Abstract
1. When red cells were so depleted of Na that Na:K exchange had almost ceased, the ouabain-sensitive K efflux seen in K-containing media was accompanied by an almost equal ouabain-sensitive K influx.
2. This suggests that the Na pump in these cells was carrying out a one-for-one K:K exchange across the erythrocyte membrane.
3. 30-40% of the 42K efflux from resealed ghosts was sensitive to ouabain when the ghosts contained 1 mM-ATP, 2 mM orthophosphate, 10 mM-K and less than 1 mM-Na, and the suspending medium contained 10 mM-K and 0-Na, choline being the predominant cation.
4. In resealed ghosts, the rate of K:K exchange saturated as internal K was increased, and was half-maximal at about 10 mM-K.
5. When internal ATP was maintained with a phosphocreatine:creatine phosphokinase regenerating system, K:K exchange saturated as internal ATP was increased, and was half-maximal at about 100 μM-ATP.
6. The rate of K:K exchange did not depend on whether the ADP concentration was roughly the same as the ATP concentration or very much less, suggesting that ADP did not affect the rate of K:K exchange.
7. GTP, ITP and UTP were unable to substitute for ATP in supporting K:K exchange. CTP was a poor substitute.
8. There was no evidence to support the hypothesis that K:K exchange is accompanied by a ouabain-sensitive hydrolysis of ATP.
9. Internal Na was a strong inhibitor of ouabain-sensitive K efflux from ghosts containing 9 mM-K. 4 mM-Na was sufficient to produce 90% inhibition.
