Abstract
The factors underlying sensory adaptation and conduction block have been studied in cutaneous mechanoreceptor neurones of the leech. A touch-sensitive cell was activated by applying mechanical or electrical stimuli to its receptive field on the skin. Impulses were recorded extracellularly from its axons and intracellularly from its cell body, which is situated within the C.N.S.
1. Activation of the touch cell by mechanical stimuli revealed two distinct types of adaptation with characteristically different time courses. Sustained pressure on the skin caused a brief burst of impulses at the onset of the stimulus. This rapid adaptation to pressure was restricted to the part of the receptive field that had been stimulated mechanically. A second type of adaptation developed more slowly during the course of repetitive mechanical stimulation. It persisted for many seconds after the end of a train of impulses and appeared as an increase in the threshold to mechanical stimuli not only in the region of skin that had been rubbed but throughout the receptive field of the cell.
2. Impulses initiated in the cell body propagated antidromically towards the skin and also raised the threshold to touch, indicating that after-effects of impulse activity were responsible for the long-lasting threshold increase.
3. Repetitive mechanical stimulation could also produce a reversible conduction block in branches of the touch cell. The block occurred in discrete regions of low safety factor such as axonal branch points both within the ganglion and in the periphery. In some experiments impulses intermittently failed to reach one axonal branch yet continued to invade a separate branch of the same cell.
4. Several lines of evidence indicate that both conduction block and the slow component of adaptation are linked to a prolonged hyperpolarization that follows repetitive stimulation of the touch cell. Strophanthidin, which blocks the after-hyperpolarization in touch cells, reduced the adaptation following trains of impulses and also relieved a conduction block previously established by repetitive stimulation. Furthermore, a comparison of the effects of hyperpolarizations