Abstract
1. Dissociation constants for sodium and potassium of a site that modulates the rate of ouabain-(Na++K+)-ATPase interaction were applied to models for potassium activation of (Na++K+)-ATPase. The constants for potassium (0·213 mM) and for sodium (13·7 mM) were defined, respectively, as activation constant, Ka and inhibitory constant, Ki.
2. Tests of the one- and the two-equivalent site models, that describe sodium and potassium competition, revealed that neither model adequately predicts the activation effects of potassium in the presence of 100 or 200 mM sodium.
3. The potassium-activation data, obtained at low potassium and high sodium, were explained by a two-nonequivalent site model where the dissociation constants of the first site are 0·213 mM for potassium and 13·7 mM for sodium. The second site was characterized by dissociation constants of 0·091 mM for potassium and 74·1 mM for sodium.
4. The two-nonequivalent site model adequately predicted the responses to concentrations of potassium between 0·25 and 5 mM in the presence of 100-500 mM sodium. At lower sodium concentrations the predicted responses formed an upper limit for the function of observed activities. This limit was reached at lower concentrations of potassium and higher concentrations of sodium, which inferred saturation of the sodium-activation sites with sodium.
5. Sodium-activation data were corrected for sodium interaction with potassium-activation sites by use of the two-nonequivalent site model for potassium activation. Tests of equivalent site models suggested that the corrected data for sodium activation may be most consistent with a model that has three-equivalent sites. Other multiequivalent site models (n = 2, 4, 5 or 6), however, cannot be statistically eliminated as possibilities. The three-equivalent site activation model was characterized by dissociation constants of 1·39 mM for sodium and 11·7 mM for potassium. The system theoretically would be half-maximally activated by 5·35 mM sodium in the absence of potassium.
6. Derivation of the model for sodium activation assumed that the affinities of these sites for sodium and potassium are independent of cation interactions with the potassium-activation sites. Therefore, the kinetic descriptions for sodium and potassium effects form a composite model that is consistent with simultaneous transport of sodium and potassium.
7. Predictions of the composite equation are in reasonable agreement with data obtained by variation of sodium (potassium = 10 mM), variation of potassium (sodium = 100 mM) and by simultaneous variation of sodium and potassium (sodium:potassium = 10). Sodium-activation data (2·5-20 mM sodium) also agree with predictions of the model in the presence of potassium concentrations which are thought to be present at the sodium-activation sites in vivo.
