Abstract
1. The use of polyacrylamide gel salt bridges enables trans-membrane potentials to be measured to an accuracy of 20 μV over long periods.
2. The technique is applied to measure electrical potentials across corneal endothelia of rabbits.
3. In de-epithelialized corneas which translocate water, a spontaneous potential of 550 μV is found across the endothelium (tissue resistance 20 Ω cm2).
4. This electrical potential (and water translocation) is reduced to zero when sodium is absent from the Ringer, and by about 80% when bicarbonate ions are absent. Removal of chloride has no such effect.
5. Under a variety of conditions, the potential correlates with the observed translocation of fluid across corneal endothelium. The translocated fluid is shown to be isotonic with sodium in the Ringer and therefore the potential correlates with `active' sodium transport.
6. The potential and water translocation are abolished in the presence of ouabain at concentrations greater than 10-5 M.
7. The potential (lens-side negative) is of the wrong polarity to explain the net sodium transport (into the lens-side) by a sodium ion `pump'.
8. The current does not equal the net sodium flux under short circuit conditions. They differ in magnitude and polarity.
9. A model is proposed where the endothelium `pumps' salt out of the corneal stroma into the aqueous humour.
10. Flux equations are derived for a condition where the membrane (corneal endothelium) separates an ion exchanger (corneal stroma) from free solution (aqueous humour), where the usual relationship for free-free solutions Δπ = csΔμs does not apply.
11. The model is of use only when the stroma is well stirred. It may be used in whole corneas retaining their epithelium but it may not be used in de-epithelialized corneas.
12. The model predicts that the presence of an `active' salt flux out across the endothelium would create passive water and salt fluxes. The passive water flux would also travel out of the stroma across the endothelium; the passive salt flux would travel, in the opposite direction, into the stroma across the endothelium.
13. The kinetics of the passive water efflux, as a swollen cornea reverts to physiological hydration (the temperature reversal phenomenon) are predicted extremely well if the `active' salt flux is chosen at 3·3 × 10-7 m-mole. cm-2 sec-1.
14. The value of the active salt flux which cannot be measured directly is extrapolated to be somewhat greater than 2·8 × 10-7 m-moles. cm-2 sec-1; in good agreement with that required by the model to explain the temperature reversal phenomenon.
