Abstract
1. At high internal K concentrations the efflux of Na from red cells increases with internal Na concentration following an S-shaped curve. As internal K is reduced the S-shaped region and the value of internal Na for which the Na efflux is half-maximal are both shifted progressively towards zero.
2. The effects of internal Na on the shape of the Na efflux curves can be quantitatively accounted for if it is assumed that the rate of Na efflux is linearly related to the number of pump units having three identical and non-interacting sites occupied by Na.
3. The effects of internal K on the shape of the Na efflux curves are fully explained if it is assumed that the inner sites for Na of the Na pump also behave as identical and non-interacting sites for internal K, being the K-carrier complexes unable to promote Na translocation. The apparent affinity of the Na pump for internal K is about 50 times less than for internal Na.
4. Internal K not only alters the apparent affinity of the Na pump for Na, but also affects its turnover rate. The turnover rate of Na: K exchange increases with internal K following a curve which saturates at about 30 mM internal K. The turnover rate for Na:Na exchange increases linearly with internal K.
5. The linear dependence of the rate of Na:Na exchange on internal K explains why, when internal Na is increased at the expense of internal K, the rate of Na:Na exchange progressively decreases after passing through a maximum.
6. The effects of external Na on the rate of Na:Na exchange can be satisfactorily explained assuming that they are due to the occupation by external Na of three identical and non-interacting sites on each pump unit. The apparent affinity of the Na pump for external Na is about 160 times less than the apparent affinity for internal Na.