produced by current injection and by repetitive firing showed that most of the threshold increase in the cell body after a train of impulses could be attributed directly to the membrane hyperpolarization.
5. These experiments suggest several ways in which repetitive activity can have pronounced and long-lasting effects on the performance of a highly branched sensory cell. Thus a relatively small number of impulses in a touch cell can markedly decrease its sensitivity to touch. The functional role of the conduction block observed during vigorous stimulation is not as clear because activity for many seconds or minutes is usually needed to establish a block in the larger branches of the cell.
Full text
PDF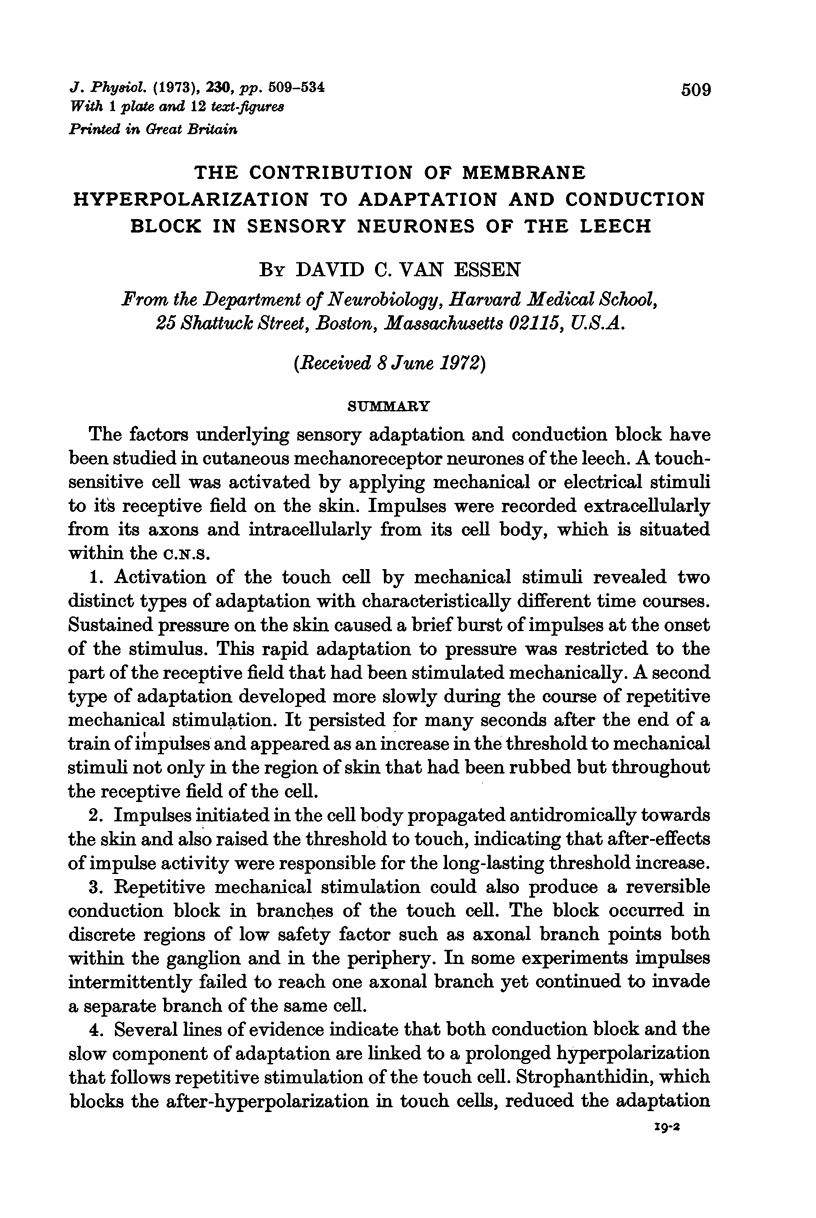
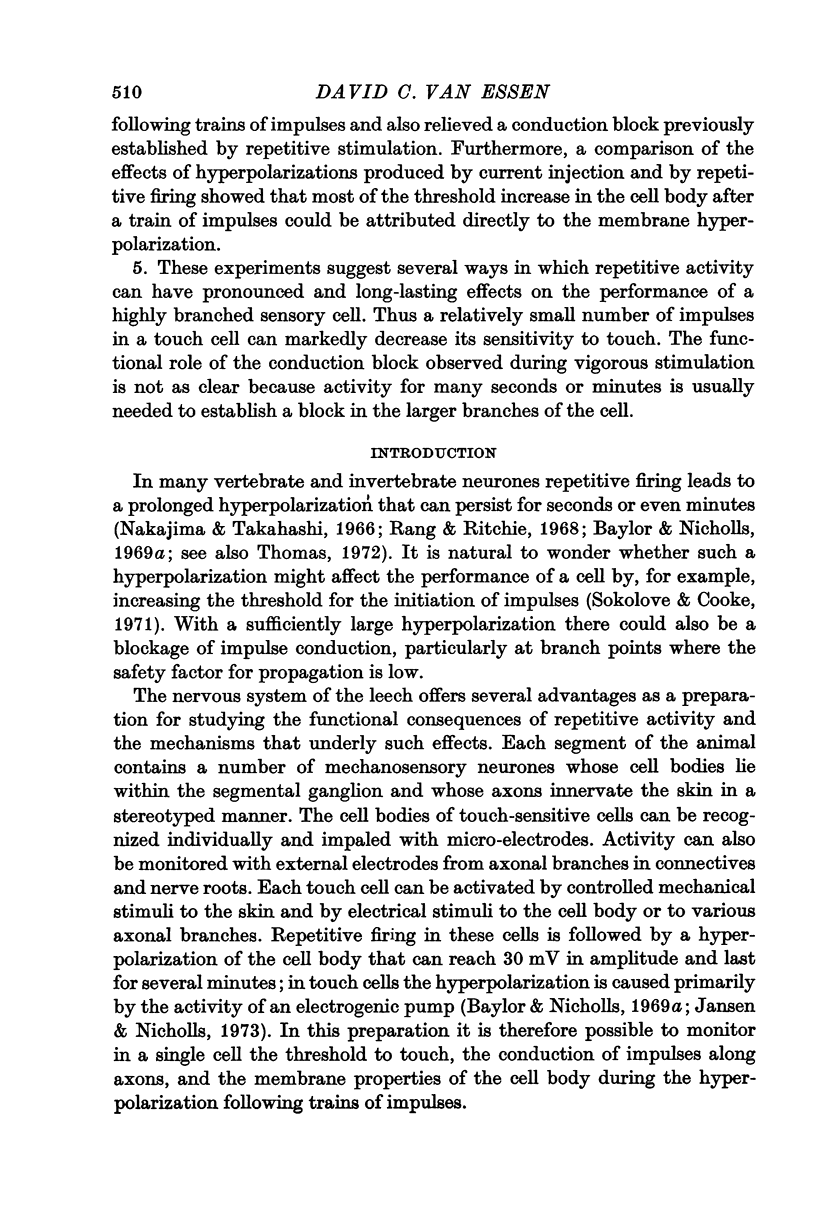
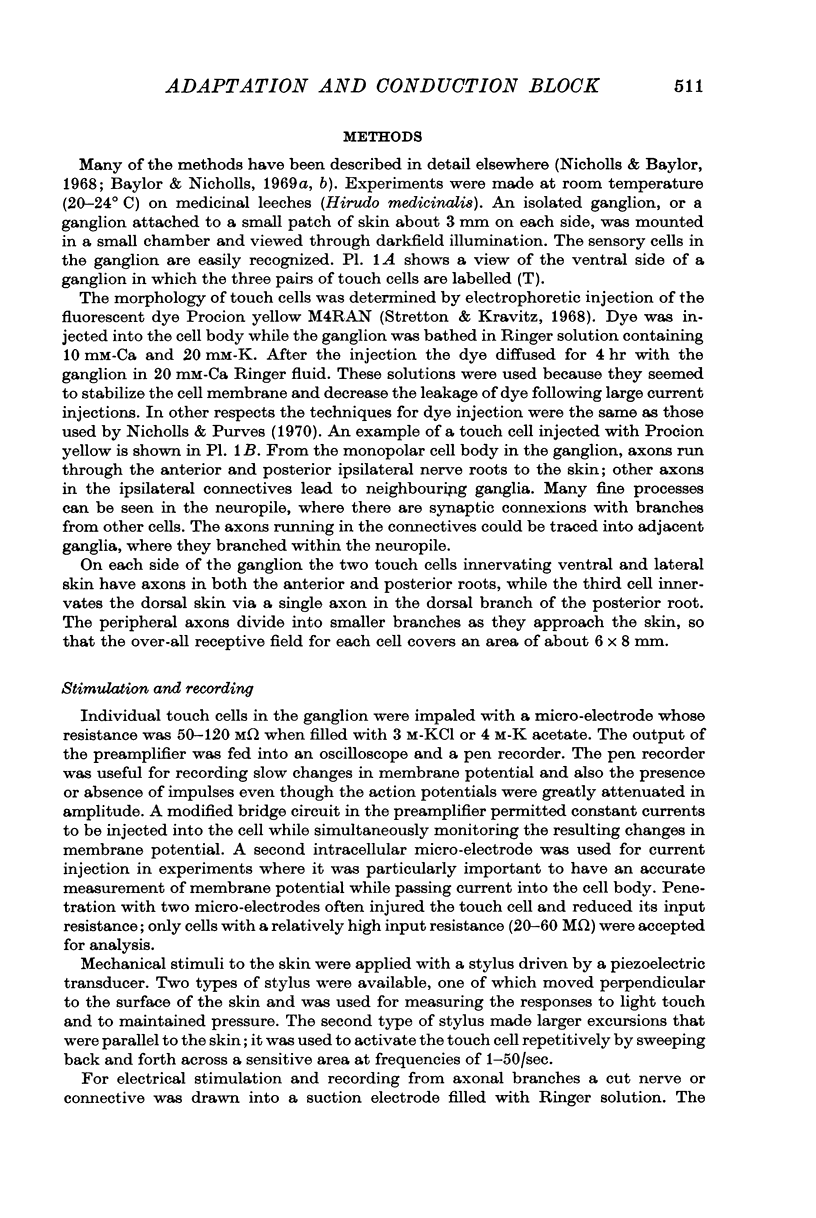
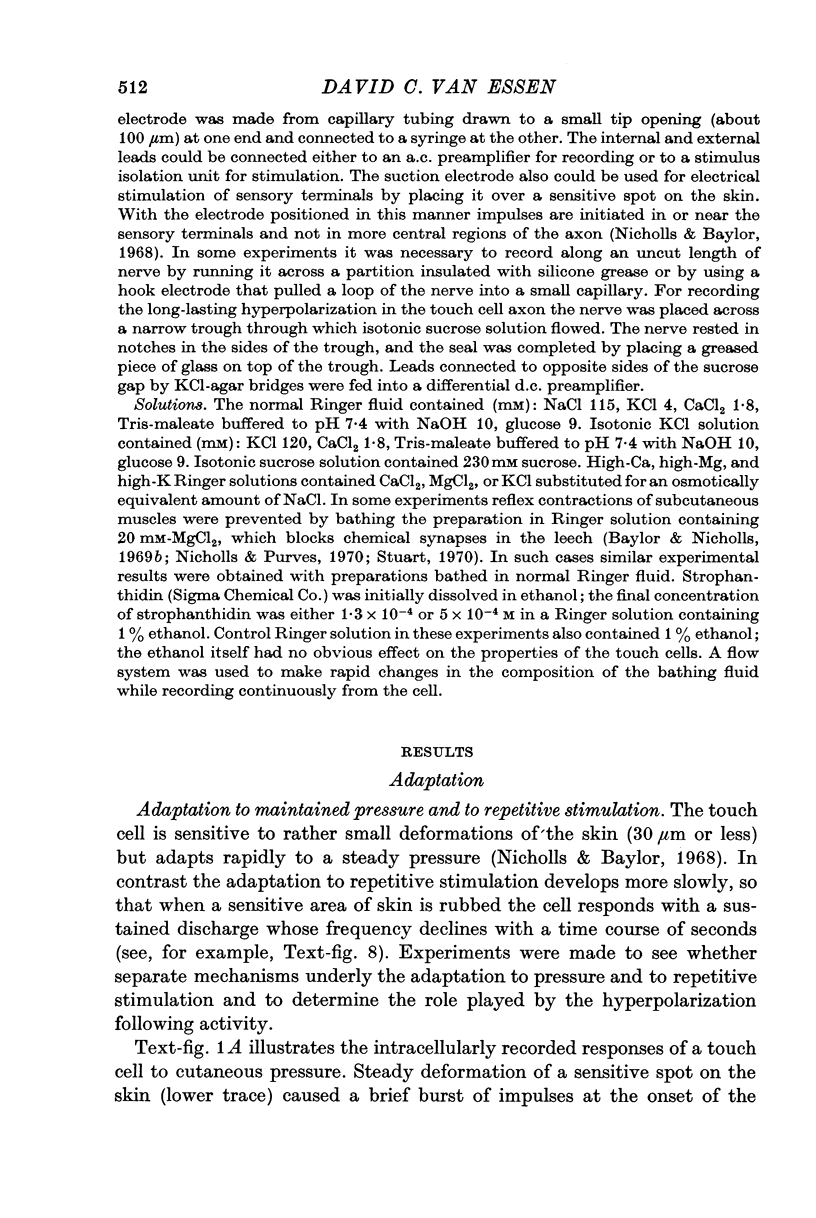
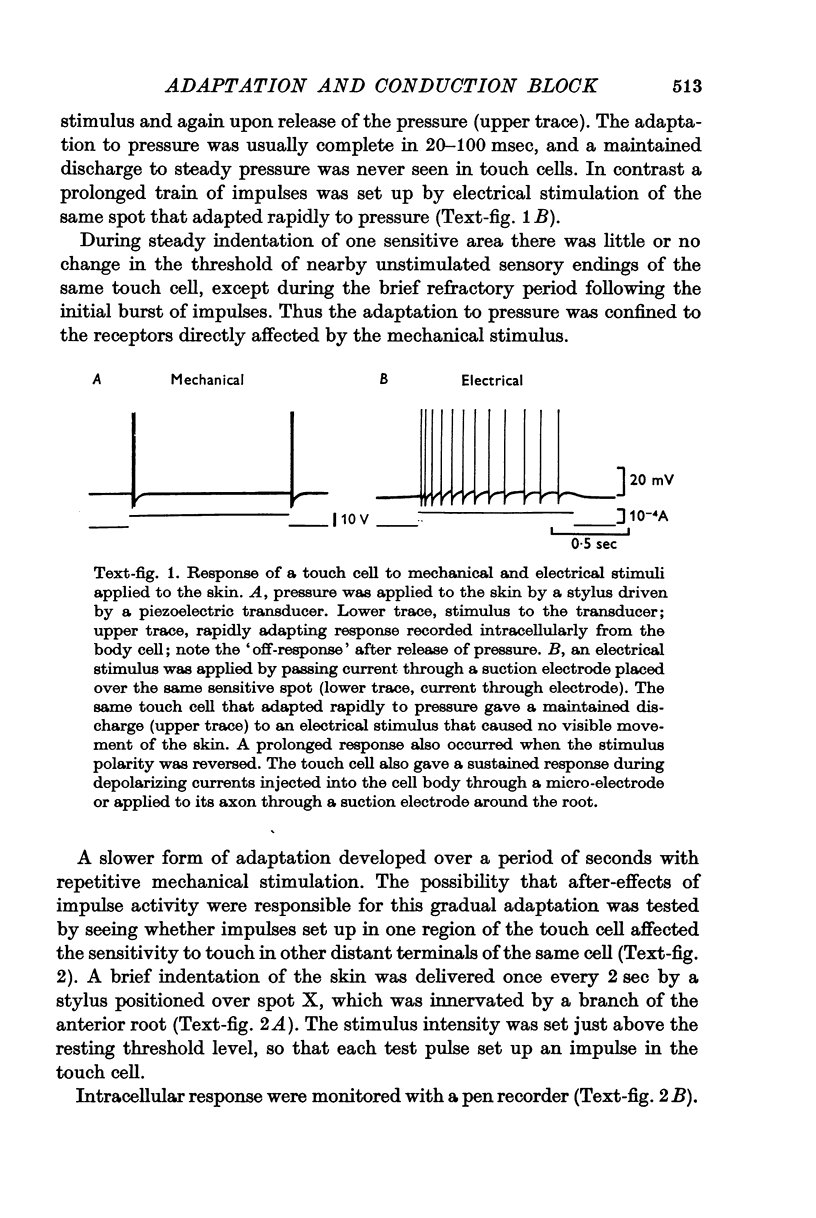
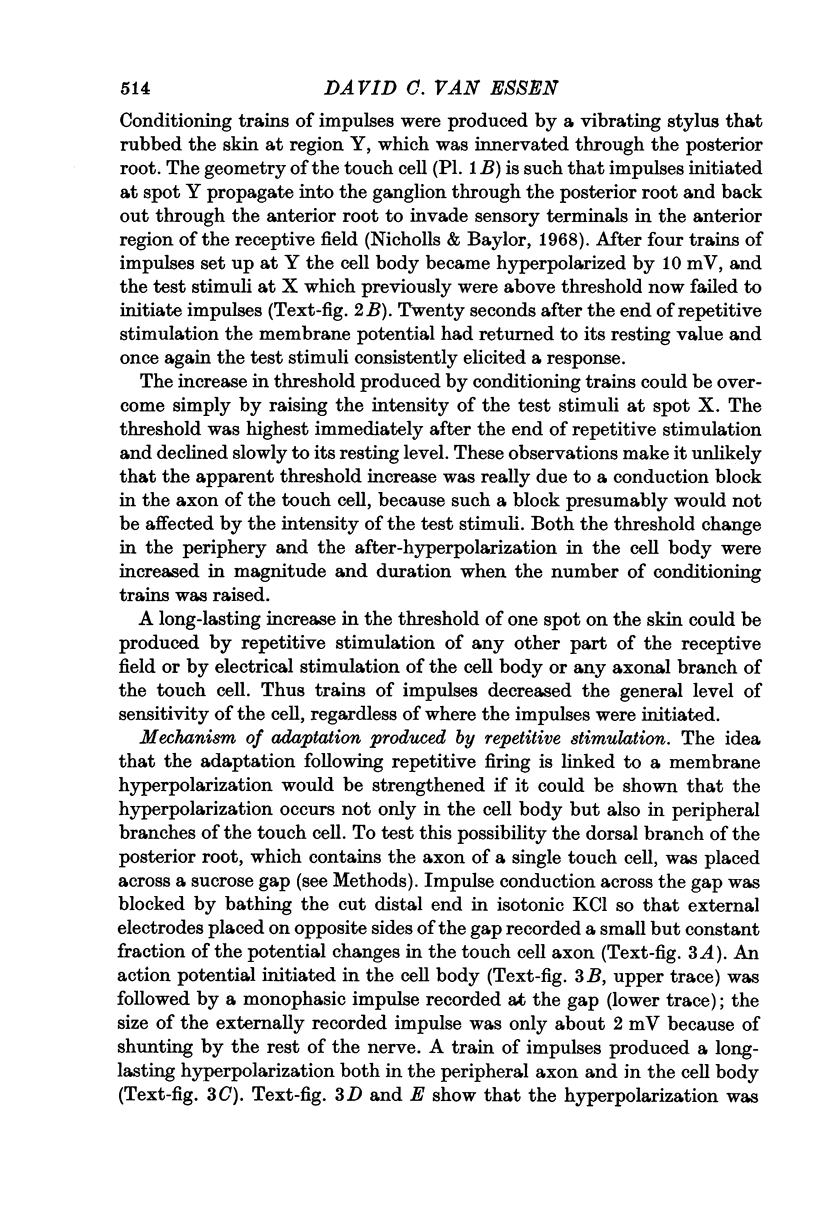
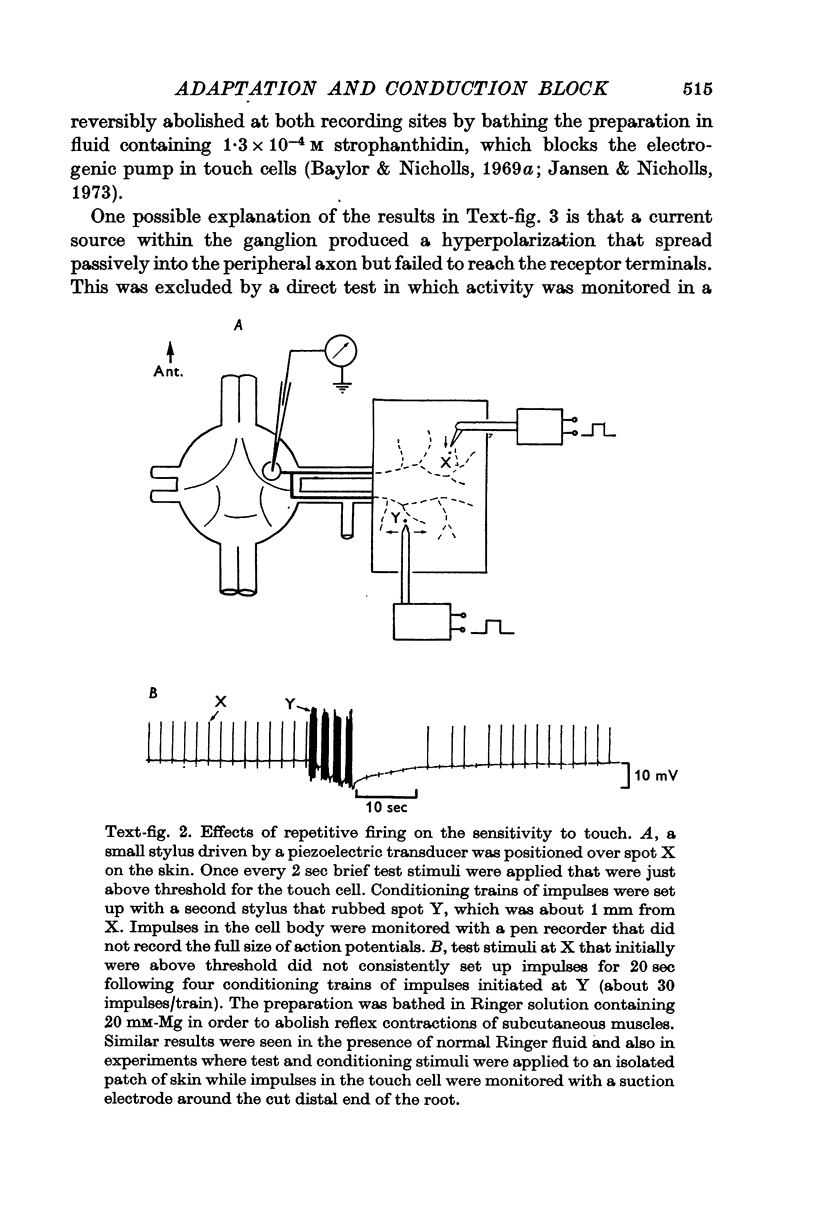
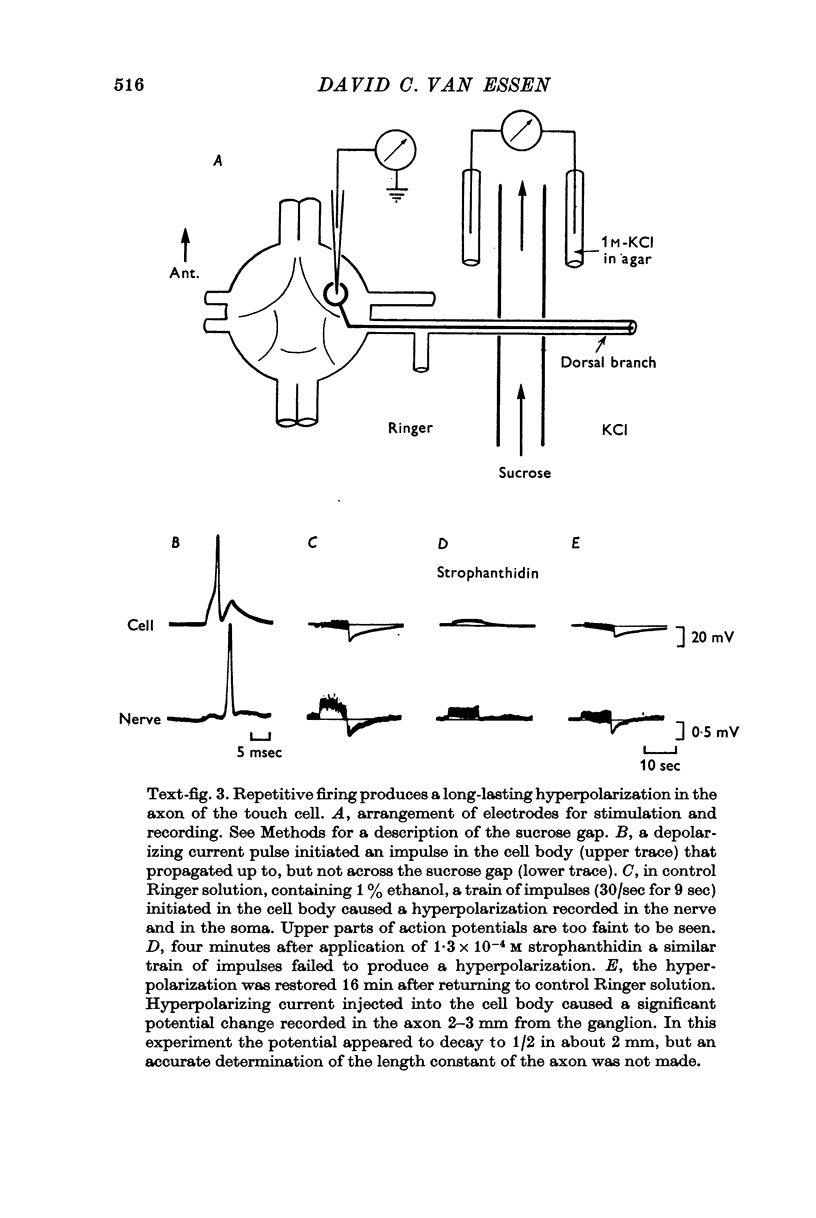
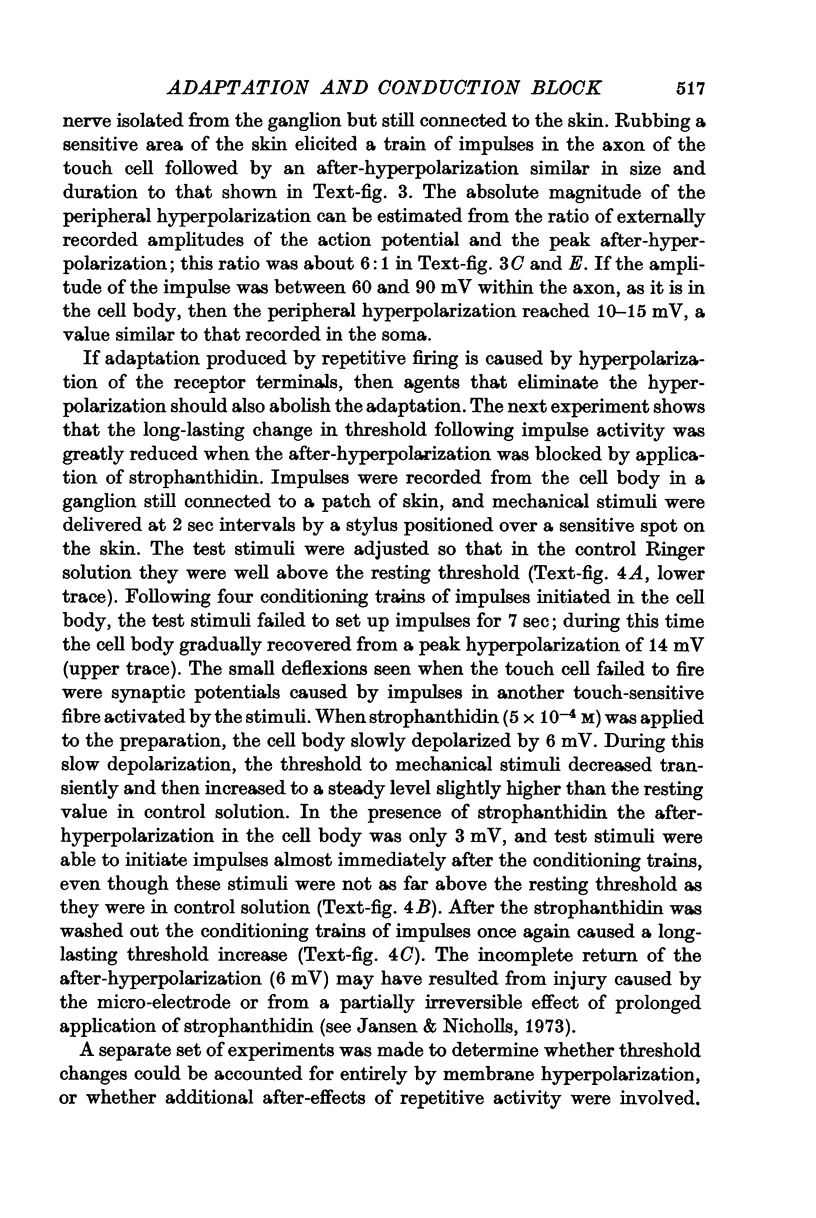