8. The kinetic description for sodium (three-equivalent sites) and potassium (two-nonequivalent sites) activation of the transport-ATPase is in accord with the probable stoichiometric requirements of the sodium pump. The model is also in general agreement with other studies on intact transporting systems and (Na++K+)-ATPase in fragmented membrane preparations with respect to potassium activation, although there is a quantitative disagreement. The model for sodium activation, though consistent with data obtained by other studies on fragmented (Na++K+)-ATPase preparations, is in apparent variance with much of the data obtained for intact transporting systems. The description for potassium activation suggests that the rates of ouabain binding to (Na++K+)-ATPase are modulated by competition between sodium and potassium for one of the two potassium-activation sites.
Full text
PDF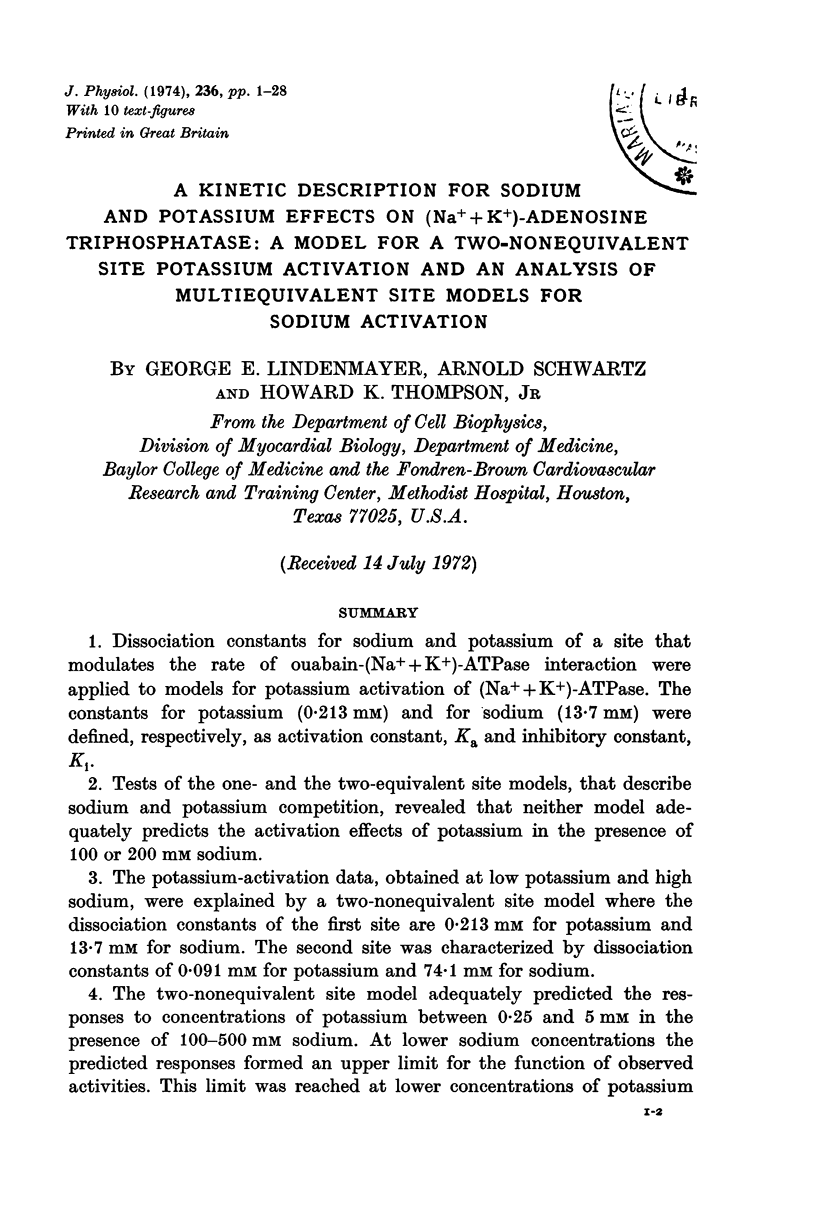
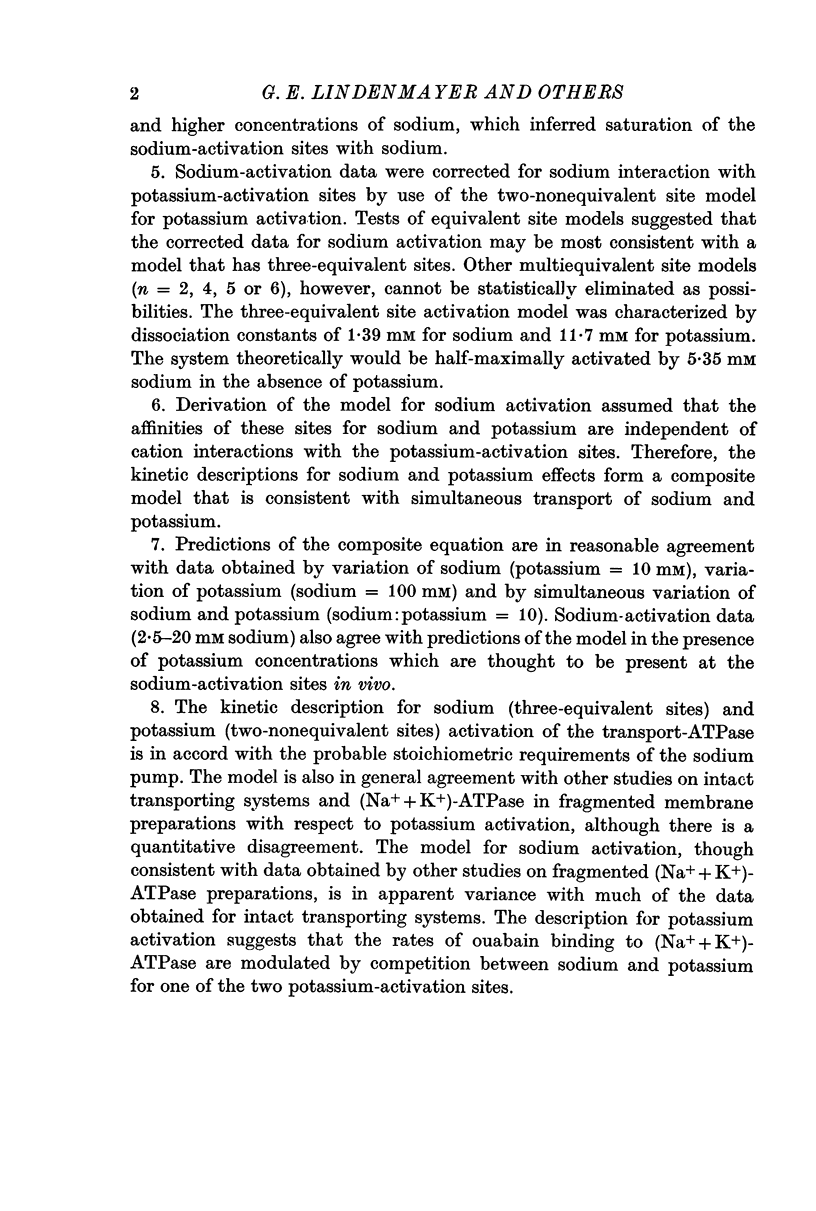
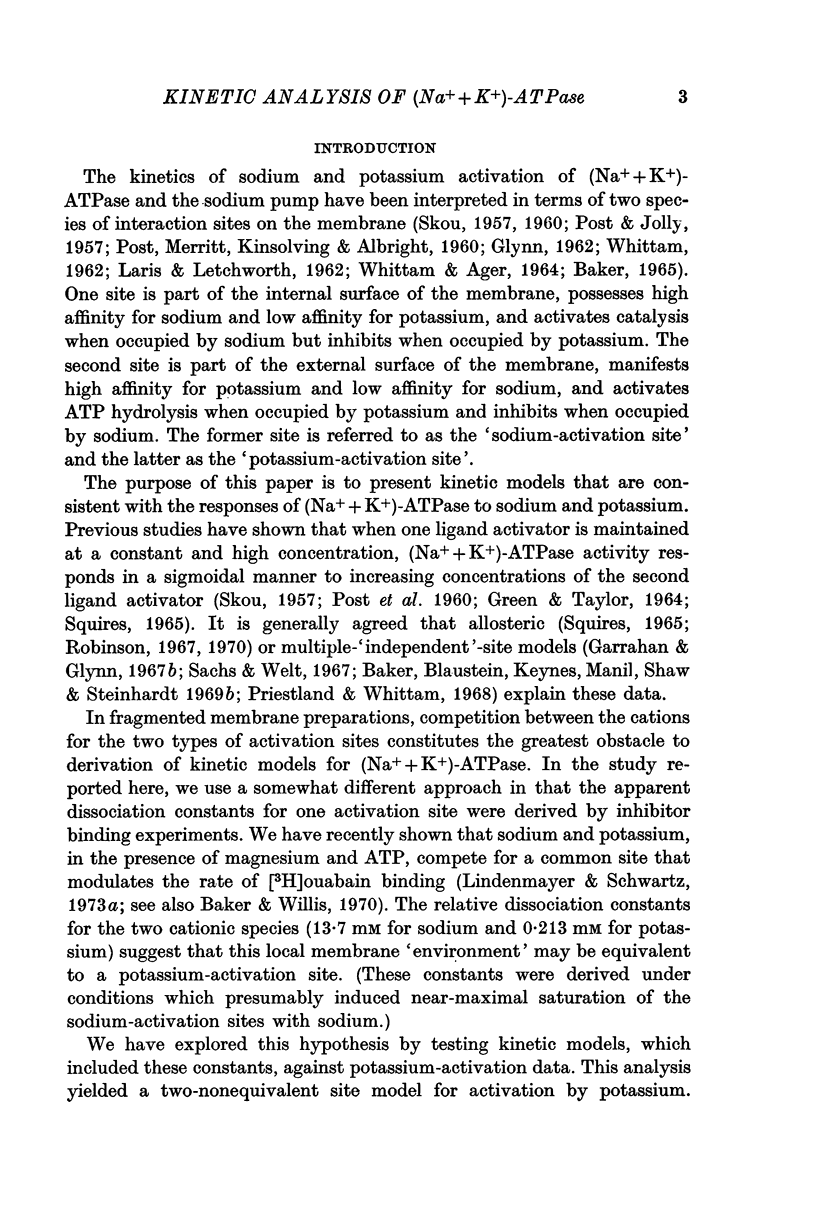
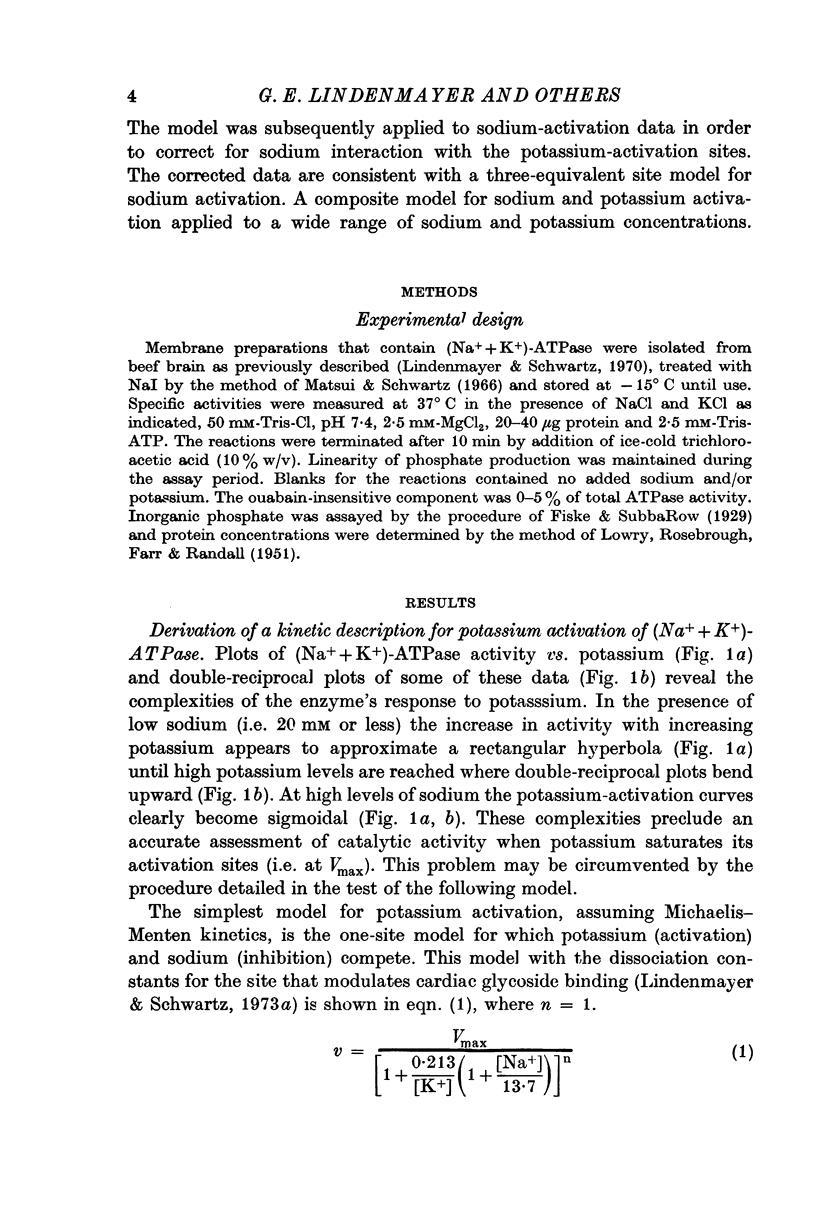
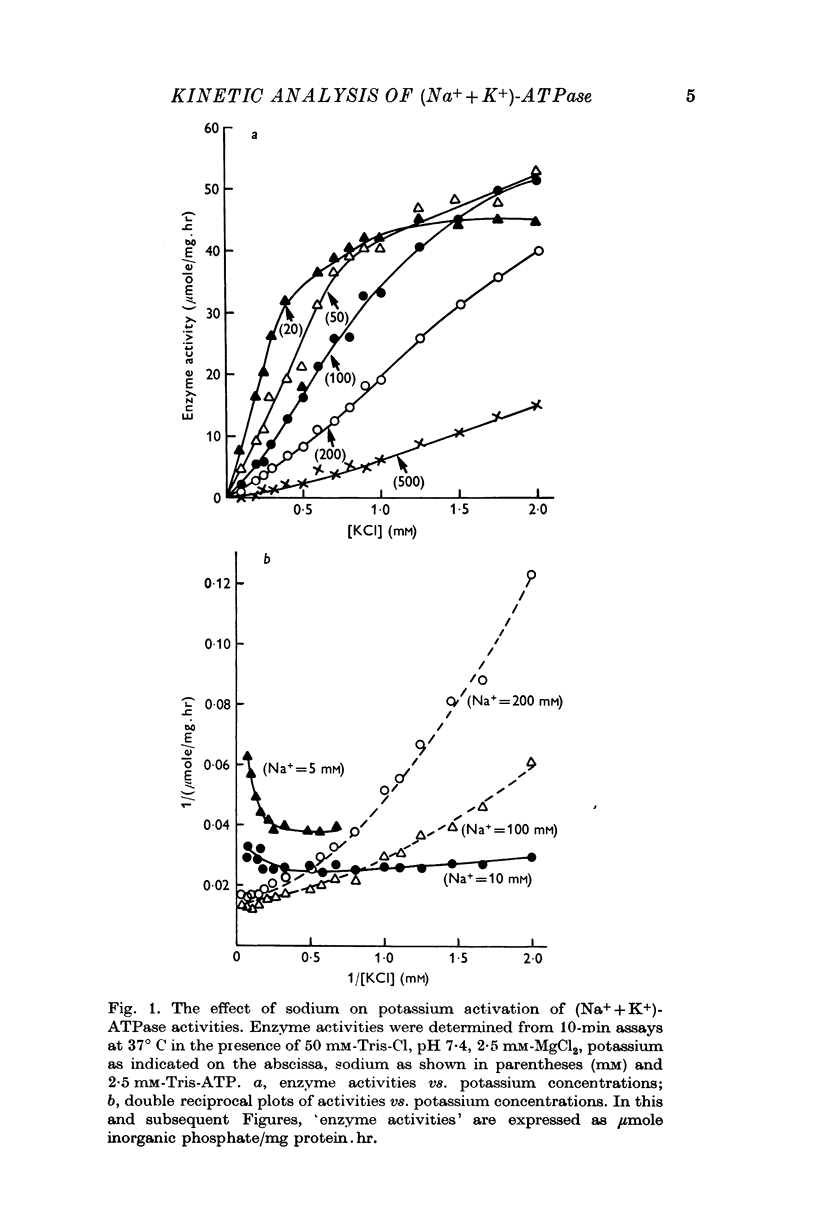
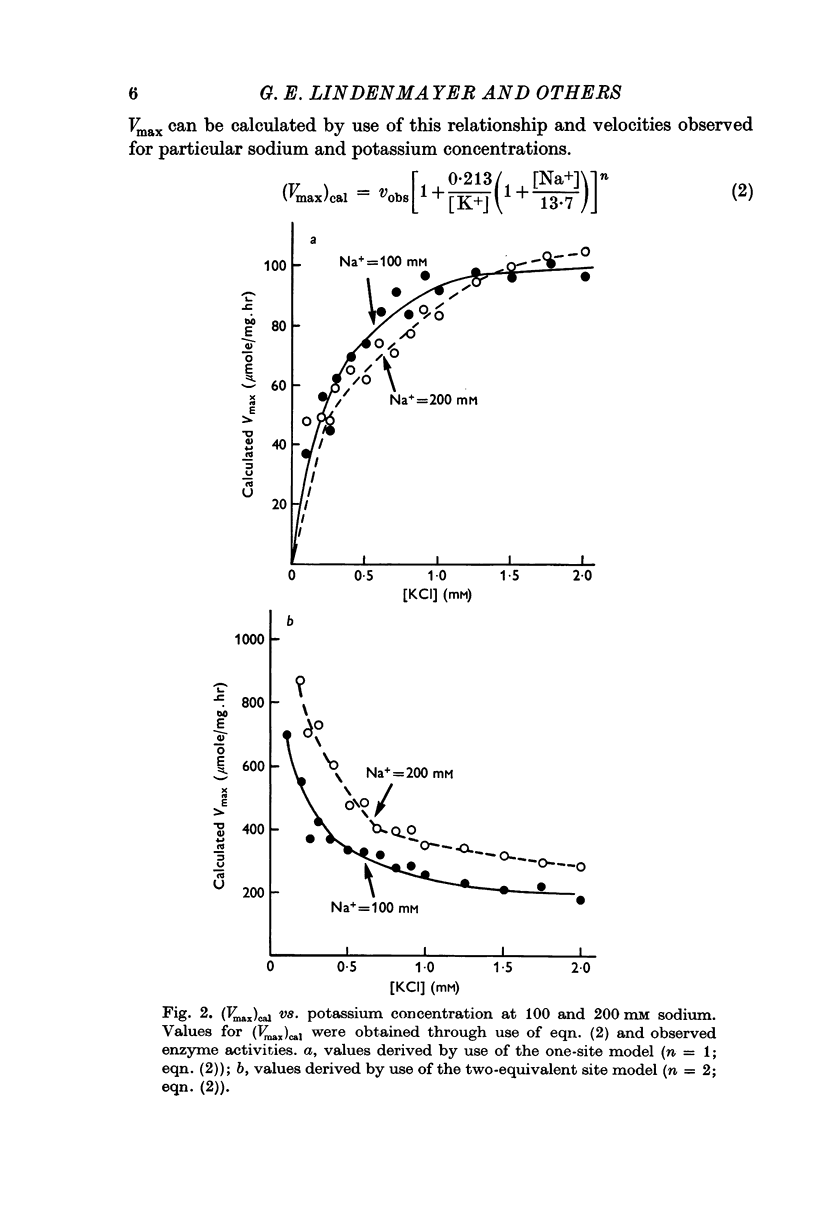
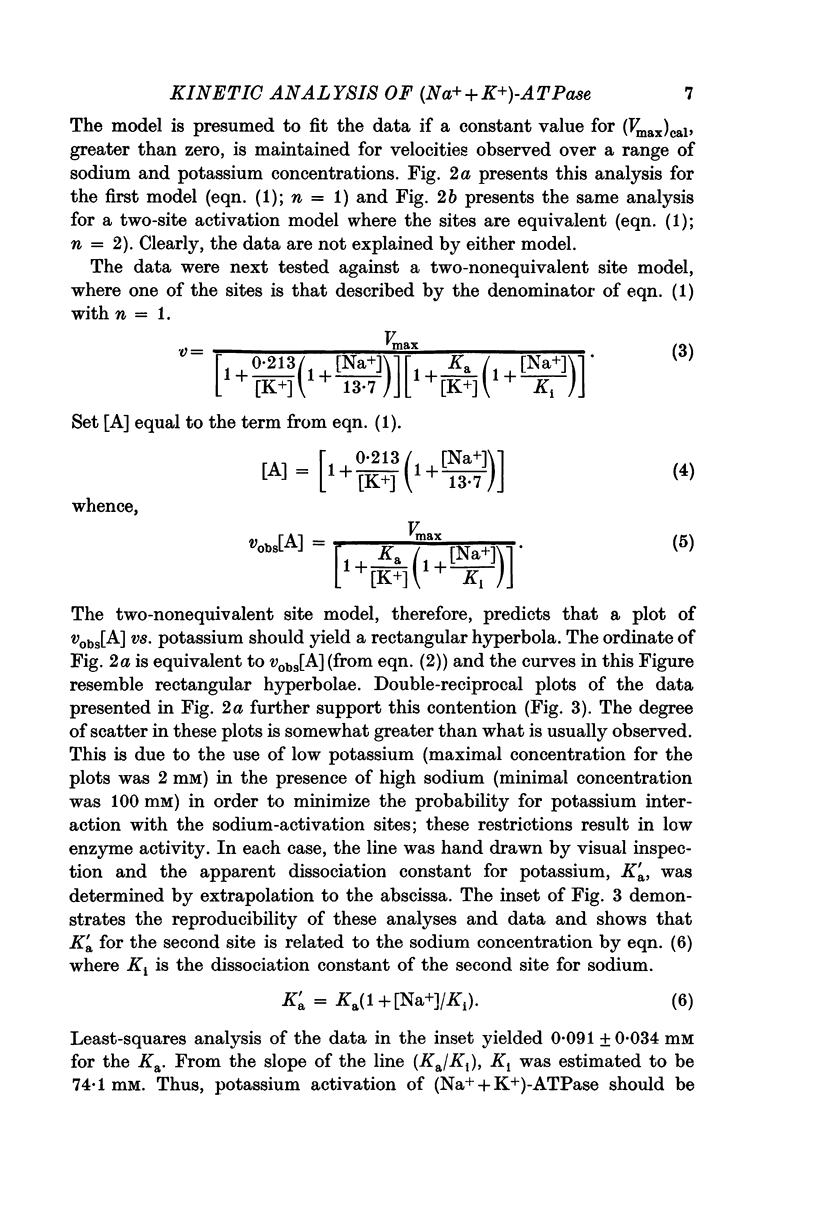
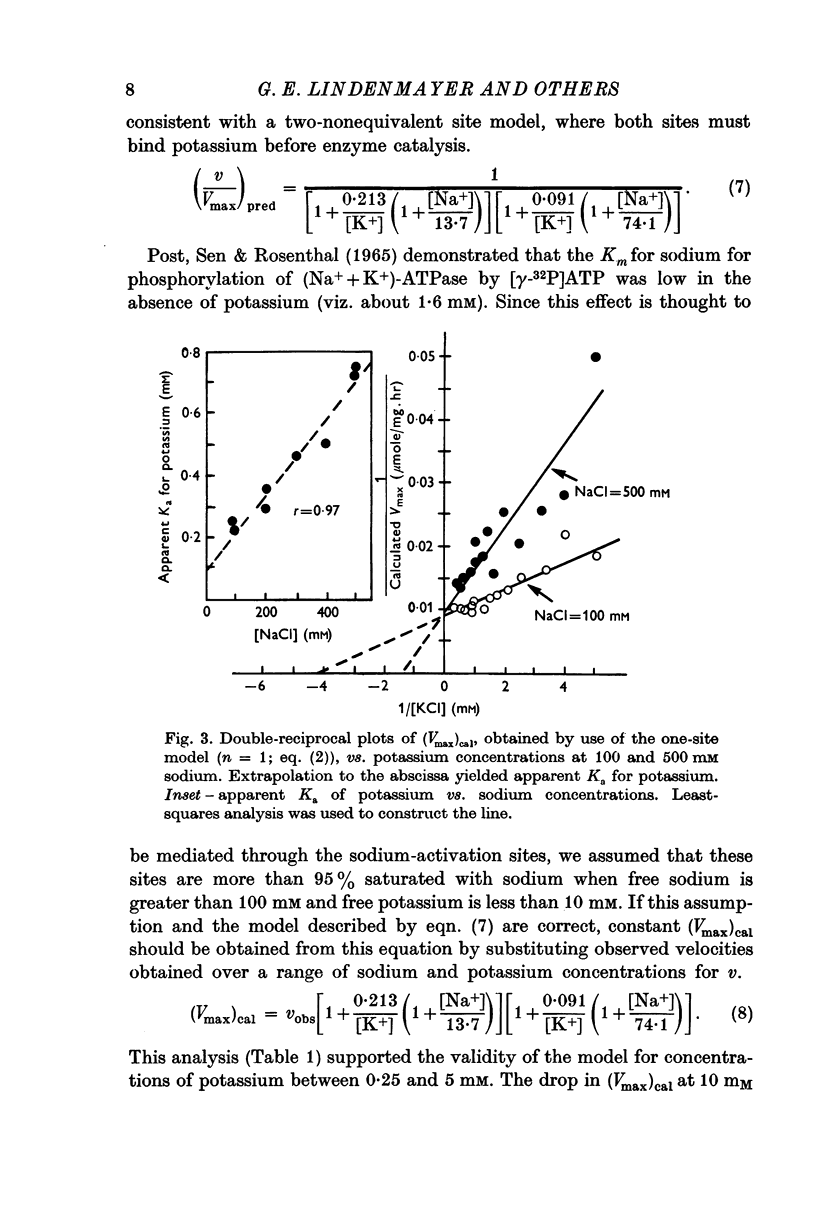
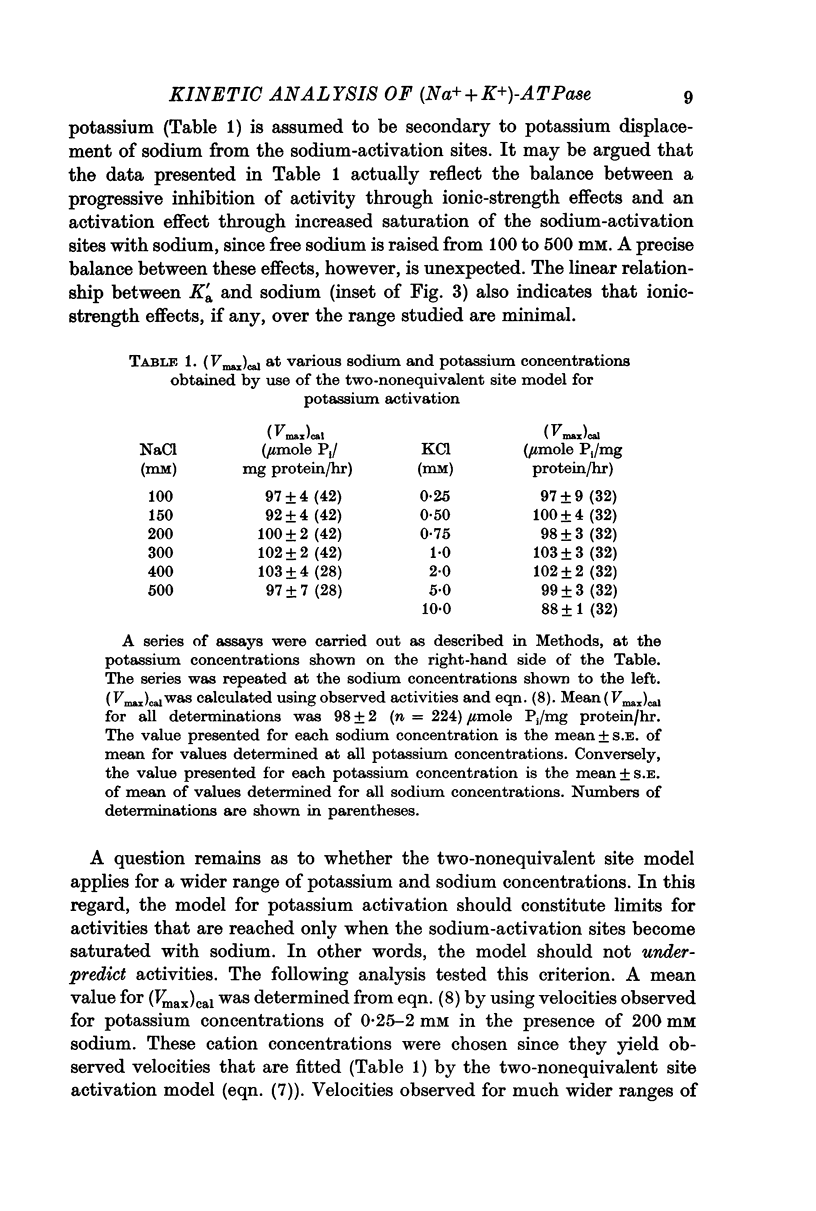
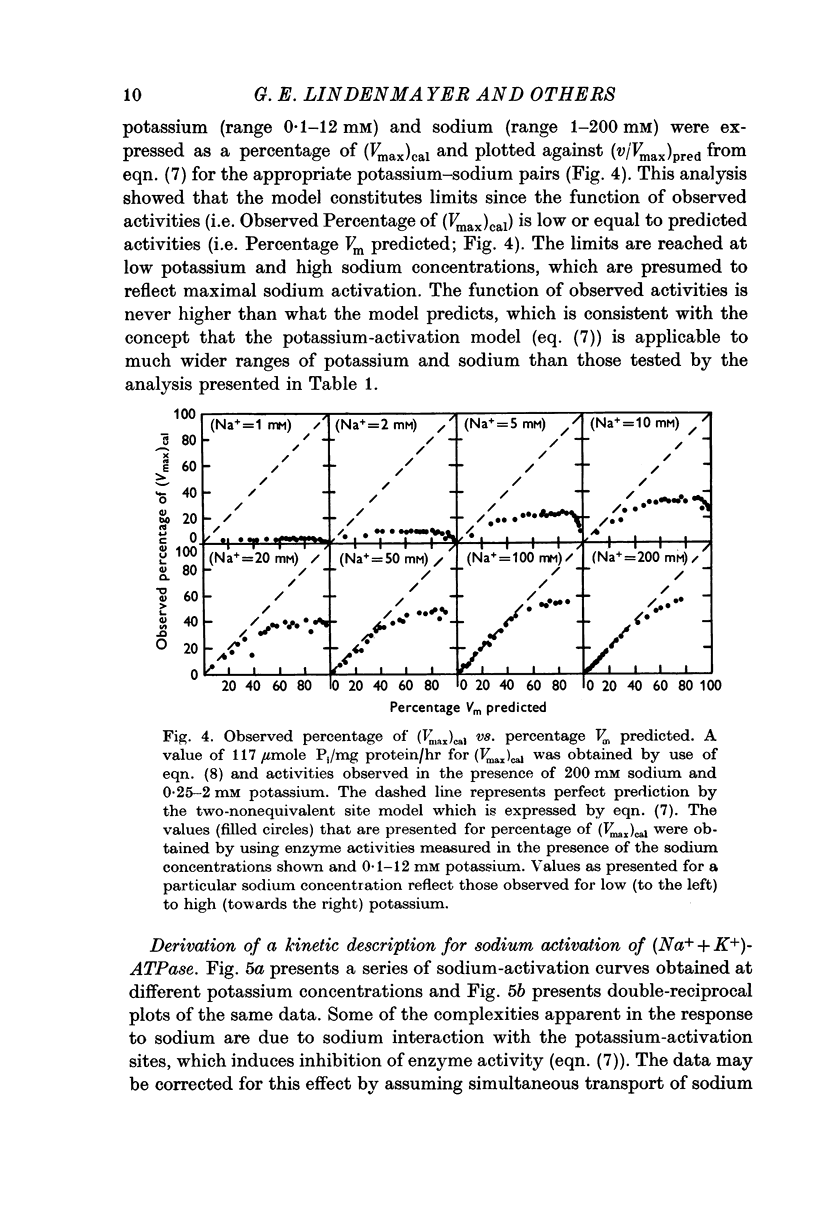
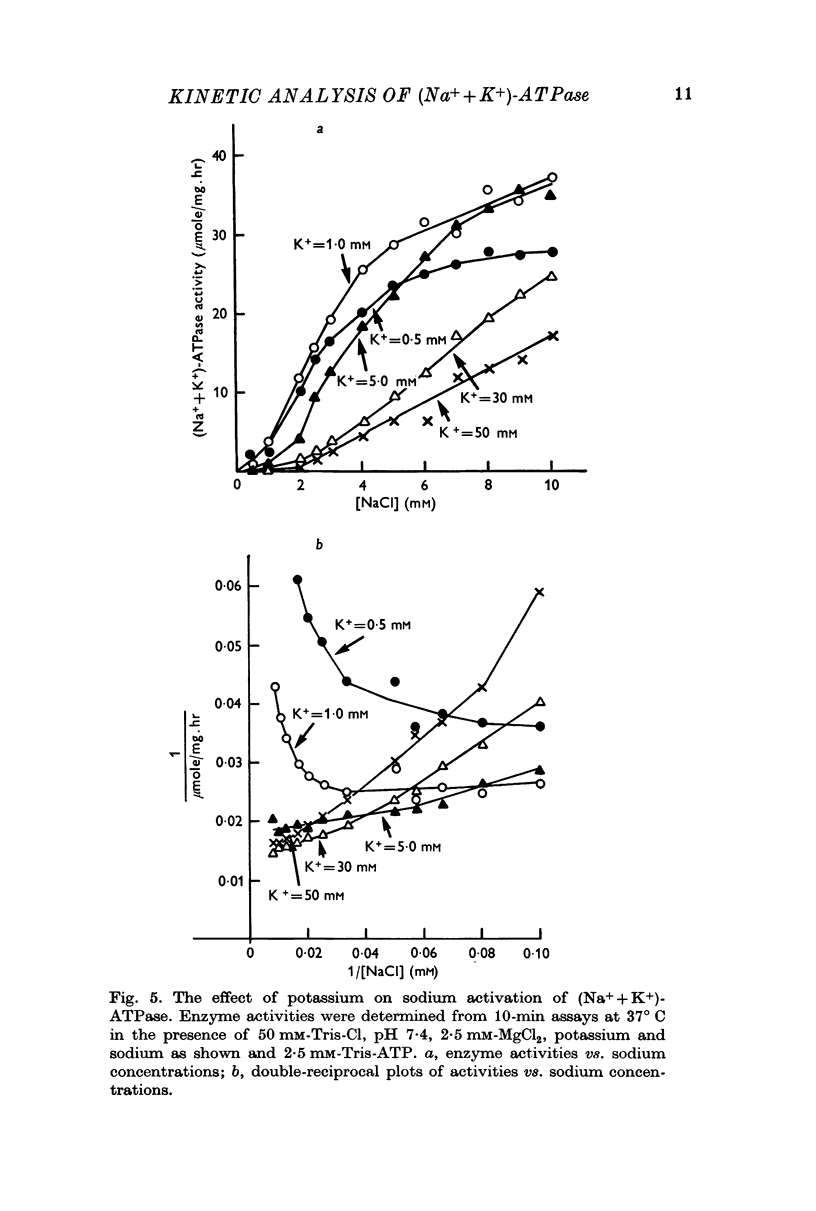
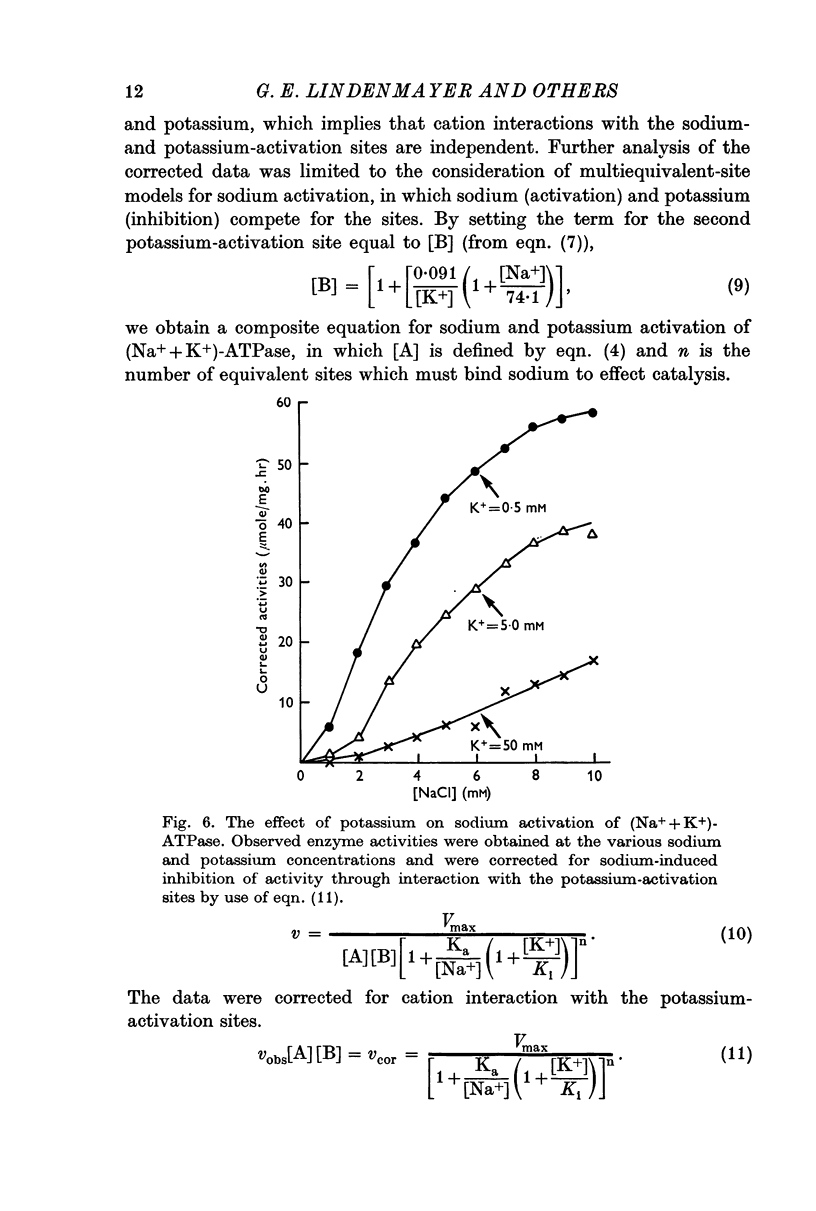
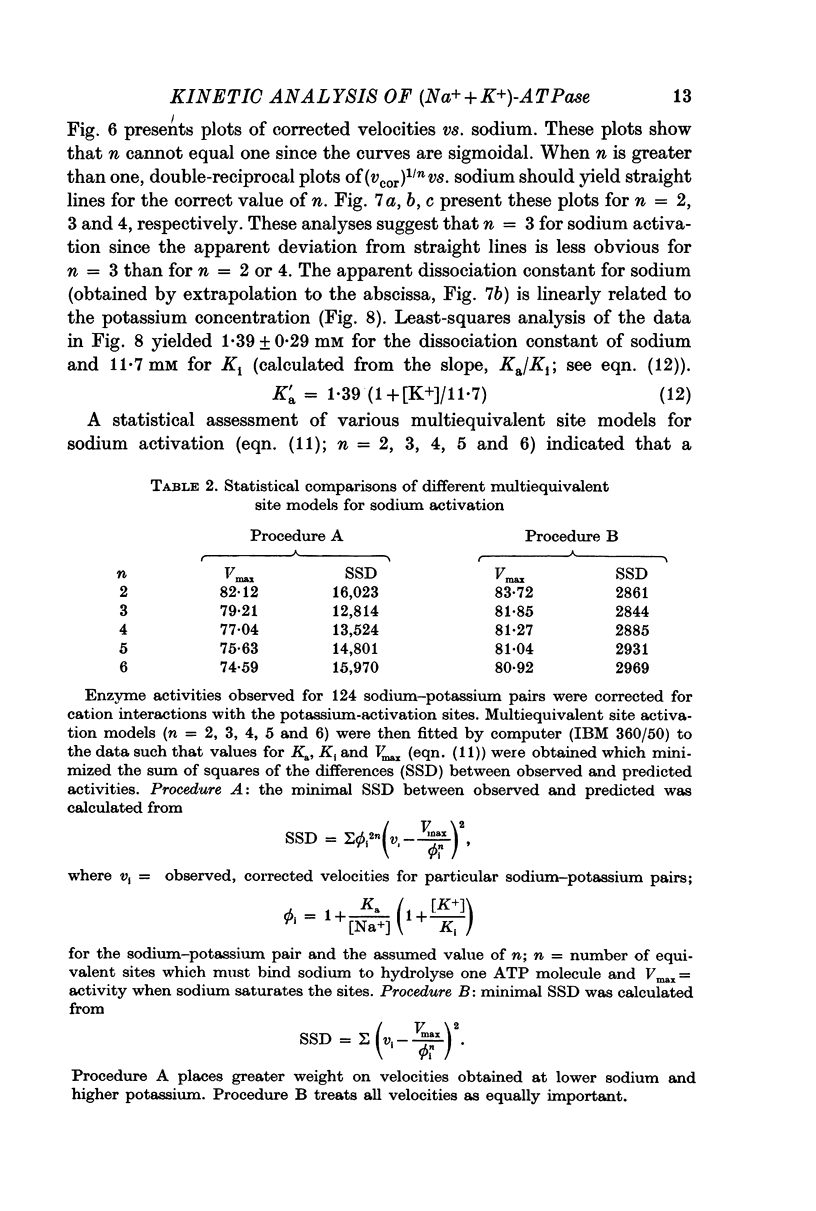
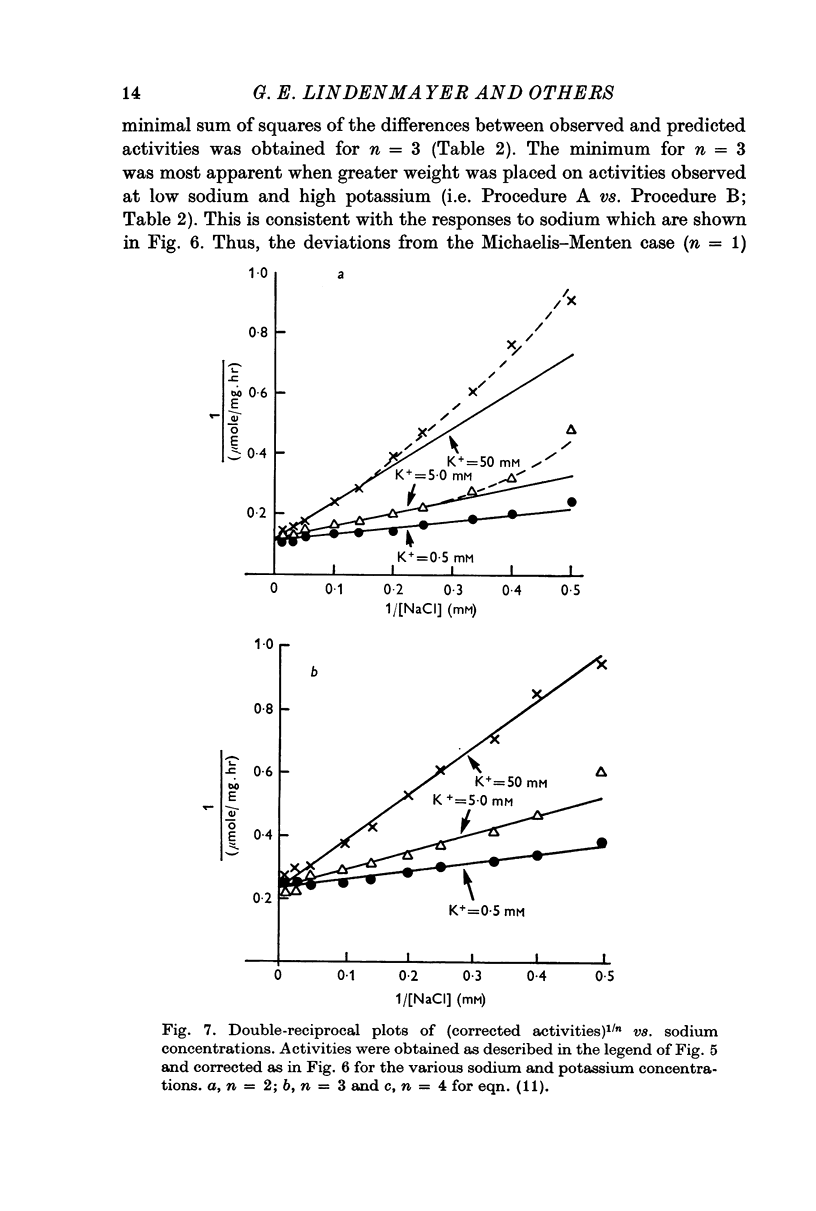
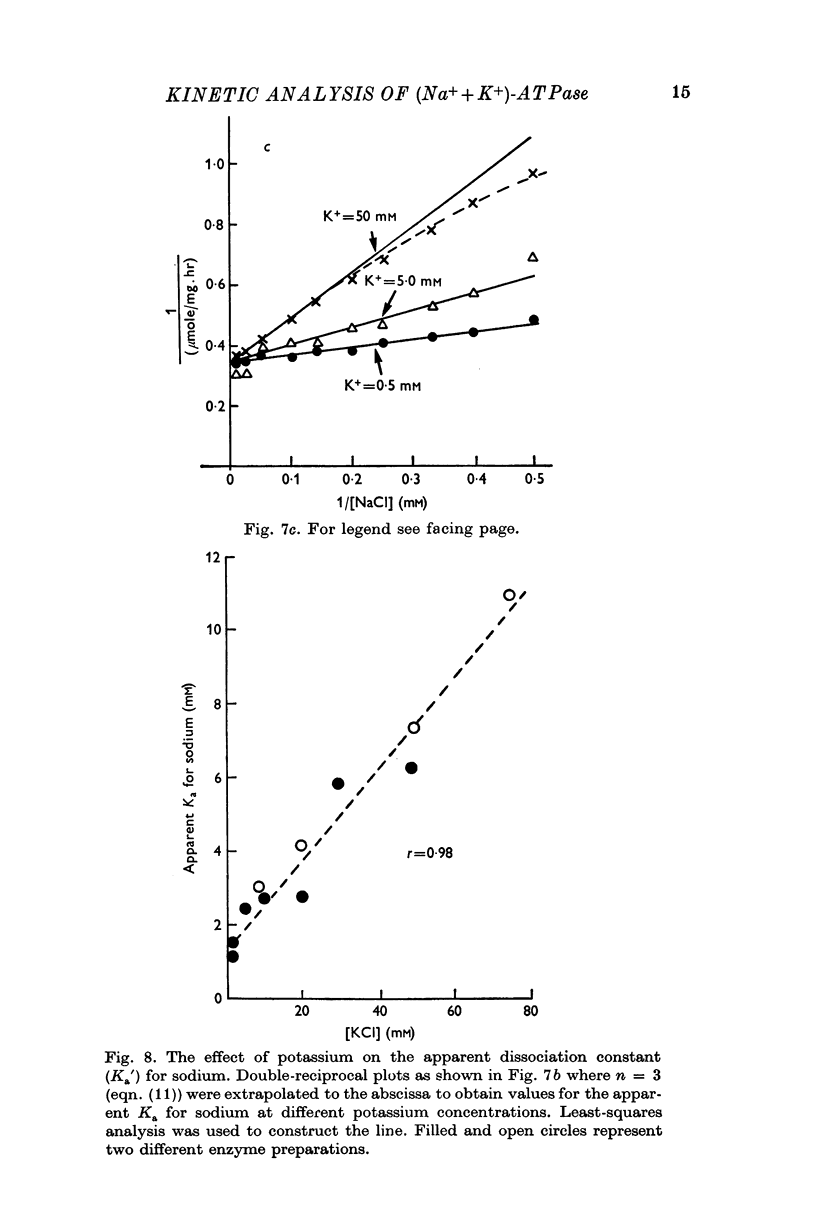
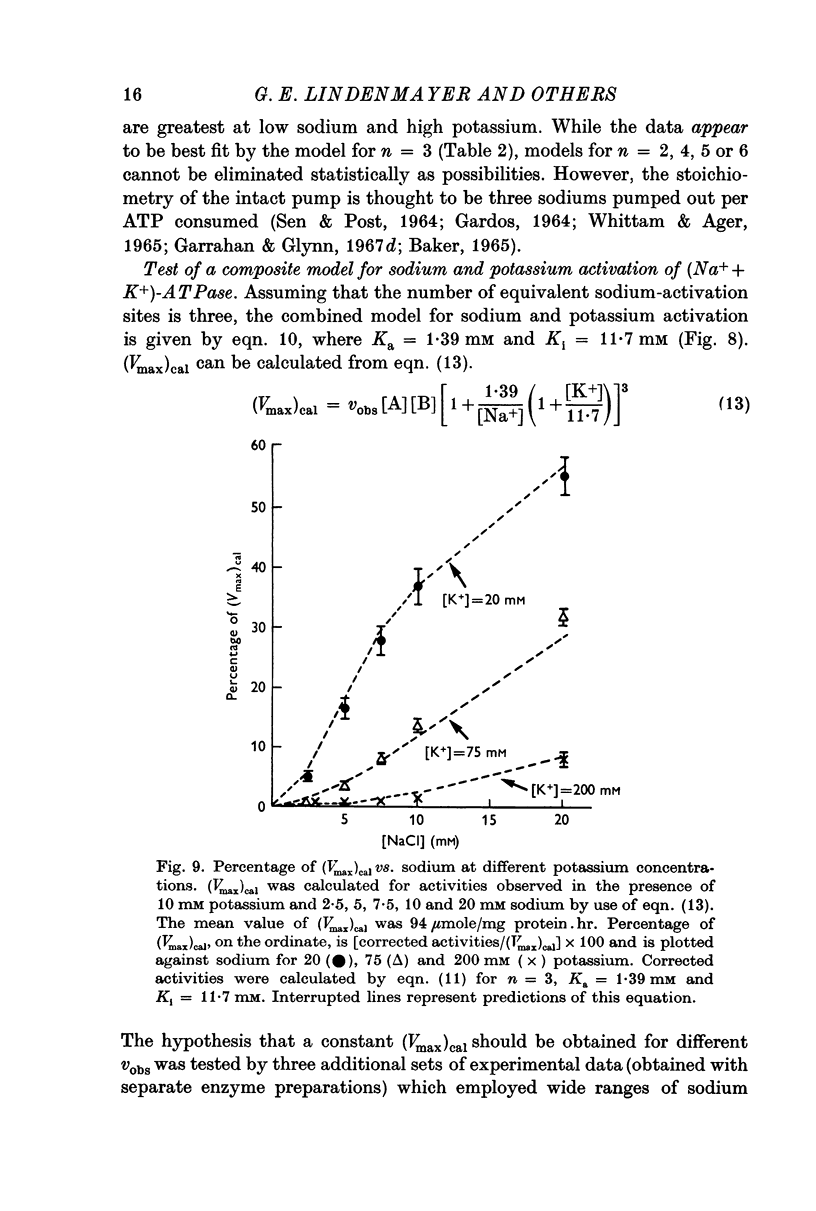
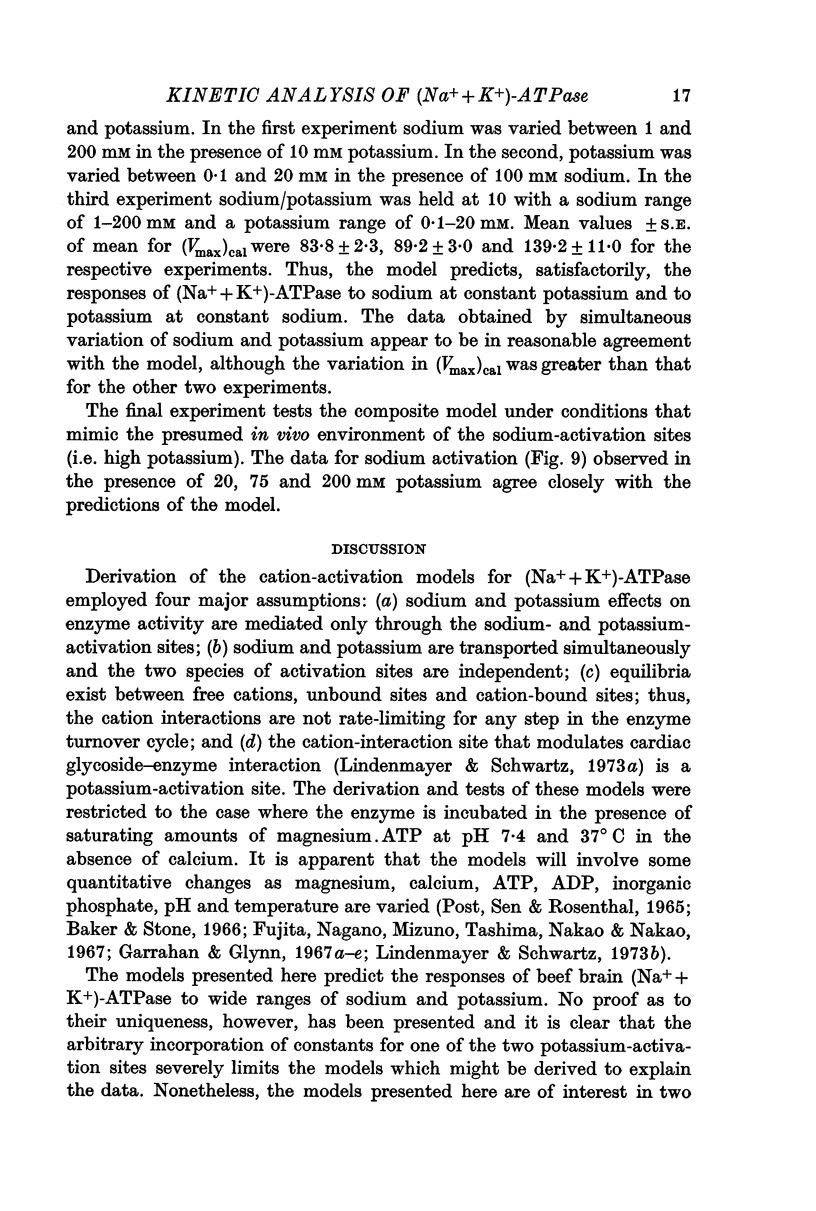
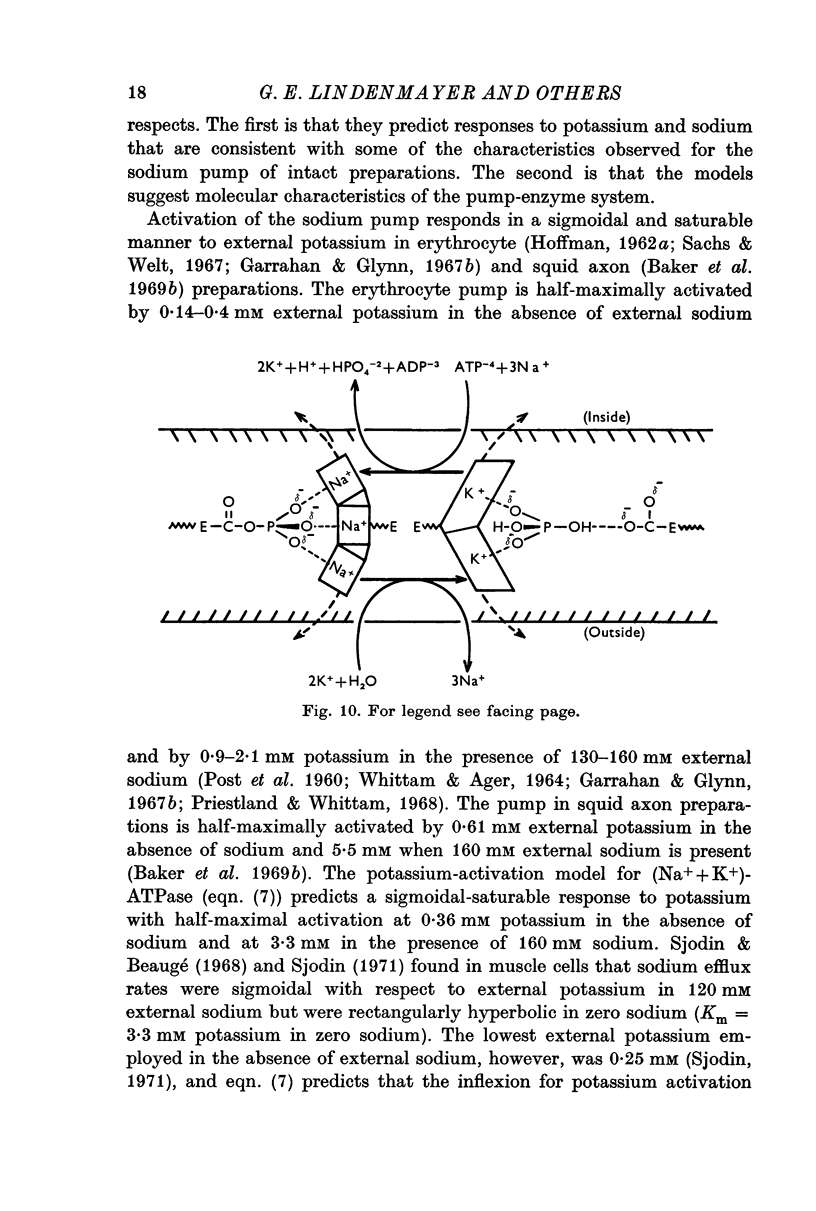
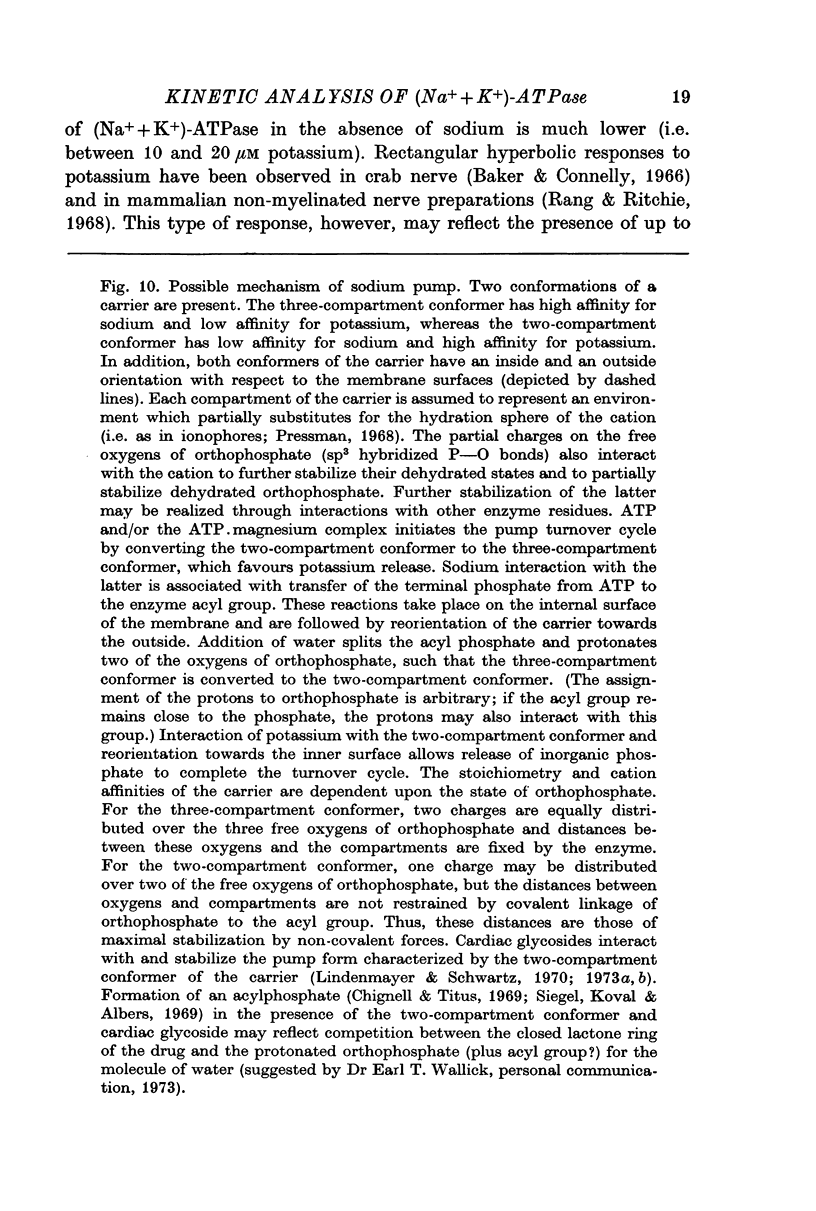
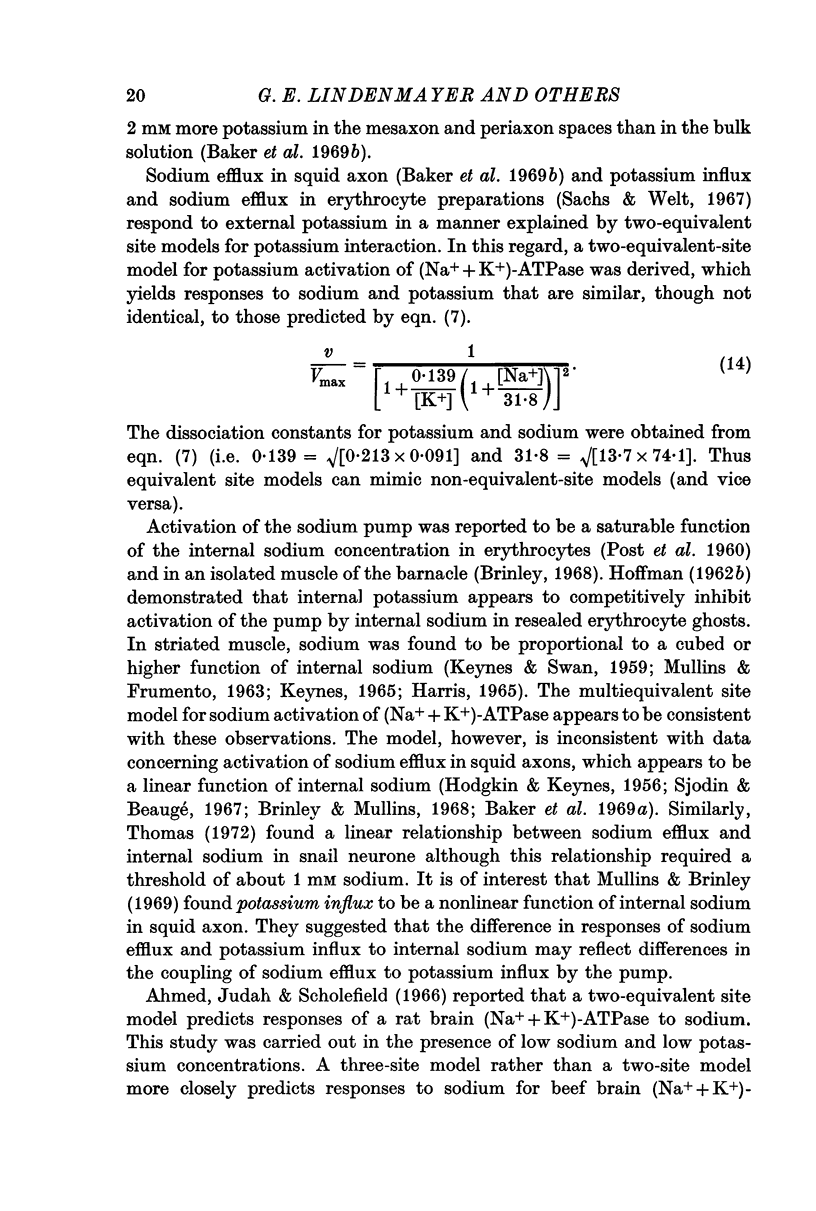
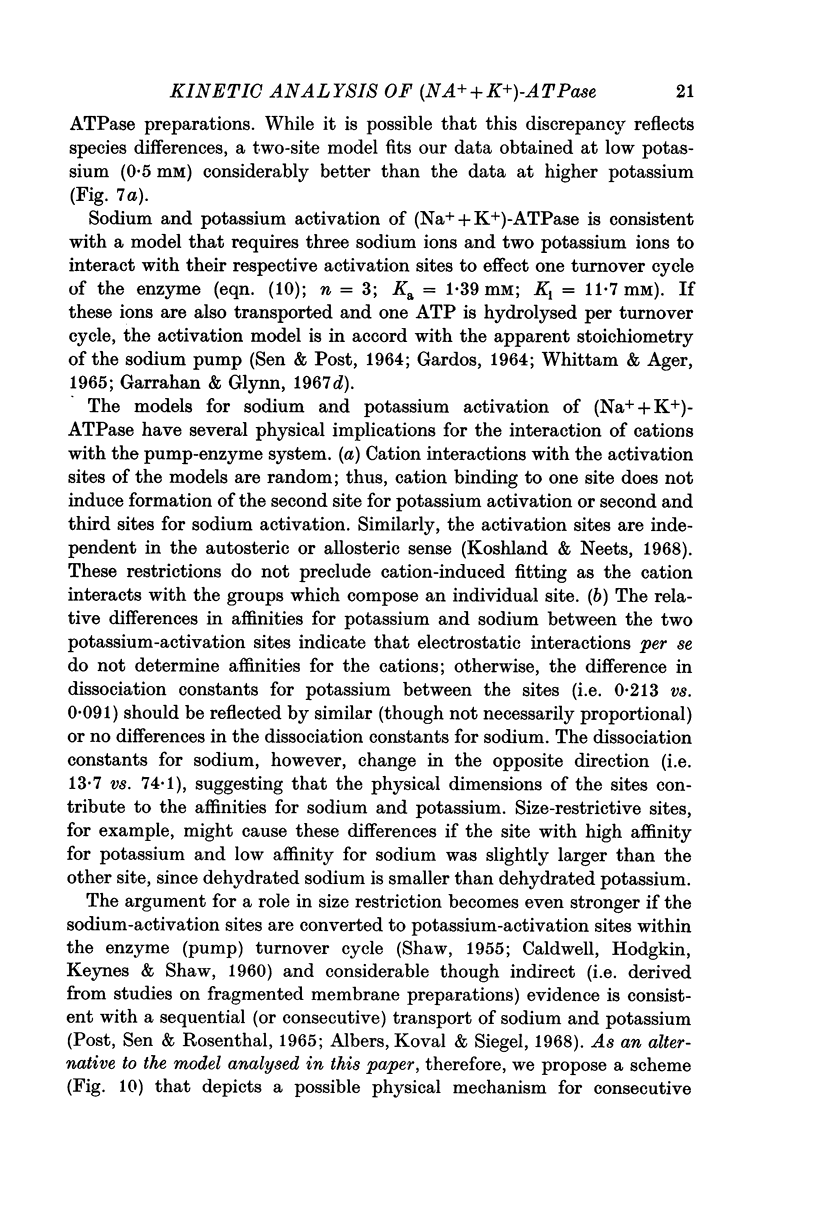
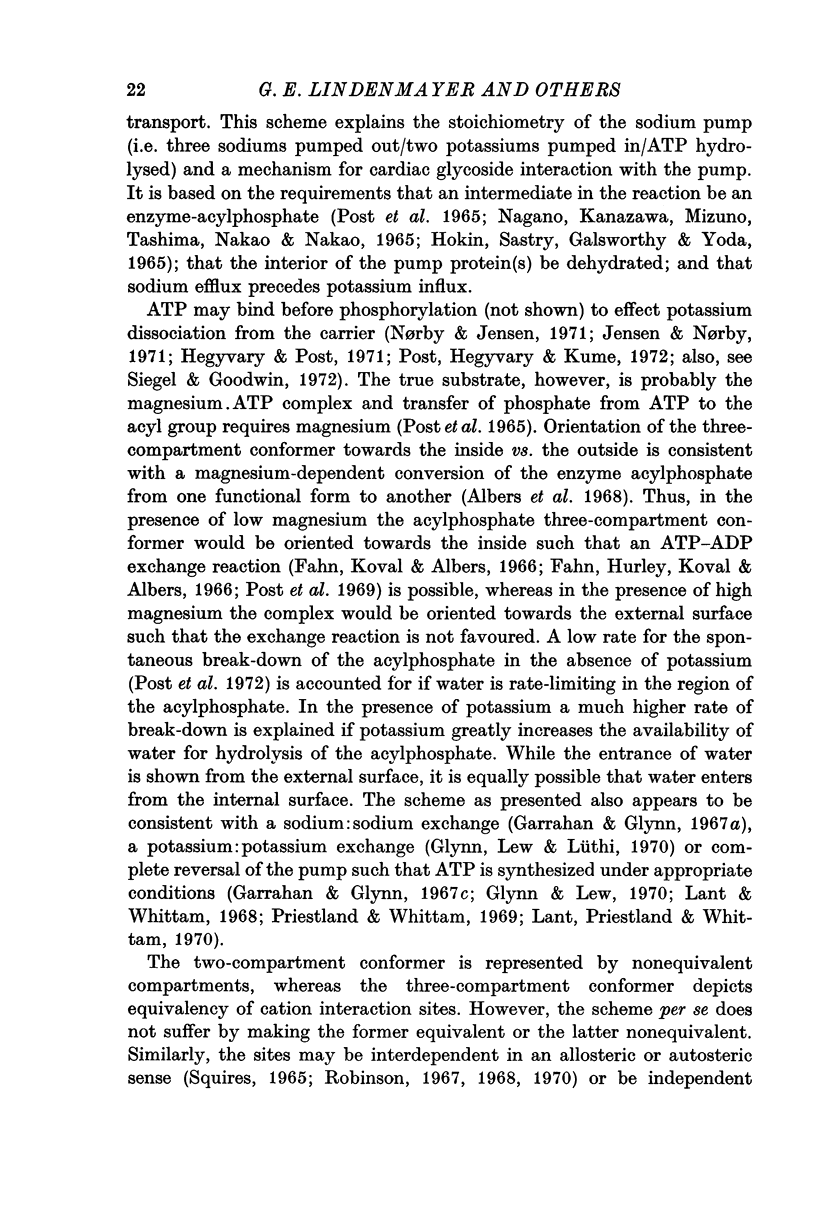
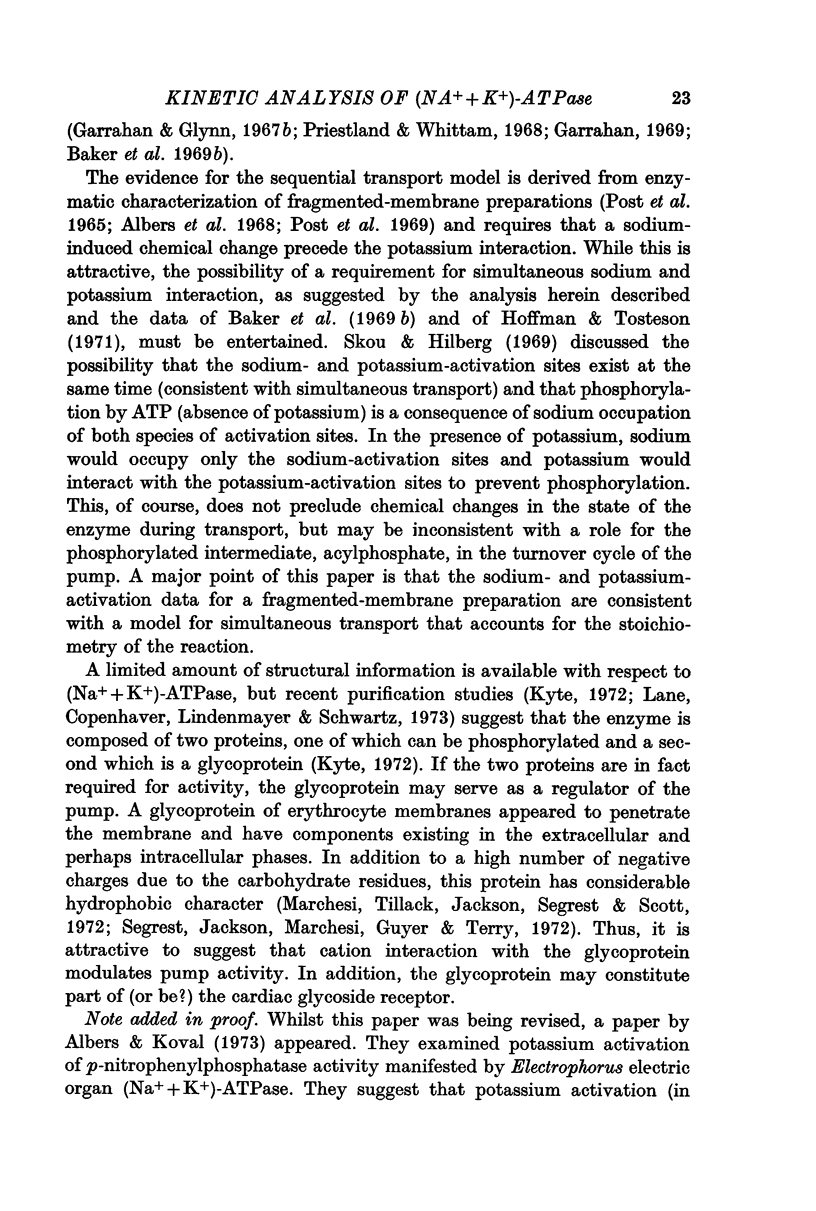
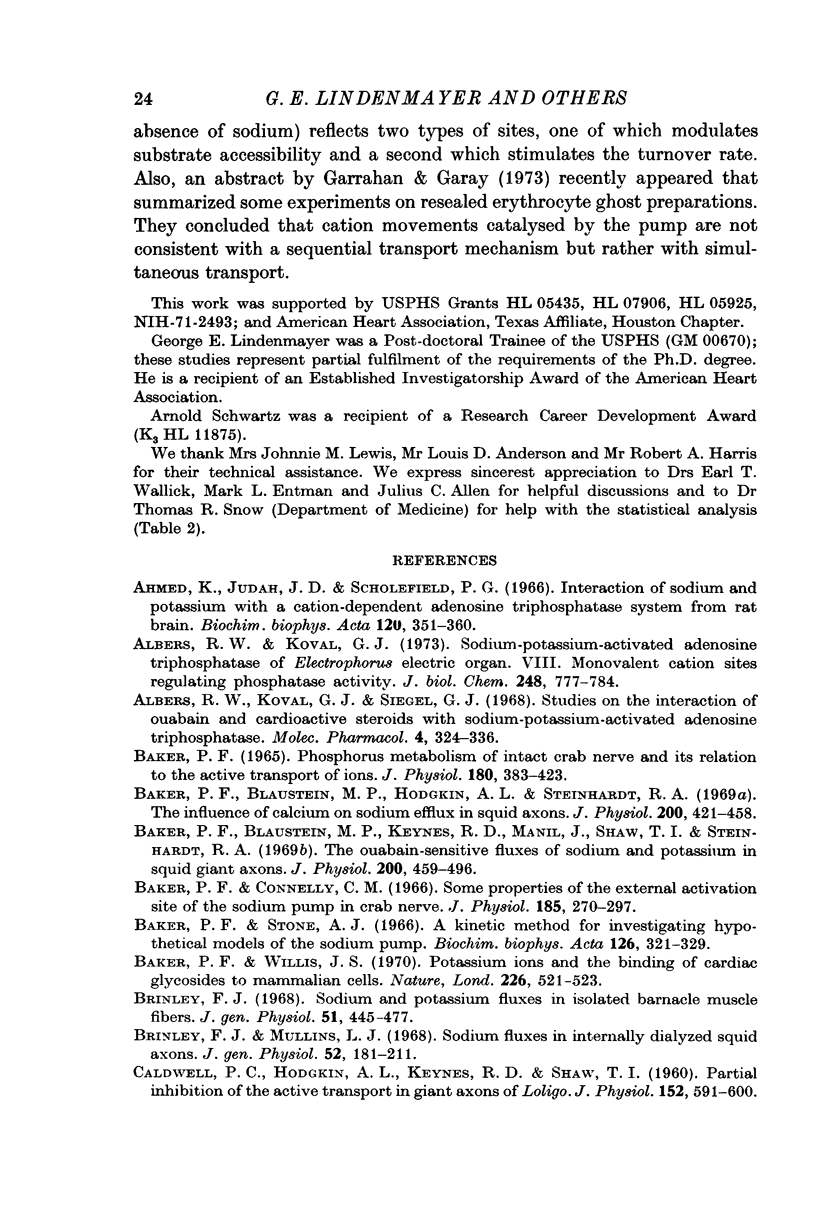
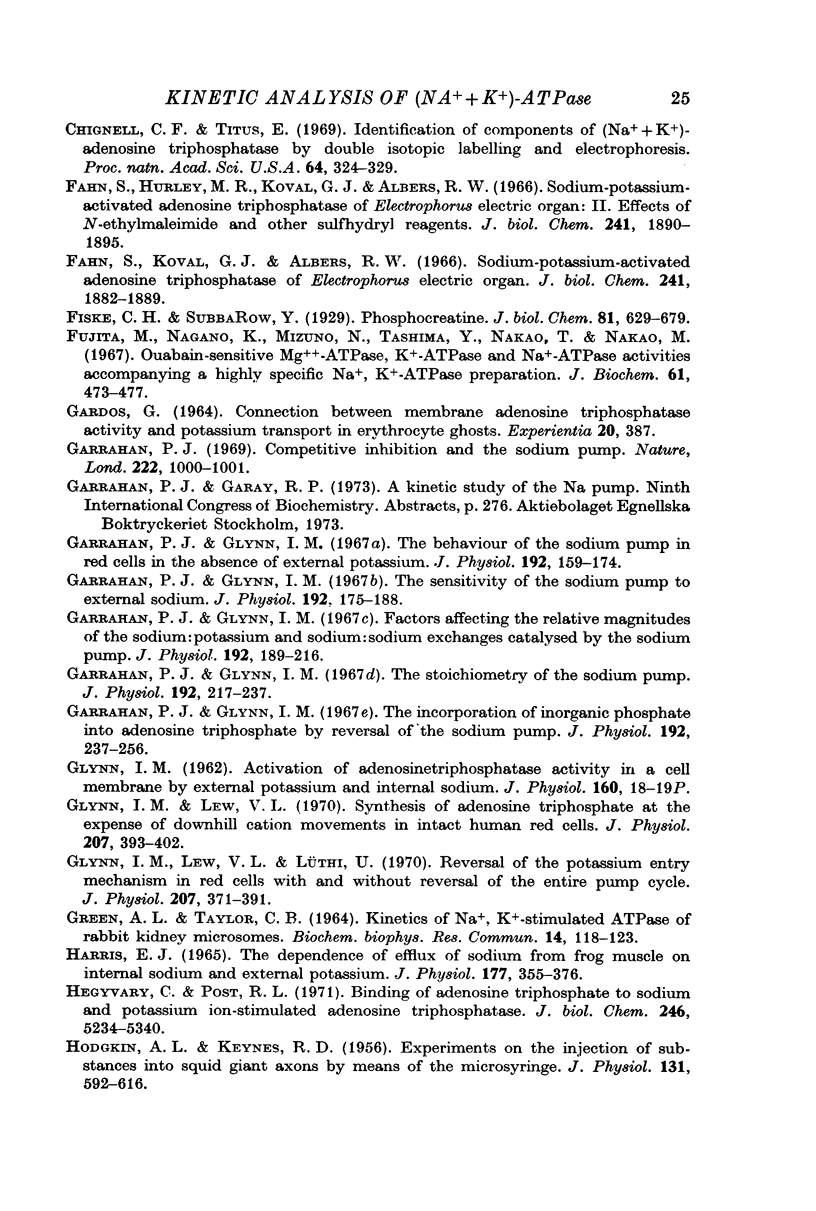
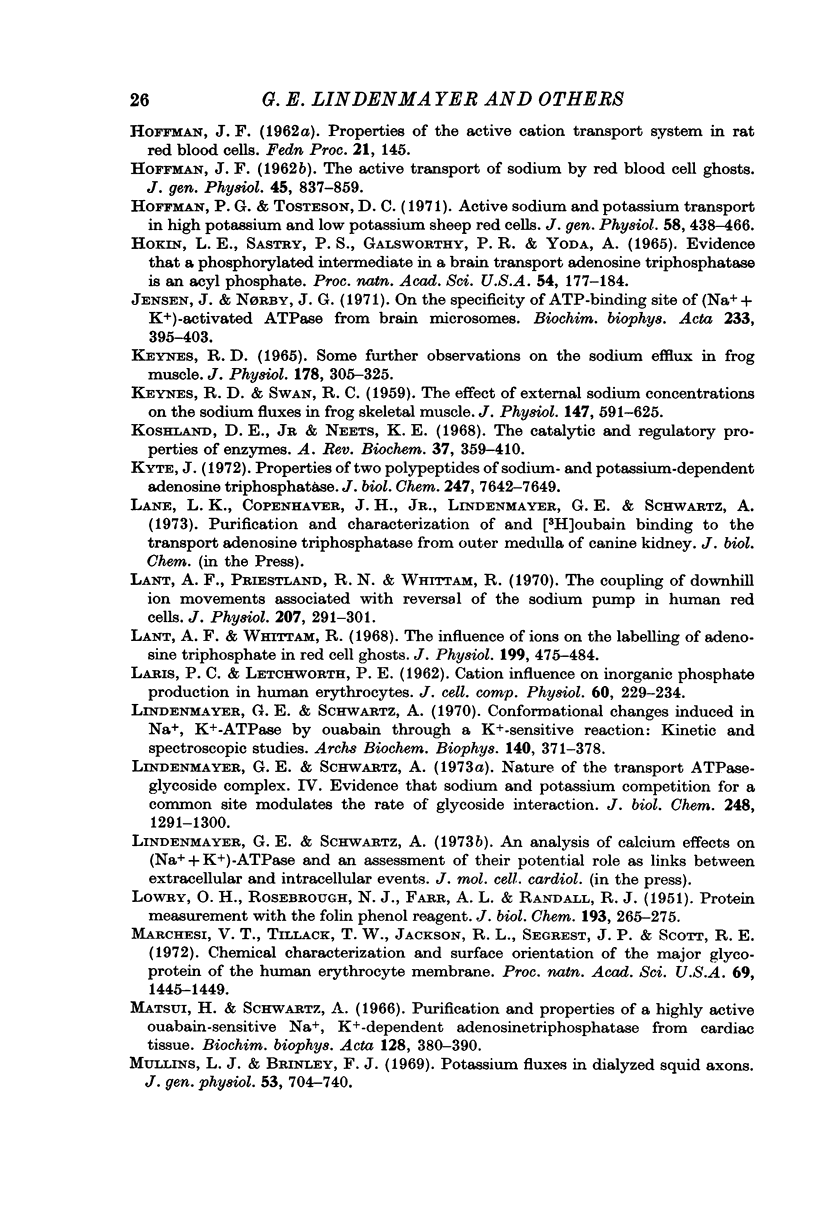
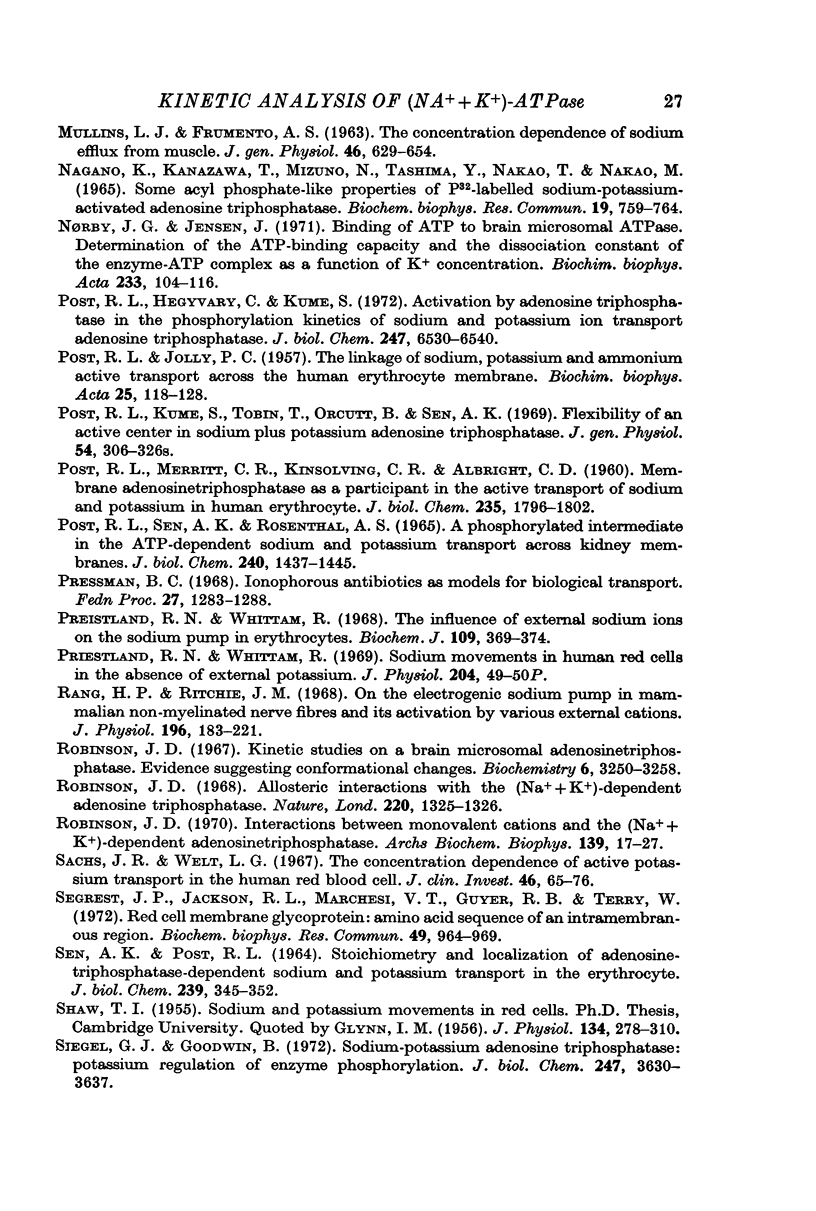
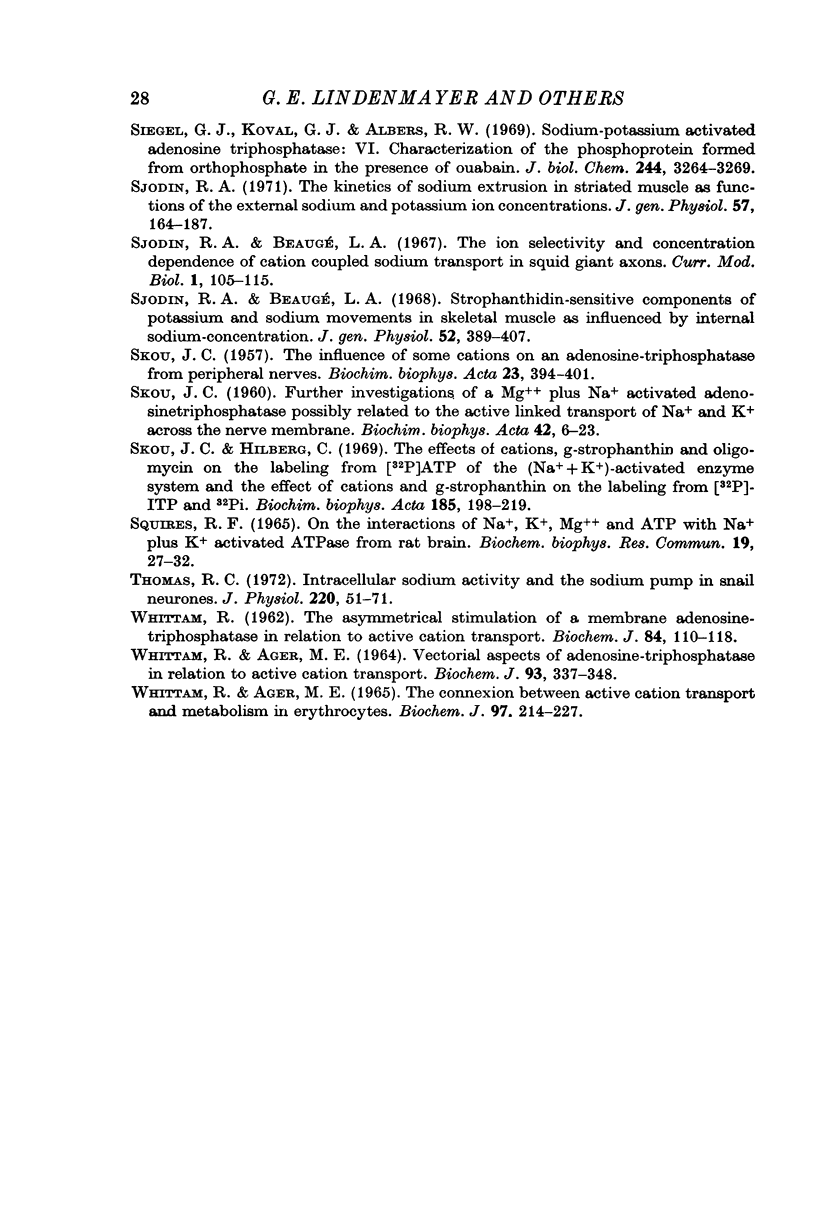
Selected References
These references are in PubMed. This may not be the complete list of references from this article.
- Ahmed K., Judah J. D., Scholefield P. G. Interaction of sodium and potassium with a cation-dependent adenosine triphosphatase system from rat brain. Biochim Biophys Acta. 1966 Jul 13;120(3):351–360. doi: 10.1016/0926-6585(66)90302-5. [DOI] [PubMed] [Google Scholar]
- Albers R. W., Koval G. J., Siegel Studies on the interaction of ouabain and other cardio-active steroids with sodium-potassium-activated adenosine triphosphatase. Mol Pharmacol. 1968 Jul;4(4):324–336. [PubMed] [Google Scholar]