7. Under all the experimental conditions tested, it was found that the relation between flux and cation concentration at one of the surfaces of the cell membrane is altered only by a constant factor by changes in the cation composition at the opposite surface of the cell membrane. This fact strongly suggests that there are no interactions between the inner and outer sites of the Na pump.
8. The effects of inner and outer cations on both the Na:K and the Na:Na exchanges catalysed by the Na pump suggest that cation fluxes are proportional to the number of pump units having its inner and outer sites simultaneously occupied by the relevant cations. It seems therefore that sequential models for ion transort do not provide an adequate description of the molecular mechanism of active transport in red cells.
Full text
PDF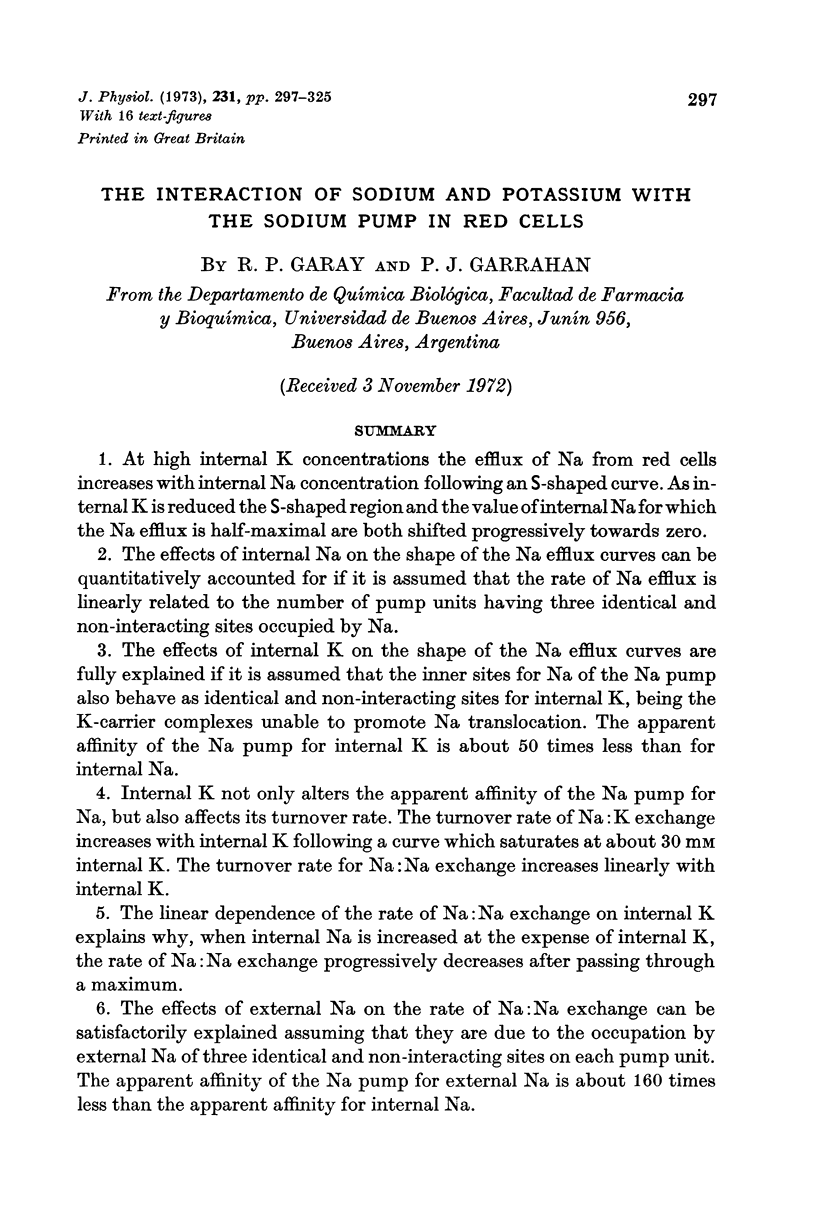
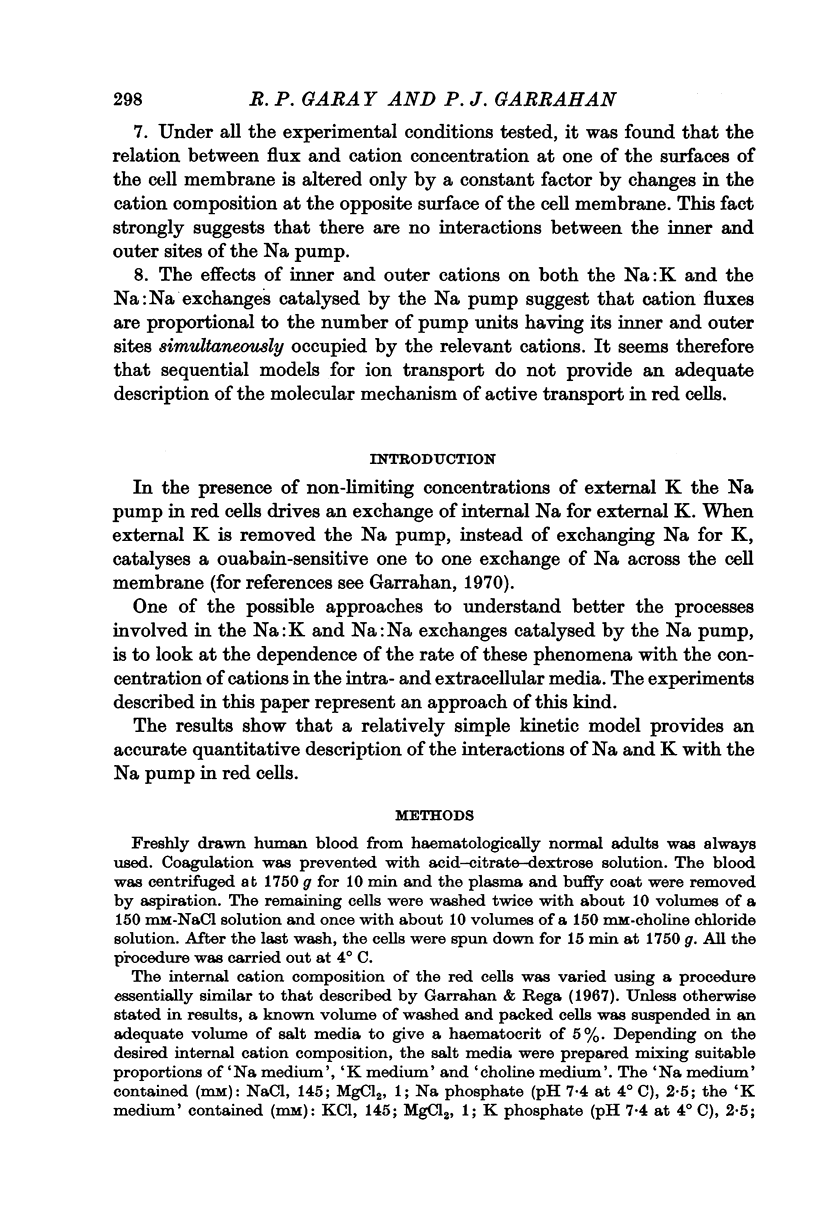
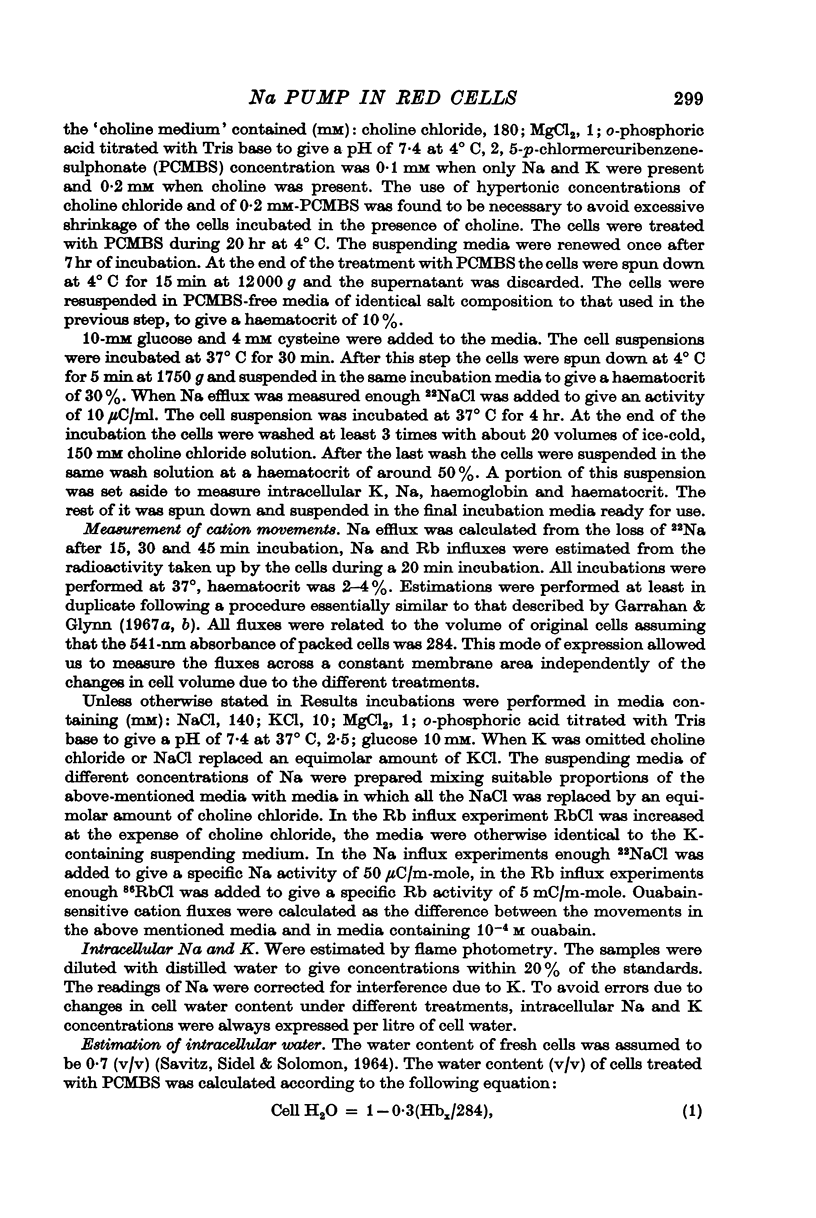
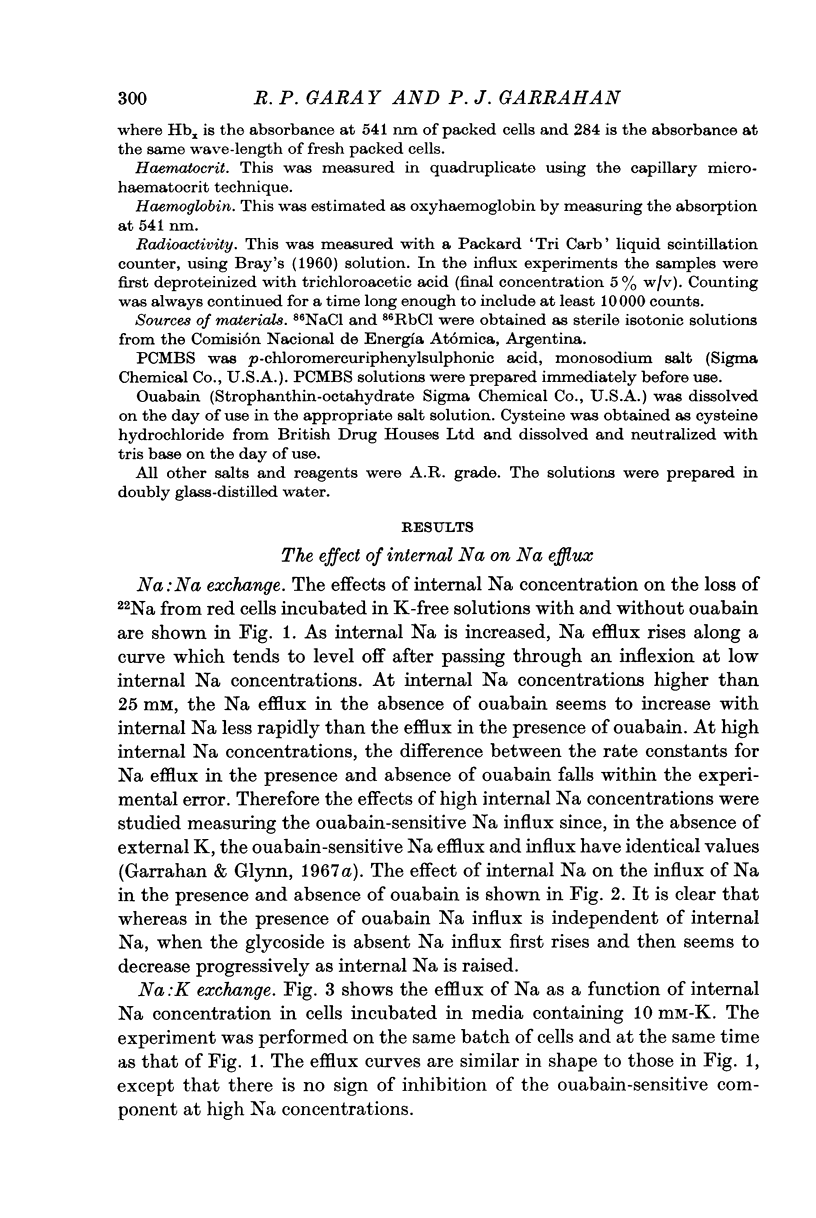
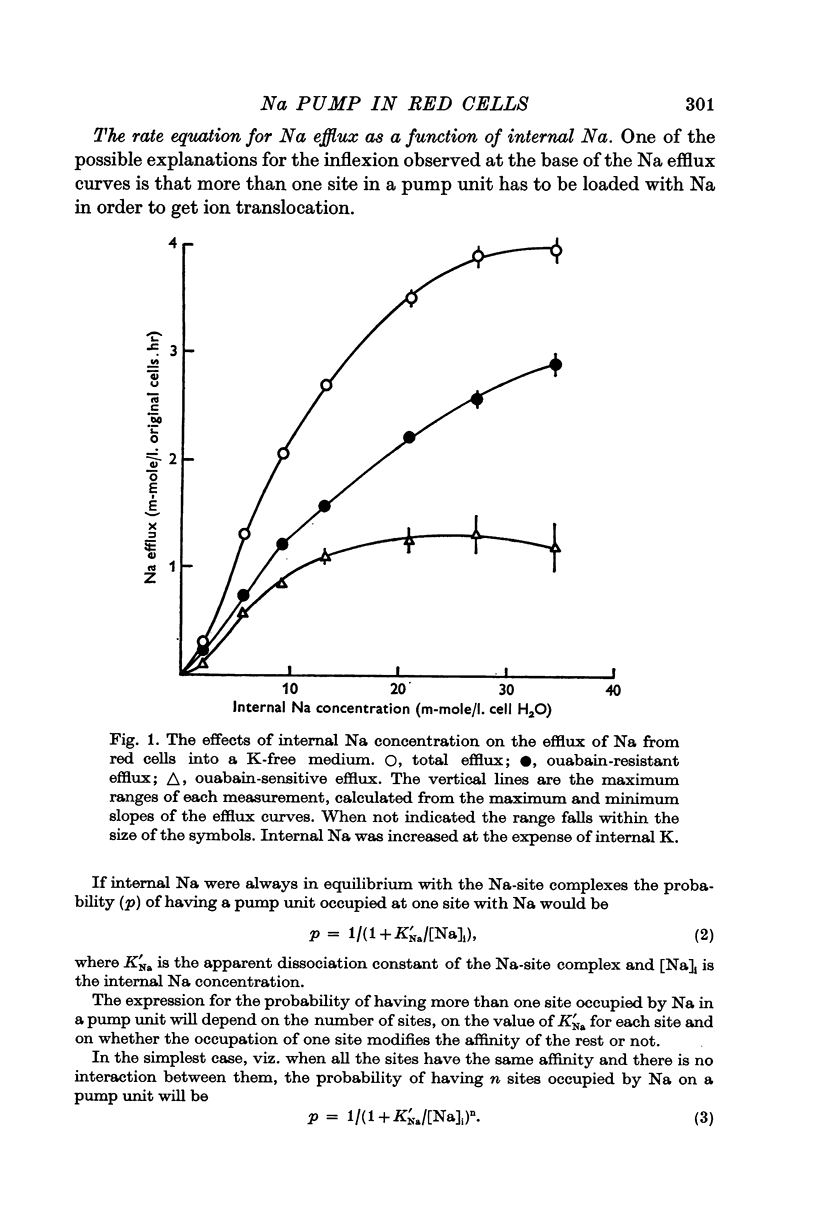
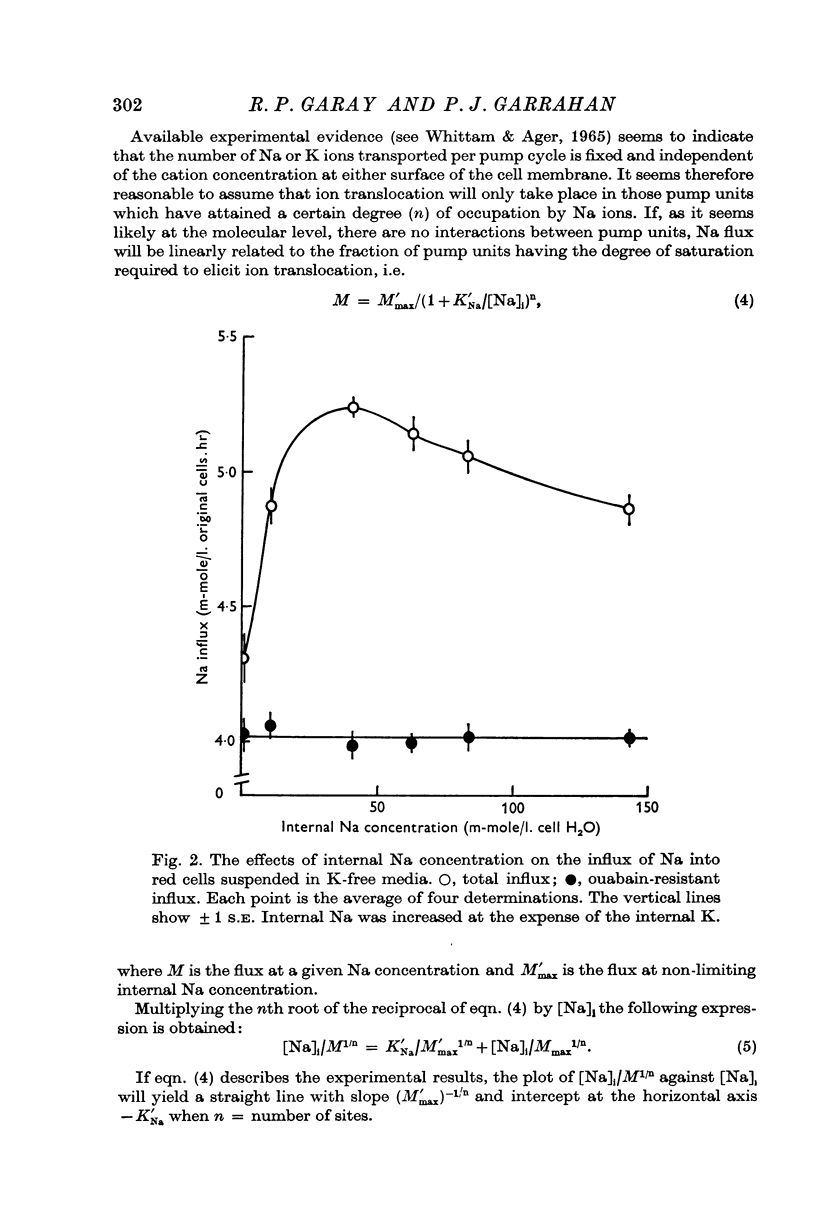