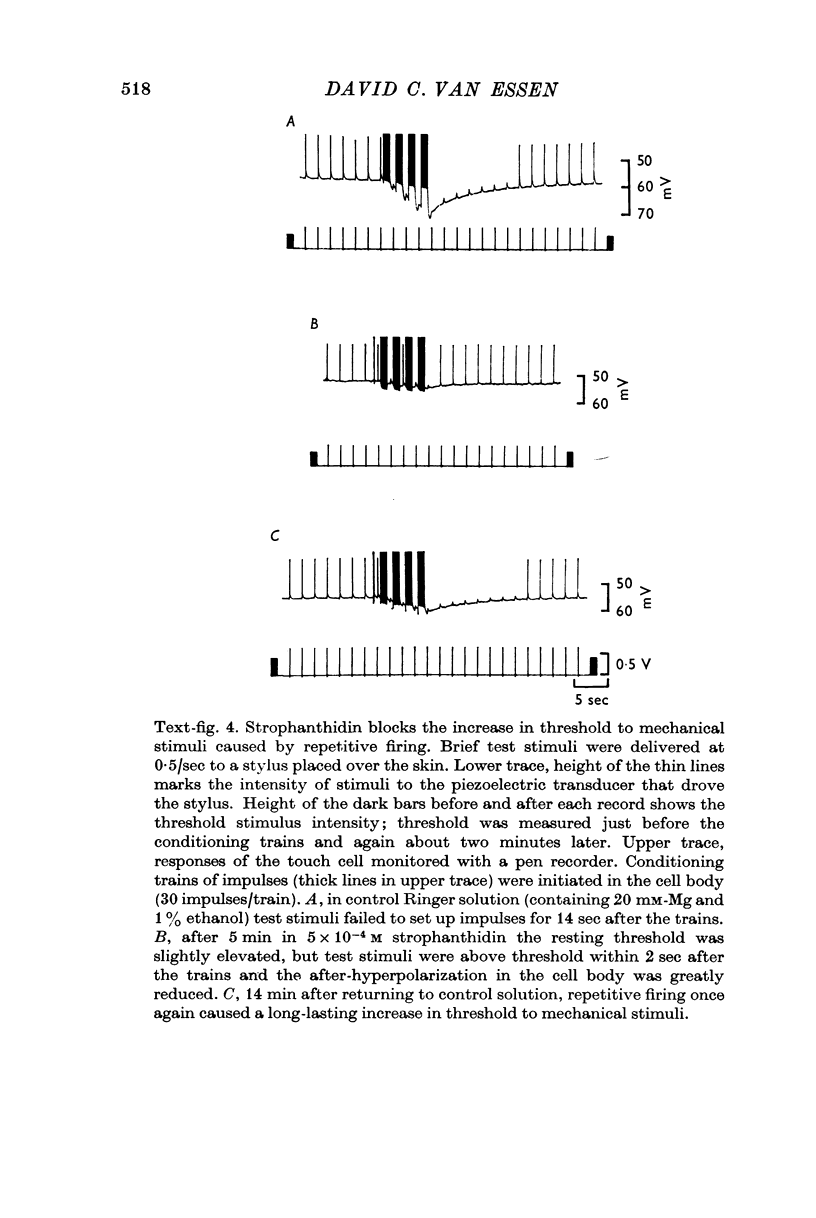
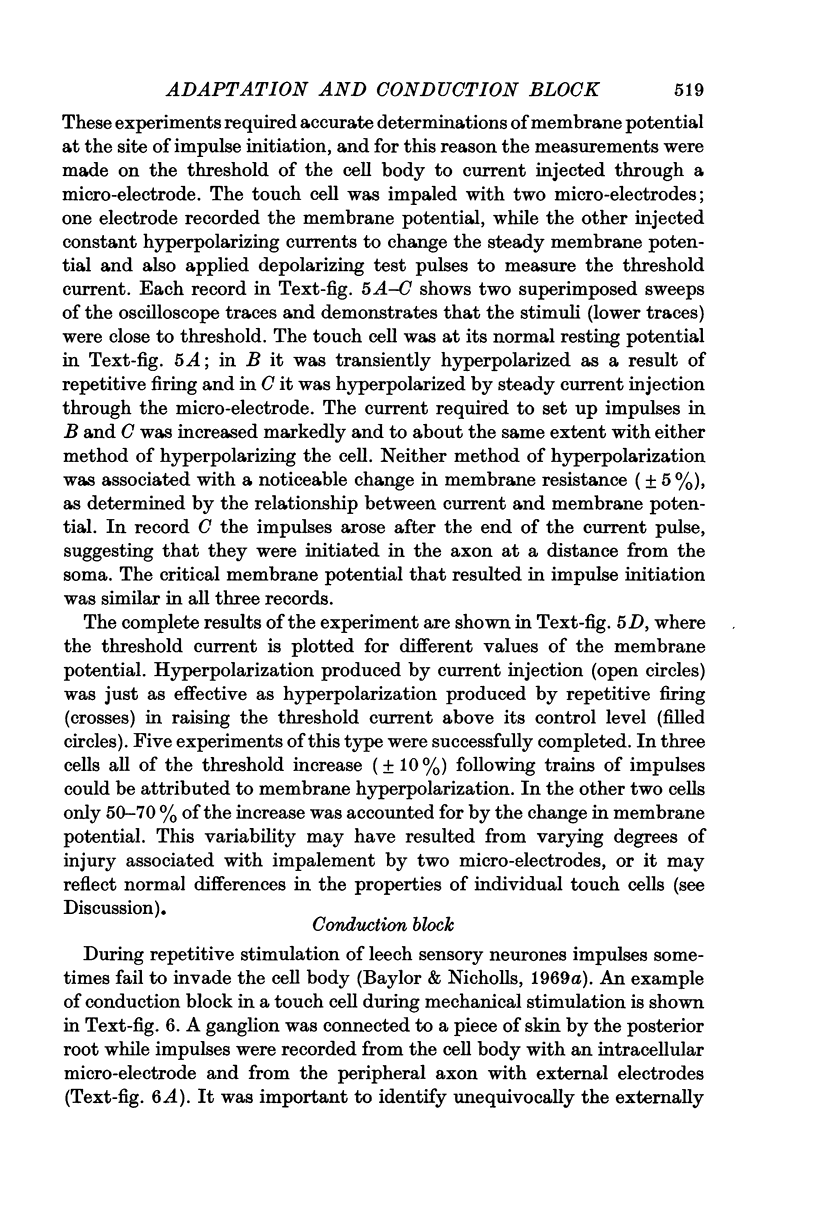
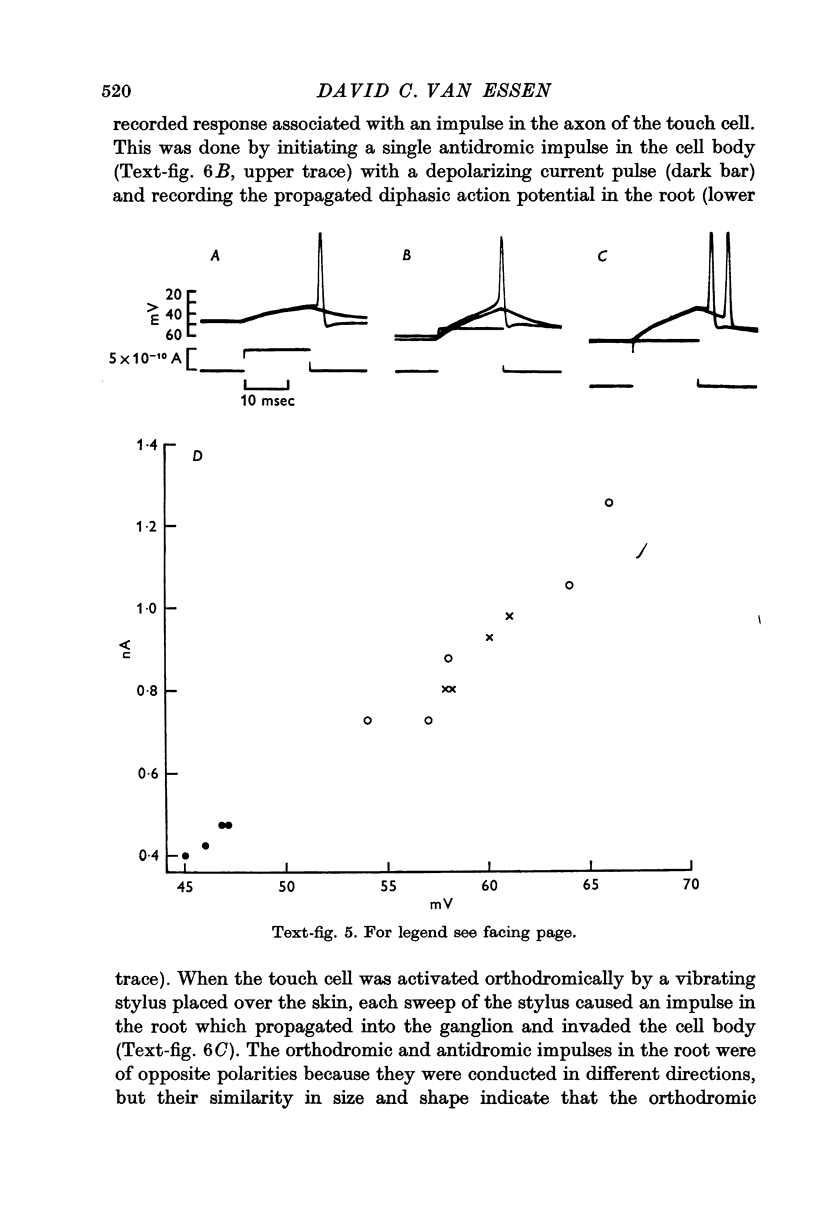
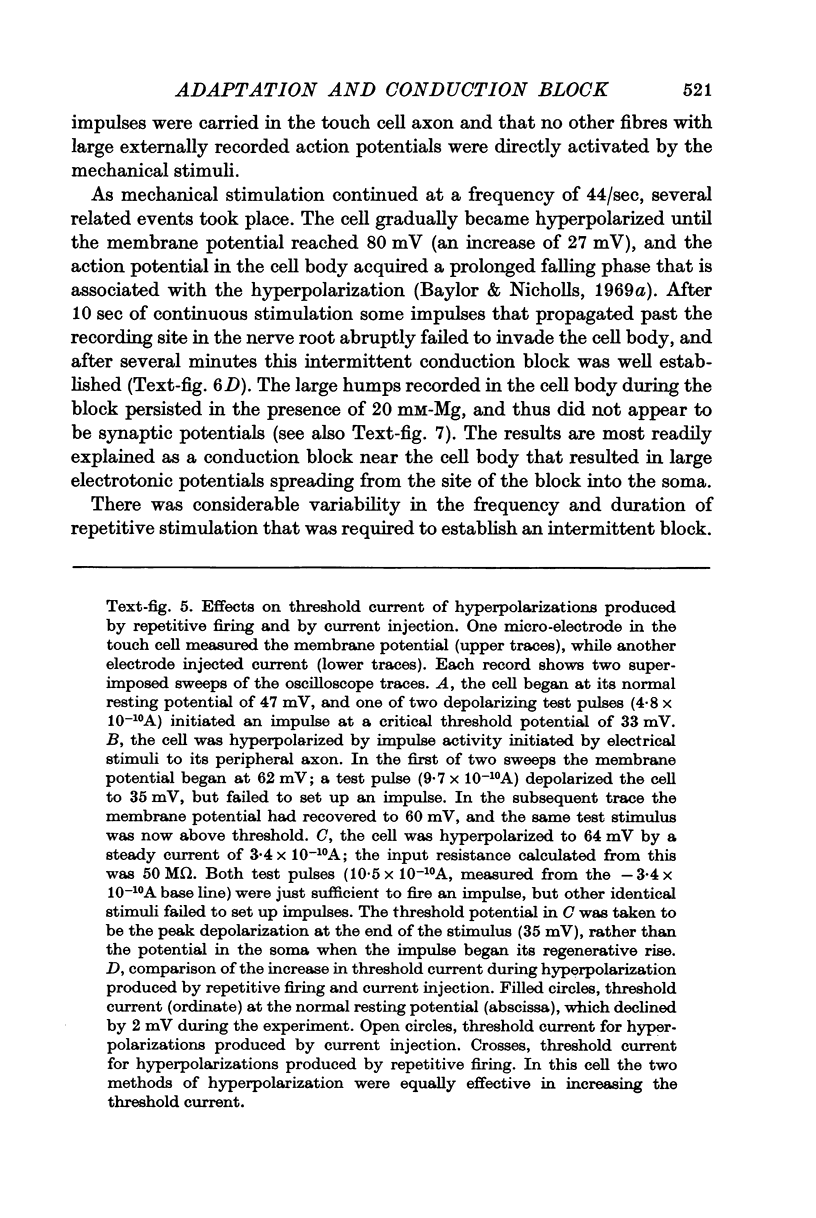
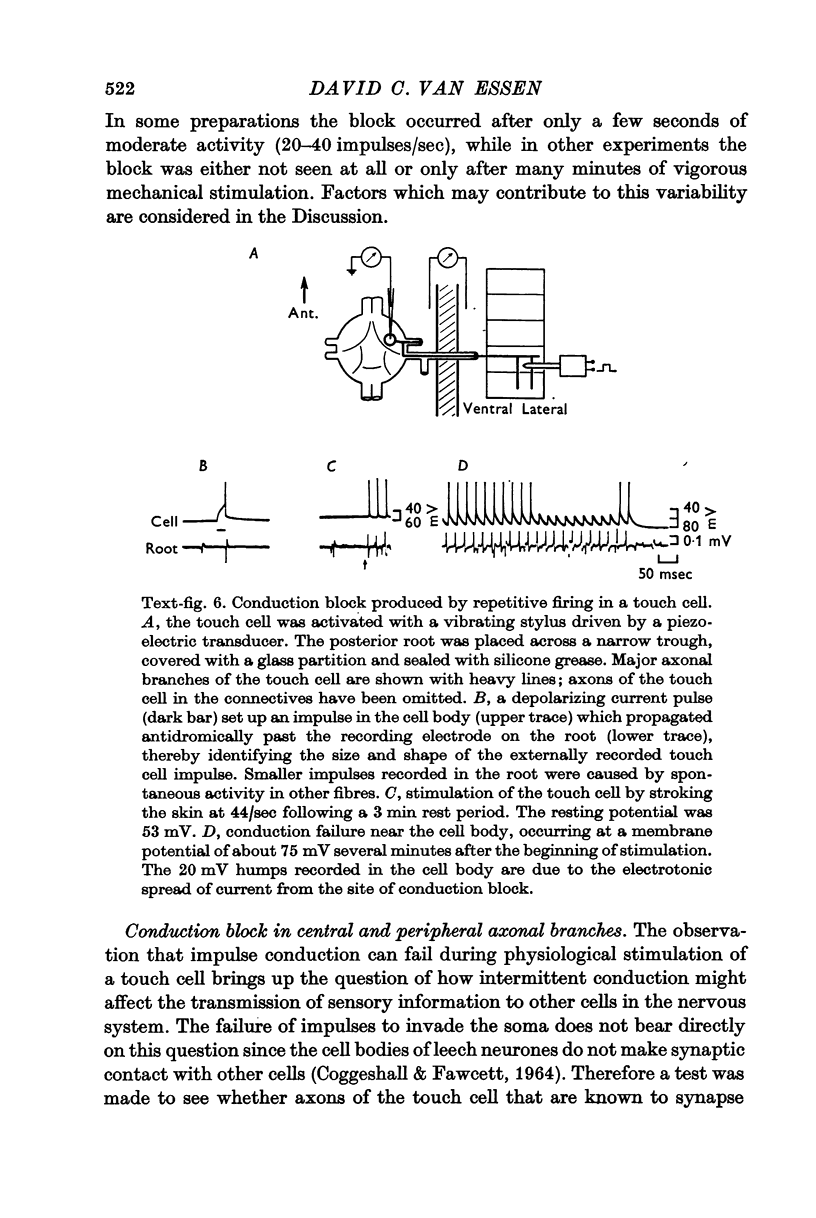
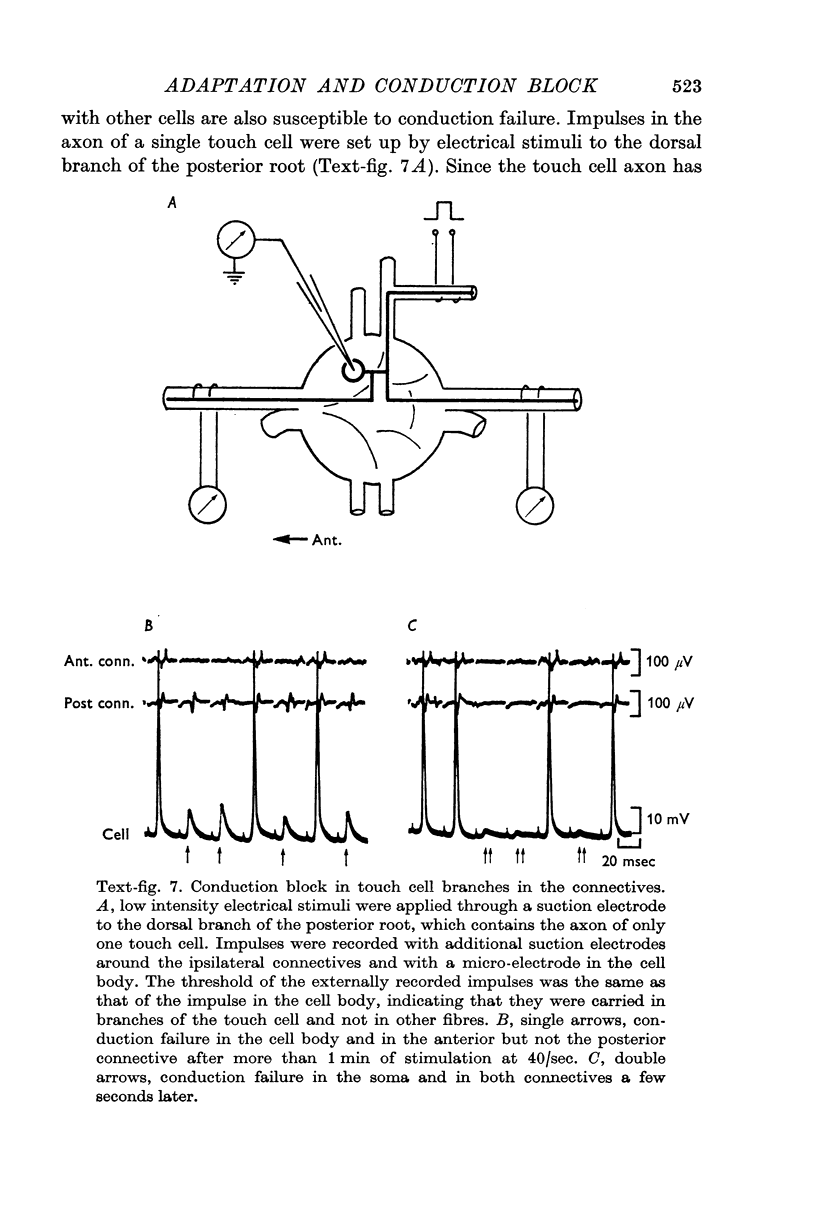
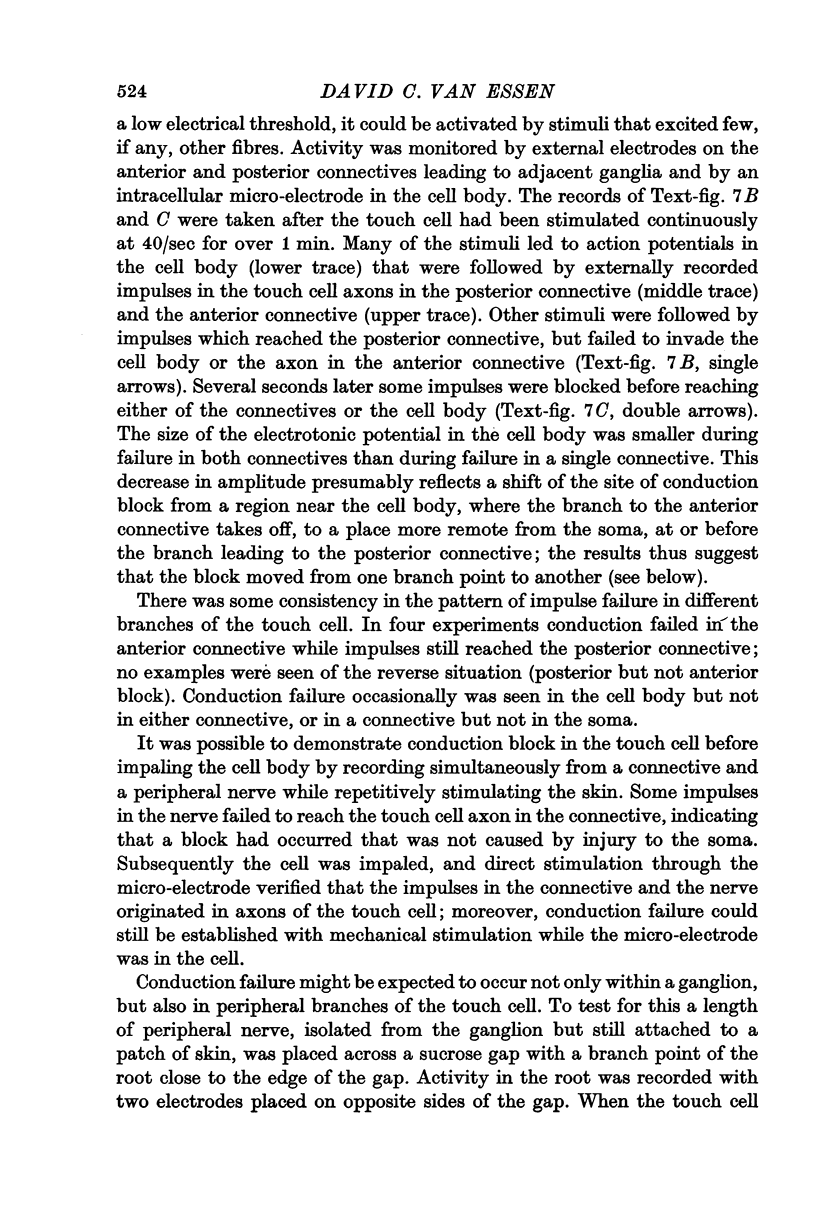
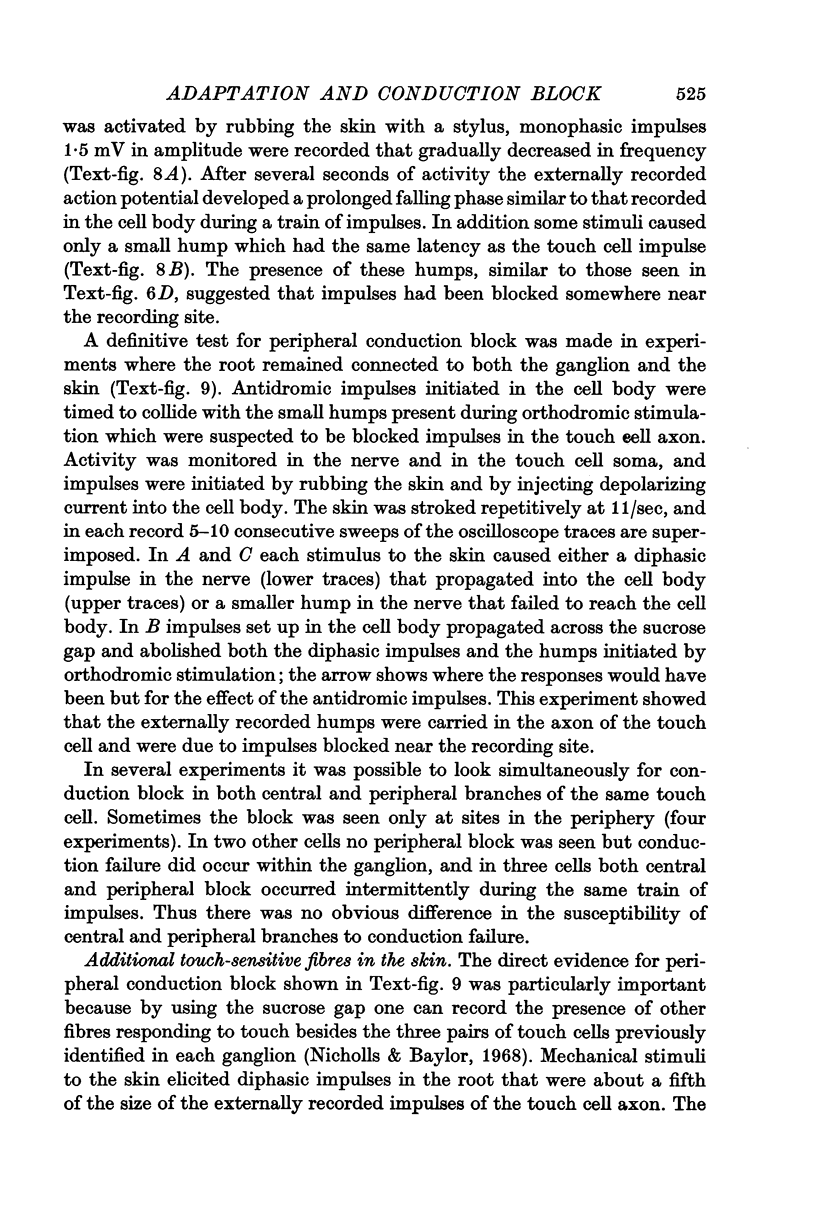
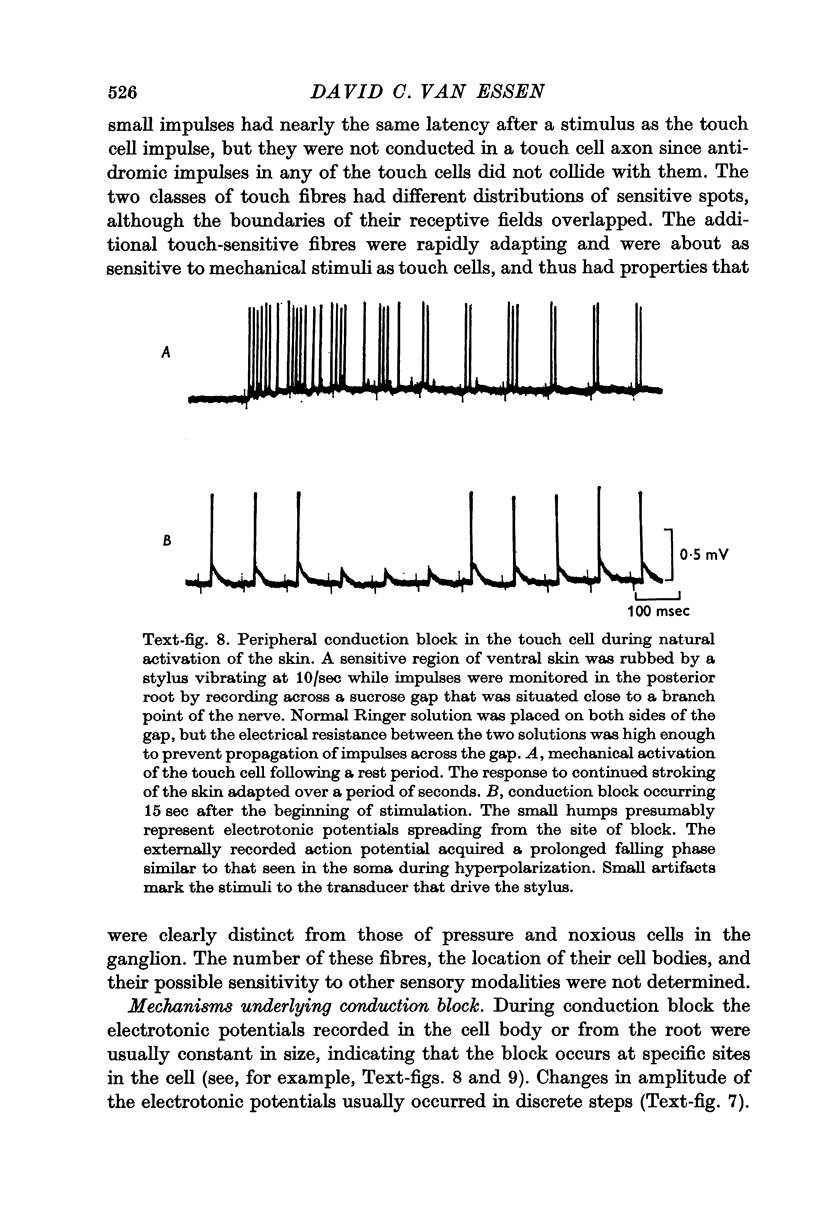
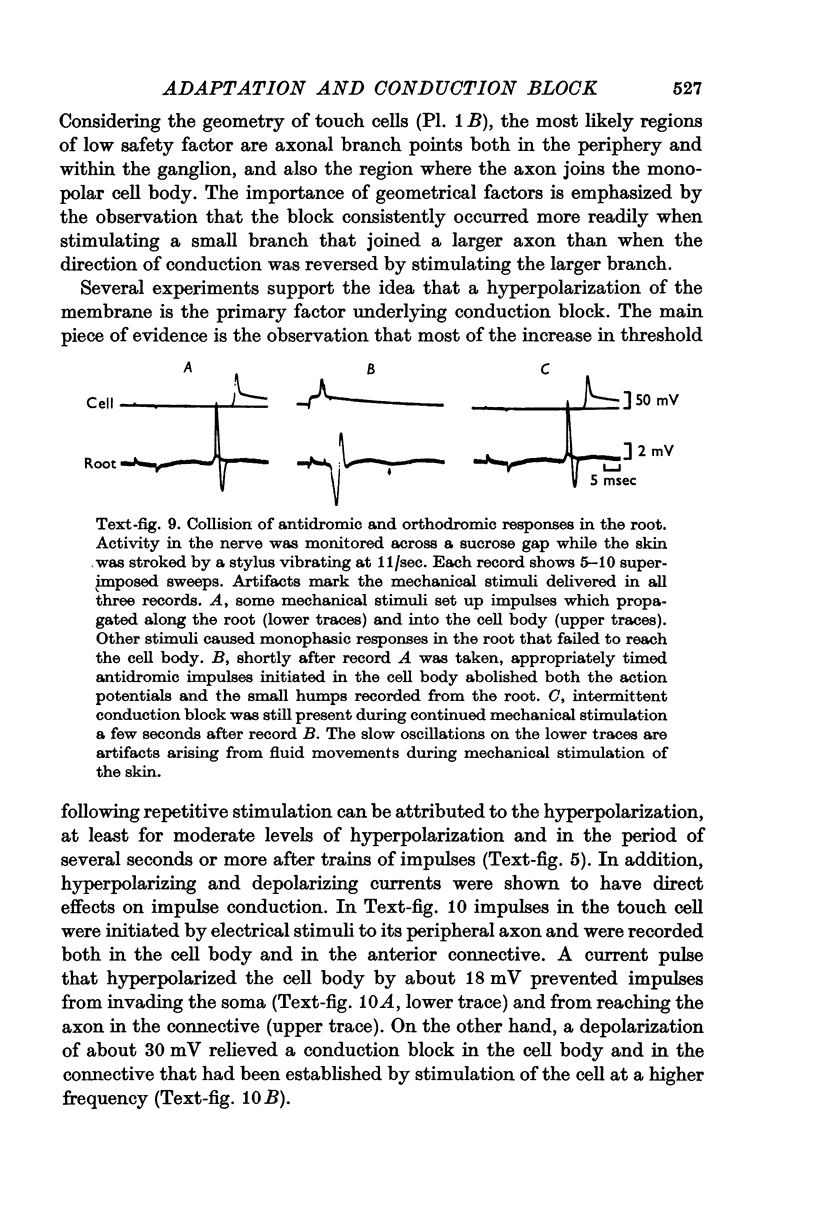
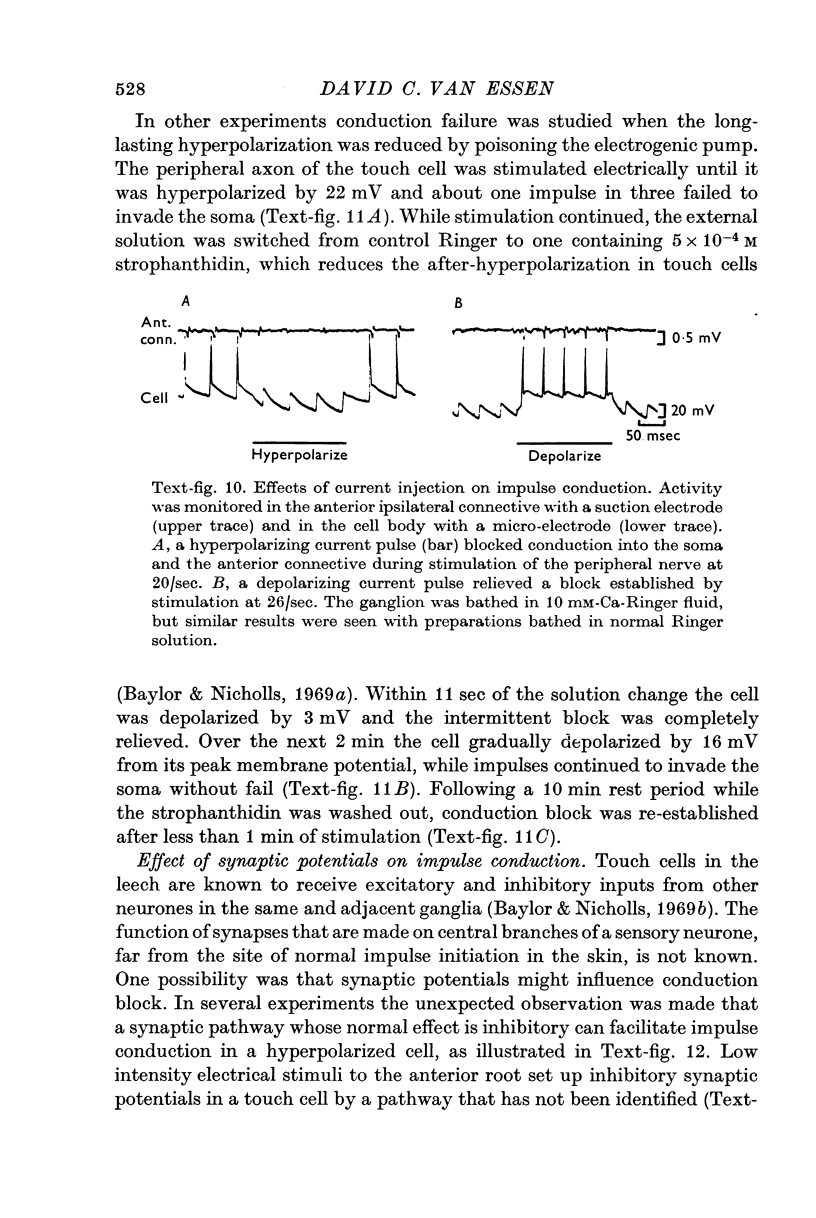
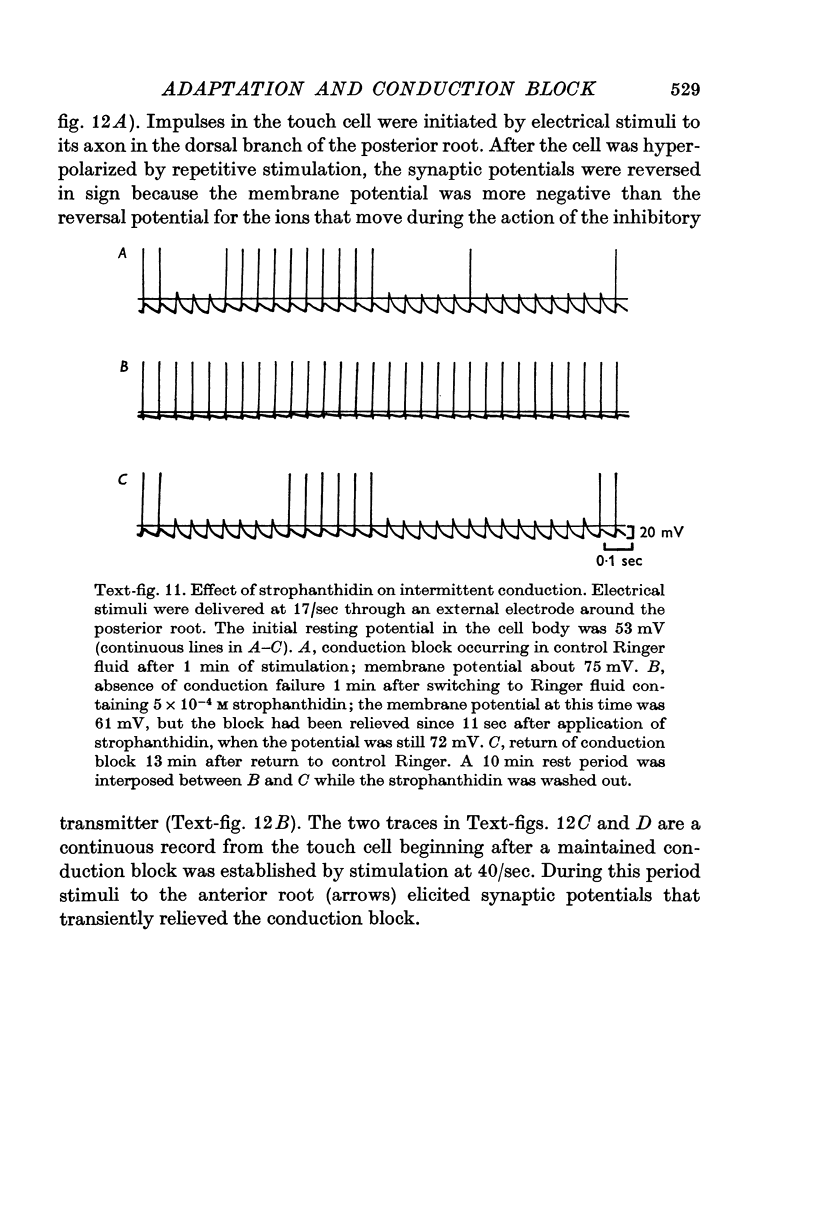
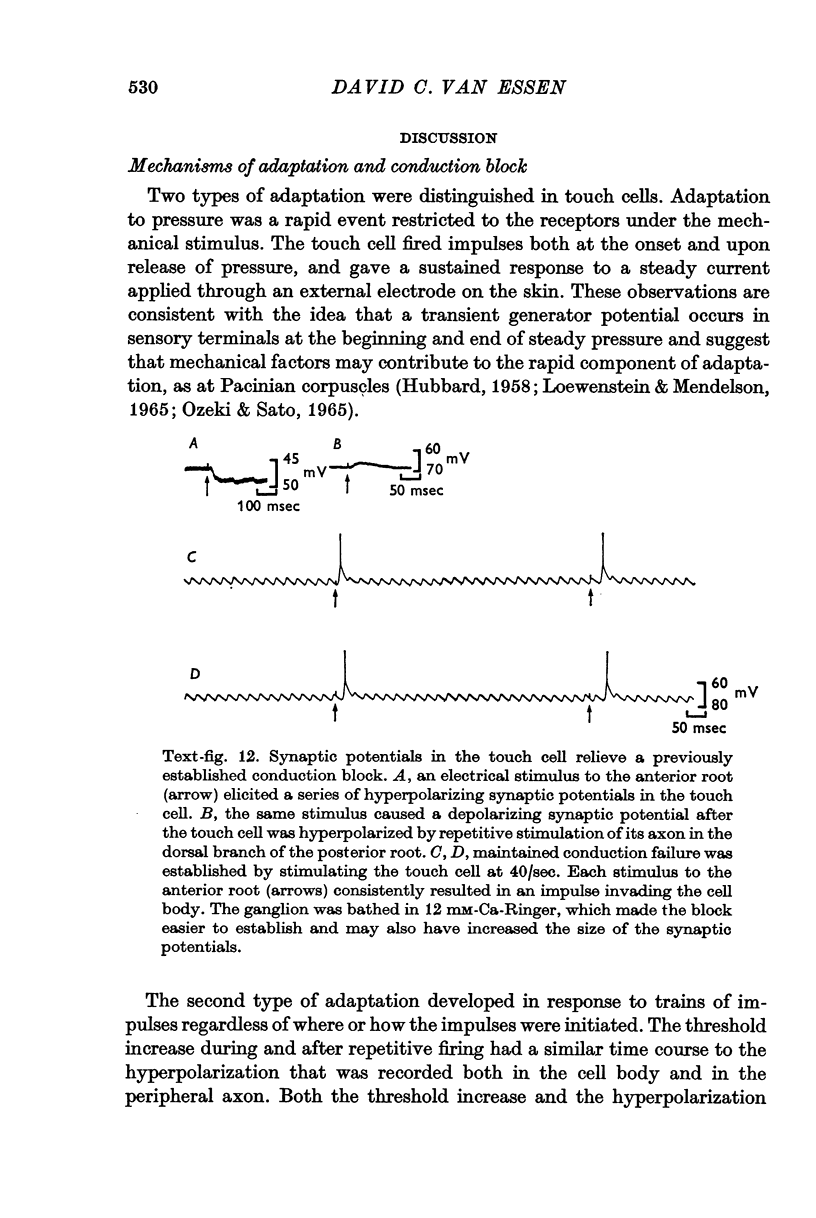
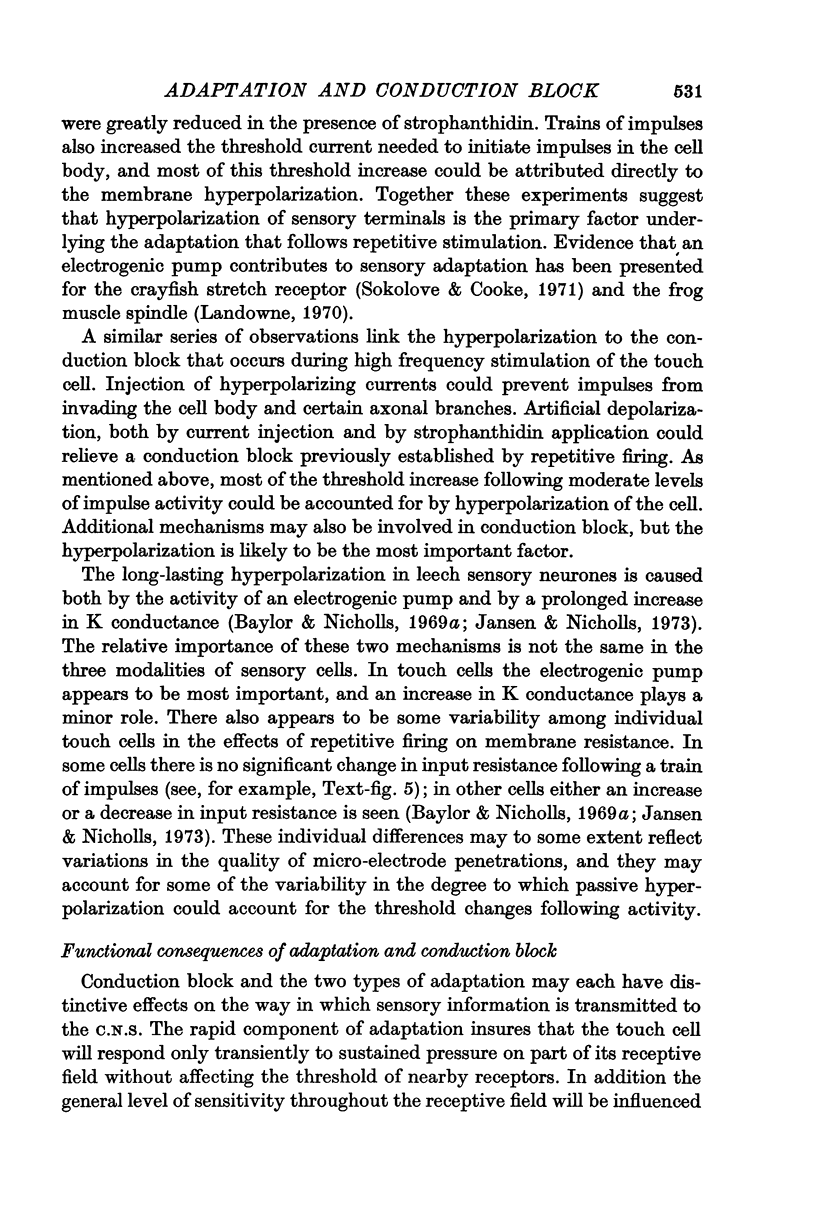
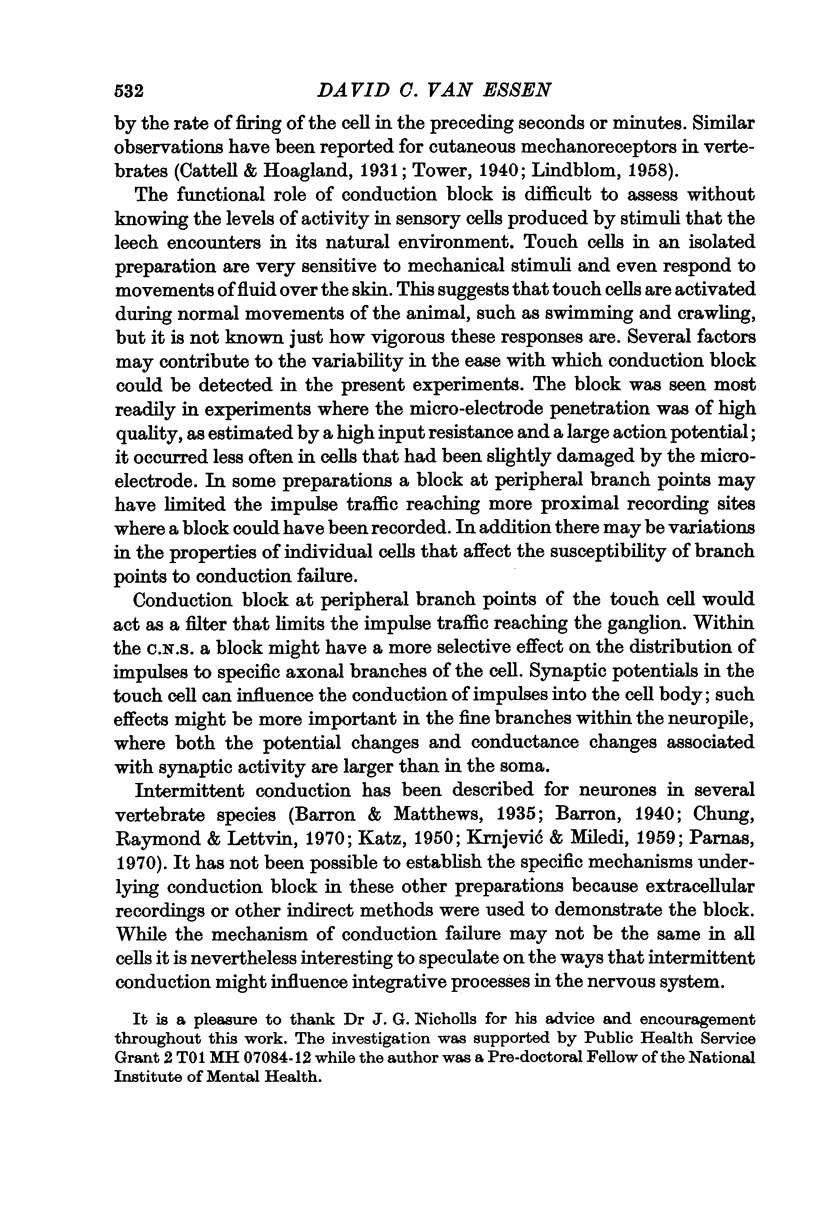
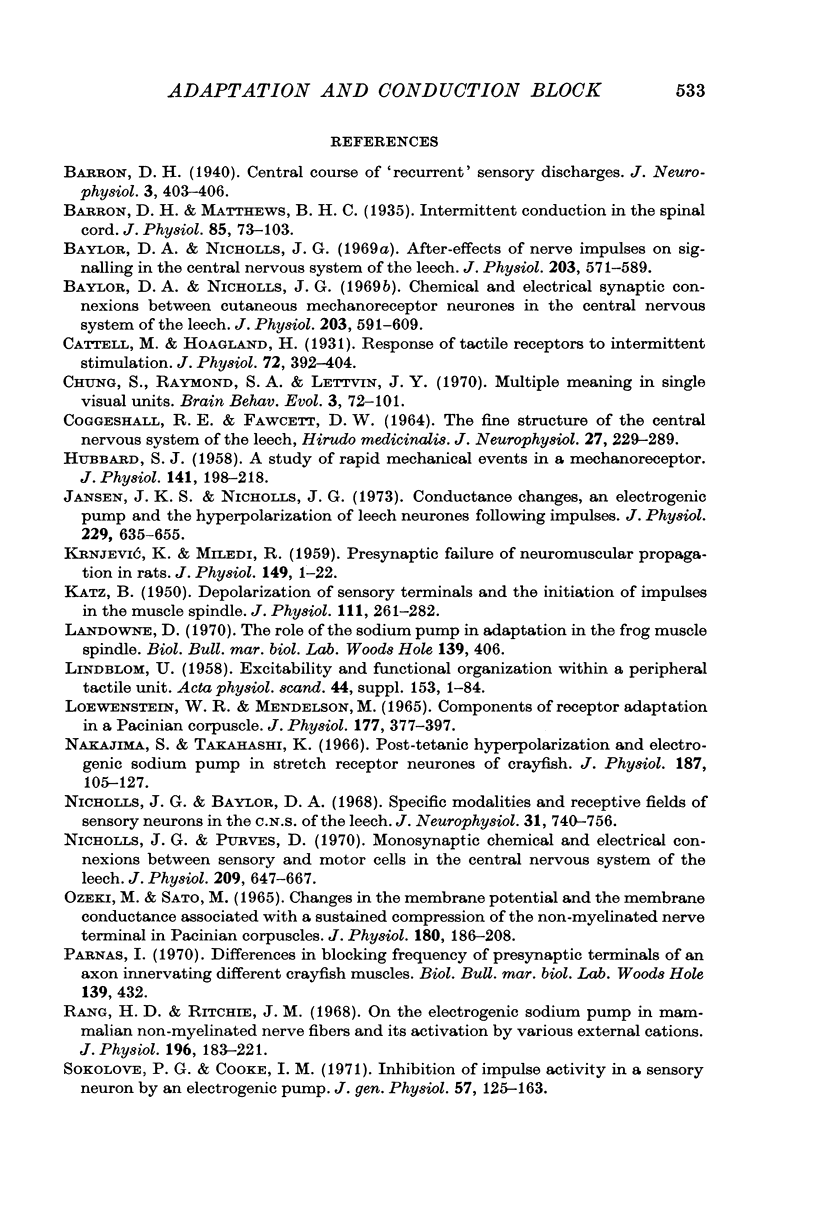
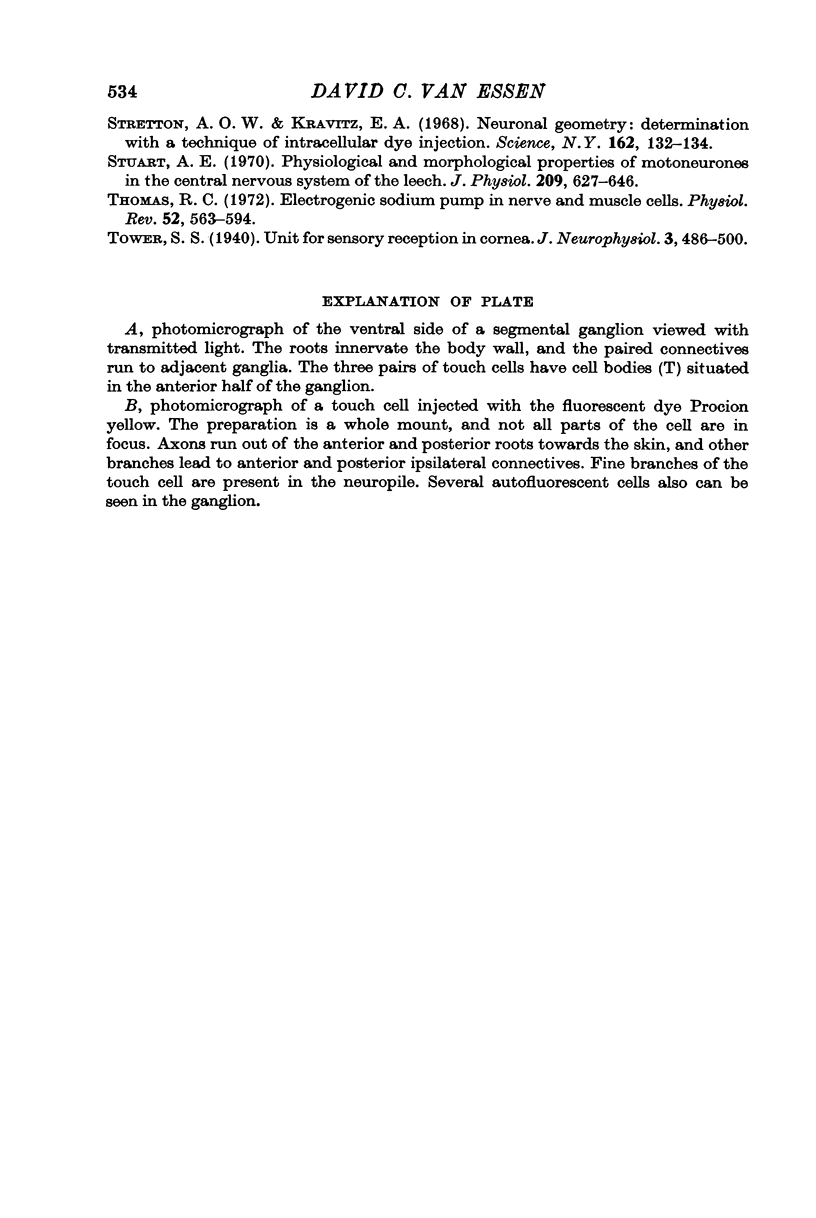
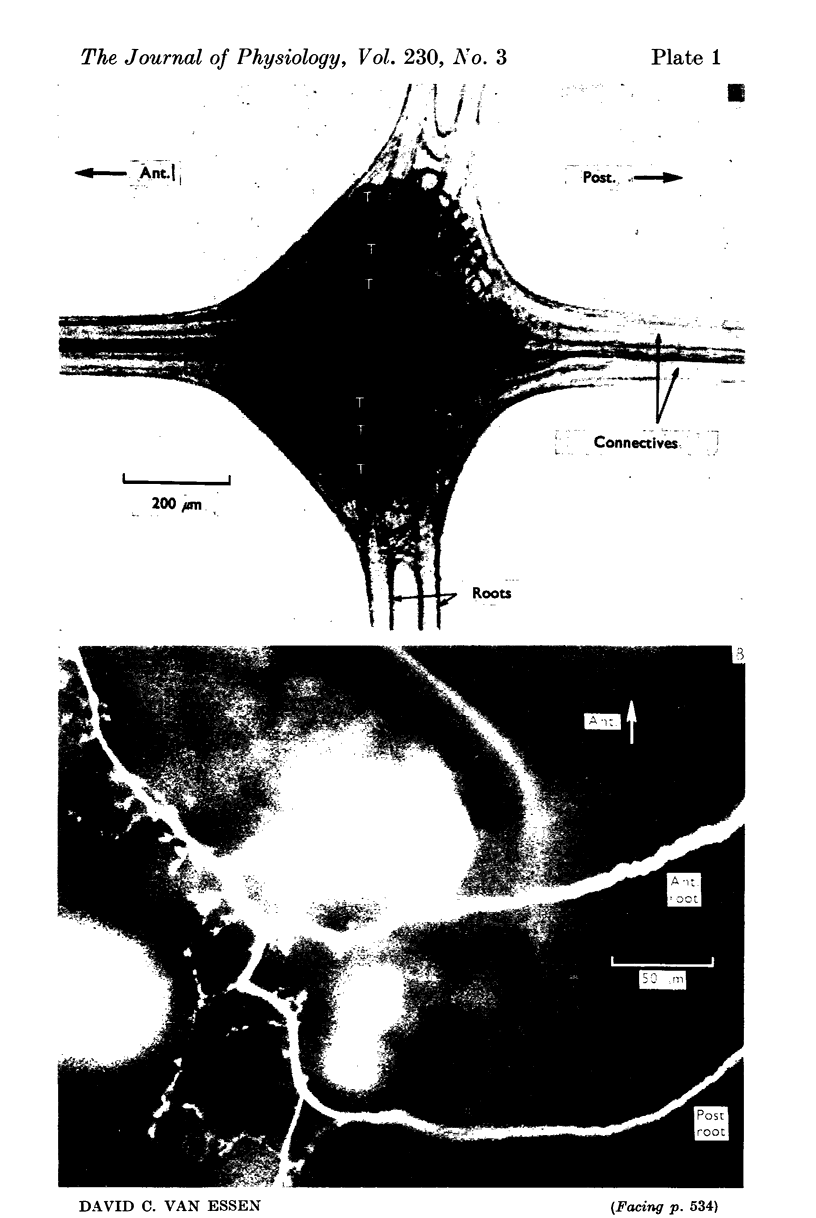
Images in this article
Selected References
These references are in PubMed. This may not be the complete list of references from this article.
- Barron D. H., Matthews B. H. Intermittent conduction in the spinal cord. J Physiol. 1935 Aug 22;85(1):73–103. doi: 10.1113/jphysiol.1935.sp003303. [DOI] [PMC free article] [PubMed] [Google Scholar]