10. The rate of K:K exchange depended on the orthophosphate concentration inside the ghosts (confirming Glynn, Lew & Lüthi, 1970). The curve obtained suggested that the rate was half-maximal at about 1·7 mM orthophosphate.
11. These experiments suggested that inhibition by internal K is an important factor affecting the Na efflux from intact red cells. Experiments measuring Na:K exchange as a function of internal Na in low-K ghosts supported this hypothesis.
12. The significance of these findings is discussed.
Full text
PDF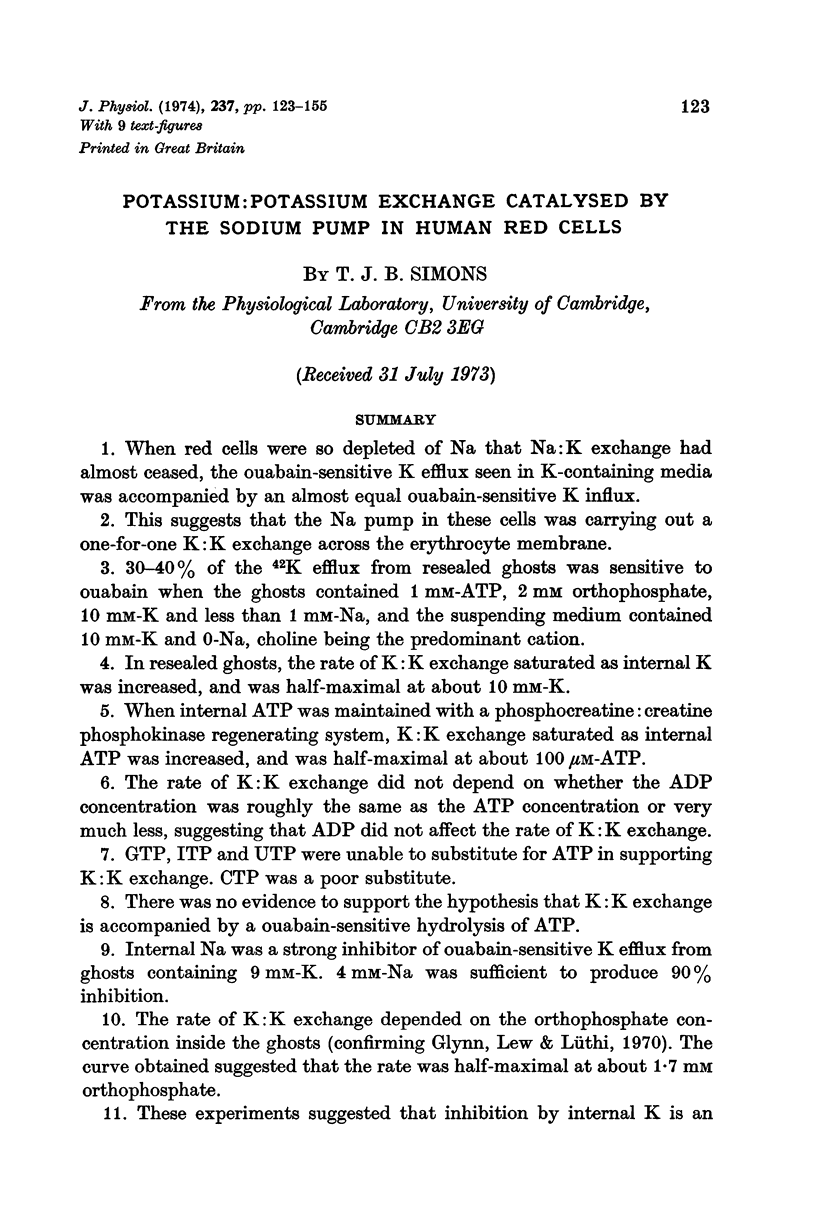
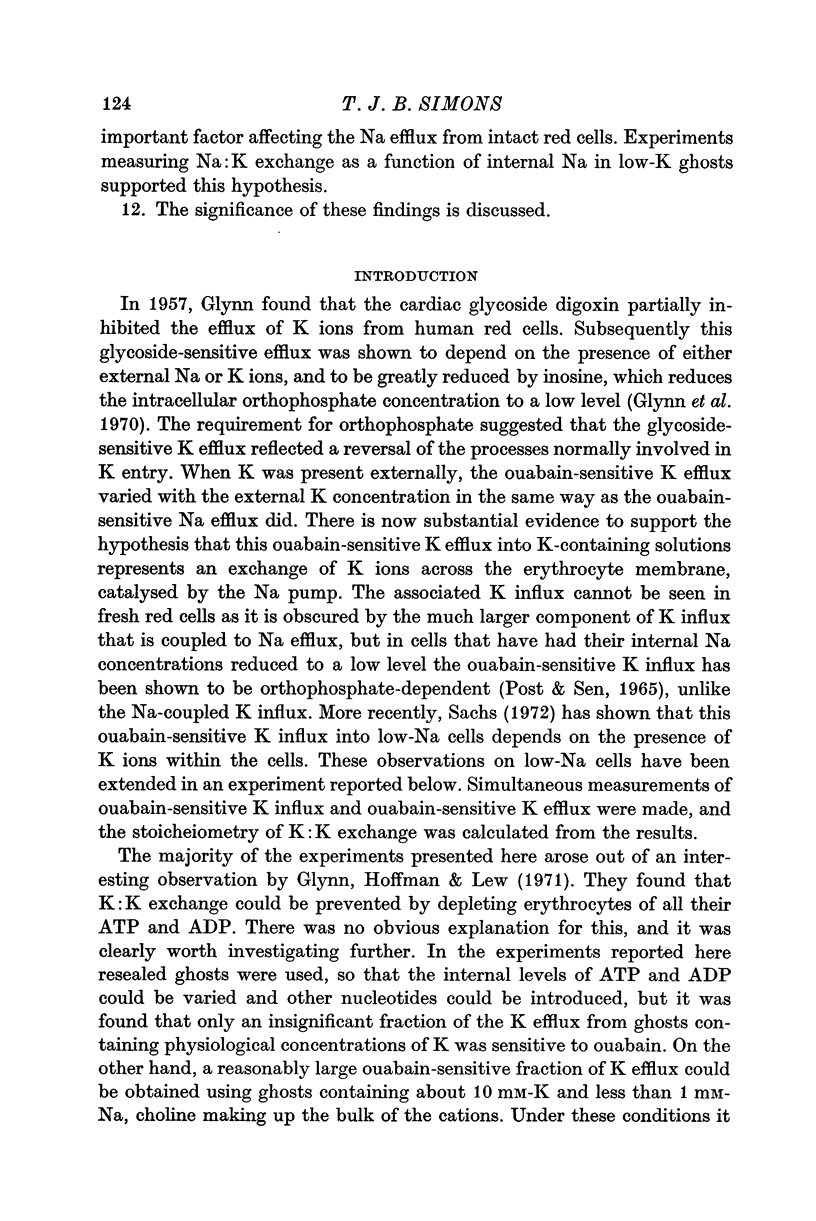