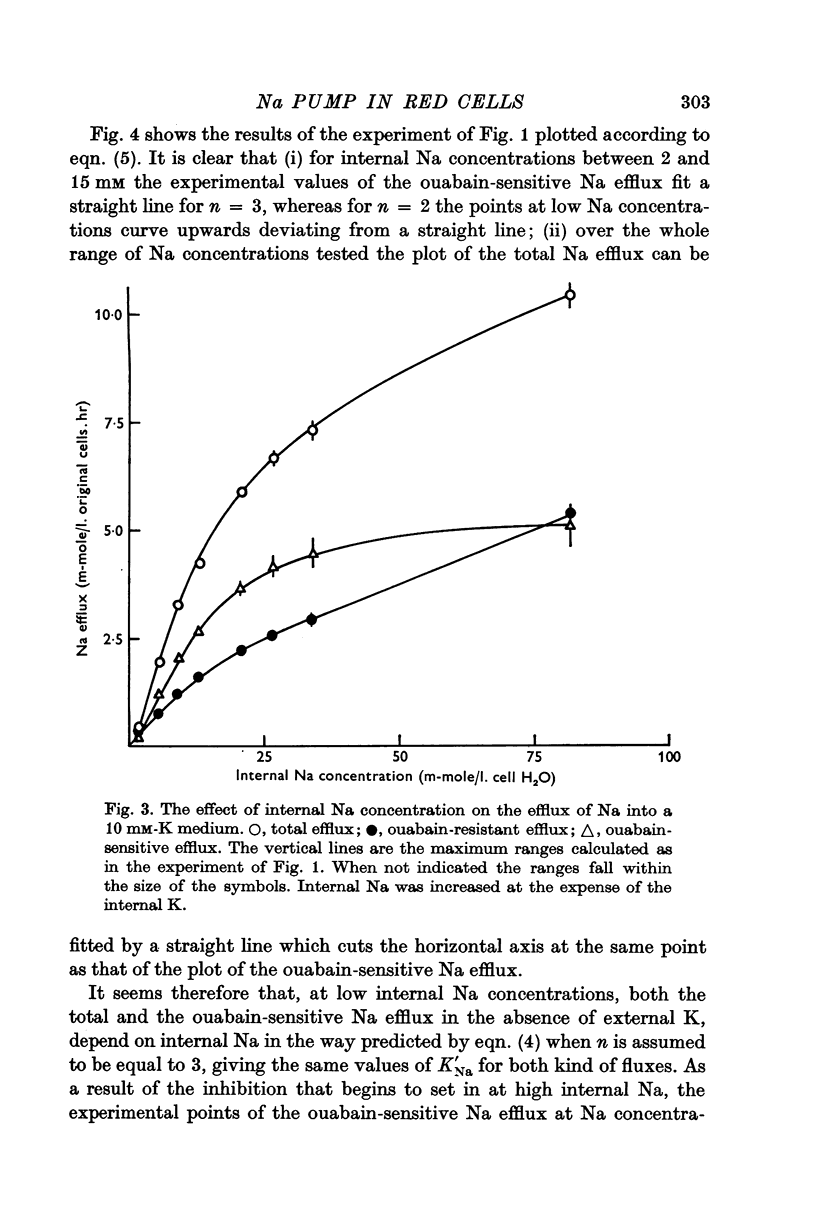
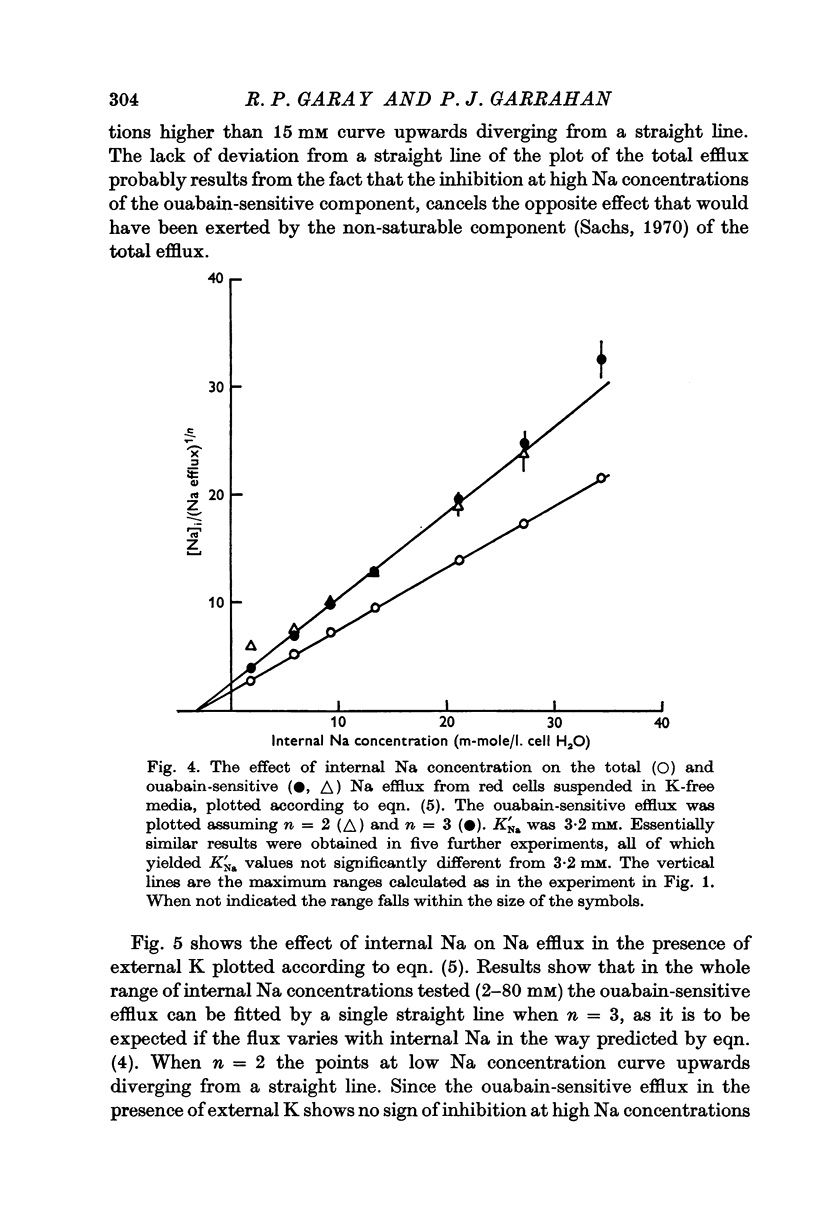
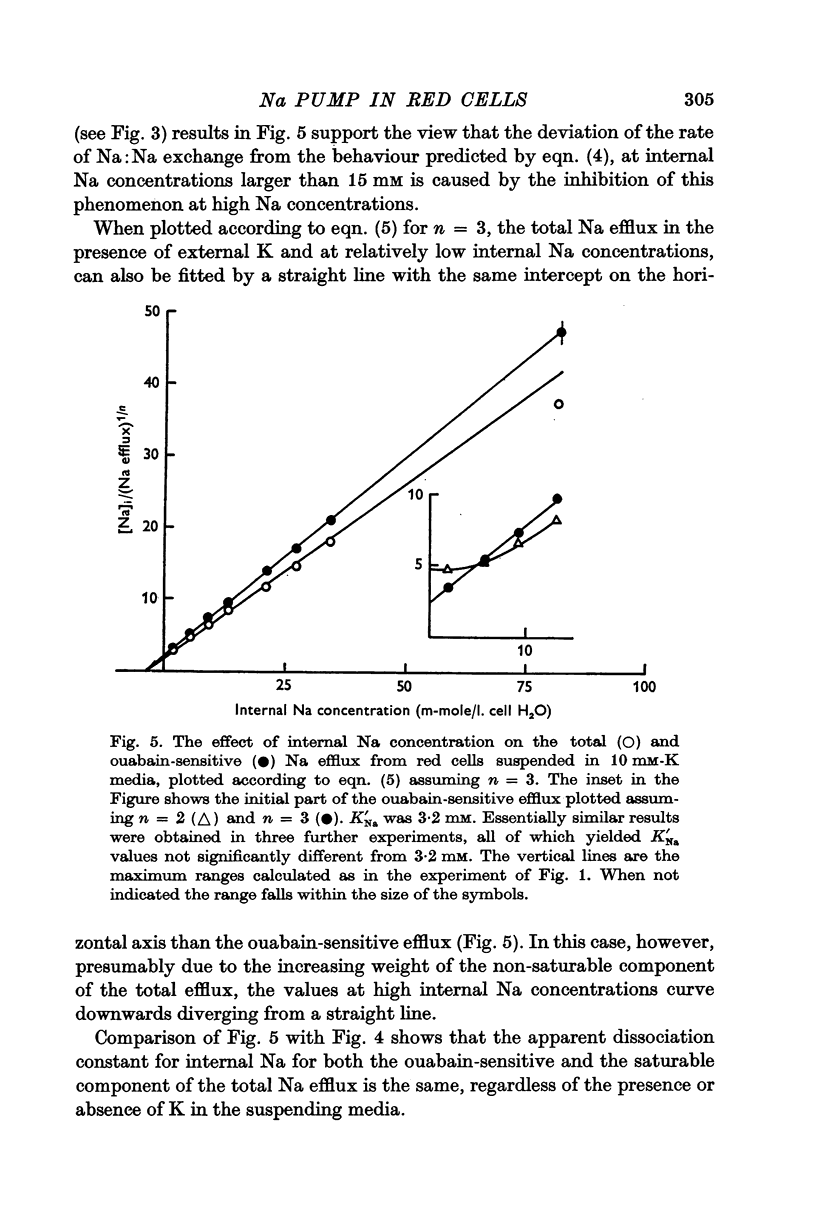
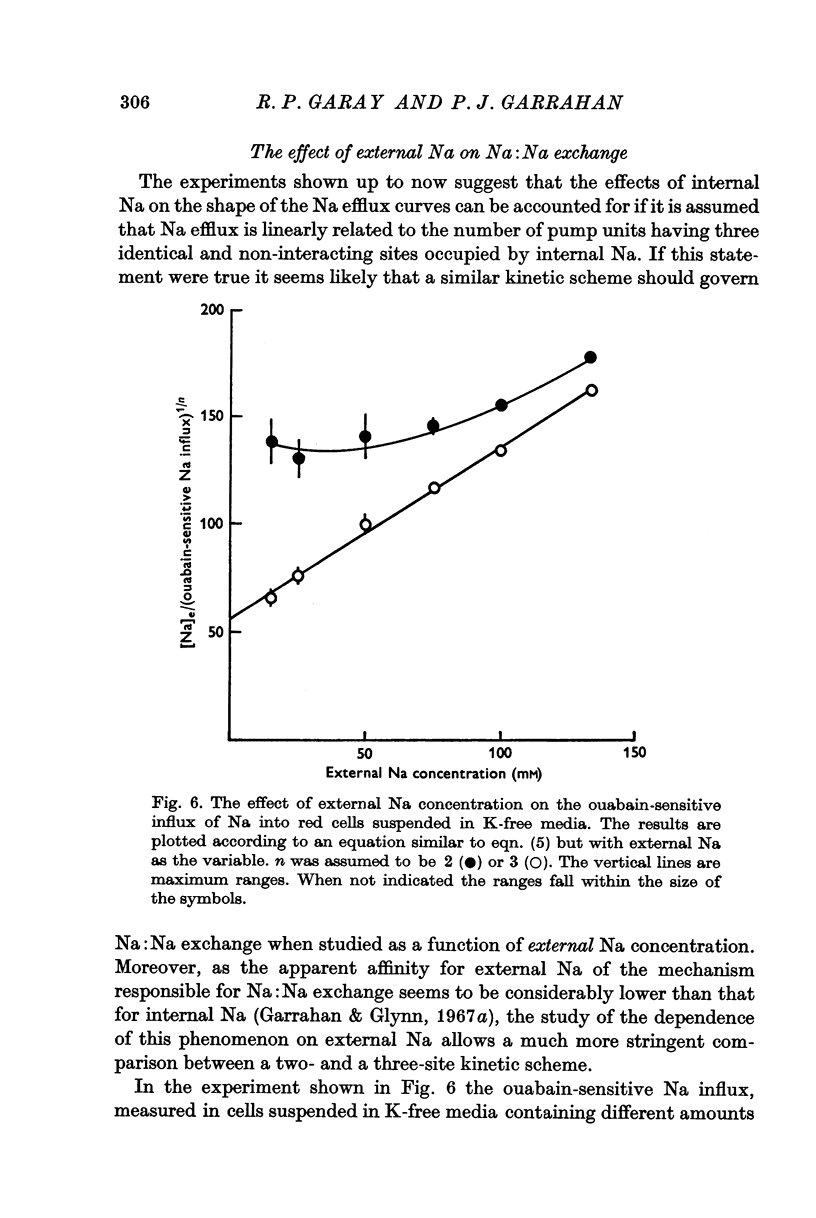
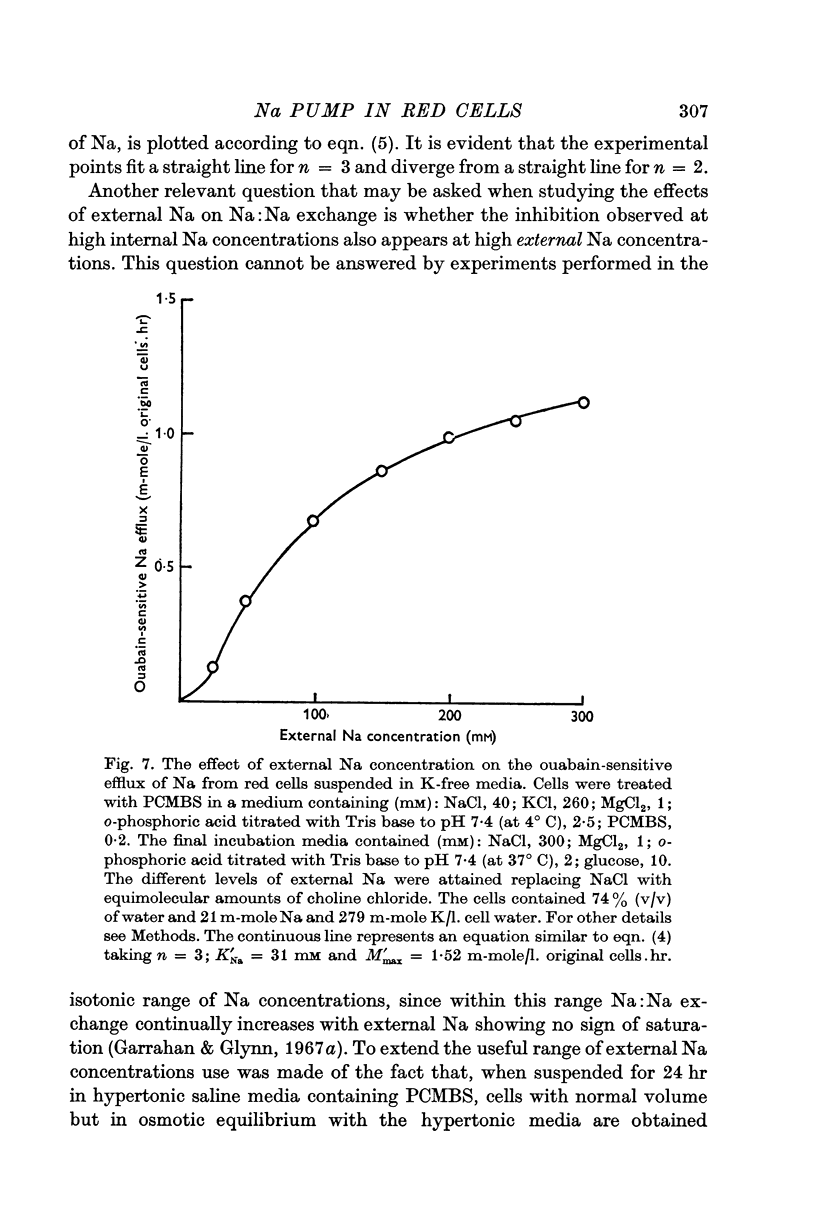
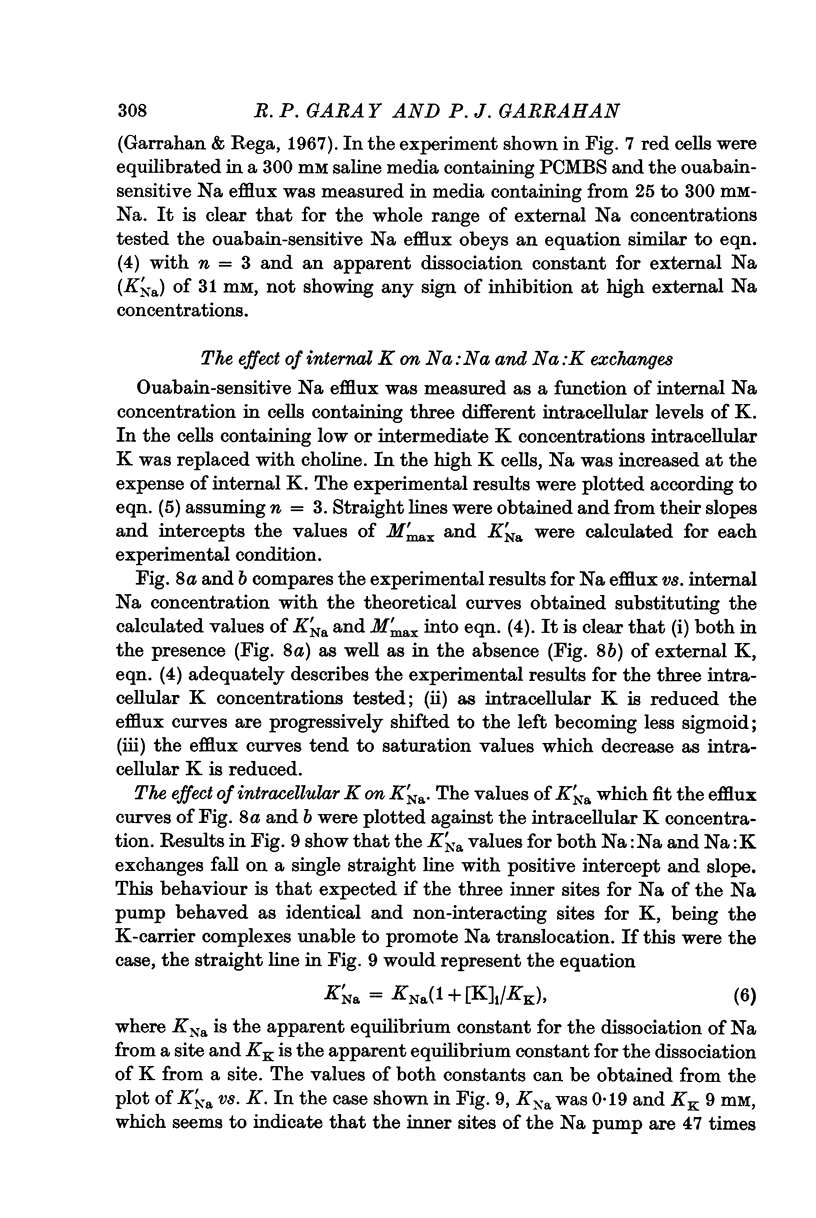
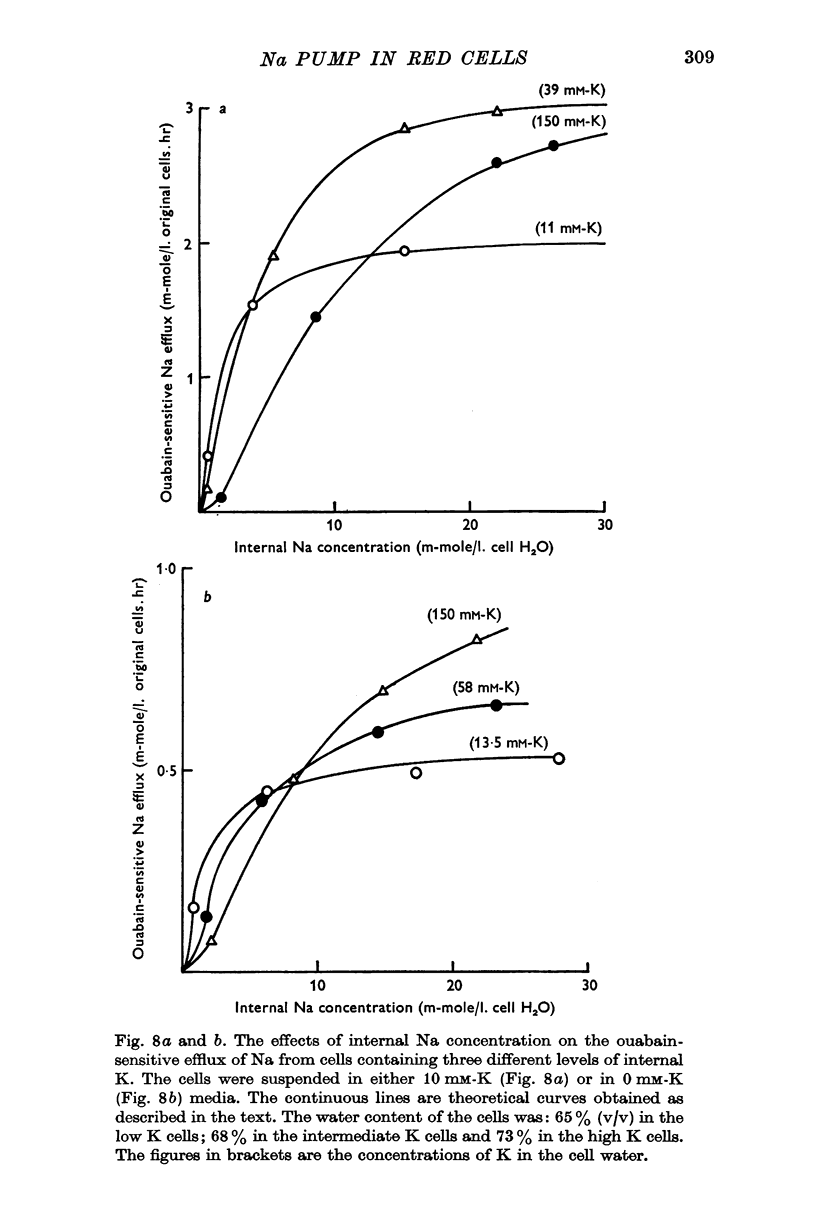
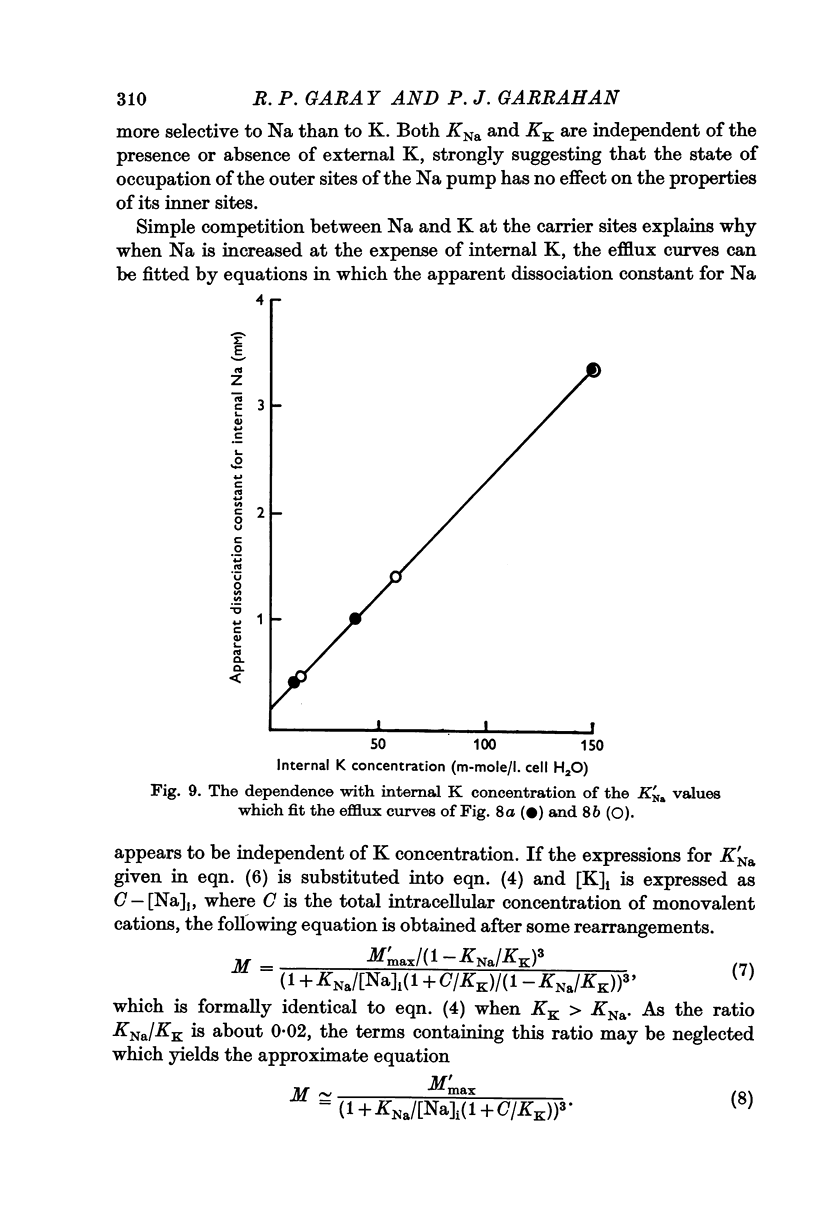
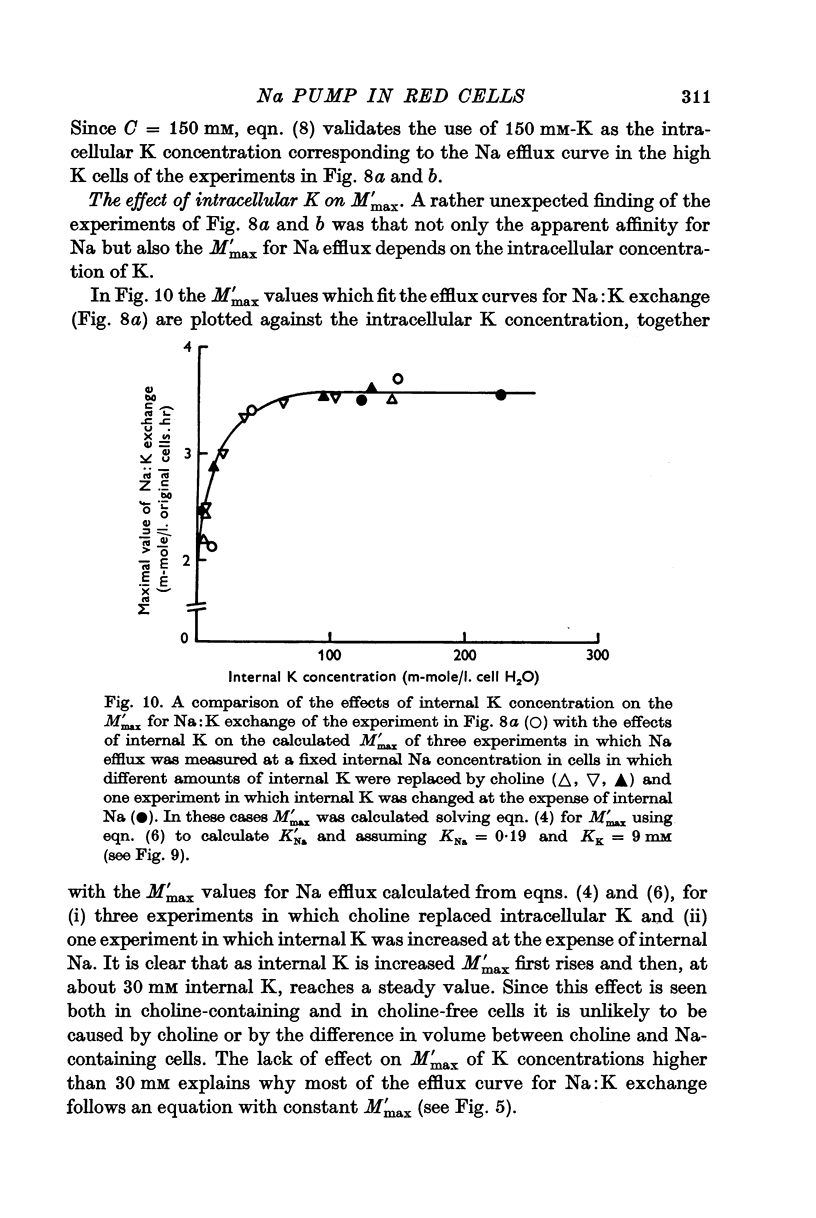
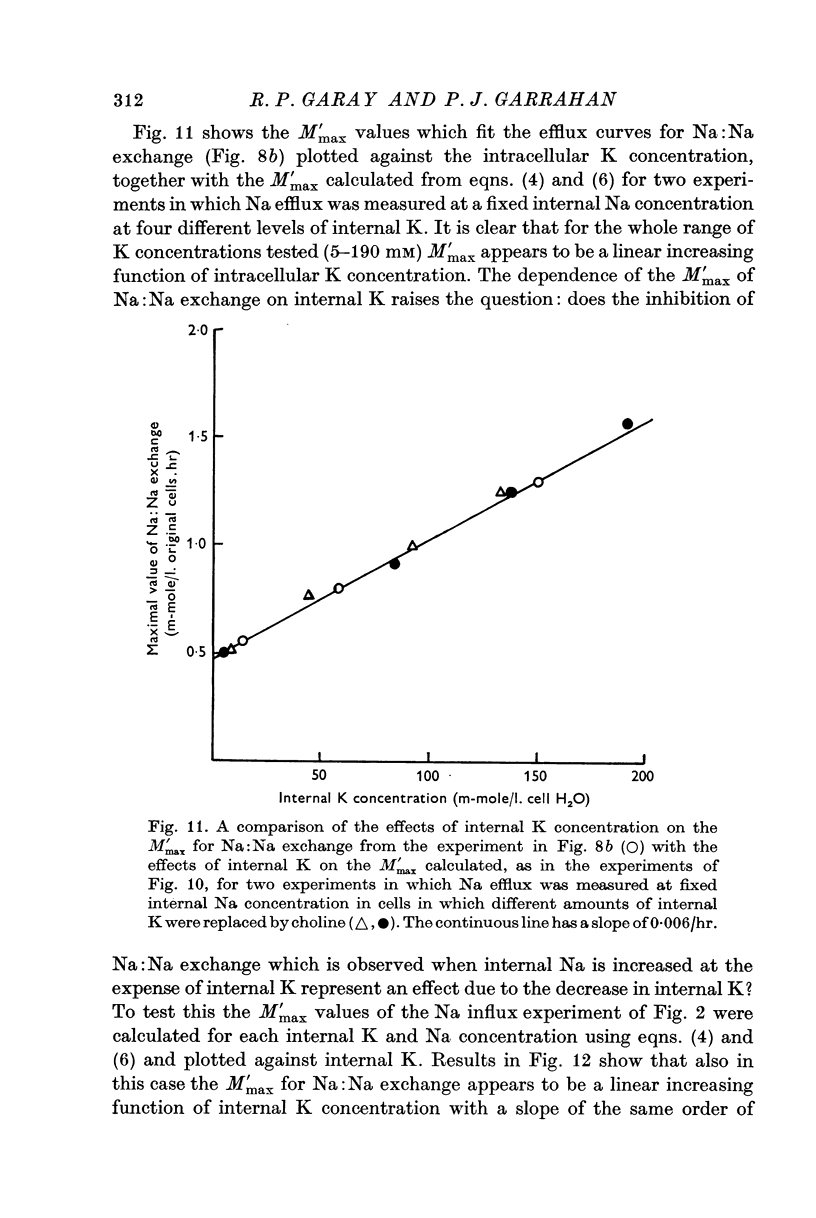
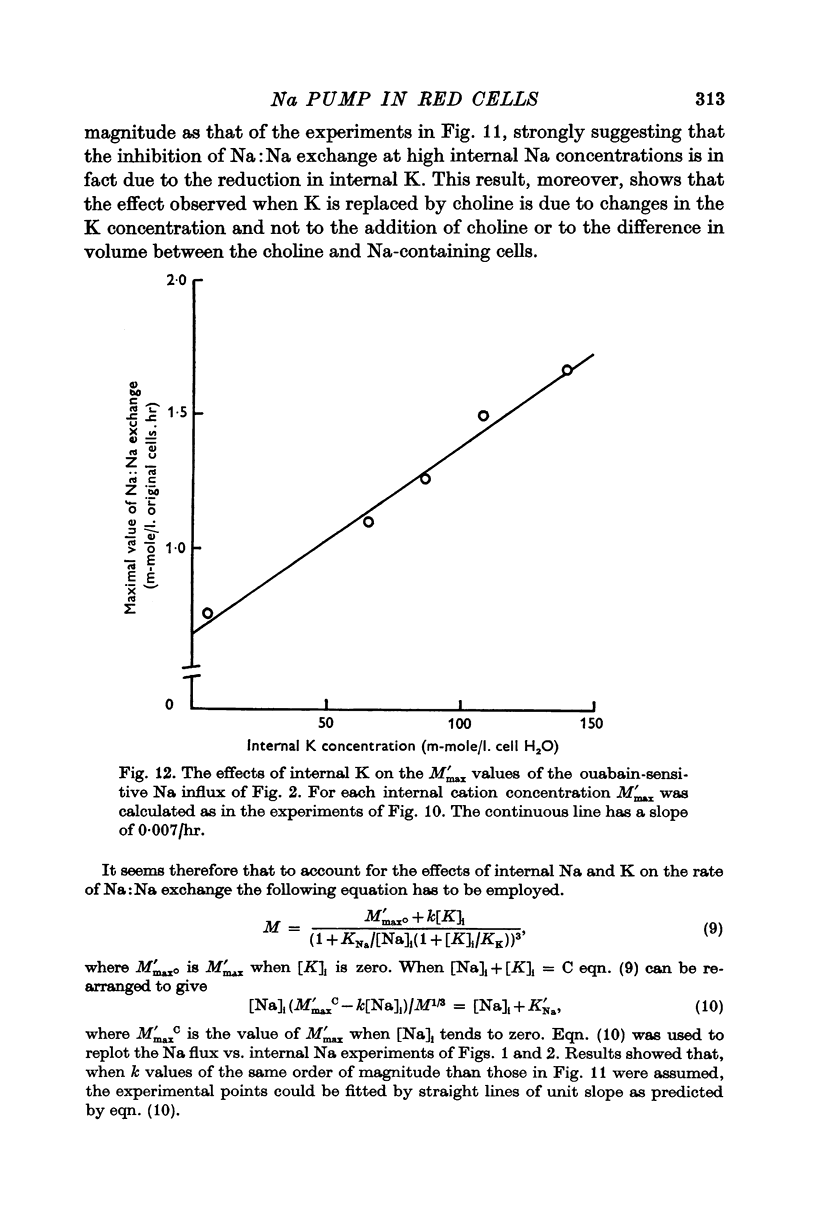
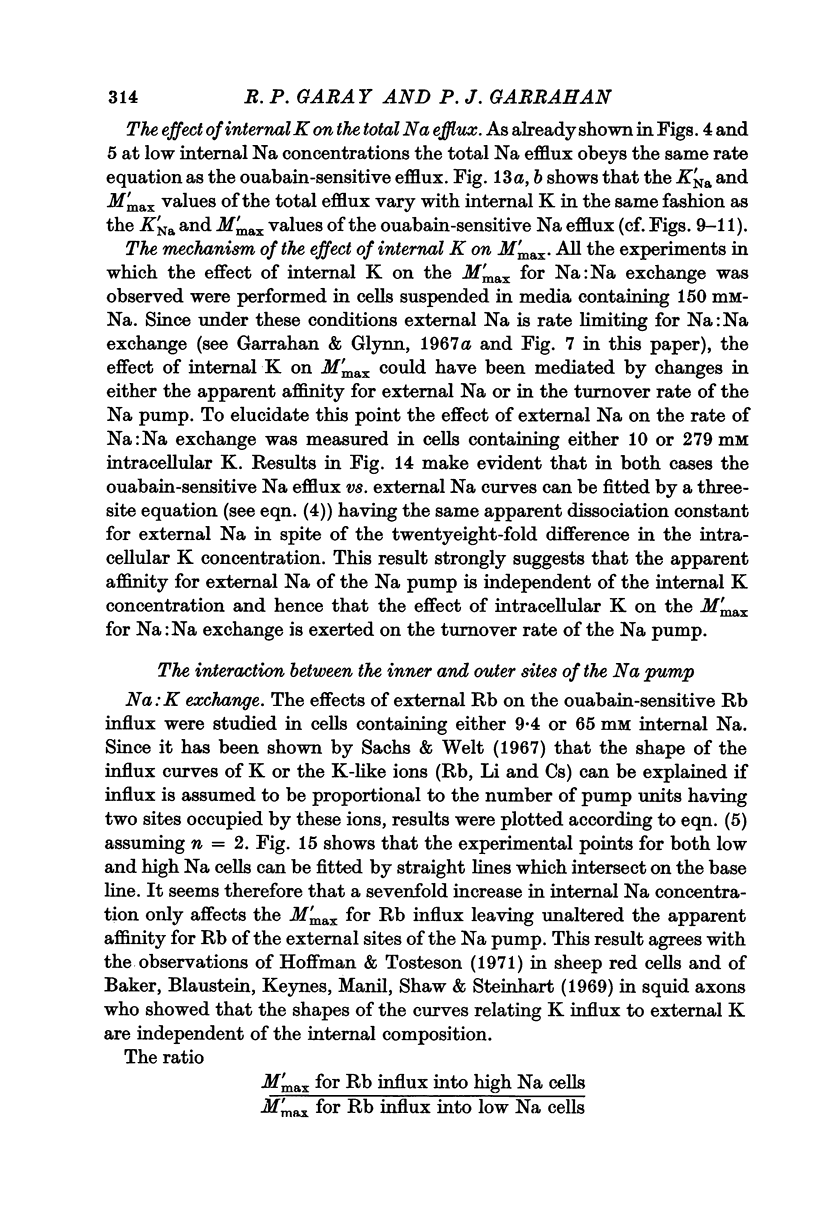
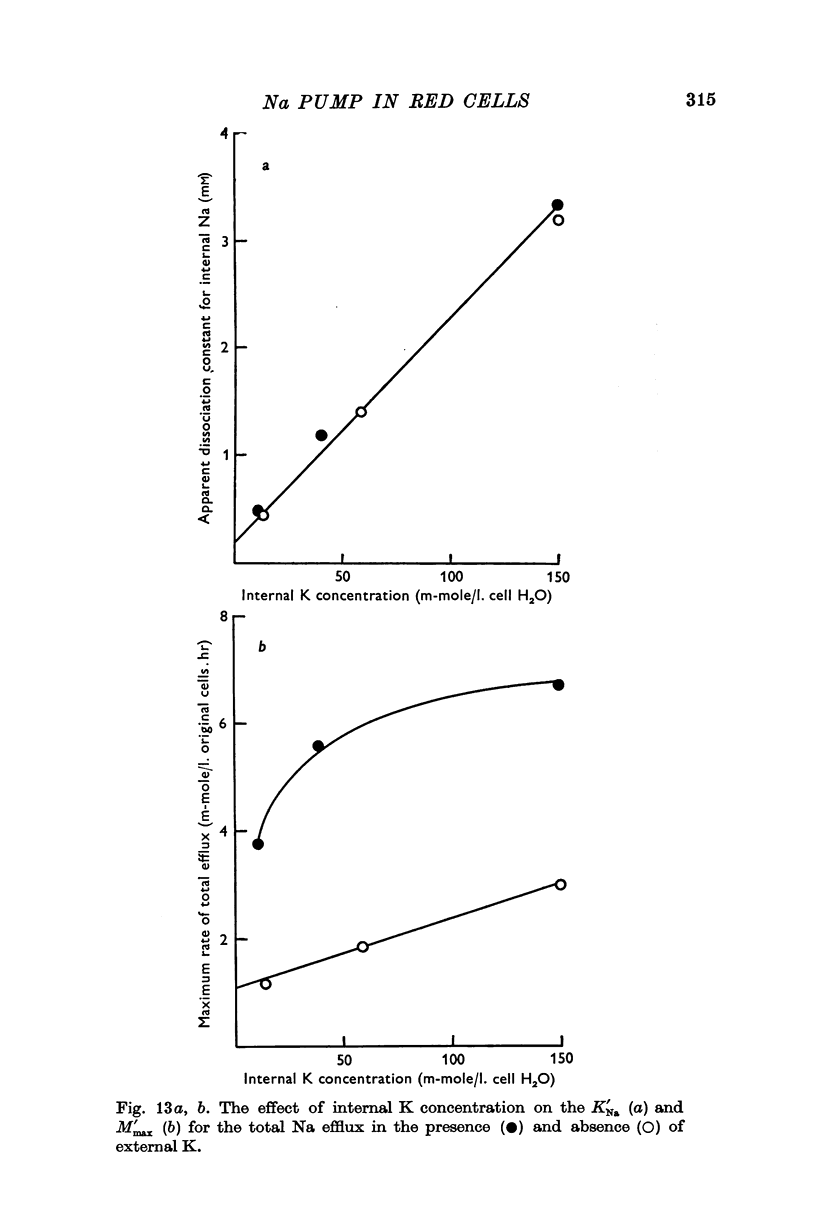
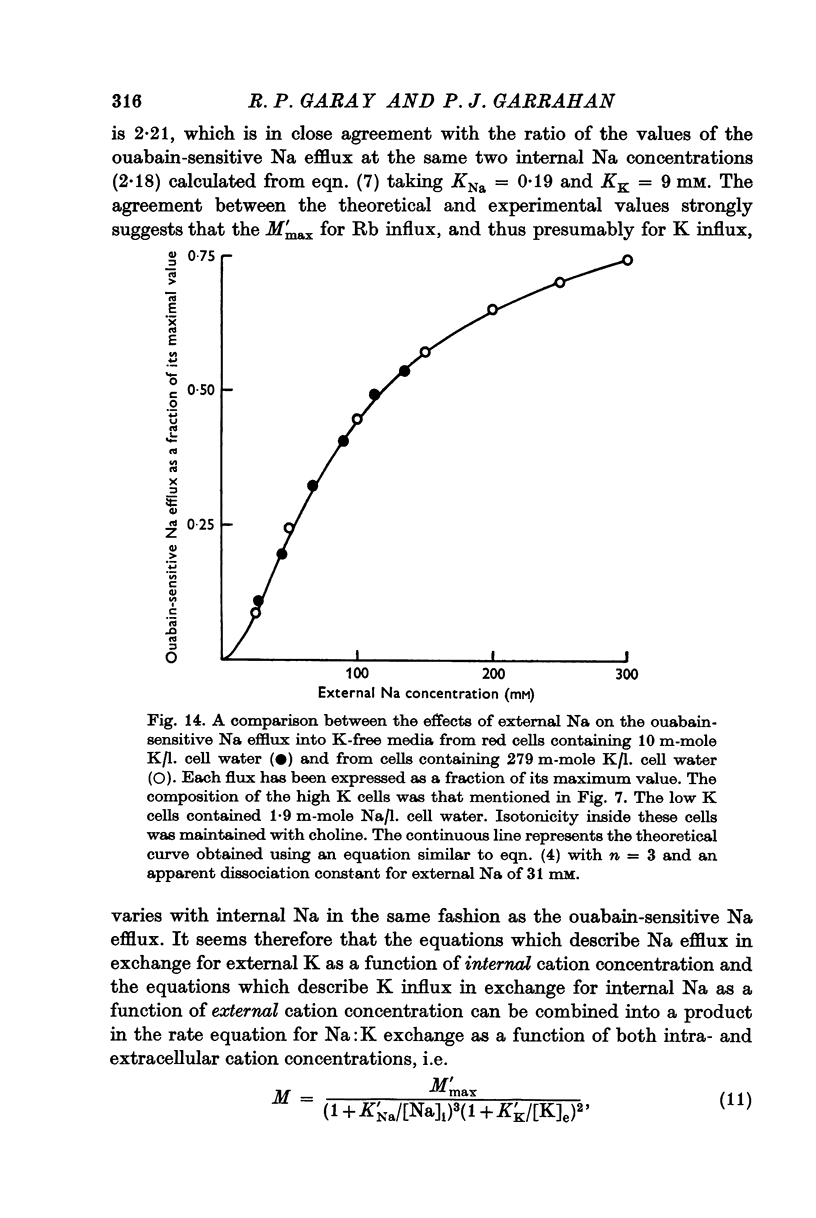
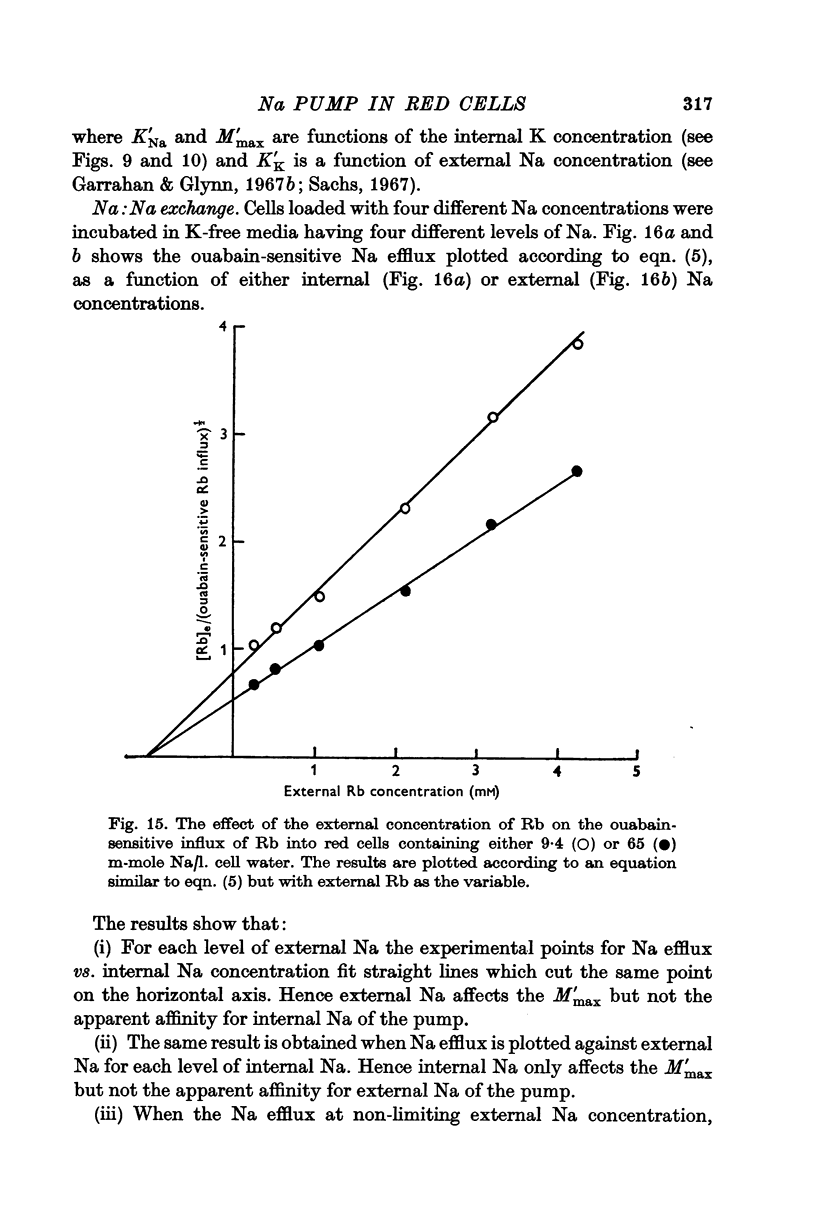
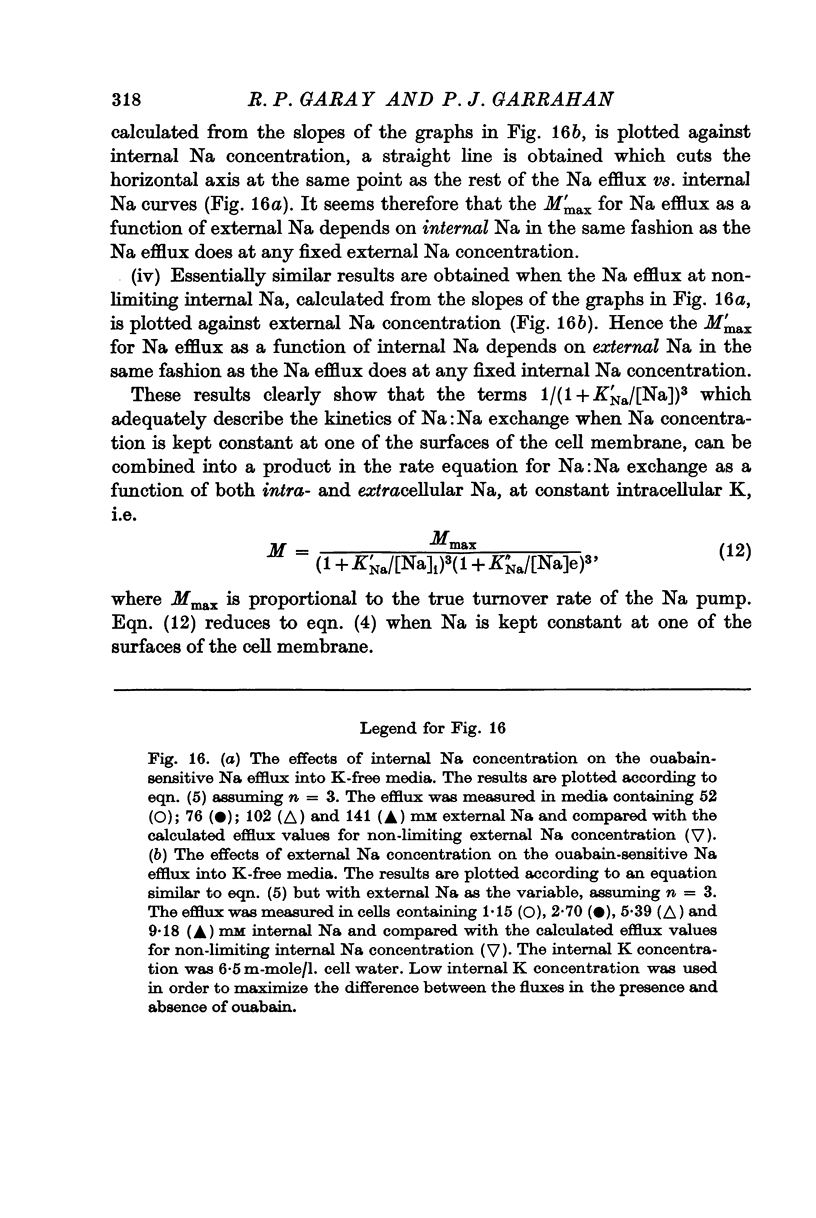
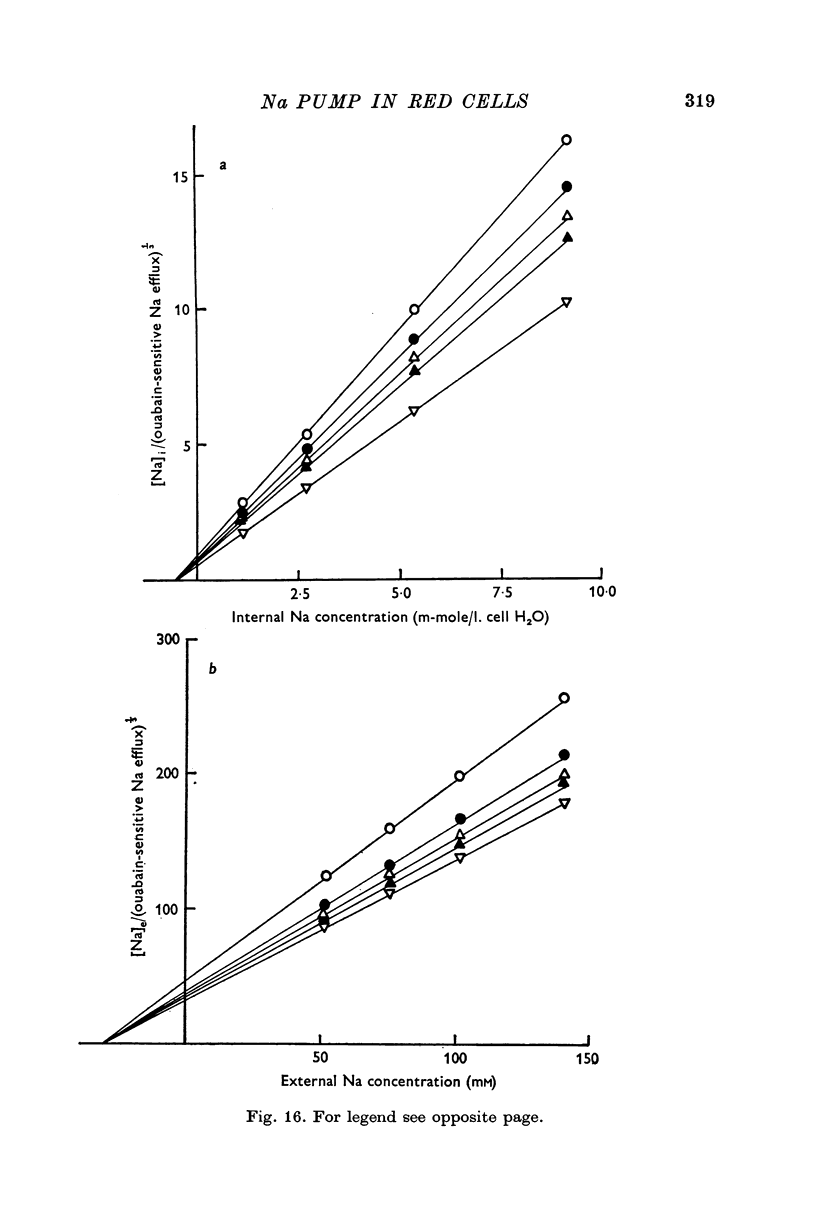
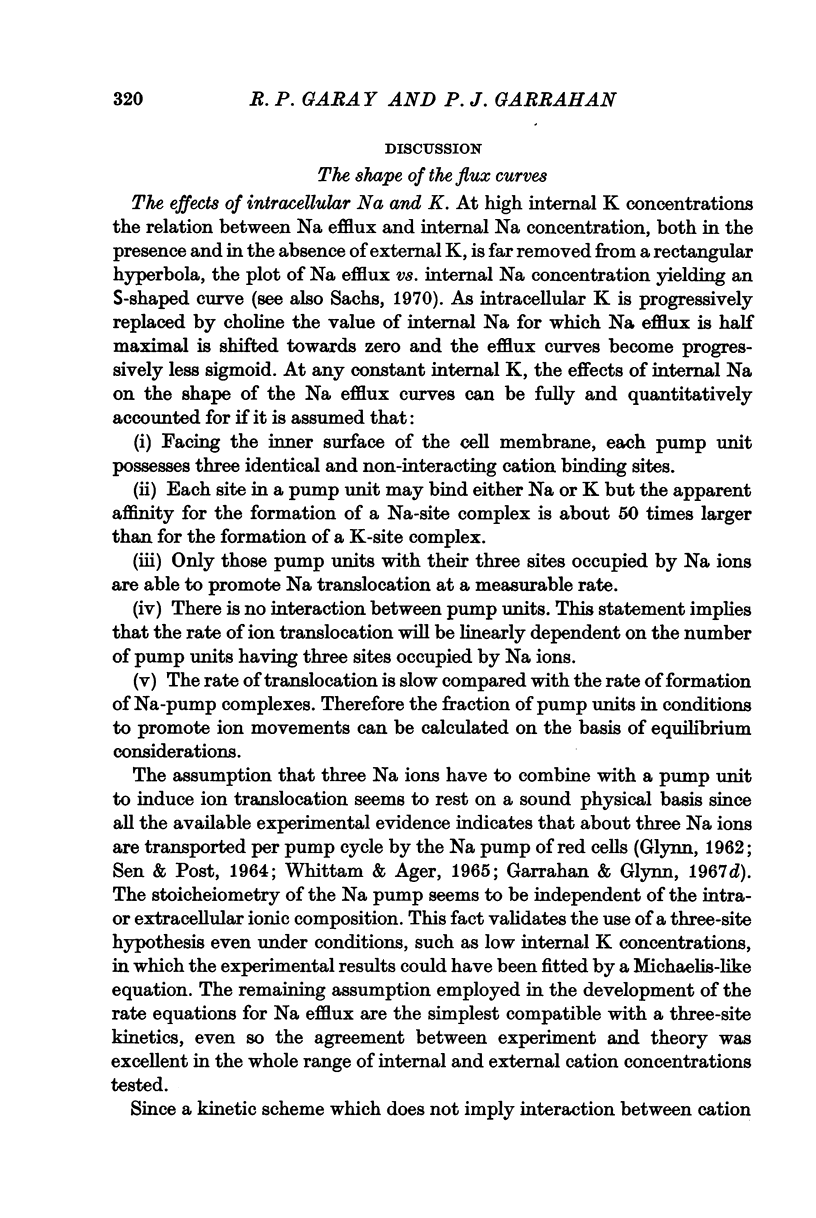
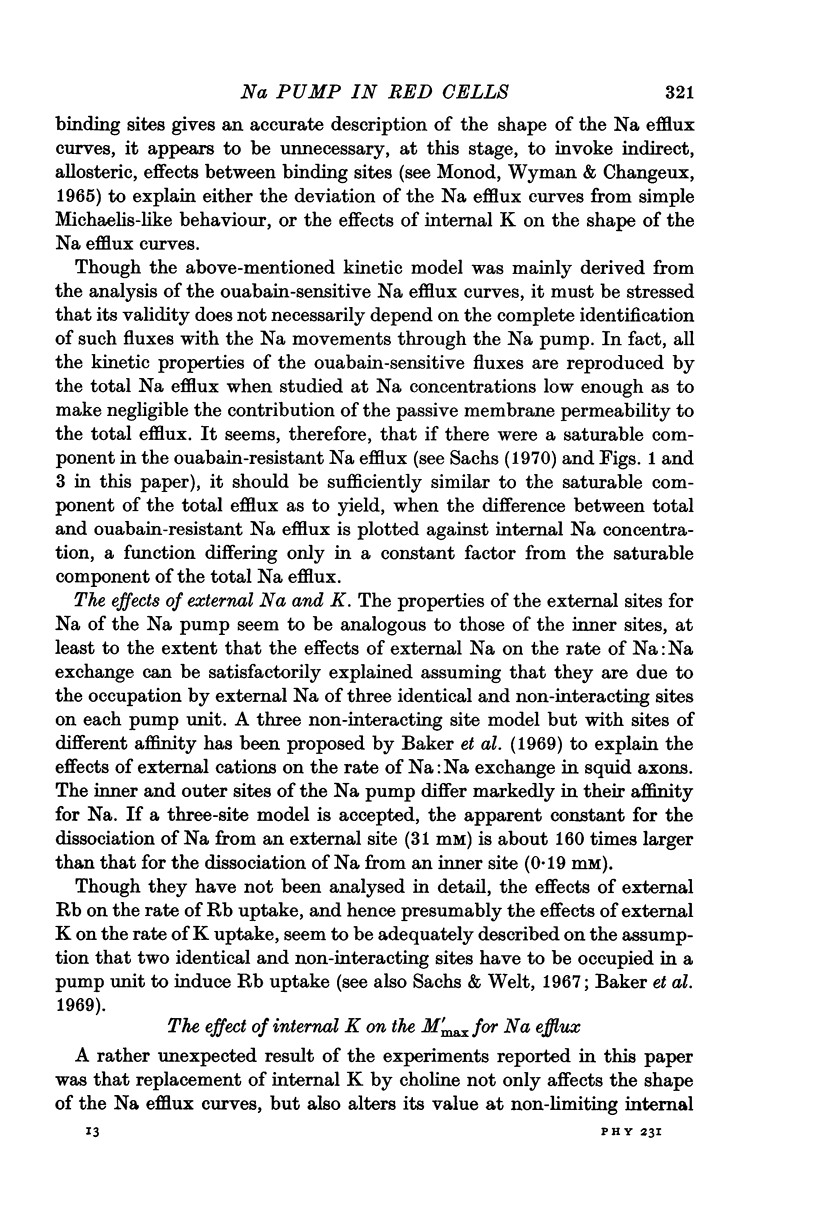
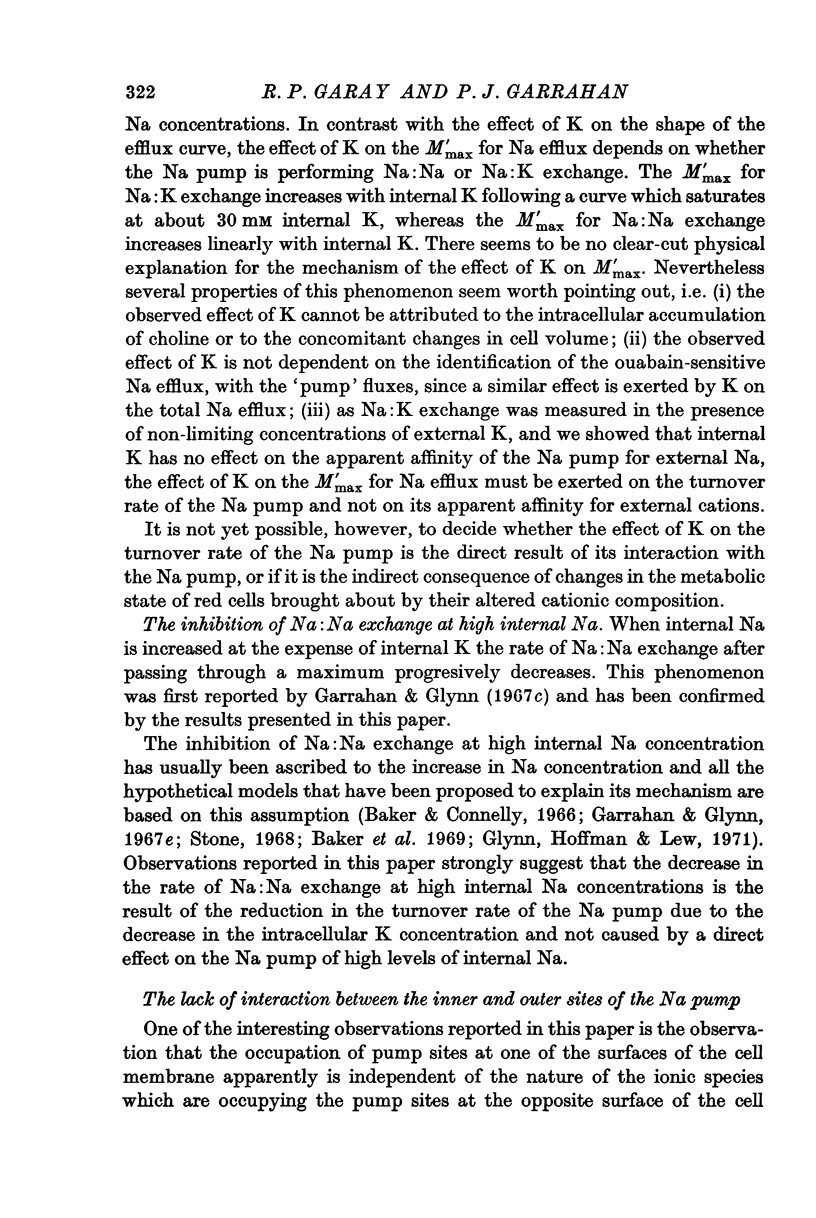
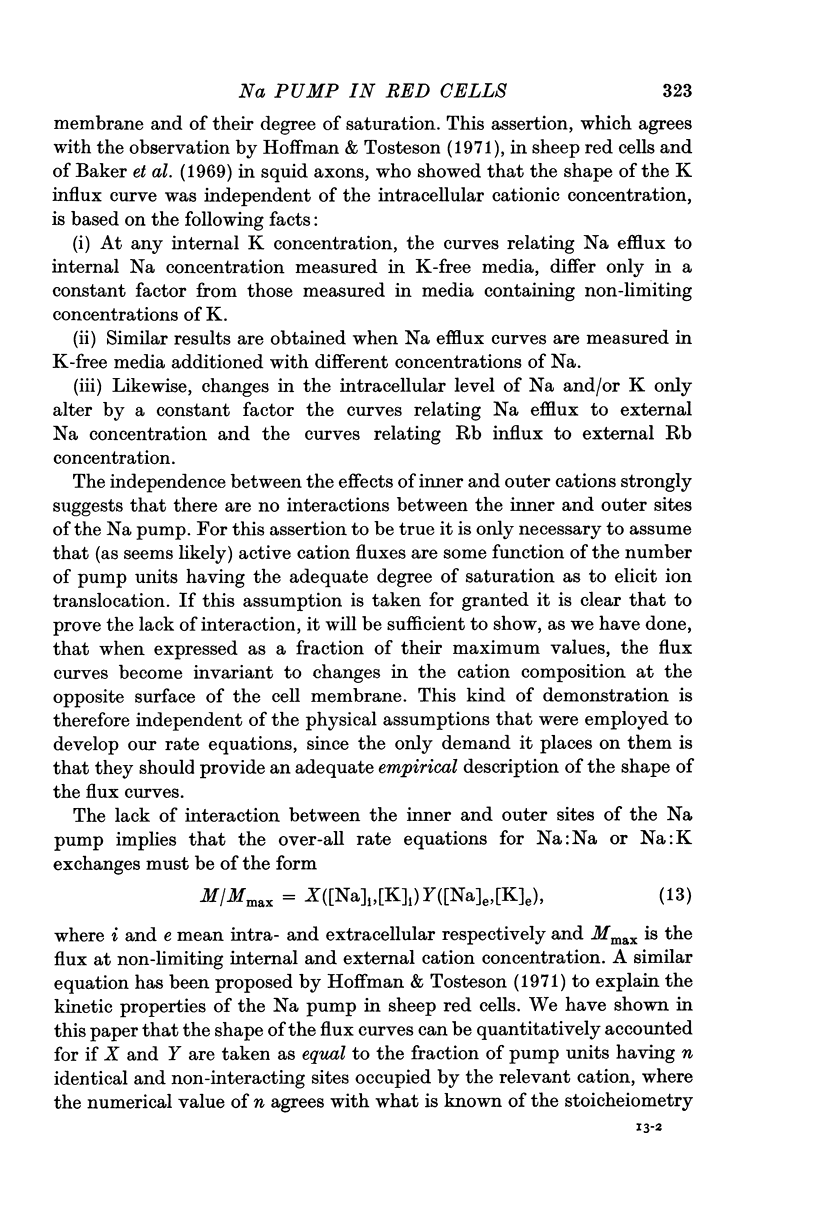
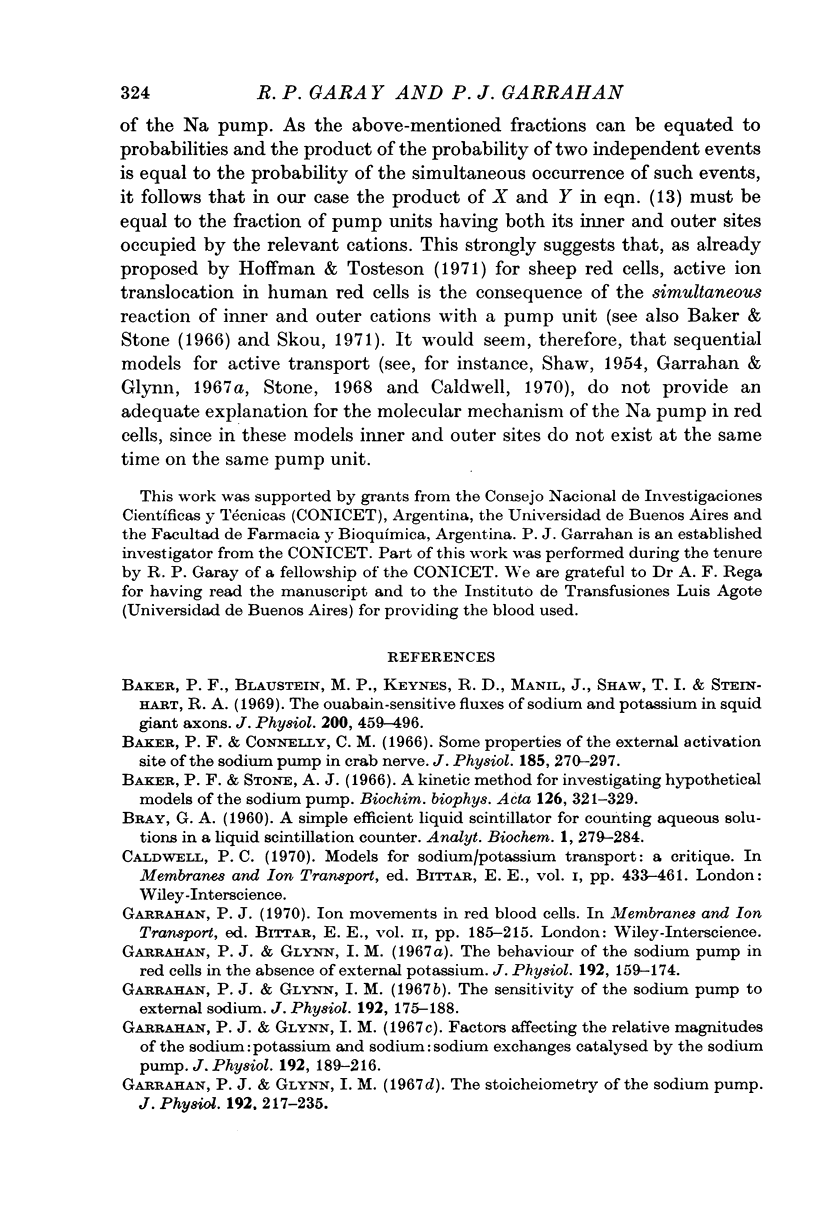
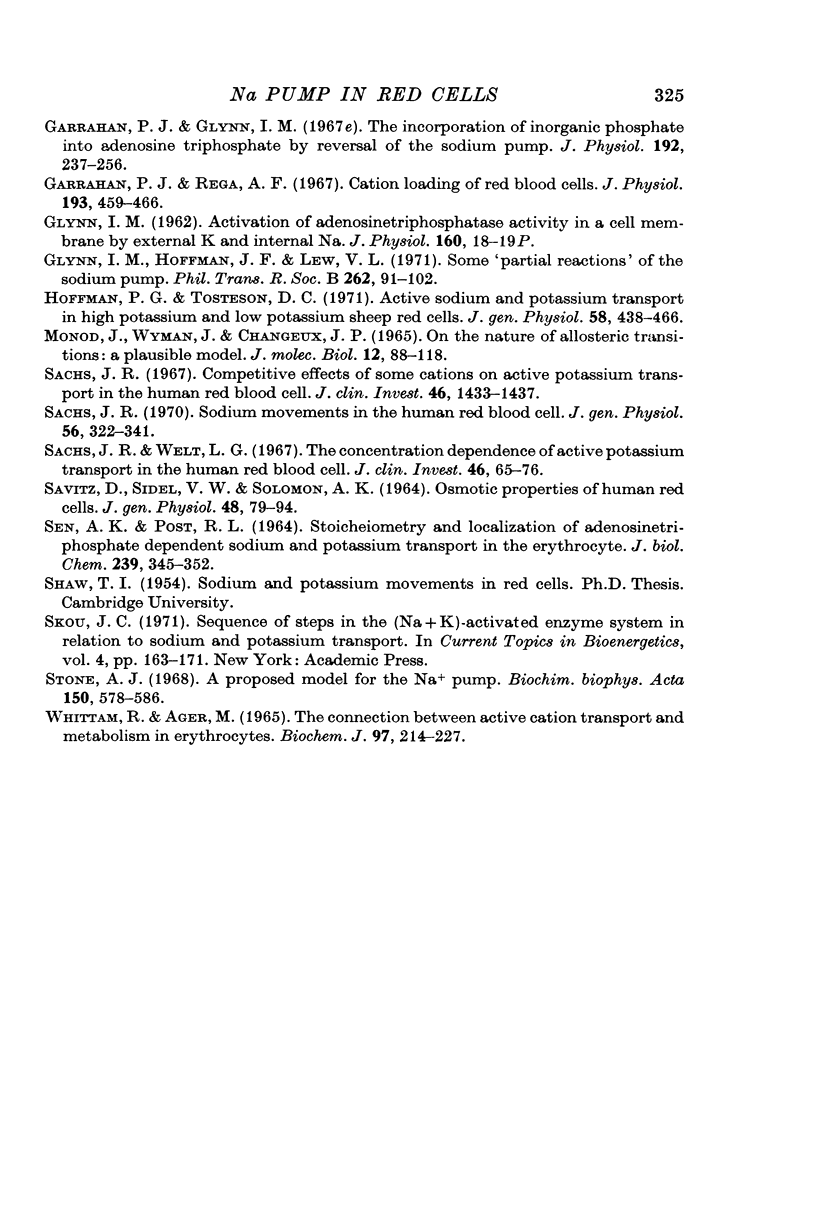