- Baylor D. A., Nicholls J. G. After-effects of nerve impulses on signalling in the central nervous system of the leech. J Physiol. 1969 Aug;203(3):571–589. doi: 10.1113/jphysiol.1969.sp008880. [DOI] [PMC free article] [PubMed] [Google Scholar]
- Baylor D. A., Nicholls J. G. Chemical and electrical synaptic connexions between cutaneous mechanoreceptor neurones in the central nervous system of the leech. J Physiol. 1969 Aug;203(3):591–609. doi: 10.1113/jphysiol.1969.sp008881. [DOI] [PMC free article] [PubMed] [Google Scholar]
- COGGESHALL R. E., FAWCETT D. W. THE FINE STRUCTURE OF THE CENTRAL NERVOUS SYSTEM OF THE LEECH, HIRUDO MEDICINALIS. J Neurophysiol. 1964 Mar;27:229–289. doi: 10.1152/jn.1964.27.2.229. [DOI] [PubMed] [Google Scholar]
- Cattell M., Hoagland H. Response of tactile receptors to intermittent stimulation. J Physiol. 1931 Aug 14;72(4):392–404. doi: 10.1113/jphysiol.1931.sp002782. [DOI] [PMC free article] [PubMed] [Google Scholar]
- Chung S. H., Raymond S. A., Lettvin J. Y. Multiple meaning in single visual units. Brain Behav Evol. 1970;3(1):72–101. doi: 10.1159/000125464. [DOI] [PubMed] [Google Scholar]
