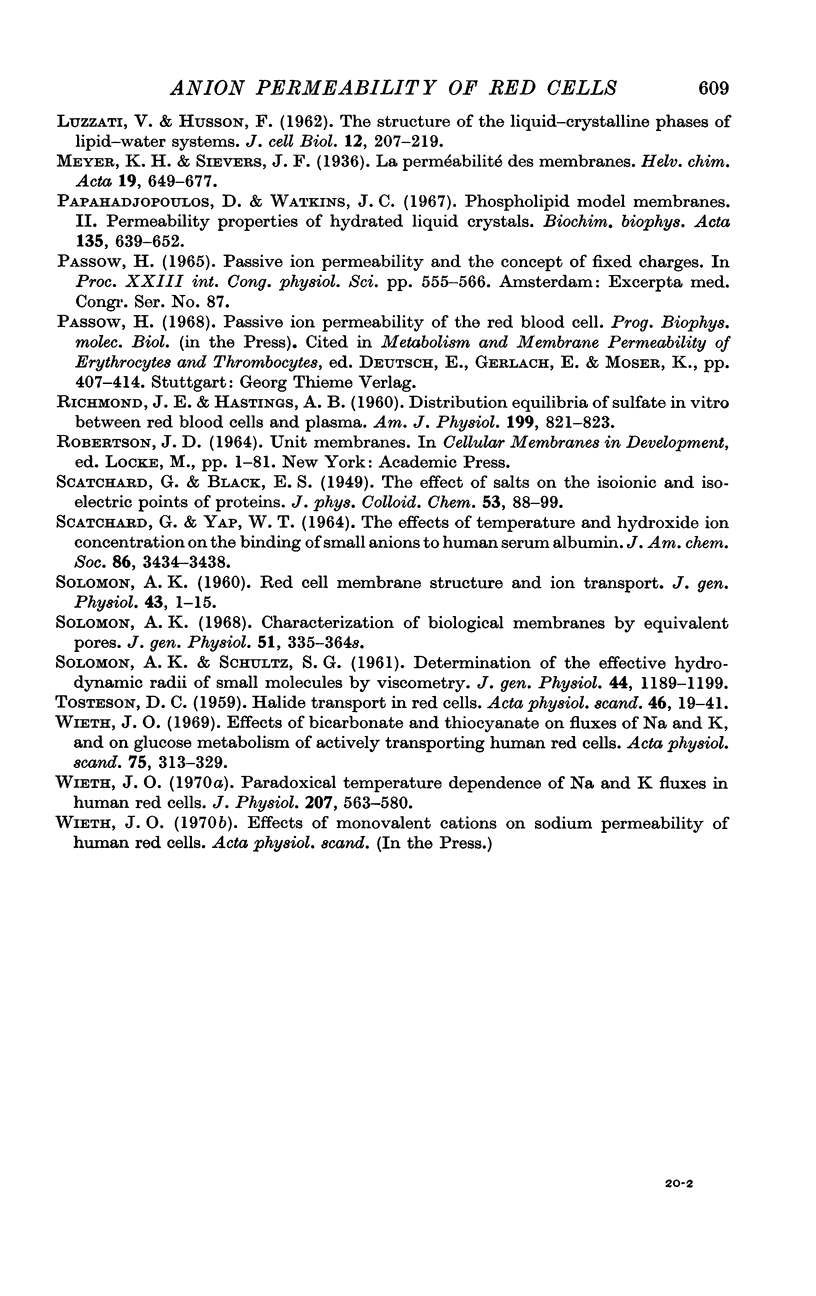
Selected References
These references are in PubMed. This may not be the complete list of references from this article.