15. The model is further used to calculate the salt concentration difference across the endothelium (which drives salt passively into the stroma) at various stromal hydrations.
16. When an appropriate salt concentration is applied across the endothelium of de-epithelialized cornea, it generates a potential of the same polarity and similar magnitude to that found across the endothelium of equilibrated whole cornea. The endothelium acts like a cation exchange membrane.
17. Additionally the calculated salt concentration difference across the endothelium correlates well with the measured transendothelial potentials in whole cornea as the corneal hydration varies.
18. It is concluded that the model of an endothelial neutral salt `pump' regulating corneal hydration is self consistent. The spontaneous potential found across the endothelium could be caused by the consequential passive flux of salt in the opposite direction.
Full text
PDF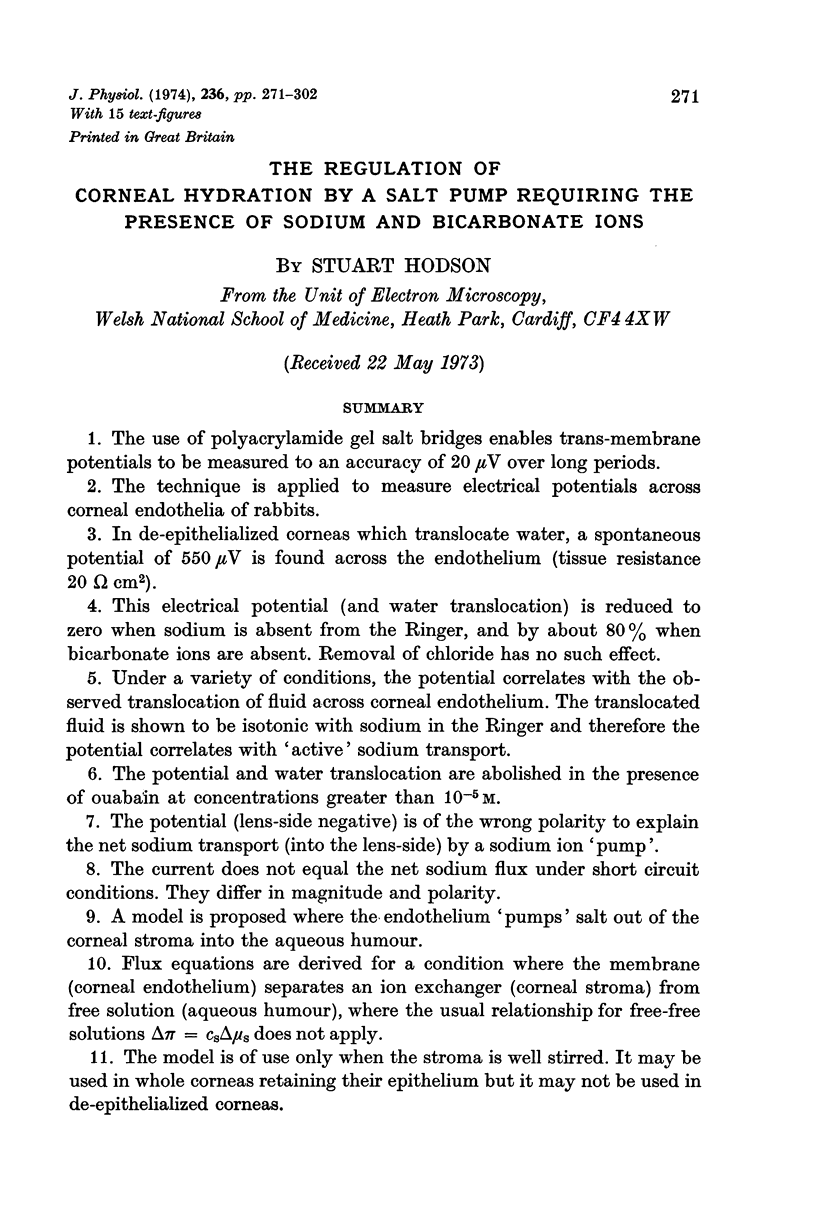
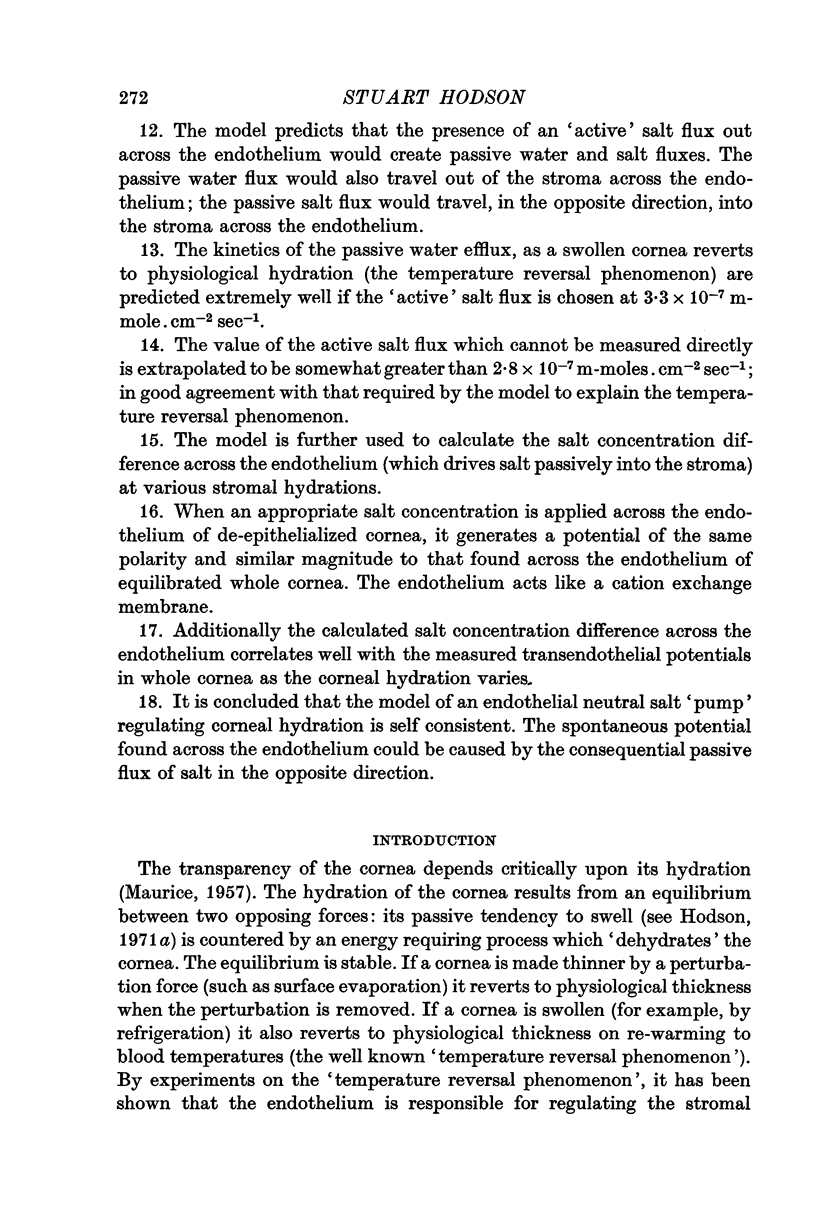
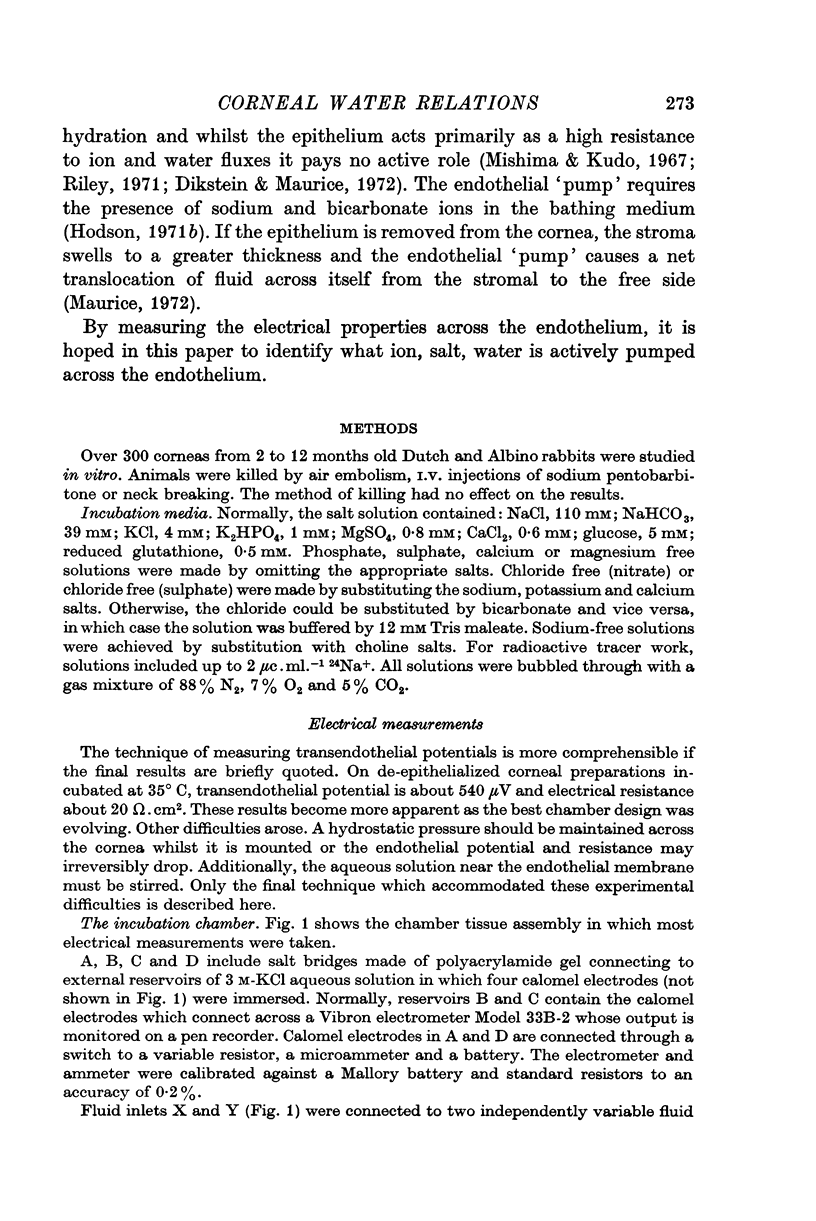
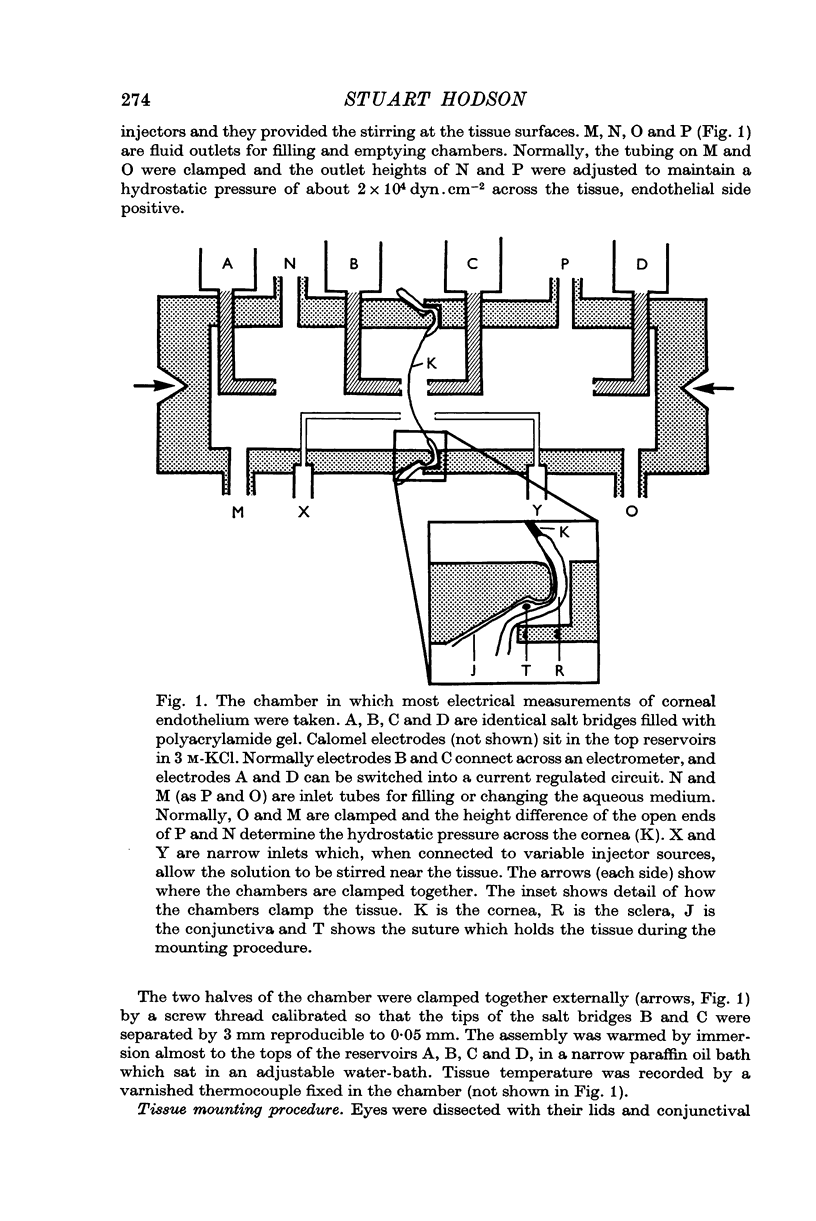
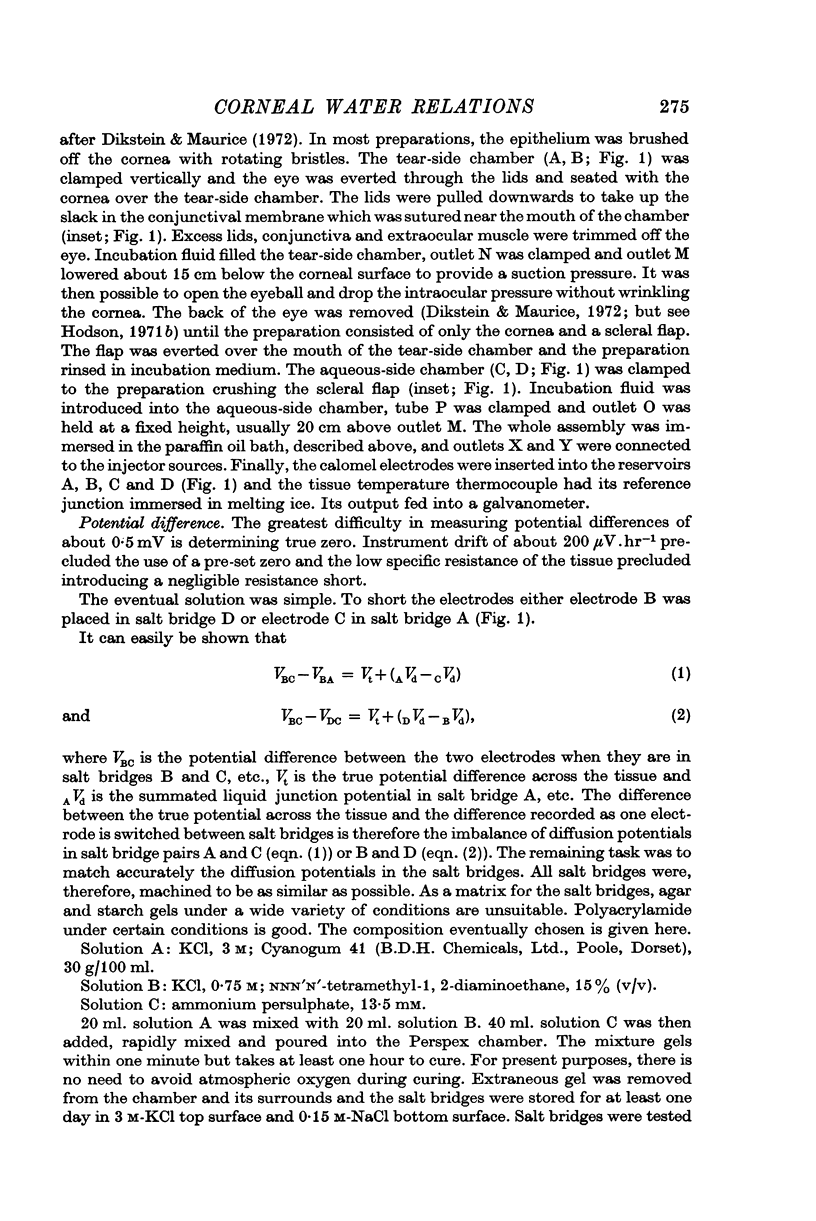
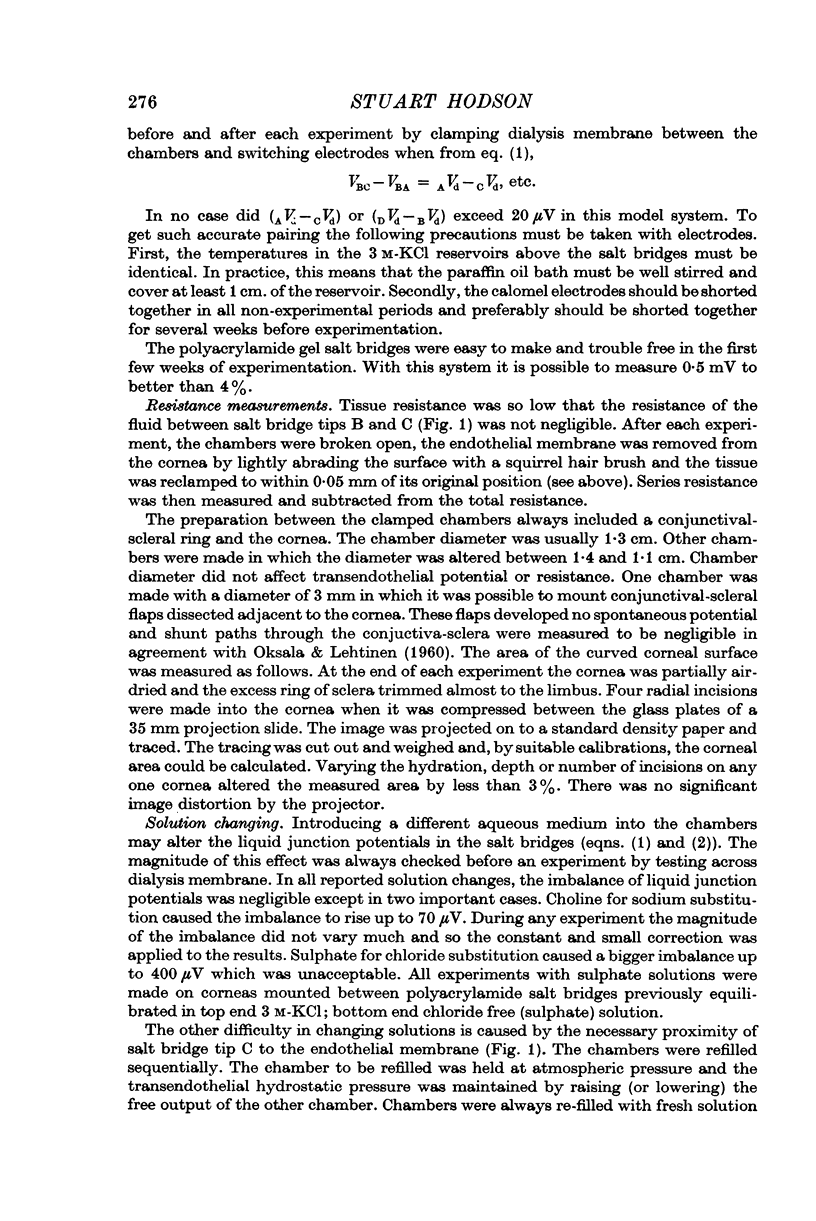
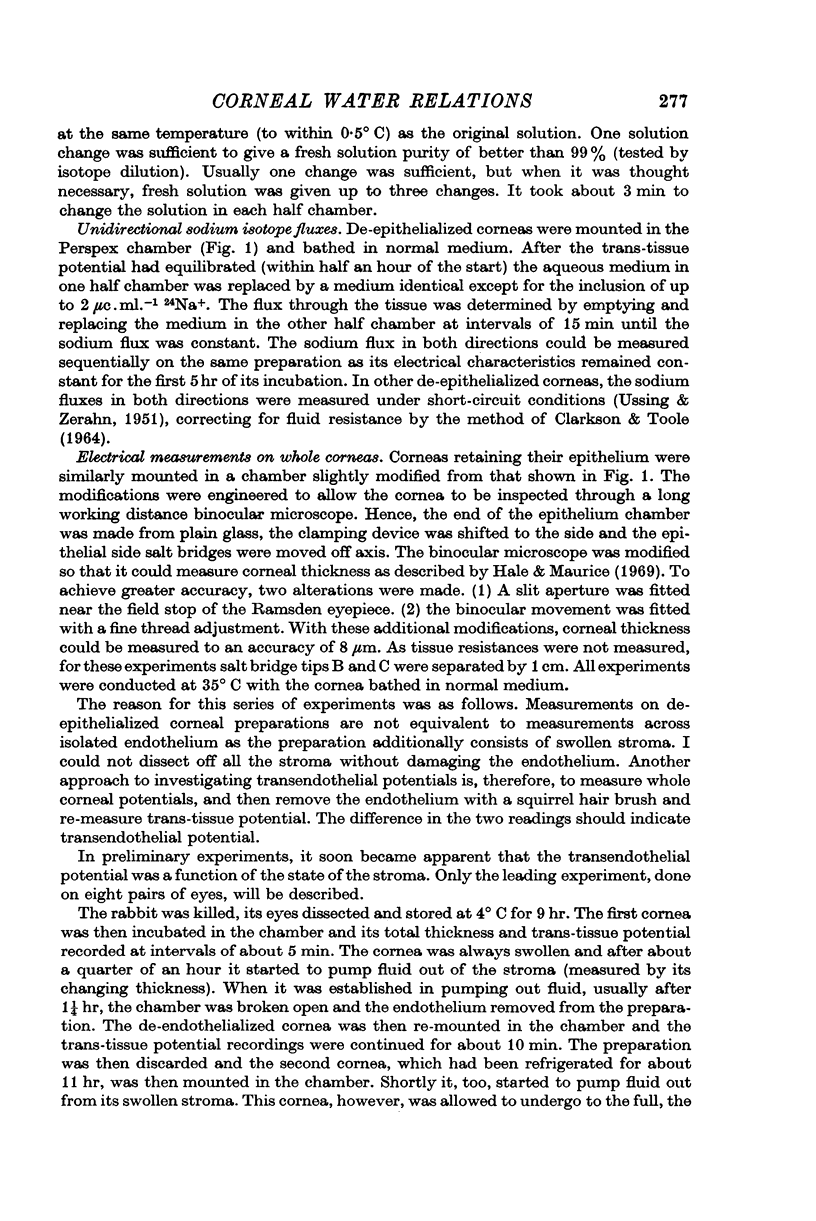
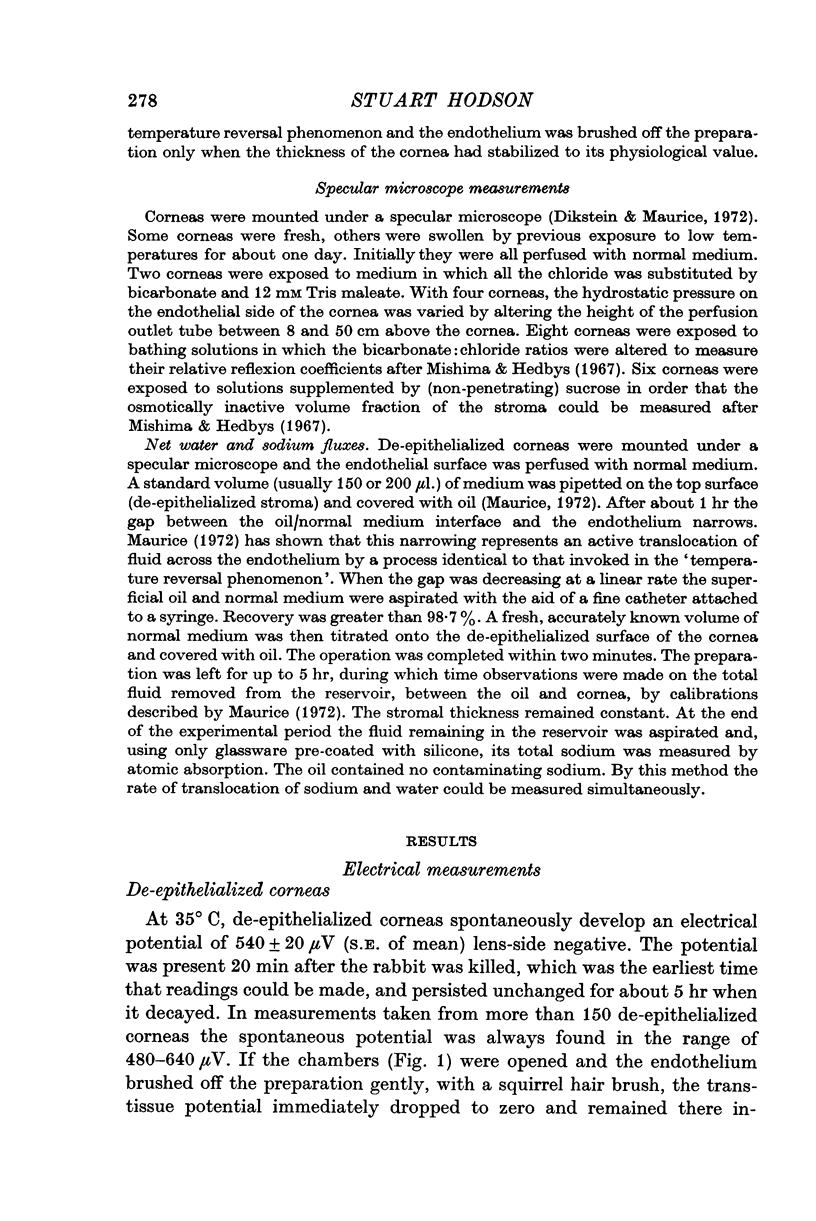