- Albers R. W., Koval G. J. Sodium-potassium-activated adenosine triphosphatase of Electrophorus electric organ. 8. Monovalent cation sites regulating phosphatase activity. J Biol Chem. 1973 Feb 10;248(3):777–784. [PubMed] [Google Scholar]
- Baker P. F., Blaustein M. P., Hodgkin A. L., Steinhardt R. A. The influence of calcium on sodium efflux in squid axons. J Physiol. 1969 Feb;200(2):431–458. doi: 10.1113/jphysiol.1969.sp008702. [DOI] [PMC free article] [PubMed] [Google Scholar]
- Baker P. F., Blaustein M. P., Keynes R. D., Manil J., Shaw T. I., Steinhardt R. A. The ouabain-sensitive fluxes of sodium and potassium in squid giant axons. J Physiol. 1969 Feb;200(2):459–496. doi: 10.1113/jphysiol.1969.sp008703. [DOI] [PMC free article] [PubMed] [Google Scholar]
- Baker P. F., Connelly C. M. Some properties of the external activation site of the sodium pump in crab nerve. J Physiol. 1966 Jul;185(2):270–297. doi: 10.1113/jphysiol.1966.sp007987. [DOI] [PMC free article] [PubMed] [Google Scholar]
- Baker P. F. Phosphorus metabolism of intact crab nerve and its relation to the active transport of ions. J Physiol. 1965 Sep;180(2):383–423. doi: 10.1113/jphysiol.1965.sp007709. [DOI] [PMC free article] [PubMed] [Google Scholar]
- Baker P. F., Stone A. J. A kinetic method for investigating hypothetical models of the sodium pump. Biochim Biophys Acta. 1966 Oct 10;126(2):321–329. doi: 10.1016/0926-6585(66)90069-0. [DOI] [PubMed] [Google Scholar]
- Baker P. F., Willis J. S. Potassium ions and the binding of cardiac glycosides to mammalian cells. Nature. 1970 May 9;226(5245):521–523. doi: 10.1038/226521a0. [DOI] [PubMed] [Google Scholar]
- Brinley F. J., Jr, Mullins L. J. Sodium fluxes in internally dialyzed squid axons. J Gen Physiol. 1968 Aug;52(2):181–211. doi: 10.1085/jgp.52.2.181. [DOI] [PMC free article] [PubMed] [Google Scholar]
- Brinley F. J., Jr Sodium and potassium fluxes in isolated barnacle muscle fibers. J Gen Physiol. 1968 Apr;51(4):445–477. doi: 10.1085/jgp.51.4.445. [DOI] [PMC free article] [PubMed] [Google Scholar]
- CALDWELL P. C., HODGKIN A. L., KEYNES R. D., SHAW T. I. Partial inhibition of the active transport of cations in the giant axons of Loligo. J Physiol. 1960 Jul;152:591–600. doi: 10.1113/jphysiol.1960.sp006510. [DOI] [PMC free article] [PubMed] [Google Scholar]
- Chignell C. F., Titus E. Identification of components of (Na+ plus K+)-adenosine triphosphatase by double isotopic labeling and electrophoresis. Proc Natl Acad Sci U S A. 1969 Sep;64(1):324–329. doi: 10.1073/pnas.64.1.324. [DOI] [PMC free article] [PubMed] [Google Scholar]
- Fahn S., Hurley M. R., Koval G. J., Albers R. W. Sodium-potassium-activated adenosine triphosphatase of Electrophorus electric organ. II. Effects of N-ethylmaleimide and other sulfhydryl reagents. J Biol Chem. 1966 Apr 25;241(8):1890–1895. [PubMed] [Google Scholar]