Selected References
These references are in PubMed. This may not be the complete list of references from this article.
- Baker P. F., Blaustein M. P., Keynes R. D., Manil J., Shaw T. I., Steinhardt R. A. The ouabain-sensitive fluxes of sodium and potassium in squid giant axons. J Physiol. 1969 Feb;200(2):459–496. doi: 10.1113/jphysiol.1969.sp008703. [DOI] [PMC free article] [PubMed] [Google Scholar]
- Baker P. F., Connelly C. M. Some properties of the external activation site of the sodium pump in crab nerve. J Physiol. 1966 Jul;185(2):270–297. doi: 10.1113/jphysiol.1966.sp007987. [DOI] [PMC free article] [PubMed] [Google Scholar]
- Baker P. F., Stone A. J. A kinetic method for investigating hypothetical models of the sodium pump. Biochim Biophys Acta. 1966 Oct 10;126(2):321–329. doi: 10.1016/0926-6585(66)90069-0. [DOI] [PubMed] [Google Scholar]
- Garrahan P. J., Glynn I. M. Facftors affecting the relative magnitudes of the sodium:potassium and sodium:sodium exchanges catalysed by the sodium pump. J Physiol. 1967 Sep;192(1):189–216. doi: 10.1113/jphysiol.1967.sp008296. [DOI] [PMC free article] [PubMed] [Google Scholar]
- Garrahan P. J., Glynn I. M. The behaviour of the sodium pump in red cells in the absence of external potassium. J Physiol. 1967 Sep;192(1):159–174. doi: 10.1113/jphysiol.1967.sp008294. [DOI] [PMC free article] [PubMed] [Google Scholar]
- Garrahan P. J., Glynn I. M. The sensitivity of the sodium pump to external sodium. J Physiol. 1967 Sep;192(1):175–188. doi: 10.1113/jphysiol.1967.sp008295. [DOI] [PMC free article] [PubMed] [Google Scholar]
- Garrahan P. J., Rega A. F. Cation loading of red blood cells. J Physiol. 1967 Nov;193(2):459–466. doi: 10.1113/jphysiol.1967.sp008371. [DOI] [PMC free article] [PubMed] [Google Scholar]
- Glynn I. M., Hoffman J. F., Lew V. L. Some "partial reactions" of the sodium pump. Philos Trans R Soc Lond B Biol Sci. 1971 Aug 20;262(842):91–102. doi: 10.1098/rstb.1971.0080. [DOI] [PubMed] [Google Scholar]