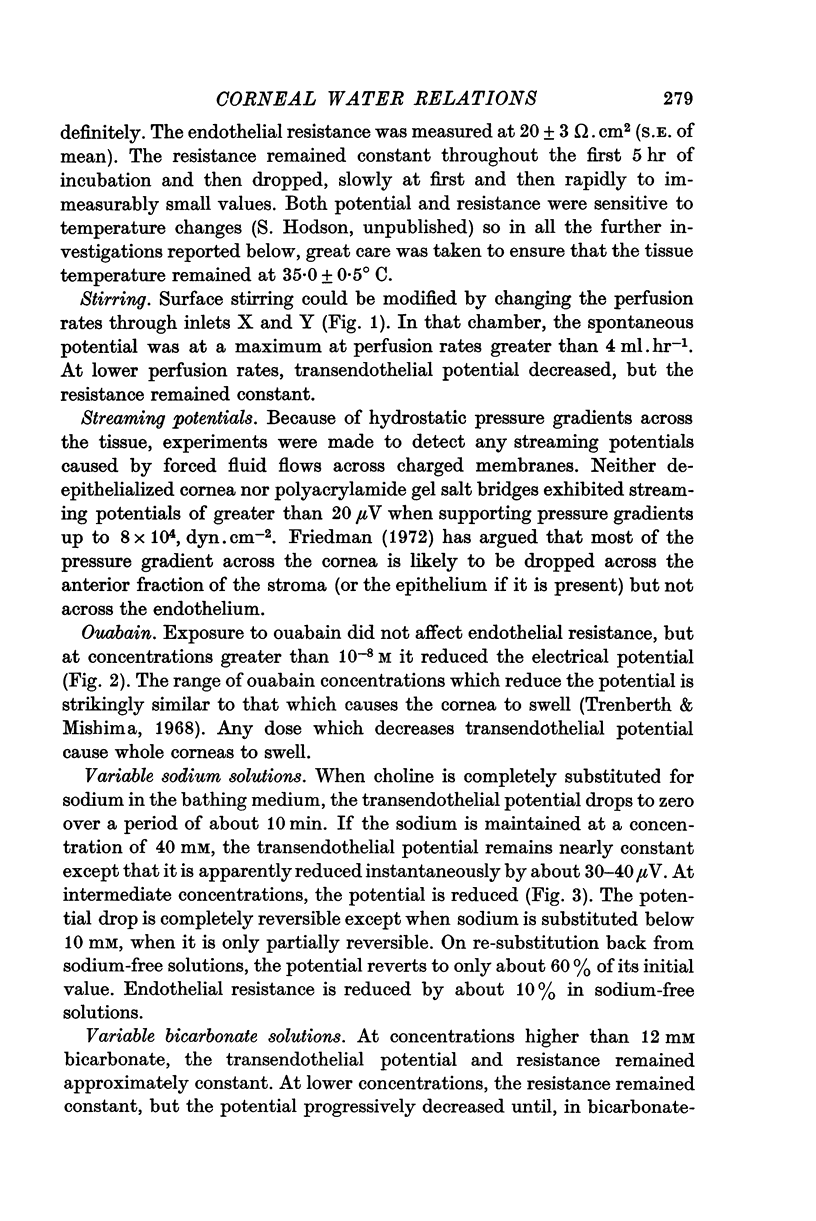
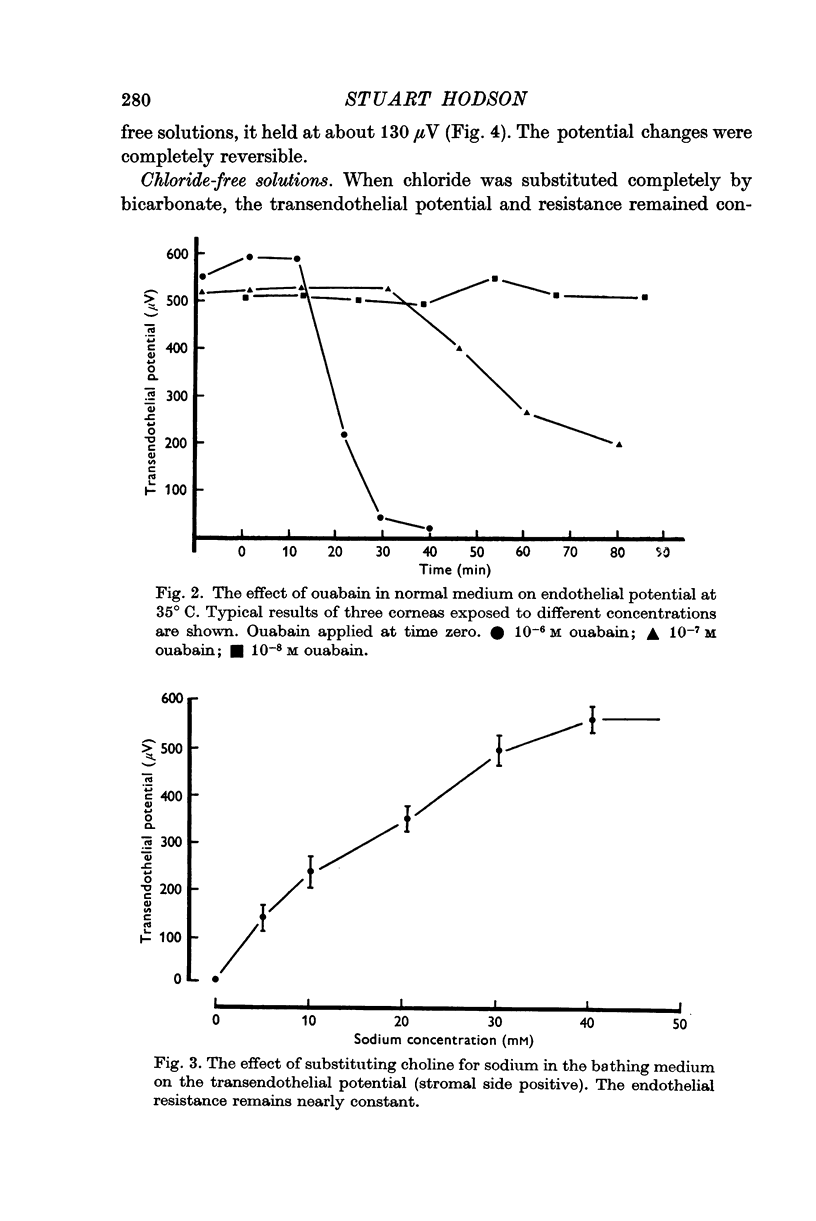
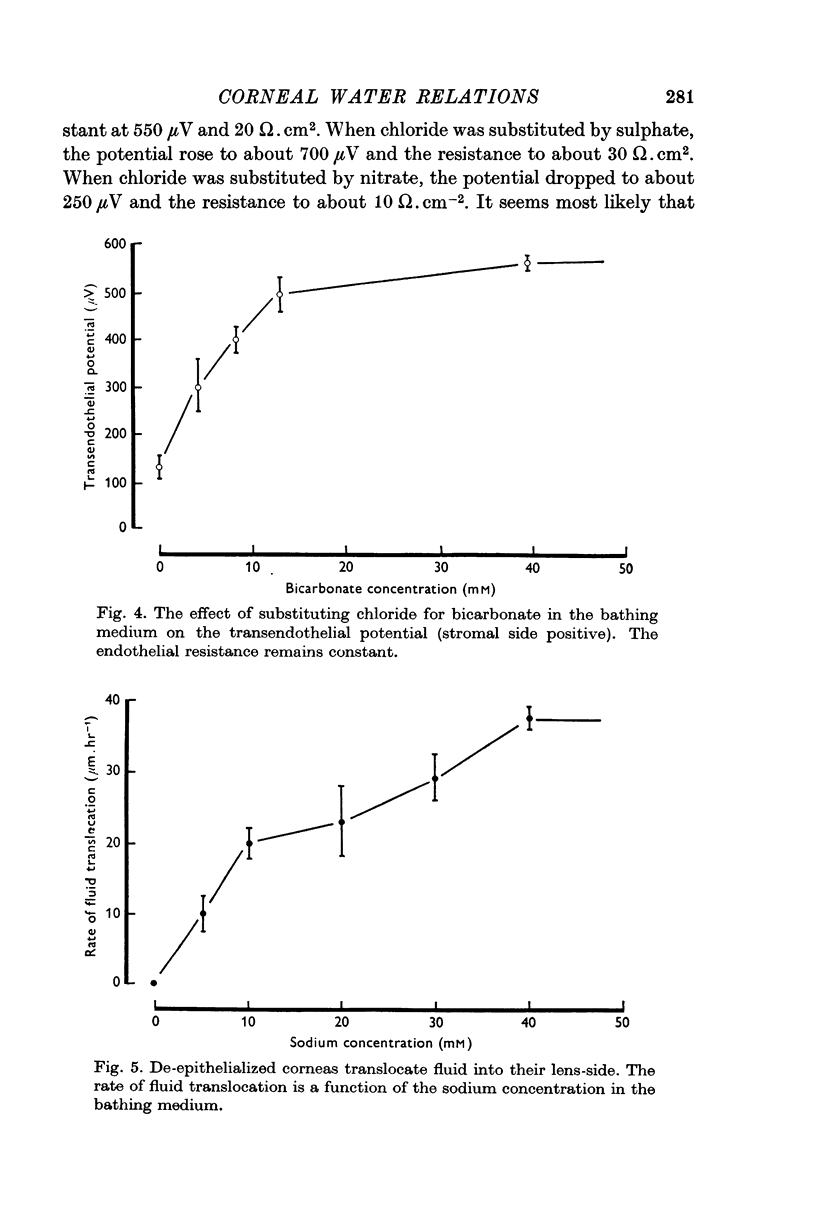
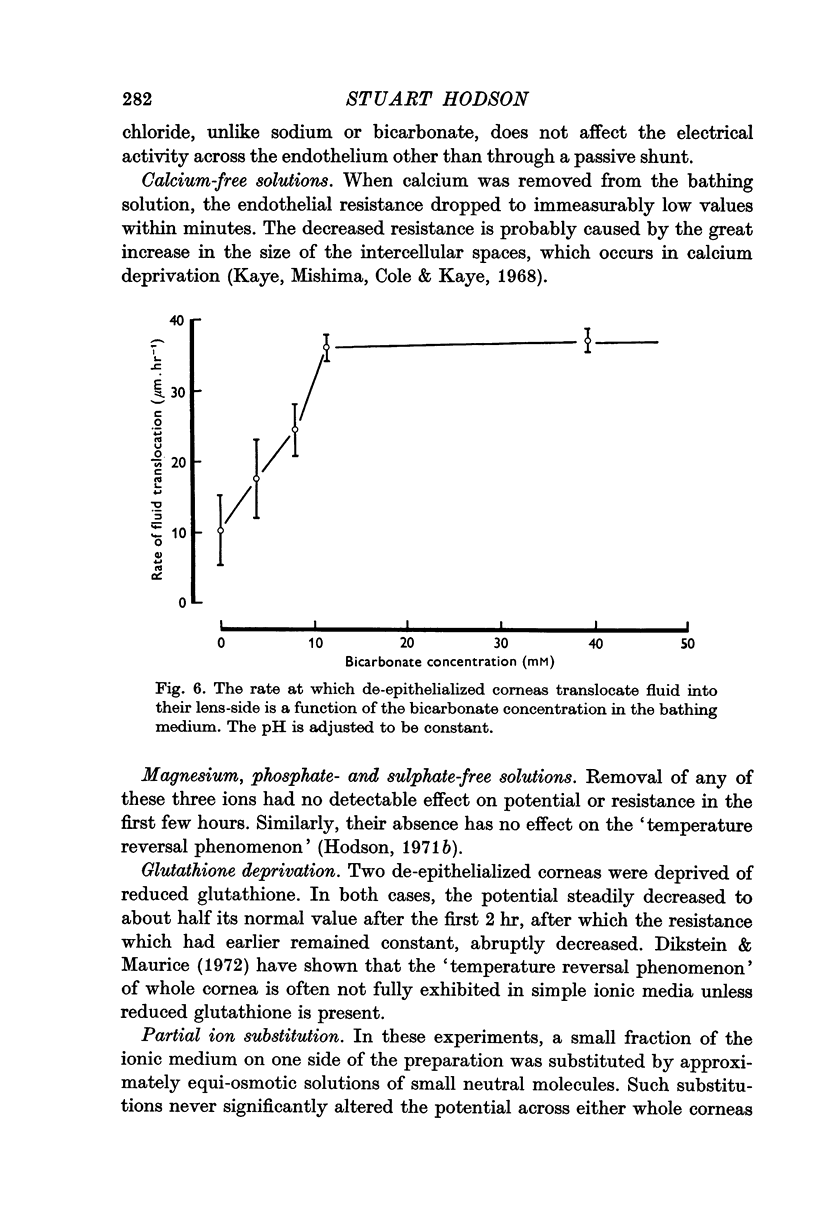
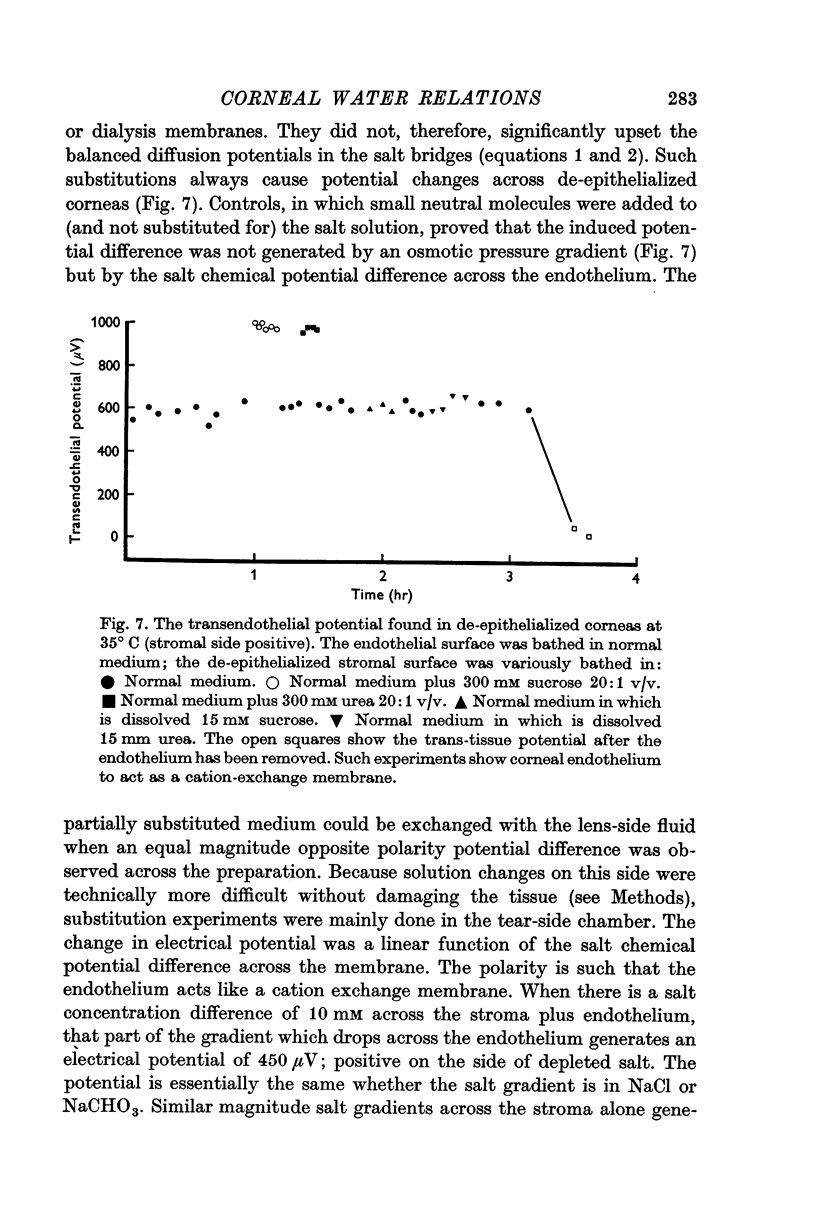
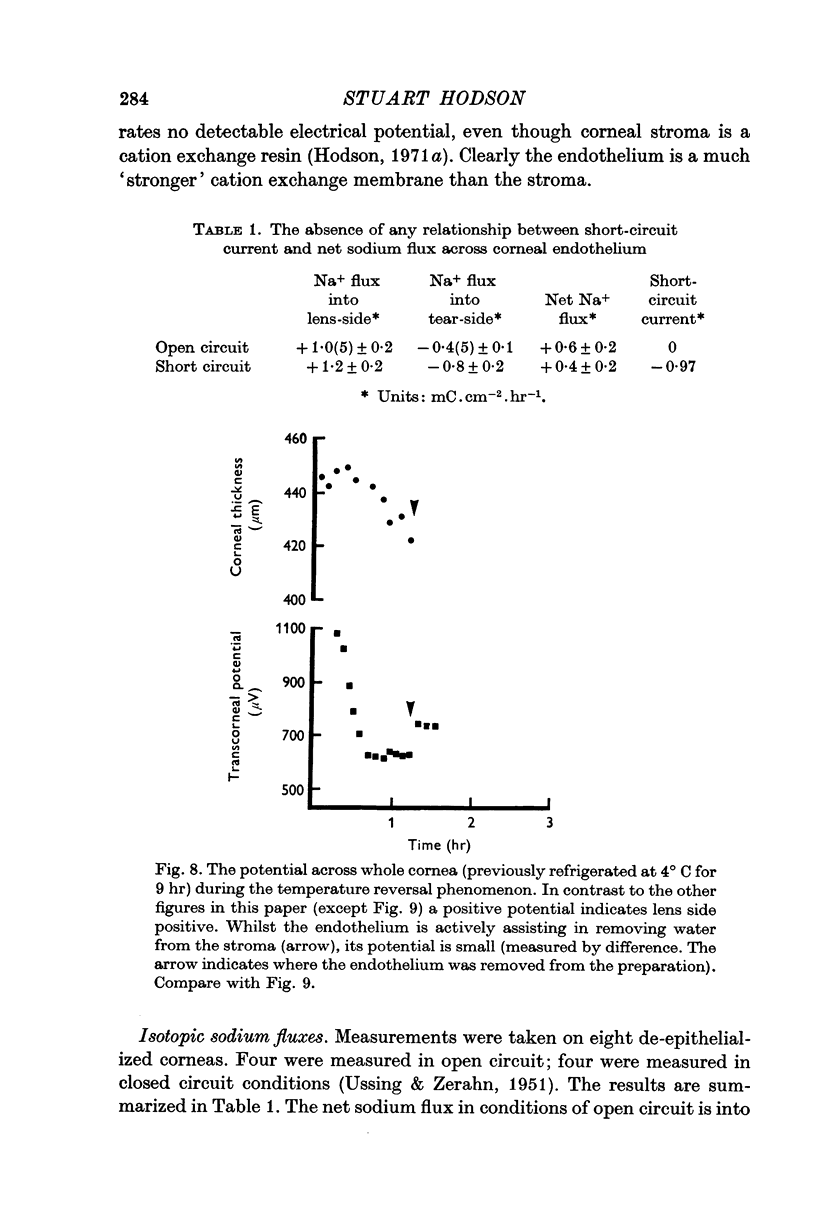
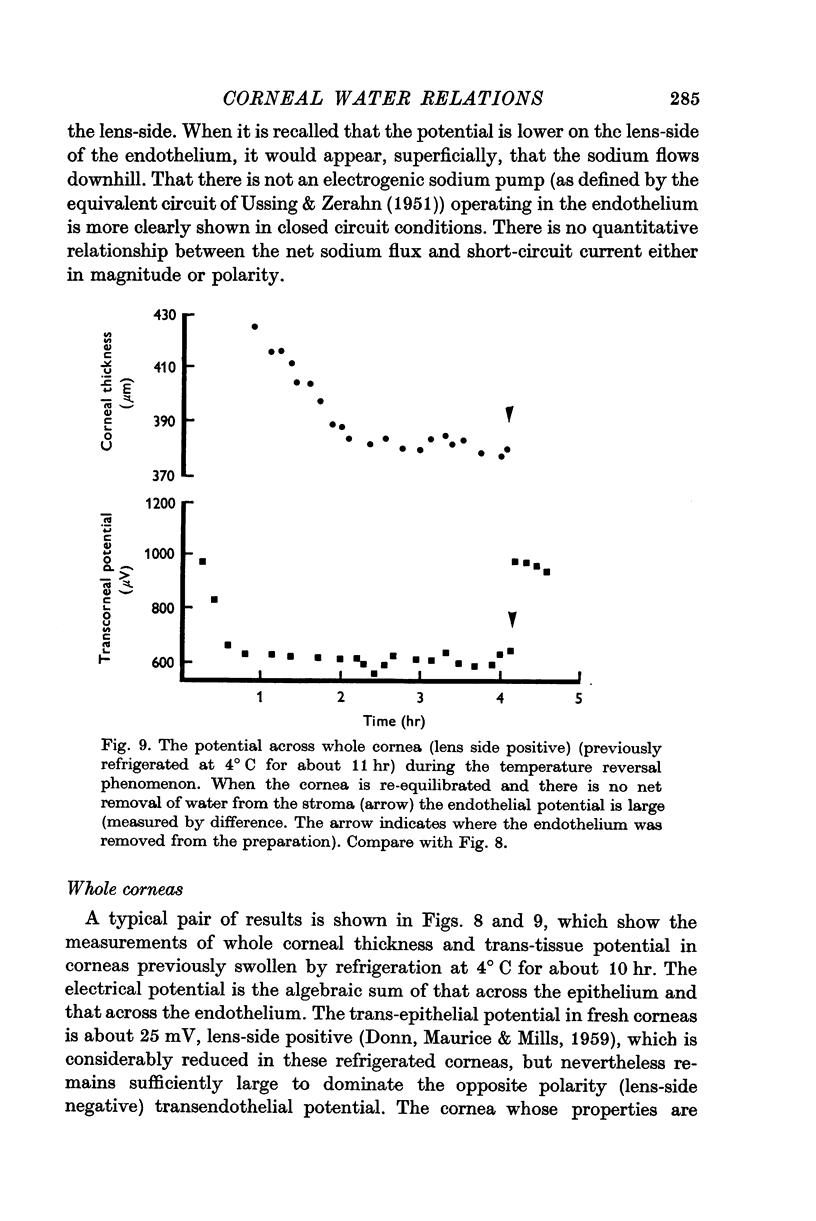
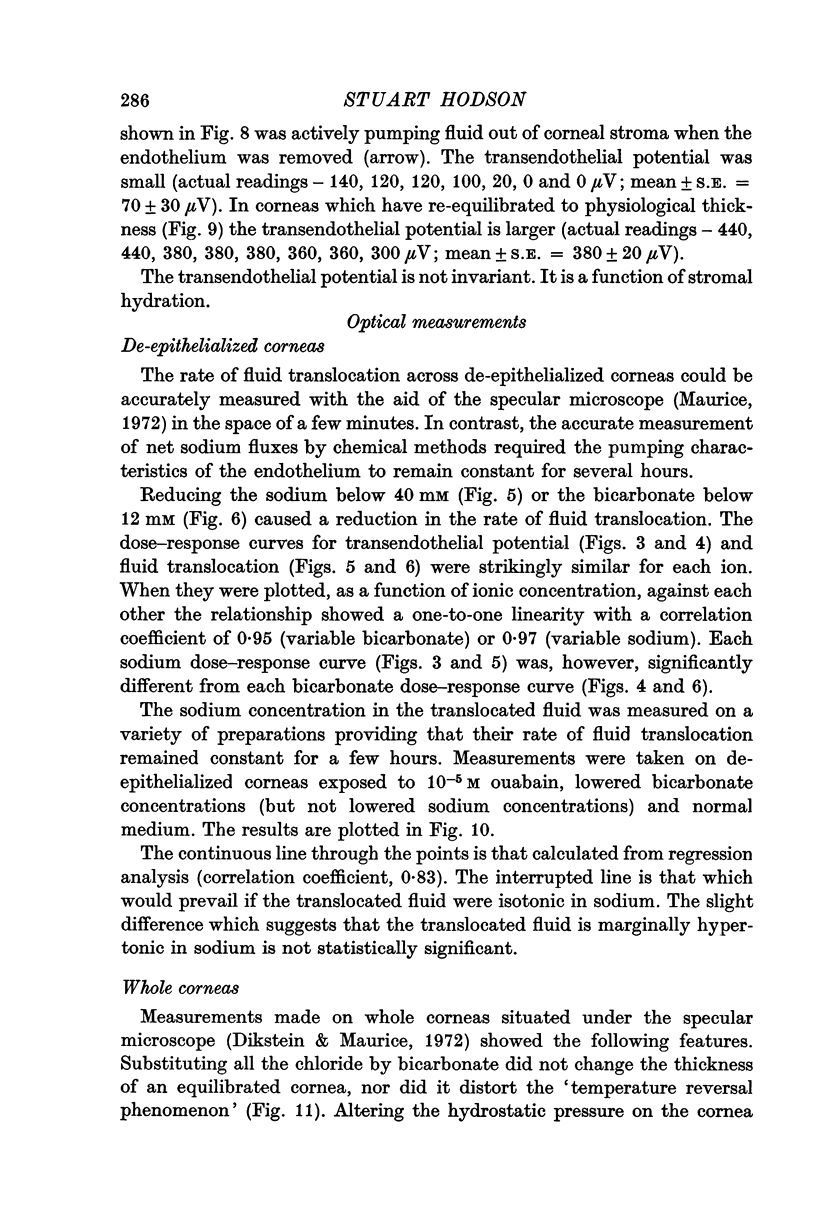
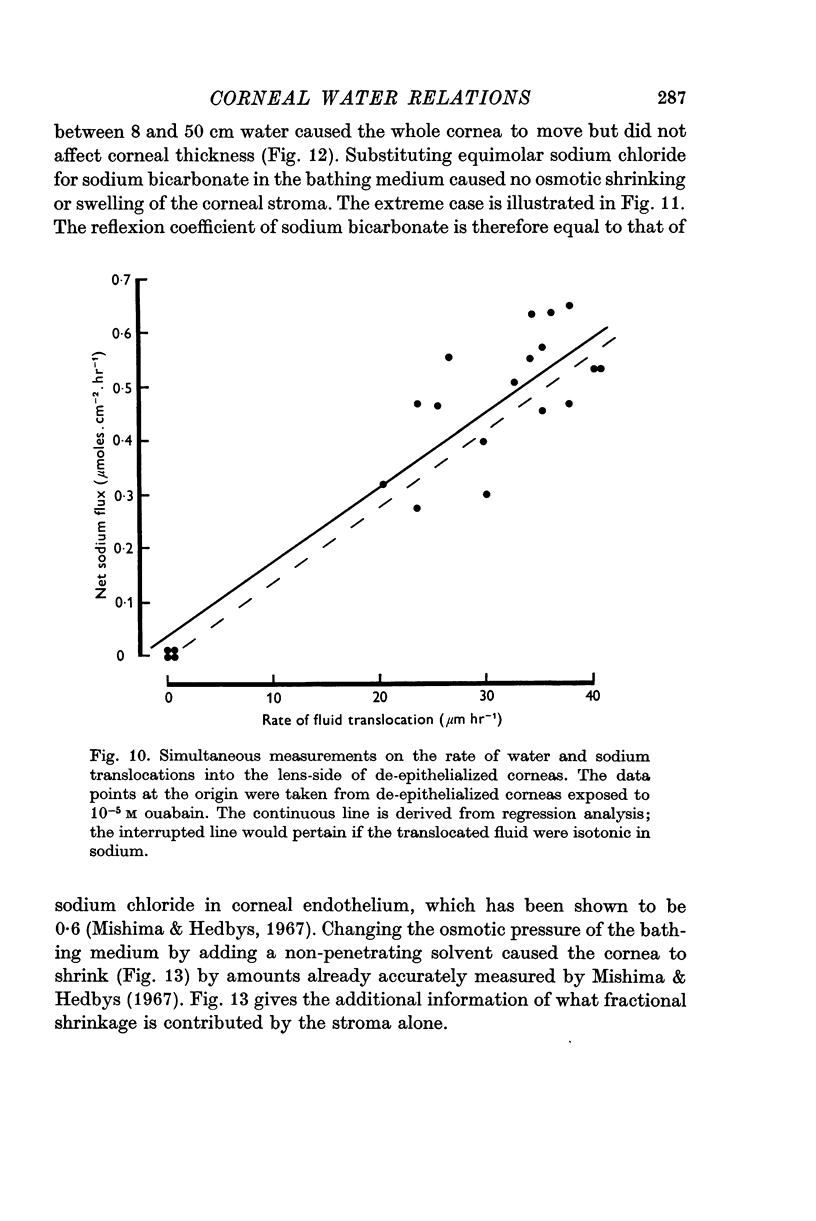
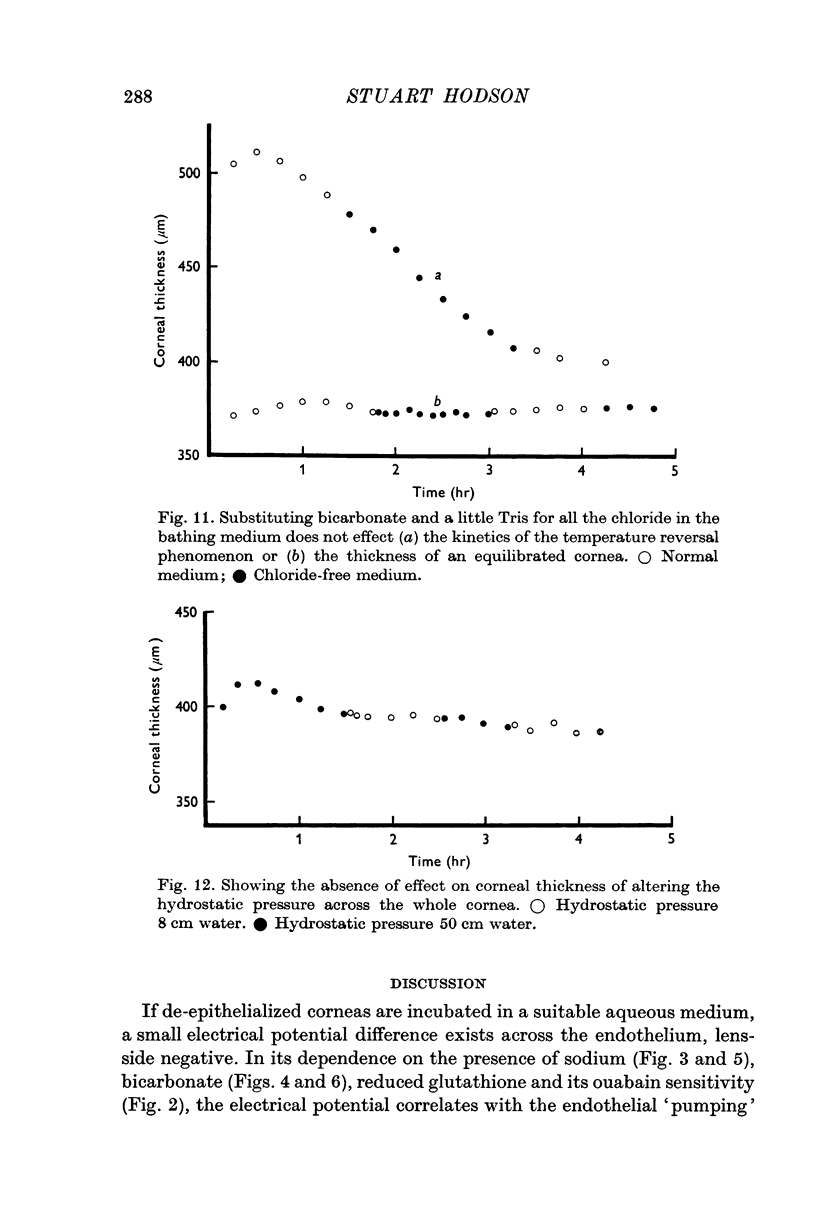
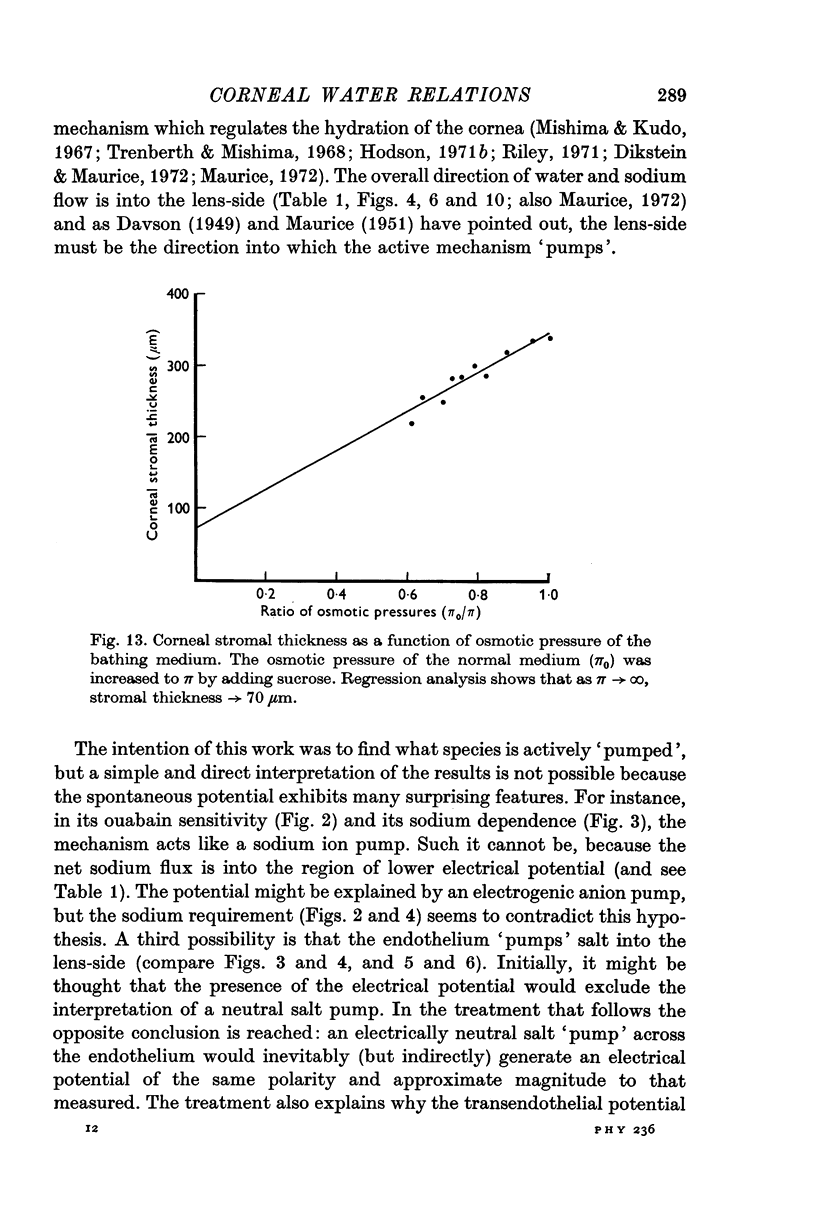
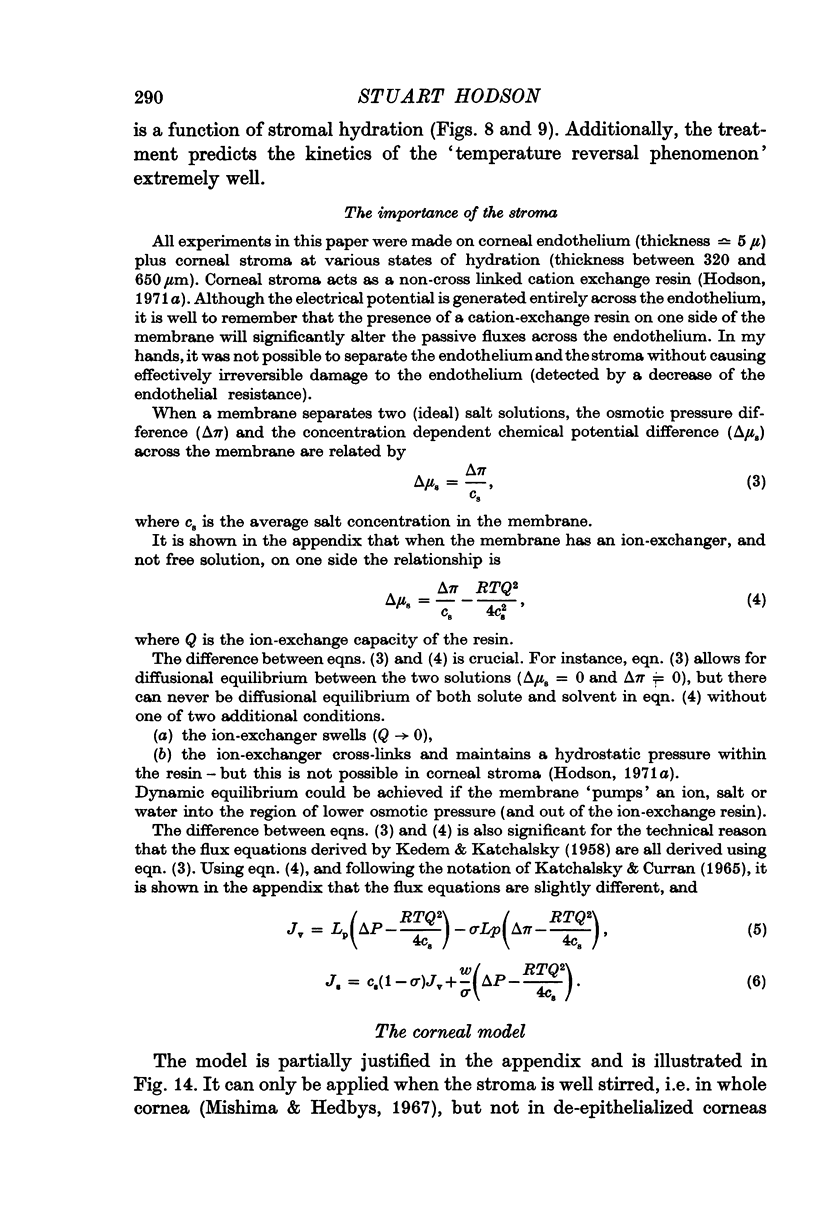
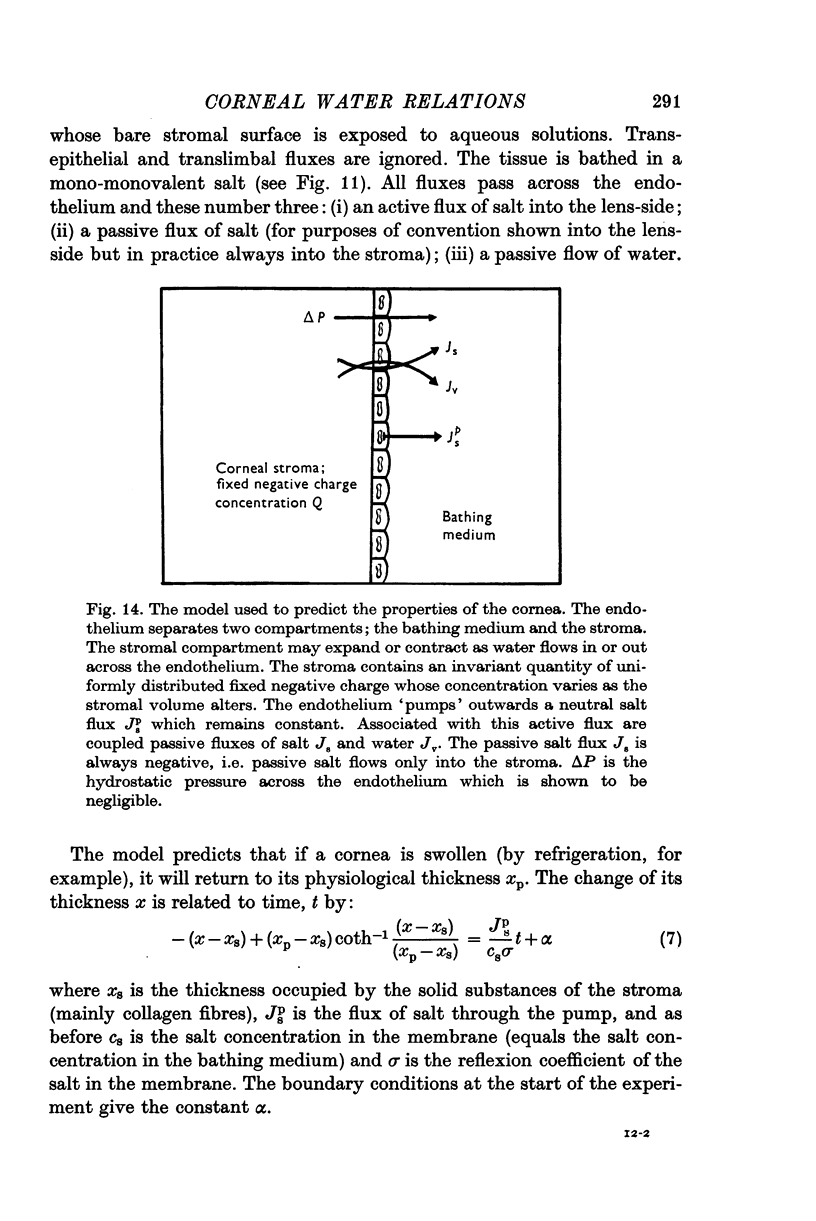
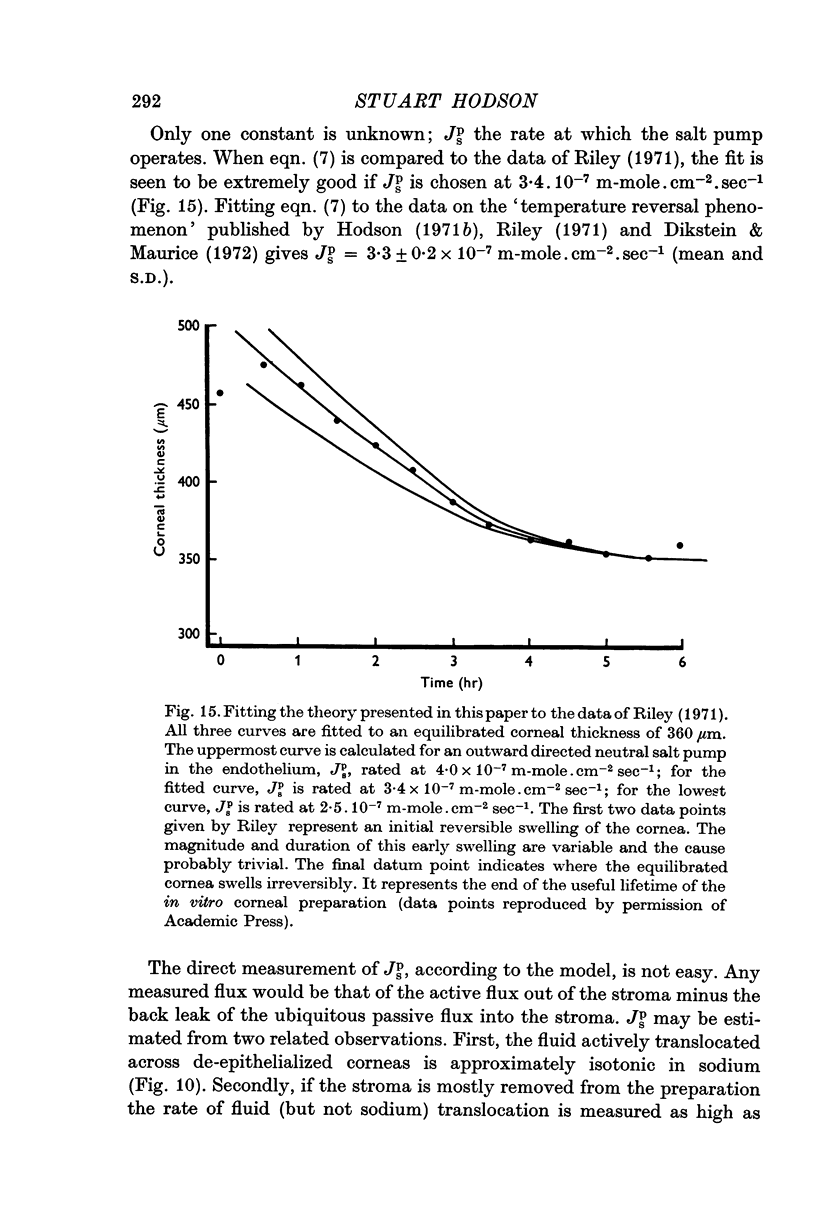
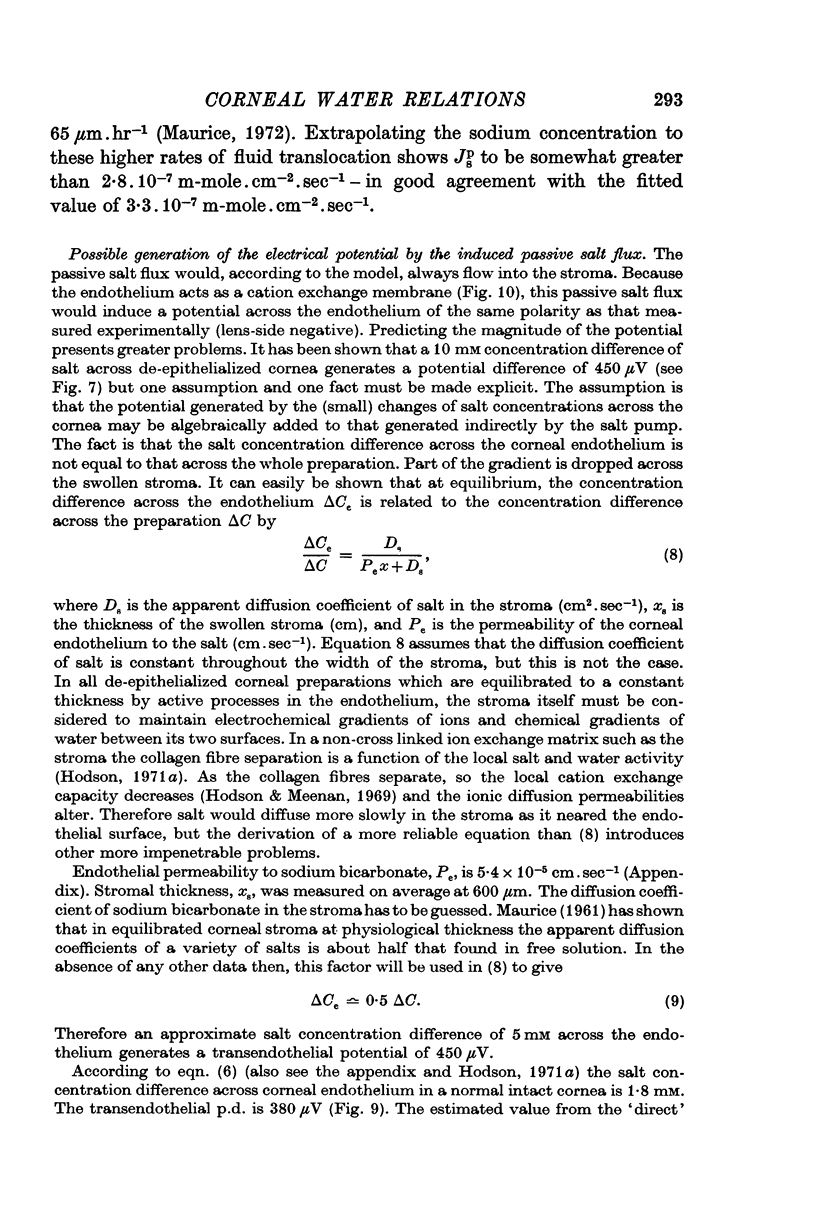
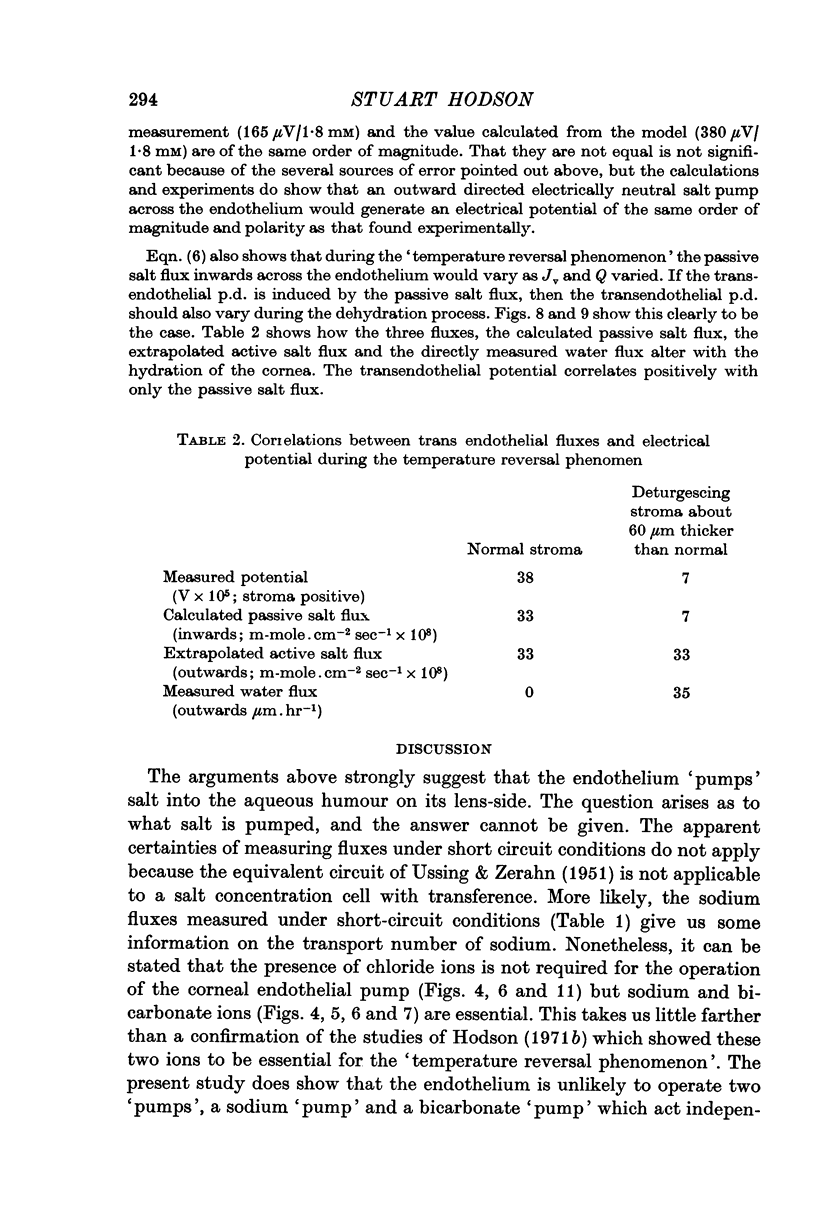
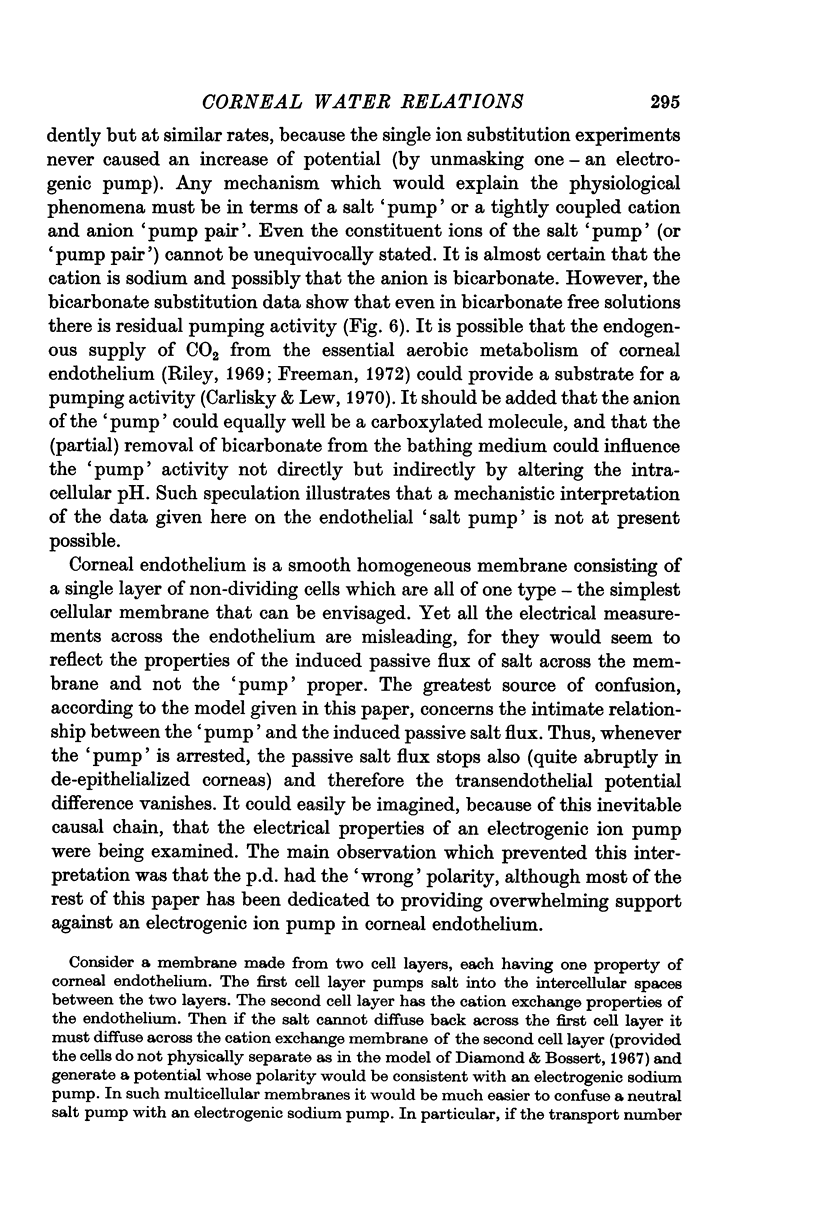
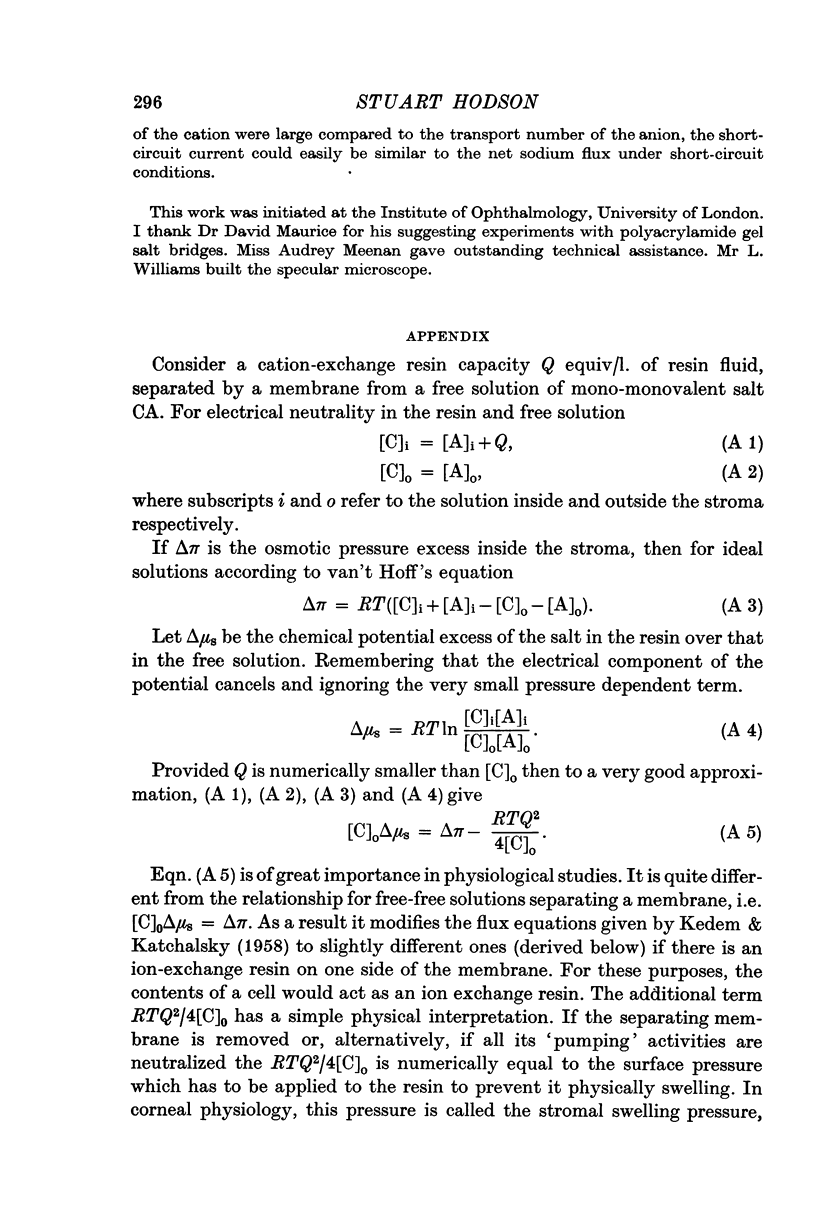
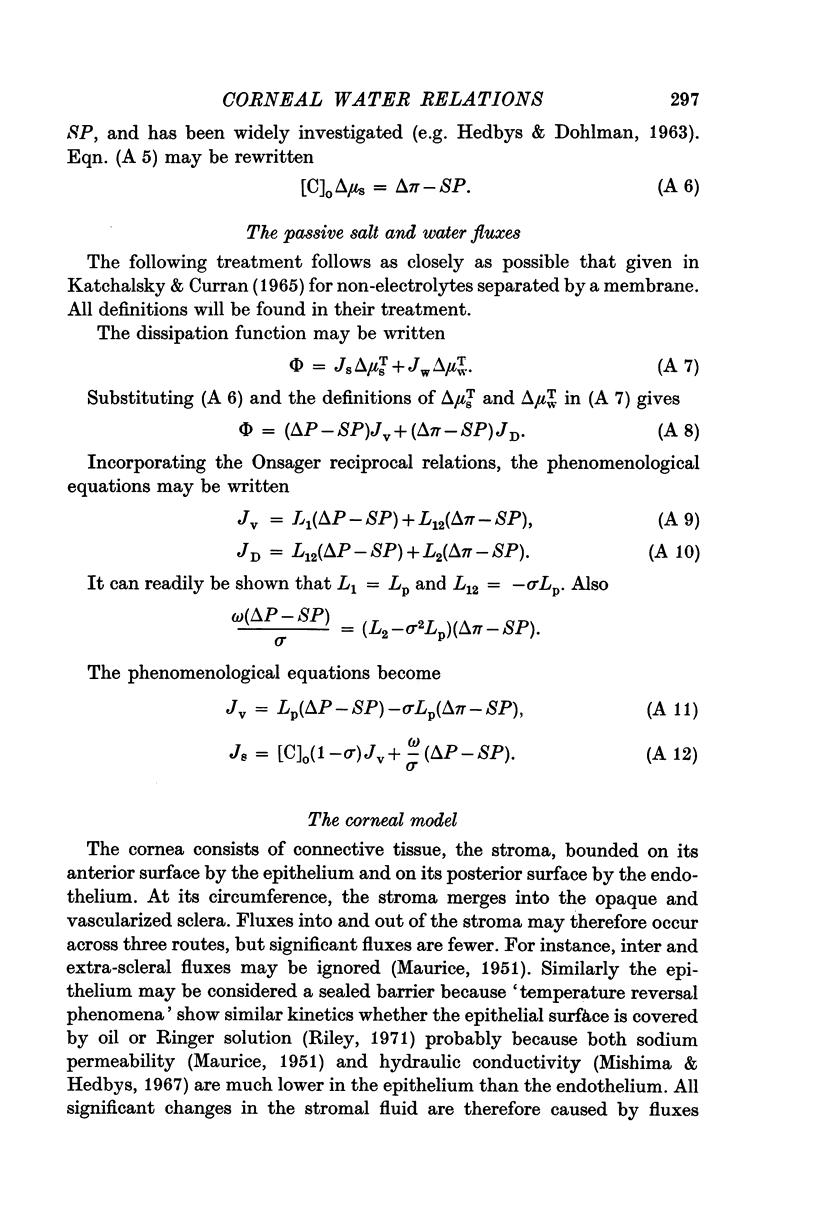
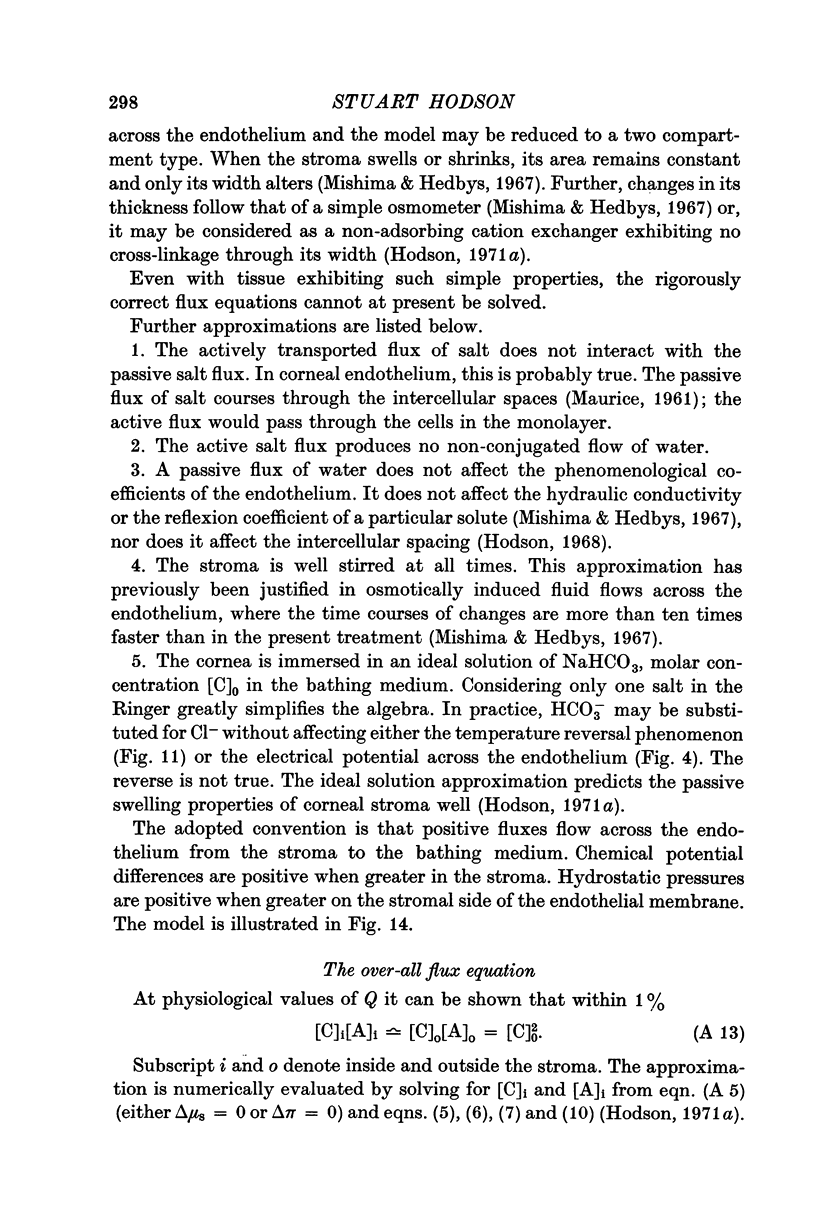
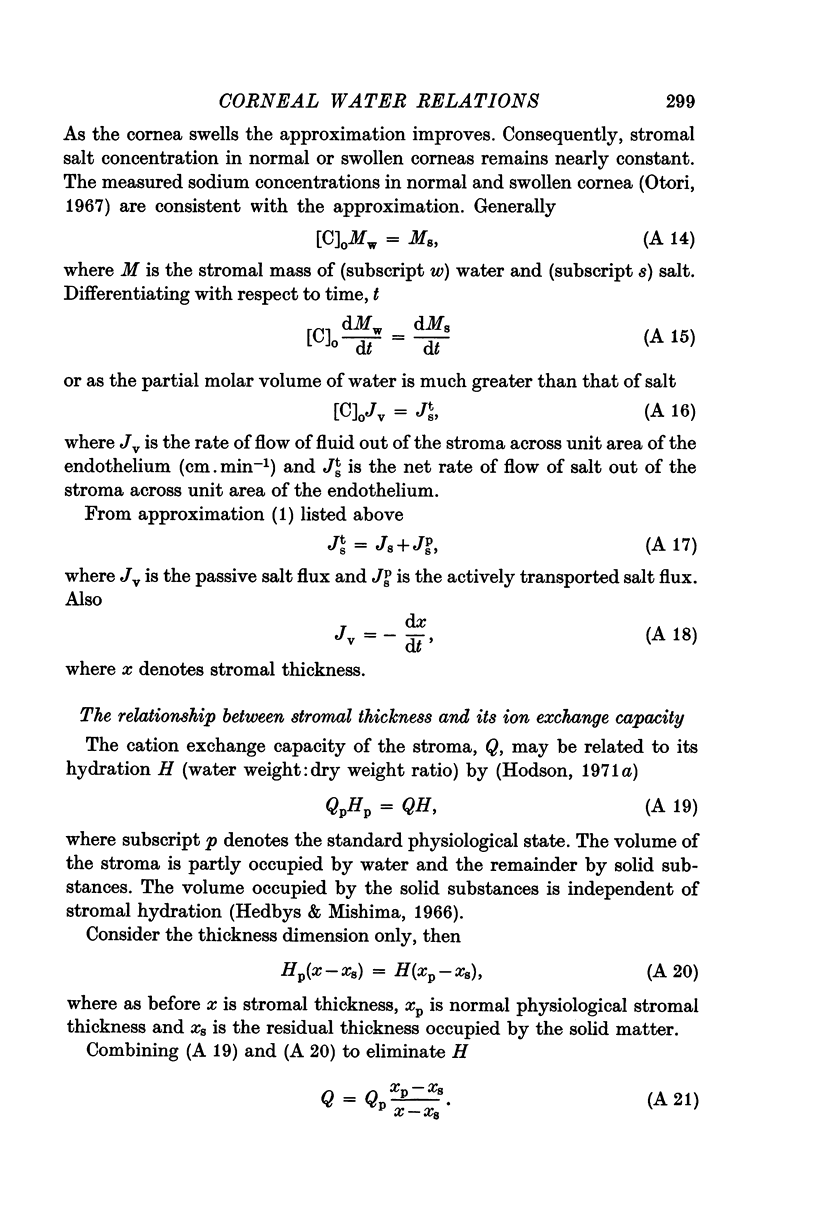
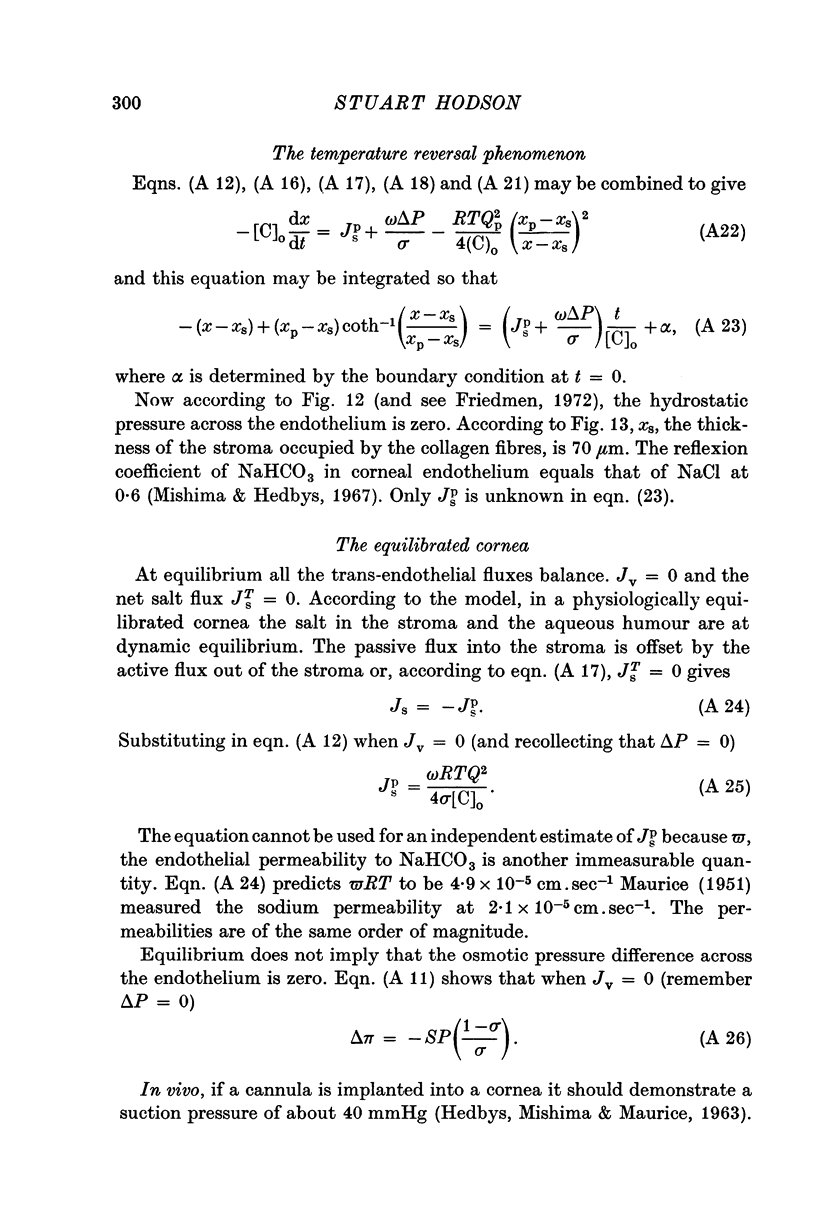
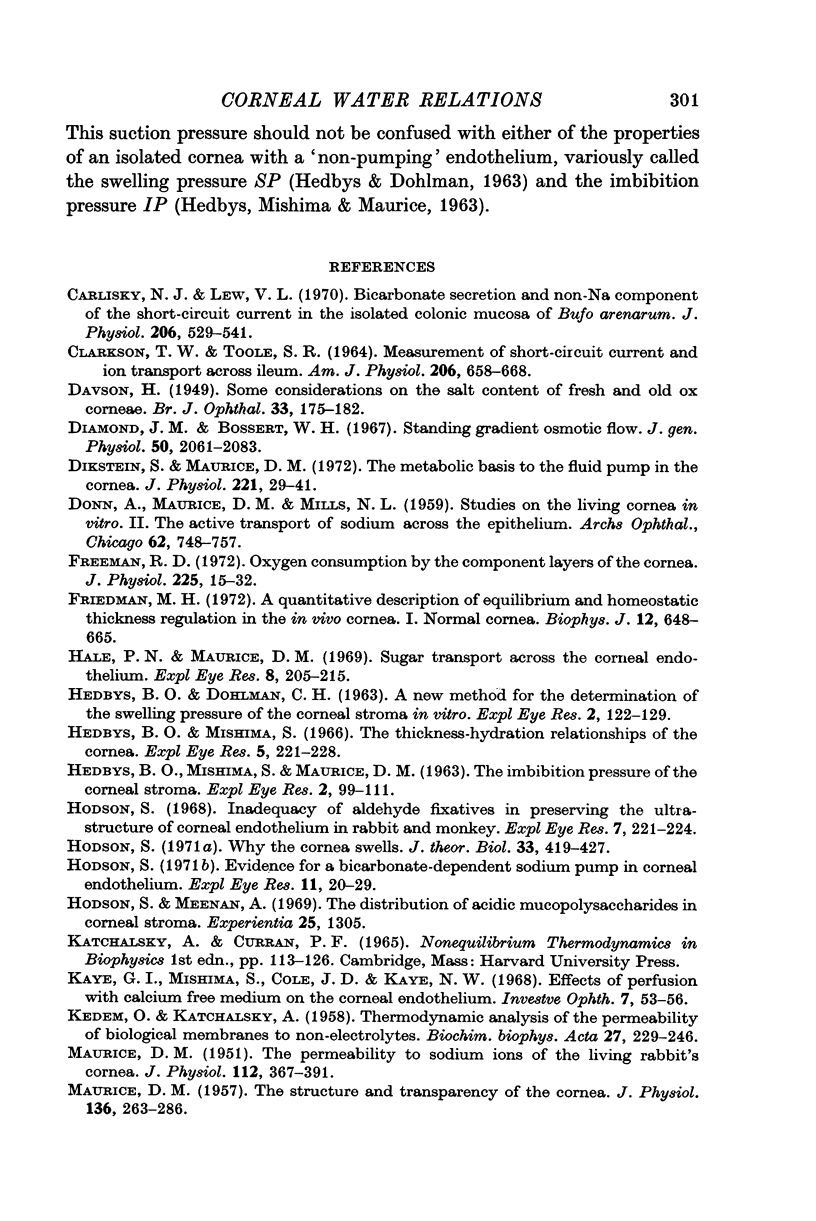
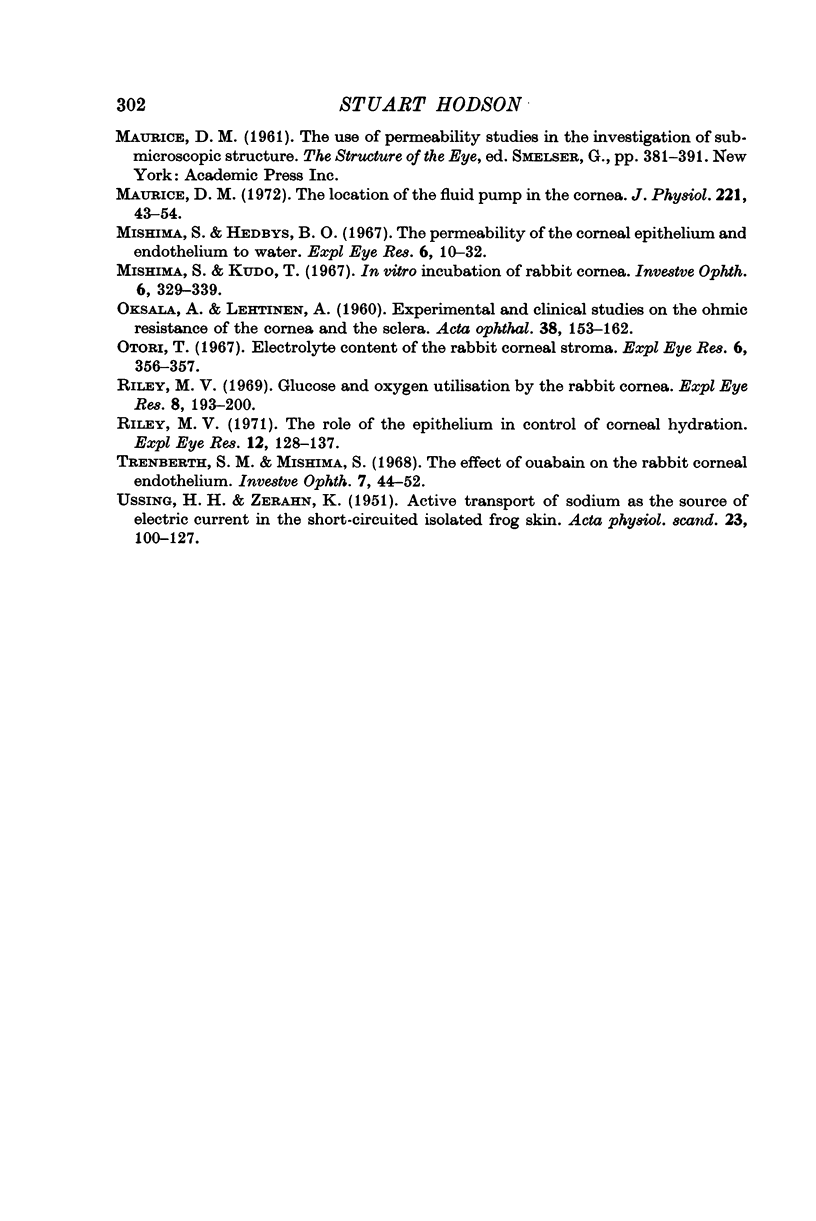
Selected References
These references are in PubMed. This may not be the complete list of references from this article.
- Carlisky N. J., Lew V. L. Bicarbonate secretion and non-Na component of the short-circuit current in the isolated colonic mucosa of Bufo arenarum. J Physiol. 1970 Mar;206(3):529–541. doi: 10.1113/jphysiol.1970.sp009029. [DOI] [PMC free article] [PubMed] [Google Scholar]