- Fahn S., Koval G. J., Albers R. W. Sodium-potassium-activated adenosine triphosphatase of Electrophorus electric organ. I. An associated sodium-activated transphosphorylation. J Biol Chem. 1966 Apr 25;241(8):1882–1889. [PubMed] [Google Scholar]
- Fujita M., Nagano K., Mizuno N., Tashima Y., Nakao T. Ouabain-sensitive Mg++-ATPase, K+-ATPase and Na+-ATPase activities accompanying a highly specific Na+-K+-ATPase preparation. J Biochem. 1967 Apr;61(4):473–477. doi: 10.1093/oxfordjournals.jbchem.a128570. [DOI] [PubMed] [Google Scholar]
- GLYNN I. M. Sodium and potassium movements in human red cells. J Physiol. 1956 Nov 28;134(2):278–310. doi: 10.1113/jphysiol.1956.sp005643. [DOI] [PMC free article] [PubMed] [Google Scholar]
- Garrahan P. J. Competitive inhibition and the sodium pump. Nature. 1969 Jun 7;222(5197):1000–1001. doi: 10.1038/2221000a0. [DOI] [PubMed] [Google Scholar]
- Garrahan P. J., Glynn I. M. Facftors affecting the relative magnitudes of the sodium:potassium and sodium:sodium exchanges catalysed by the sodium pump. J Physiol. 1967 Sep;192(1):189–216. doi: 10.1113/jphysiol.1967.sp008296. [DOI] [PMC free article] [PubMed] [Google Scholar]
- Garrahan P. J., Glynn I. M. The behaviour of the sodium pump in red cells in the absence of external potassium. J Physiol. 1967 Sep;192(1):159–174. doi: 10.1113/jphysiol.1967.sp008294. [DOI] [PMC free article] [PubMed] [Google Scholar]
- Garrahan P. J., Glynn I. M. The incorporation of inorganic phosphate into adenosine triphosphate by reversal of the sodium pump. J Physiol. 1967 Sep;192(1):237–256. doi: 10.1113/jphysiol.1967.sp008298. [DOI] [PMC free article] [PubMed] [Google Scholar]
- Garrahan P. J., Glynn I. M. The sensitivity of the sodium pump to external sodium. J Physiol. 1967 Sep;192(1):175–188. doi: 10.1113/jphysiol.1967.sp008295. [DOI] [PMC free article] [PubMed] [Google Scholar]
- Glynn I. M., Lew V. L., Lüthi U. Reversal of the potassium entry mechanism in red cells, with and without reversal of the entire pump cycle. J Physiol. 1970 Apr;207(2):371–391. doi: 10.1113/jphysiol.1970.sp009067. [DOI] [PMC free article] [PubMed] [Google Scholar]
- Glynn I. M., Lew V. L. Synthesis of adenosine triphosphate at the expense of downhill cation movements in intact human red cells. J Physiol. 1970 Apr;207(2):393–402. doi: 10.1113/jphysiol.1970.sp009068. [DOI] [PMC free article] [PubMed] [Google Scholar]
- Green A. L., Taylor C. B. Kinetics of (Na+ + K+)-stimulated ATPase of rabbit kidney microsomes. Biochem Biophys Res Commun. 1964;14:118–123. doi: 10.1016/0006-291x(64)90240-2. [DOI] [PubMed] [Google Scholar]