- HUBBARD S. J. A study of rapid mechanical events in a mechanoreceptor. J Physiol. 1958 Apr 30;141(2):198–218. doi: 10.1113/jphysiol.1958.sp005968. [DOI] [PMC free article] [PubMed] [Google Scholar]
- Jansen J. K., Nicholls J. G. Conductance changes, an electrogenic pump and the hyperpolarization of leech neurones following impulses. J Physiol. 1973 Mar;229(3):635–655. doi: 10.1113/jphysiol.1973.sp010158. [DOI] [PMC free article] [PubMed] [Google Scholar]
- KATZ B. Depolarization of sensory terminals and the initiation of impulses in the muscle spindle. J Physiol. 1950 Oct 16;111(3-4):261–282. doi: 10.1113/jphysiol.1950.sp004479. [DOI] [PMC free article] [PubMed] [Google Scholar]
- KRNJEVIC K., MILEDI R. Presynaptic failure of neuromuscular propagation in rats. J Physiol. 1959 Dec;149:1–22. doi: 10.1113/jphysiol.1959.sp006321. [DOI] [PMC free article] [PubMed] [Google Scholar]
- LOEWENSTEIN W. R., MENDELSON M. COMPONENTS OF RECEPTOR ADAPTATION IN A PACINIAN CORPUSCLE. J Physiol. 1965 Apr;177:377–397. doi: 10.1113/jphysiol.1965.sp007598. [DOI] [PMC free article] [PubMed] [Google Scholar]