- DAVSON H. Some considerations on the salt content of fresh and old ox corneae. Br J Ophthalmol. 1949 Mar;33(3):175–182. doi: 10.1136/bjo.33.3.175. [DOI] [PMC free article] [PubMed] [Google Scholar]
- DONN A., MAURICE D. M., MILLS N. L. Studies on the living cornea in vitro. II. The active transport of sodium across the epithelium. Arch Ophthalmol. 1959 Nov;62:748–757. doi: 10.1001/archopht.1959.04220050010002. [DOI] [PubMed] [Google Scholar]
- Diamond J. M., Bossert W. H. Standing-gradient osmotic flow. A mechanism for coupling of water and solute transport in epithelia. J Gen Physiol. 1967 Sep;50(8):2061–2083. doi: 10.1085/jgp.50.8.2061. [DOI] [PMC free article] [PubMed] [Google Scholar]
- Dikstein S., Maurice D. M. The metabolic basis to the fluid pump in the cornea. J Physiol. 1972 Feb;221(1):29–41. doi: 10.1113/jphysiol.1972.sp009736. [DOI] [PMC free article] [PubMed] [Google Scholar]
- Freeman R. D. Oxygen consumption by the component layers of the cornea. J Physiol. 1972 Aug;225(1):15–32. doi: 10.1113/jphysiol.1972.sp009927. [DOI] [PMC free article] [PubMed] [Google Scholar]