- BOWYER F., WIDDAS W. F. Erythrocyte permeability to erythritol. J Physiol. 1955 Jul 28;129(1):7–8P. [PubMed] [Google Scholar]
- Bangham A. D., Standish M. M., Watkins J. C. Diffusion of univalent ions across the lamellae of swollen phospholipids. J Mol Biol. 1965 Aug;13(1):238–252. doi: 10.1016/s0022-2836(65)80093-6. [DOI] [PubMed] [Google Scholar]
- Berg H. C., Diamond J. M., Marfey P. S. Erythrocyte membrane: chemical modification. Science. 1965 Oct 1;150(3692):64–67. doi: 10.1126/science.150.3692.64. [DOI] [PubMed] [Google Scholar]
- Deuticke B. Uber die Kinetik der Phosphat-Permeation in den menschen-Erythrocyten bei Variation von extracellulärer Phosphat-Konzentration, anionen-Milieu and Zell-Volumen. Pflugers Arch Gesamte Physiol Menschen Tiere. 1967;296(1):21–38. [PubMed] [Google Scholar]
- Diamond J. M., Wright E. M. Biological membranes: the physical basis of ion and nonelectrolyte selectivity. Annu Rev Physiol. 1969;31:581–646. doi: 10.1146/annurev.ph.31.030169.003053. [DOI] [PubMed] [Google Scholar]
- Funder J., Wieth J. O. Effect of ouabain on gluclose metabolism and on fluxes of sodium and potassium of human blood cells. Acta Physiol Scand. 1967 Sep;71(1):113–124. doi: 10.1111/j.1748-1716.1967.tb03716.x. [DOI] [PubMed] [Google Scholar]
- Funder J., Wieth J. O. Effects of some monovalent anions on fluxes of Na and K, and on glucose metabolism of ouabain treated human red cells. Acta Physiol Scand. 1967 Oct-Nov;71(2):168–185. doi: 10.1111/j.1748-1716.1967.tb03723.x. [DOI] [PubMed] [Google Scholar]
- Goldman D. E. POTENTIAL, IMPEDANCE, AND RECTIFICATION IN MEMBRANES. J Gen Physiol. 1943 Sep 20;27(1):37–60. doi: 10.1085/jgp.27.1.37. [DOI] [PMC free article] [PubMed] [Google Scholar]
- Korn E. D. Structure and Function of the Plasma Membrane : A biochemical perspective. J Gen Physiol. 1968 Jul 1;52(1):257–278. [PMC free article] [PubMed] [Google Scholar]
- LUZZATI V., HUSSON F. The structure of the liquid-crystalline phasis of lipid-water systems. J Cell Biol. 1962 Feb;12:207–219. doi: 10.1083/jcb.12.2.207. [DOI] [PMC free article] [PubMed] [Google Scholar]
- LaCelle P. L., Rothsteto A. The passive permeability of the red blood cell in cations. J Gen Physiol. 1966 Sep;50(1):171–188. doi: 10.1085/jgp.50.1.171. [DOI] [PMC free article] [PubMed] [Google Scholar]
- Lucy J. A. Ultrastructure of membranes: micellar organization. Br Med Bull. 1968 May;24(2):127–129. doi: 10.1093/oxfordjournals.bmb.a070613. [DOI] [PubMed] [Google Scholar]
- Papahadjopoulos D., Watkins J. C. Phospholipid model membranes. II. Permeability properties of hydrated liquid crystals. Biochim Biophys Acta. 1967 Sep 9;135(4):639–652. doi: 10.1016/0005-2736(67)90095-8. [DOI] [PubMed] [Google Scholar]
- RICHMOND J. E., HASTINGS A. B. Distribution equilibria of sulfate in vitro between red blood cells and plasma. Am J Physiol. 1960 Nov;199:821–823. doi: 10.1152/ajplegacy.1960.199.5.821. [DOI] [PubMed] [Google Scholar]
- SCATCHARD G., BLACK E. S. The effect of salts on the isoionic and isoelectric points of proteins. J Phys Colloid Chem. 1949 Jan;53(1):88–99. [PubMed] [Google Scholar]
- SCHULTZ S. G., SOLOMON A. K. Determination of the effective hydrodynamic radii of small molecules by viscometry. J Gen Physiol. 1961 Jul;44:1189–1199. doi: 10.1085/jgp.44.6.1189. [DOI] [PMC free article] [PubMed] [Google Scholar]
- SOLOMON A. K. Red cell membrane structure and ion transport. J Gen Physiol. 1960 May;43:1–15. doi: 10.1085/jgp.43.5.1. [DOI] [PMC free article] [PubMed] [Google Scholar]
- Solomon A. K. Characterization of biological membranes by equivalent pores. J Gen Physiol. 1968 May 1;51(5):335–364. [PMC free article] [PubMed] [Google Scholar]
- Wieth J. O. Effects of bicarbonate and thiocyanate on fluxes of Na and K, and on glucose metabolism of actively transporting human red cells. Acta Physiol Scand. 1969 Mar;75(3):313–329. doi: 10.1111/j.1748-1716.1969.tb04384.x. [DOI] [PubMed] [Google Scholar]
- Wieth J. O. Paradoxical temperature dependence of sodium and potassium fluxes in human red cells. J Physiol. 1970 May;207(3):563–580. doi: 10.1113/jphysiol.1970.sp009081. [DOI] [PMC free article] [PubMed] [Google Scholar]