- Gárdos G. Connection between membrane adenosine triphosphatase activity and potassium transport in erythrocyte ghosts. Experientia. 1964 Jul 15;20(7):387–387. doi: 10.1007/BF02147979. [DOI] [PubMed] [Google Scholar]
- HARRIS E. J. THE DEPENDENCE OF EFFLUX OF SODIUM FROM FROG MUSCLE ON INTERNAL SODIUM AND EXTERNAL POTASSIUM. J Physiol. 1965 Apr;177:355–376. doi: 10.1113/jphysiol.1965.sp007597. [DOI] [PMC free article] [PubMed] [Google Scholar]
- HODGKIN A. L., KEYNES R. D. Experiments on the injection of substances into squid giant axons by means of a microsyringe. J Physiol. 1956 Mar 28;131(3):592–616. doi: 10.1113/jphysiol.1956.sp005485. [DOI] [PMC free article] [PubMed] [Google Scholar]
- HOFFMAN J. F. The active transport of sodium by ghosts of human red blood cells. J Gen Physiol. 1962 May;45:837–859. doi: 10.1085/jgp.45.5.837. [DOI] [PMC free article] [PubMed] [Google Scholar]
- Hegyvary C., Post R. L. Binding of adenosine triphosphate to sodium and potassium ion-stimulated adenosine triphosphatase. J Biol Chem. 1971 Sep 10;246(17):5234–5240. [PubMed] [Google Scholar]
- Hoffman P. G., Tosteson D. C. Active sodium and potassium transport in high potassium and low potassium sheep red cells. J Gen Physiol. 1971 Oct;58(4):438–466. doi: 10.1085/jgp.58.4.438. [DOI] [PMC free article] [PubMed] [Google Scholar]
- Hokin L. E., Sastry P. S., Galsworthy P. R., Yoda A. Evidence that a phosphorylated intermediate in a brain transport adenosine triphosphatase is an acyl phosphate. Proc Natl Acad Sci U S A. 1965 Jul;54(1):177–184. doi: 10.1073/pnas.54.1.177. [DOI] [PMC free article] [PubMed] [Google Scholar]
- Jensen J., Norby J. G. On the specificity of the ATP-binding site of (Na+ + K+)-activated ATPase from brain microsomes. Biochim Biophys Acta. 1971 Apr 13;233(2):395–403. doi: 10.1016/0005-2736(71)90336-1. [DOI] [PubMed] [Google Scholar]
- KEYNES R. D. SOME FURTHER OBSERVATIONS ON THE SODIUM EFFLUX IN FROG MUSCLE. J Physiol. 1965 May;178:305–325. doi: 10.1113/jphysiol.1965.sp007629. [DOI] [PMC free article] [PubMed] [Google Scholar]
- KEYNES R. D., SWAN R. C. The effect of external sodium concentration on the sodium fluxes in frog skeletal muscle. J Physiol. 1959 Oct;147:591–625. doi: 10.1113/jphysiol.1959.sp006264. [DOI] [PMC free article] [PubMed] [Google Scholar]
- Kyte J. Properties of the two polypeptides of sodium- and potassium-dependent adenosine triphosphatase. J Biol Chem. 1972 Dec 10;247(23):7642–7649. [PubMed] [Google Scholar]
- LOWRY O. H., ROSEBROUGH N. J., FARR A. L., RANDALL R. J. Protein measurement with the Folin phenol reagent. J Biol Chem. 1951 Nov;193(1):265–275. [PubMed] [Google Scholar]