- Friedman M. H. A quantitative description of equilibrium and homeostatic thickness regulation in the in vivo cornea. I. Normal cornea. Biophys J. 1972 Jun;12(6):648–665. doi: 10.1016/S0006-3495(72)86110-1. [DOI] [PMC free article] [PubMed] [Google Scholar]
- HEDBYS B. O., DOHLMAN C. H. A new method for the determination of the swelling pressure of the corneal stroma in vitro. Exp Eye Res. 1963 Apr;2:122–129. doi: 10.1016/s0014-4835(63)80003-2. [DOI] [PubMed] [Google Scholar]
- HEDBYS B. O., MISHIMA S., MAURICE D. M. The inbibition pressure of the corneal stroma. Exp Eye Res. 1963 Apr;2:99–111. doi: 10.1016/s0014-4835(63)80001-9. [DOI] [PubMed] [Google Scholar]
- Hale P. N., Maurice D. M. Sugar transport across the corneal endothelium. Exp Eye Res. 1969 Apr;8(2):205–215. doi: 10.1016/s0014-4835(69)80033-3. [DOI] [PubMed] [Google Scholar]
- Hedbys B. O., Mishima S. The thickness-hydration relationship of the cornea. Exp Eye Res. 1966 Jul;5(3):221–228. doi: 10.1016/s0014-4835(66)80010-6. [DOI] [PubMed] [Google Scholar]
- Hodson S. Evidence for a bicarbonate-dependent sodium pump in corneal endothelium. Exp Eye Res. 1971 Jan;11(1):20–29. doi: 10.1016/s0014-4835(71)80060-x. [DOI] [PubMed] [Google Scholar]
- Hodson S. Inadequacy of aldehyde fixatives in preserving the ultrastructure of corneal endothelium in rabbit and monkey. Exp Eye Res. 1968 Apr;7(2):221–224. doi: 10.1016/s0014-4835(68)80070-3. [DOI] [PubMed] [Google Scholar]