- Hoffman P. G., Tosteson D. C. Active sodium and potassium transport in high potassium and low potassium sheep red cells. J Gen Physiol. 1971 Oct;58(4):438–466. doi: 10.1085/jgp.58.4.438. [DOI] [PMC free article] [PubMed] [Google Scholar]
- MONOD J., WYMAN J., CHANGEUX J. P. ON THE NATURE OF ALLOSTERIC TRANSITIONS: A PLAUSIBLE MODEL. J Mol Biol. 1965 May;12:88–118. doi: 10.1016/s0022-2836(65)80285-6. [DOI] [PubMed] [Google Scholar]
- SAVITZ D., SIDEL V. W., SOLOMON A. K. OSMOTIC PROPERTIES OF HUMAN RED CELLS. J Gen Physiol. 1964 Sep;48:79–94. doi: 10.1085/jgp.48.1.79. [DOI] [PMC free article] [PubMed] [Google Scholar]
- SEN A. K., POST R. L. STOICHIOMETRY AND LOCALIZATION OF ADENOSINE TRIPHOSPHATE-DEPENDENT SODIUM AND POTASSIUM TRANSPORT IN THE ERYTHROCYTE. J Biol Chem. 1964 Jan;239:345–352. [PubMed] [Google Scholar]
- Sachs J. R. Competitive effects of some cations on active potassium transport in the human red blood cell. J Clin Invest. 1967 Sep;46(9):1433–1441. doi: 10.1172/JCI105635. [DOI] [PMC free article] [PubMed] [Google Scholar]
- Sachs J. R. Sodium movements in the human red blood cell. J Gen Physiol. 1970 Sep;56(3):322–341. doi: 10.1085/jgp.56.3.322. [DOI] [PMC free article] [PubMed] [Google Scholar]
- Sachs J. R., Welt L. G. The concentration dependence of active potassium transport in the human red blood cell. J Clin Invest. 1967 Jan;46(1):65–76. doi: 10.1172/JCI105512. [DOI] [PMC free article] [PubMed] [Google Scholar]
- Stone A. J. A proposed model for the Na+ pump. Biochim Biophys Acta. 1968 Jun 11;150(4):578–586. doi: 10.1016/0005-2736(68)90047-3. [DOI] [PubMed] [Google Scholar]
- Whittam R., Ager M. E. The connexion between active cation transport and metabolism in erythrocytes. Biochem J. 1965 Oct;97(1):214–227. doi: 10.1042/bj0970214. [DOI] [PMC free article] [PubMed] [Google Scholar]