- Lant A. F., Priestland R. N., Whittam R. The coupling of downhill ion movements associated with reversal of the sodium pump in human red cells. J Physiol. 1970 Apr;207(2):291–301. doi: 10.1113/jphysiol.1970.sp009062. [DOI] [PMC free article] [PubMed] [Google Scholar]
- Lant A. F., Whittam R. The influence of ions on the labelling of adenosine triphosphate in red cell ghosts. J Physiol. 1968 Dec;199(2):457–484. doi: 10.1113/jphysiol.1968.sp008663. [DOI] [PMC free article] [PubMed] [Google Scholar]
- Lindenmayer G. E., Schwartz A. Conformational changes induced in Na+, K+-ATPase by ouabain through a K+-sensitive reaction: kinetic and spectroscopic studies. Arch Biochem Biophys. 1970 Oct;140(2):371–378. doi: 10.1016/0003-9861(70)90078-0. [DOI] [PubMed] [Google Scholar]
- Lindenmayer G. E., Schwartz A. Nature of the transport adenosine triphosphatase digitalis complex. IV. Evidence that sodium-potassium competition modulates the rate of ouabain interaction iwth (Na + +K + ) adenosine triphosphatase during enzyme catalysis. J Biol Chem. 1973 Feb 25;248(4):1291–1300. [PubMed] [Google Scholar]
- MULLINS L. J., FRUMENTO A. S. The concentration dependence of sodium efflux from muscle. J Gen Physiol. 1963 Mar;46:629–654. doi: 10.1085/jgp.46.4.629. [DOI] [PMC free article] [PubMed] [Google Scholar]
- Marchesi V. T., Tillack T. W., Jackson R. L., Segrest J. P., Scott R. E. Chemical characterization and surface orientation of the major glycoprotein of the human erythrocyte membrane. Proc Natl Acad Sci U S A. 1972 Jun;69(6):1445–1449. doi: 10.1073/pnas.69.6.1445. [DOI] [PMC free article] [PubMed] [Google Scholar]
- Matsui H., Schwartz A. Purification and properties of a highly active ouabain-sensitive Na+, K+-dependent adenosinetriphosphatase from cardiac tissue. Biochim Biophys Acta. 1966 Nov 15;128(2):380–390. doi: 10.1016/0926-6593(66)90185-8. [DOI] [PubMed] [Google Scholar]
- Mullins L. J., Brinley F. J., Jr Potassium fluxes in dialyzed squid axons. J Gen Physiol. 1969 Jun;53(6):704–740. doi: 10.1085/jgp.53.6.704. [DOI] [PMC free article] [PubMed] [Google Scholar]
- Nagano K., Kanazawa T., Mizuno N., Tashima Y., Nakao T., Nakao M. Some acyl phosphate-like properties of P32-labeled sodium-potassium-activated adenosine triphosphatase. Biochem Biophys Res Commun. 1965 Jun 9;19(6):759–764. doi: 10.1016/0006-291x(65)90324-4. [DOI] [PubMed] [Google Scholar]
- Norby J. G., Jensen J. Binding of ATP to brain microsomal ATPase. Determination of the ATP-binding capacity and the dissociation constant of the enzyme-ATP complex as a function of K+ concentration. Biochim Biophys Acta. 1971 Mar 9;233(1):104–116. doi: 10.1016/0005-2736(71)90362-2. [DOI] [PubMed] [Google Scholar]
- POST R. L., JOLLY P. C. The linkage of sodium, potassium, and ammonium active transport across the human erythrocyte membrane. Biochim Biophys Acta. 1957 Jul;25(1):118–128. doi: 10.1016/0006-3002(57)90426-2. [DOI] [PubMed] [Google Scholar]