- Hodson S., Meenan A. The distribution of acidic mucopolysaccharides in corneal stroma. Experientia. 1969 Dec 15;25(12):1305–1305. doi: 10.1007/BF01897515. [DOI] [PubMed] [Google Scholar]
- Hodson S. Why the cornea swells. J Theor Biol. 1971 Dec;33(3):419–427. doi: 10.1016/0022-5193(71)90090-7. [DOI] [PubMed] [Google Scholar]
- KEDEM O., KATCHALSKY A. Thermodynamic analysis of the permeability of biological membranes to non-electrolytes. Biochim Biophys Acta. 1958 Feb;27(2):229–246. doi: 10.1016/0006-3002(58)90330-5. [DOI] [PubMed] [Google Scholar]
- Kaye G. I., Mishima S., Cole J. D., Kaye N. W. Studies on the cornea. VII. Effects of perfusion with a Ca++-free medium on the corneal endothelium. Invest Ophthalmol. 1968 Feb;7(1):53–66. [PubMed] [Google Scholar]
- MAURICE D. M. The permeability to sodium ions of the living rabbit's cornea. J Physiol. 1951 Feb;112(3-4):367–391. doi: 10.1113/jphysiol.1951.sp004535. [DOI] [PMC free article] [PubMed] [Google Scholar]
- MAURICE D. M. The structure and transparency of the cornea. J Physiol. 1957 Apr 30;136(2):263–286. doi: 10.1113/jphysiol.1957.sp005758. [DOI] [PMC free article] [PubMed] [Google Scholar]