- Nakajima S., Takahashi K. Post-tetanic hyperpolarization and electrogenic Na pump in stretch receptor neurone of crayfish. J Physiol. 1966 Nov;187(1):105–127. doi: 10.1113/jphysiol.1966.sp008078. [DOI] [PMC free article] [PubMed] [Google Scholar]
- Nicholls J. G., Baylor D. A. Specific modalities and receptive fields of sensory neurons in CNS of the leech. J Neurophysiol. 1968 Sep;31(5):740–756. doi: 10.1152/jn.1968.31.5.740. [DOI] [PubMed] [Google Scholar]
- Nicholls J. G., Purves D. Monosynaptic chemical and electrical connexions between sensory and motor cells in the central nervous system of the leech. J Physiol. 1970 Aug;209(3):647–667. doi: 10.1113/jphysiol.1970.sp009184. [DOI] [PMC free article] [PubMed] [Google Scholar]
- Ozeki M., Sato M. Changes in the membrane potential and the membrane conductance associated with a sustained compression of the non-myelinated nerve terminal in Pacinian corpuscles. J Physiol. 1965 Sep;180(1):186–208. [PMC free article] [PubMed] [Google Scholar]
- Rang H. P., Ritchie J. M. On the electrogenic sodium pump in mammalian non-myelinated nerve fibres and its activation by various external cations. J Physiol. 1968 May;196(1):183–221. doi: 10.1113/jphysiol.1968.sp008502. [DOI] [PMC free article] [PubMed] [Google Scholar]
- Sokolove P. G., Cooke I. M. Inhibition of impulse activity in a sensory neuron by an electrogenic pump. J Gen Physiol. 1971 Feb;57(2):125–163. doi: 10.1085/jgp.57.2.125. [DOI] [PMC free article] [PubMed] [Google Scholar]
- Stretton A. O., Kravitz E. A. Neuronal geometry: determination with a technique of intracellular dye injection. Science. 1968 Oct 4;162(3849):132–134. doi: 10.1126/science.162.3849.132. [DOI] [PubMed] [Google Scholar]
- Stuart A. E. Physiological and morphological properties of motoneurones in the central nervous system of the leech. J Physiol. 1970 Aug;209(3):627–646. doi: 10.1113/jphysiol.1970.sp009183. [DOI] [PMC free article] [PubMed] [Google Scholar]
- Thomas R. C. Electrogenic sodium pump in nerve and muscle cells. Physiol Rev. 1972 Jul;52(3):563–594. doi: 10.1152/physrev.1972.52.3.563. [DOI] [PubMed] [Google Scholar]