- POST R. L., MERRITT C. R., KINSOLVING C. R., ALBRIGHT C. D. Membrane adenosine triphosphatase as a participant in the active transport of sodium and potassium in the human erythrocyte. J Biol Chem. 1960 Jun;235:1796–1802. [PubMed] [Google Scholar]
- POST R. L., SEN A. K., ROSENTHAL A. S. A PHOSPHORYLATED INTERMEDIATE IN ADENOSINE TRIPHOSPHATE-DEPENDENT SODIUM AND POTASSIUM TRANSPORT ACROSS KIDNEY MEMBRANES. J Biol Chem. 1965 Mar;240:1437–1445. [PubMed] [Google Scholar]
- Post R. L., Hegyvary C., Kume S. Activation by adenosine triphosphate in the phosphorylation kinetics of sodium and potassium ion transport adenosine triphosphatase. J Biol Chem. 1972 Oct 25;247(20):6530–6540. [PubMed] [Google Scholar]
- Post R. L., Kume S., Tobin T., Orcutt B., Sen A. K. Flexibility of an active center in sodium-plus-potassium adenosine triphosphatase. J Gen Physiol. 1969 Jul 1;54(1):306–326. doi: 10.1085/jgp.54.1.306. [DOI] [PMC free article] [PubMed] [Google Scholar]
- Pressman B. C. Ionophorous antibiotics as models for biological transport. Fed Proc. 1968 Nov-Dec;27(6):1283–1288. [PubMed] [Google Scholar]
- Priestland R. N., Whittam R. Sodium movements in human red cells in the absence of external potassium. J Physiol. 1969 Sep;204(1):49P–50P. [PubMed] [Google Scholar]
- Rang H. P., Ritchie J. M. On the electrogenic sodium pump in mammalian non-myelinated nerve fibres and its activation by various external cations. J Physiol. 1968 May;196(1):183–221. doi: 10.1113/jphysiol.1968.sp008502. [DOI] [PMC free article] [PubMed] [Google Scholar]
- Robinson J. D. Allosteric interactions with the (Na+ + K+)-dependent adenosine triphosphatase. Nature. 1968 Dec 28;220(5174):1325–1326. doi: 10.1038/2201325a0. [DOI] [PubMed] [Google Scholar]
- Robinson J. D. Interactions between monovalent cations and the (Na+ + K+)-dependent adenosine triphosphatase. Arch Biochem Biophys. 1970 Jul;139(1):17–27. doi: 10.1016/0003-9861(70)90040-8. [DOI] [PubMed] [Google Scholar]
- Robinson J. D. Kinetic studies on a brain microsomal adenosine triphosphatase. Evidence suggesting conformational changes. Biochemistry. 1967 Oct;6(10):3250–3258. doi: 10.1021/bi00862a034. [DOI] [PubMed] [Google Scholar]
- SEN A. K., POST R. L. STOICHIOMETRY AND LOCALIZATION OF ADENOSINE TRIPHOSPHATE-DEPENDENT SODIUM AND POTASSIUM TRANSPORT IN THE ERYTHROCYTE. J Biol Chem. 1964 Jan;239:345–352. [PubMed] [Google Scholar]
- SKOU J. C. The influence of some cations on an adenosine triphosphatase from peripheral nerves. Biochim Biophys Acta. 1957 Feb;23(2):394–401. doi: 10.1016/0006-3002(57)90343-8. [DOI] [PubMed] [Google Scholar]