- Maurice D. M. The location of the fluid pump in the cornea. J Physiol. 1972 Feb;221(1):43–54. doi: 10.1113/jphysiol.1972.sp009737. [DOI] [PMC free article] [PubMed] [Google Scholar]
- Mishima S., Hedbys B. O. The permeability of the corneal epithelium and endothelium to water. Exp Eye Res. 1967 Jan;6(1):10–32. doi: 10.1016/s0014-4835(67)80049-6. [DOI] [PubMed] [Google Scholar]
- OKSALA A., LEHTINEN A. Experimental and clinical studies on the ohmic resistance of the cornea and the sclera. Acta Ophthalmol (Copenh) 1960;38:153–162. doi: 10.1111/j.1755-3768.1960.tb00192.x. [DOI] [PubMed] [Google Scholar]
- Otori T. Electrolyte content of the rabbit corneal stroma. Exp Eye Res. 1967 Oct;6(4):356–367. doi: 10.1016/s0014-4835(67)80010-1. [DOI] [PubMed] [Google Scholar]
- Riley M. V. The role of the epithelium in control of corneal hydration. Exp Eye Res. 1971 Jul;12(1):128–137. doi: 10.1016/0014-4835(71)90137-0. [DOI] [PubMed] [Google Scholar]
- Trenberth S. M., Mishima S. The effect of ouabain on the rabbit corneal endothelium. Invest Ophthalmol. 1968 Feb;7(1):44–52. [PubMed] [Google Scholar]
- USSING H. H., ZERAHN K. Active transport of sodium as the source of electric current in the short-circuited isolated frog skin. Acta Physiol Scand. 1951 Aug 25;23(2-3):110–127. doi: 10.1111/j.1748-1716.1951.tb00800.x. [DOI] [PubMed] [Google Scholar]