- Sachs J. R., Welt L. G. The concentration dependence of active potassium transport in the human red blood cell. J Clin Invest. 1967 Jan;46(1):65–76. doi: 10.1172/JCI105512. [DOI] [PMC free article] [PubMed] [Google Scholar]
- Segrest J. P., Jackson R. L., Marchesi V. T., Guyer R. B., Terry W. Red cell membrane glycoprotein: amino acid sequence of an intramembranous region. Biochem Biophys Res Commun. 1972 Nov 15;49(4):964–969. doi: 10.1016/0006-291x(72)90306-3. [DOI] [PubMed] [Google Scholar]
- Siegel G. J., Goodwin B. Sodium-potassium-activated adenosine triphosphatase: potassium regulation of enzyme phosphorylation. Sodium-stimulated, potassium-inhibited uridine triphosphate hydrolysis. J Biol Chem. 1972 Jun 10;247(11):3630–3637. [PubMed] [Google Scholar]
- Siegel G. J., Koval G. J., Albers R. W. Sodium-potassium-activated adenosine triphosphatase. IV. Characterization of the phosphoprotein formed from orthophosphate in the presence of ouabain. J Biol Chem. 1969 Jun 25;244(12):3264–3269. [PubMed] [Google Scholar]
- Sjodin R. A., Beaugé L. A. Strophanthidin-sensitive components of potassium and sodium movements in skeletal muscle as influenced by the internal sodium concentration. J Gen Physiol. 1968 Sep;52(3):389–407. doi: 10.1085/jgp.52.3.389. [DOI] [PMC free article] [PubMed] [Google Scholar]
- Sjodin R. A., Beaugé L. A. The ion selectivity and concentration dependence of cation coupled active sodium transport in squid giant axons. Curr Mod Biol. 1967 May;1(2):105–115. doi: 10.1016/0303-2647(67)90022-6. [DOI] [PubMed] [Google Scholar]
- Sjodin R. A. The kinetics of sodium extrusion in striated muscle as functions of the external sodium and potassium ion concentrations. J Gen Physiol. 1971 Feb;57(2):164–187. doi: 10.1085/jgp.57.2.164. [DOI] [PMC free article] [PubMed] [Google Scholar]
- Skou J. C., Hilberg C. The effect of cations, g-strophanthin and oligomycin on the labeling from [32P] ATP of the (Na+ + K+)-activated enzyme system and the effect of cations and g-strophanthin on the labeling from [32P] ITP and 32Pi. Biochim Biophys Acta. 1969 Jul 8;185(1):198–219. doi: 10.1016/0005-2744(69)90295-2. [DOI] [PubMed] [Google Scholar]
- Thomas R. C. Intracellular sodium activity and the sodium pump in snail neurones. J Physiol. 1972 Jan;220(1):55–71. doi: 10.1113/jphysiol.1972.sp009694. [DOI] [PMC free article] [PubMed] [Google Scholar]
- Whittam R., Ager M. E. The connexion between active cation transport and metabolism in erythrocytes. Biochem J. 1965 Oct;97(1):214–227. doi: 10.1042/bj0970214. [DOI] [PMC free article] [PubMed] [Google Scholar]
- Whittam R., Ager M. E. Vectorial aspects of adenosine-triphosphatase activity in erythrocyte membranes. Biochem J. 1964 Nov;93(2):337–348. doi: 10.1042/bj0930337. [DOI] [PMC free article] [PubMed] [Google Scholar]