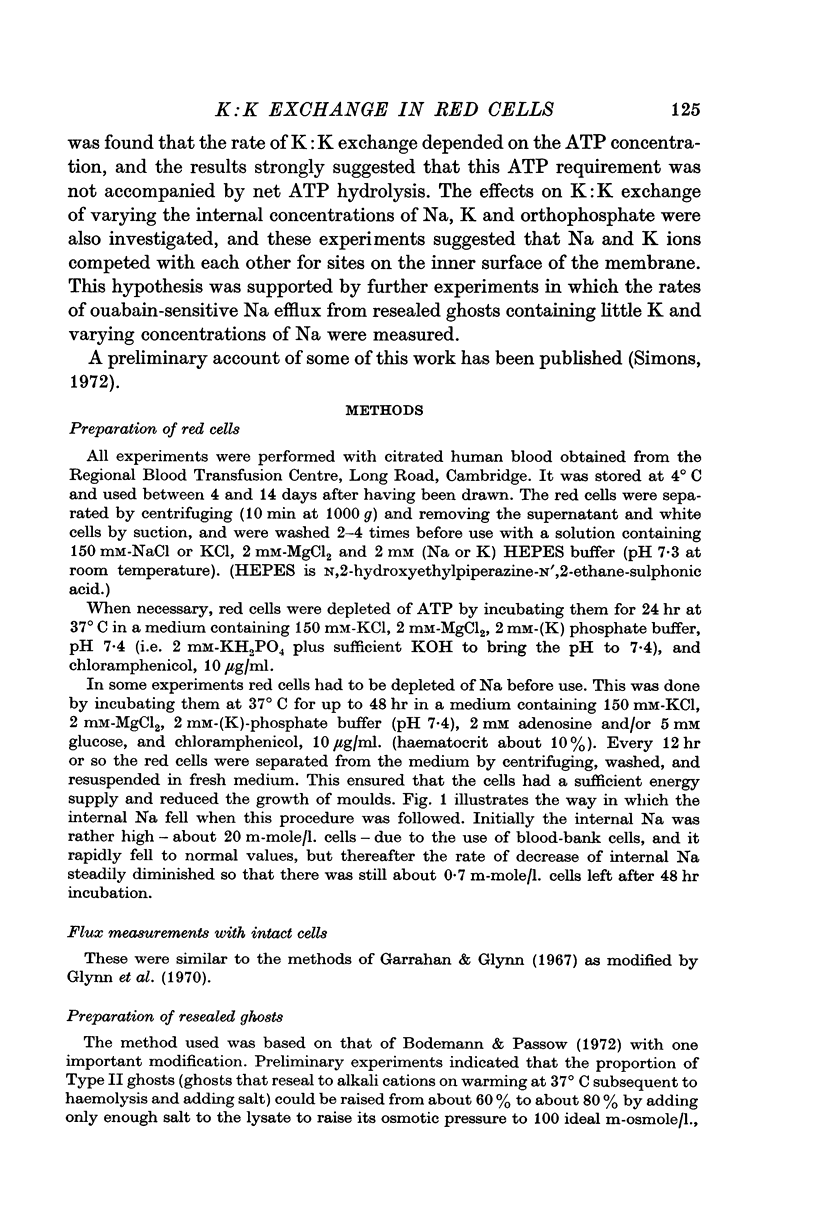
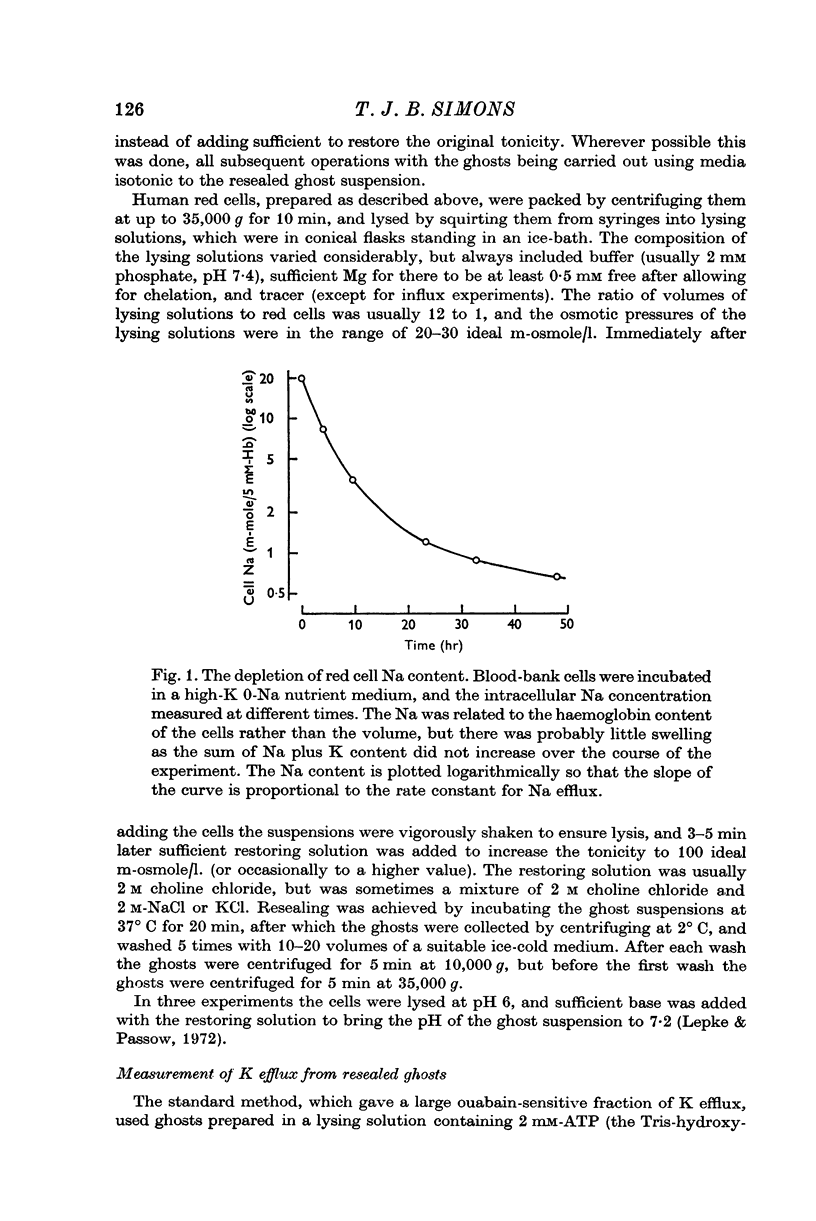
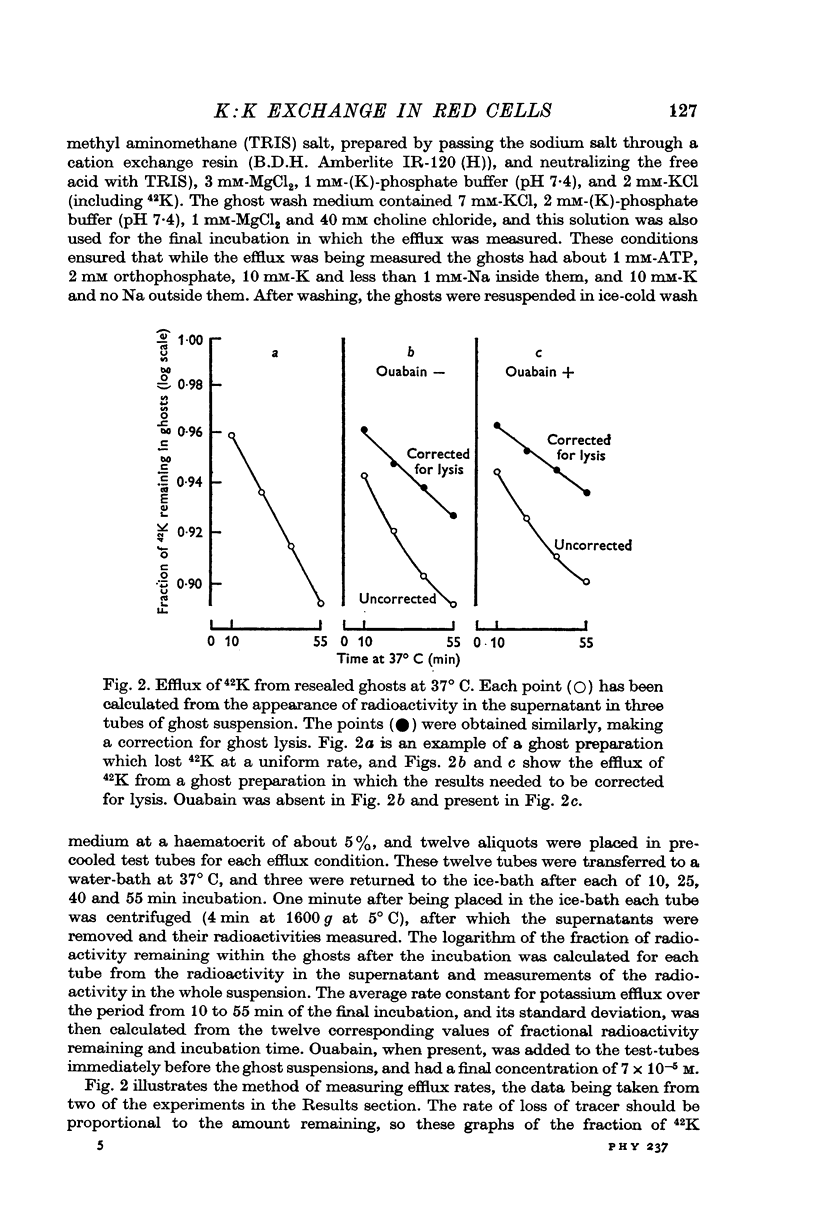
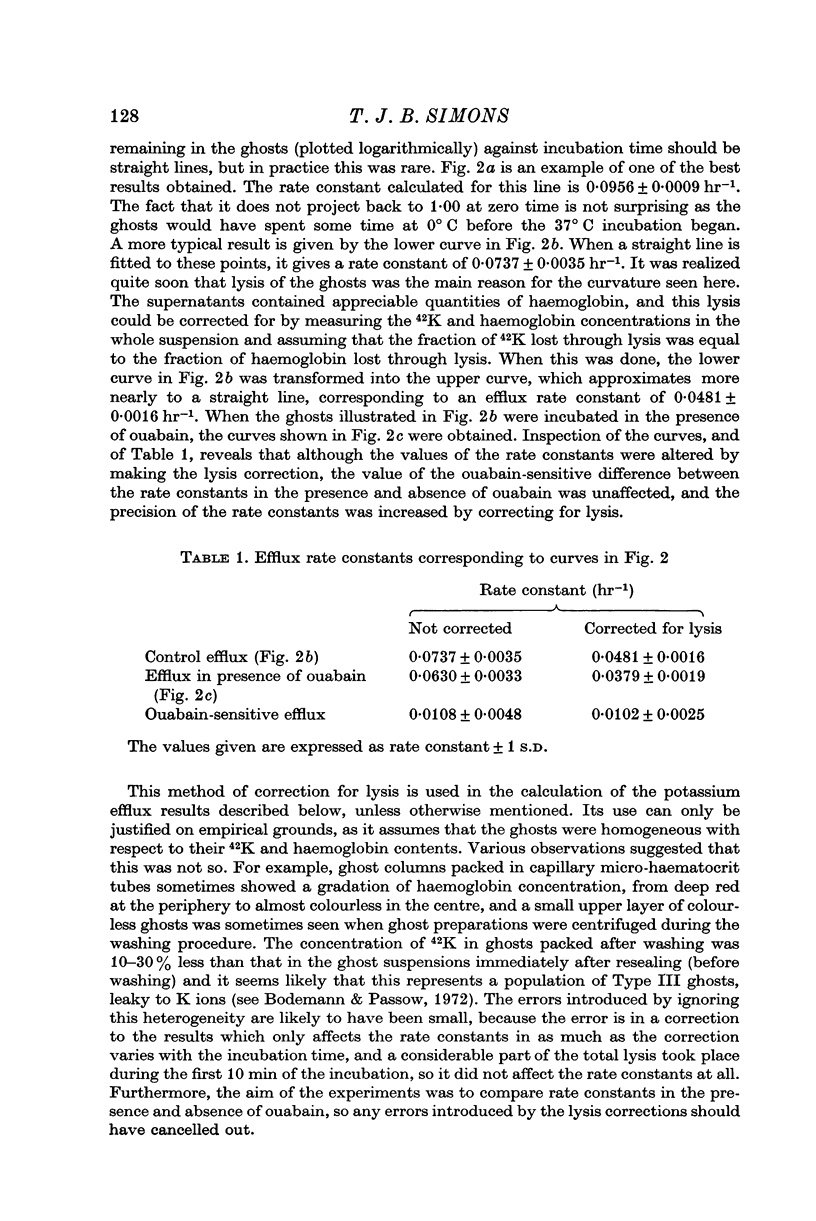
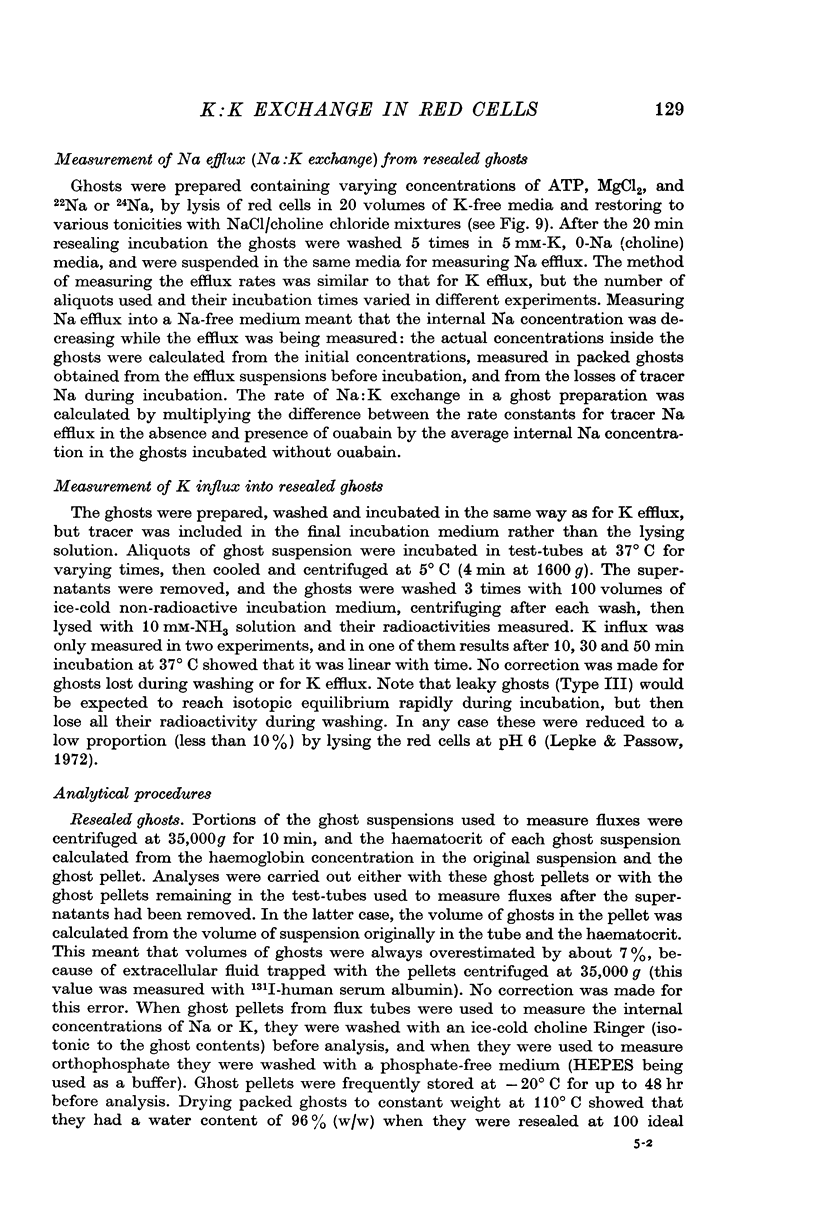
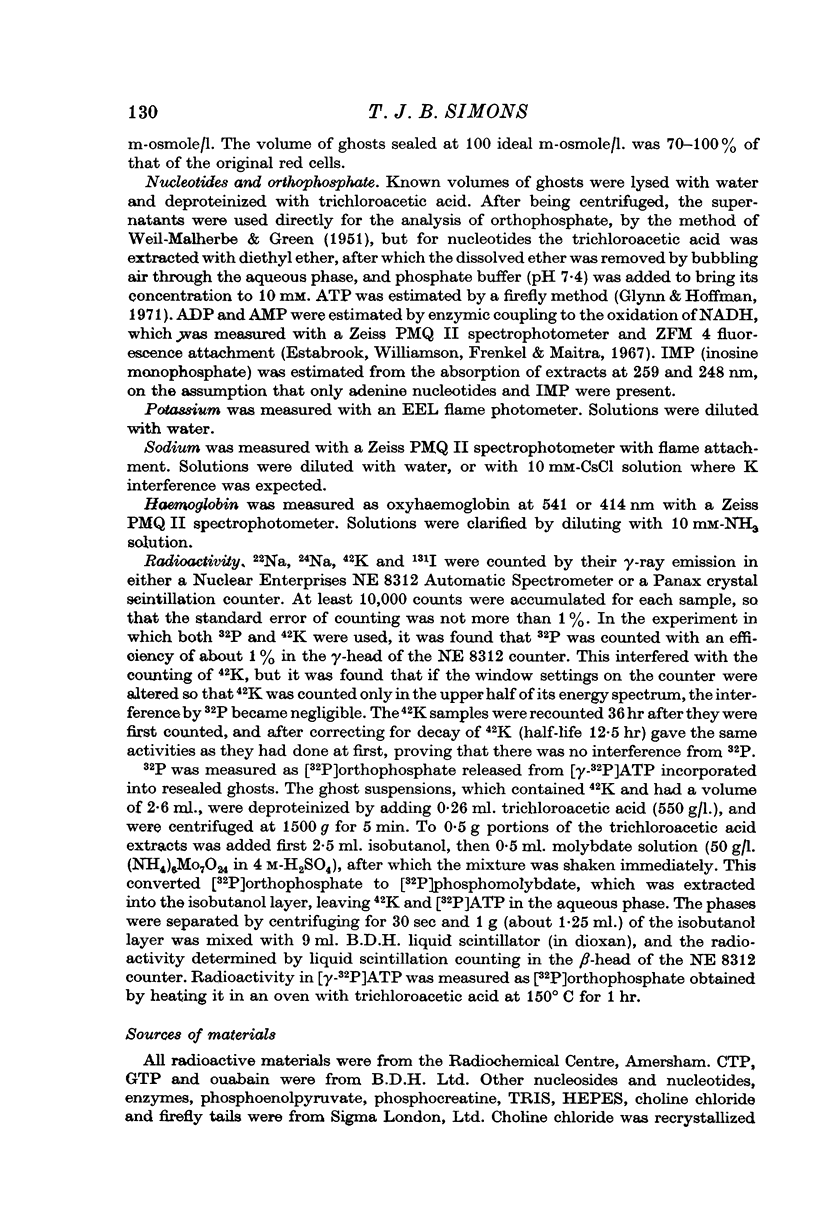
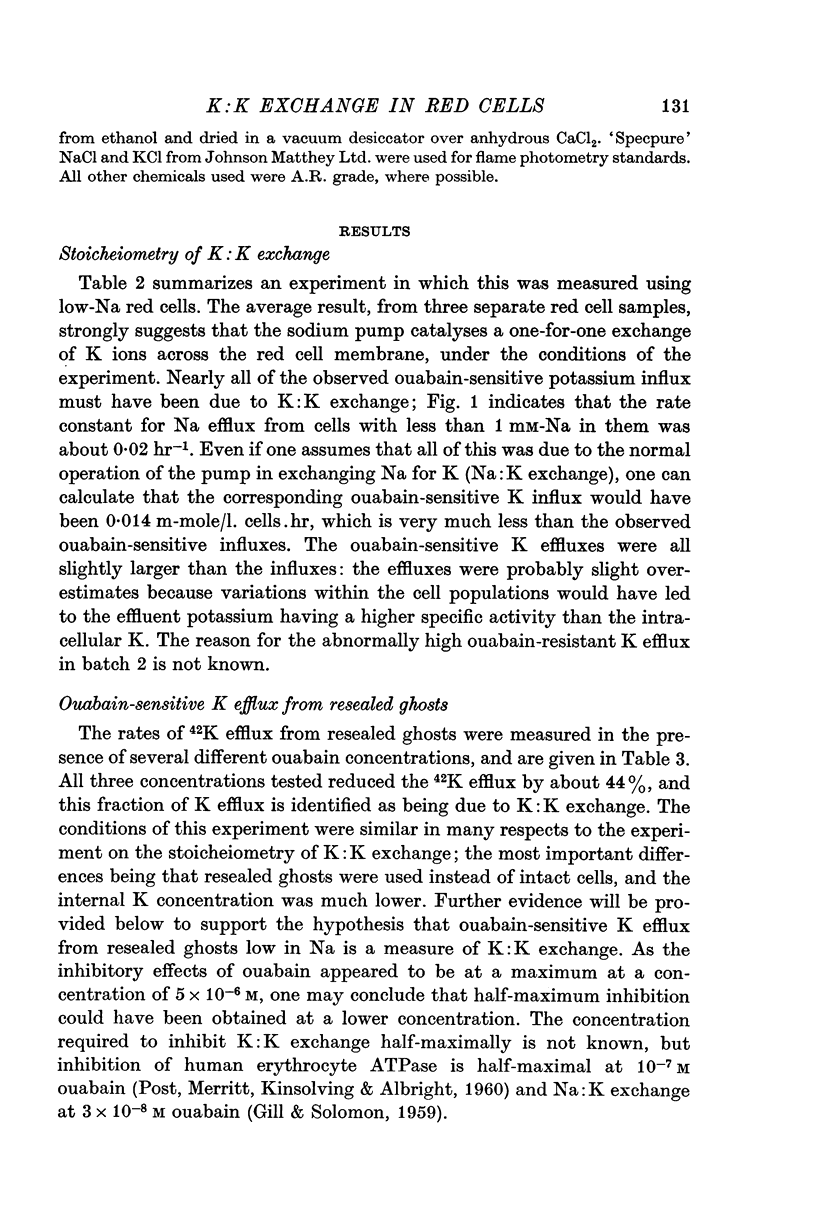
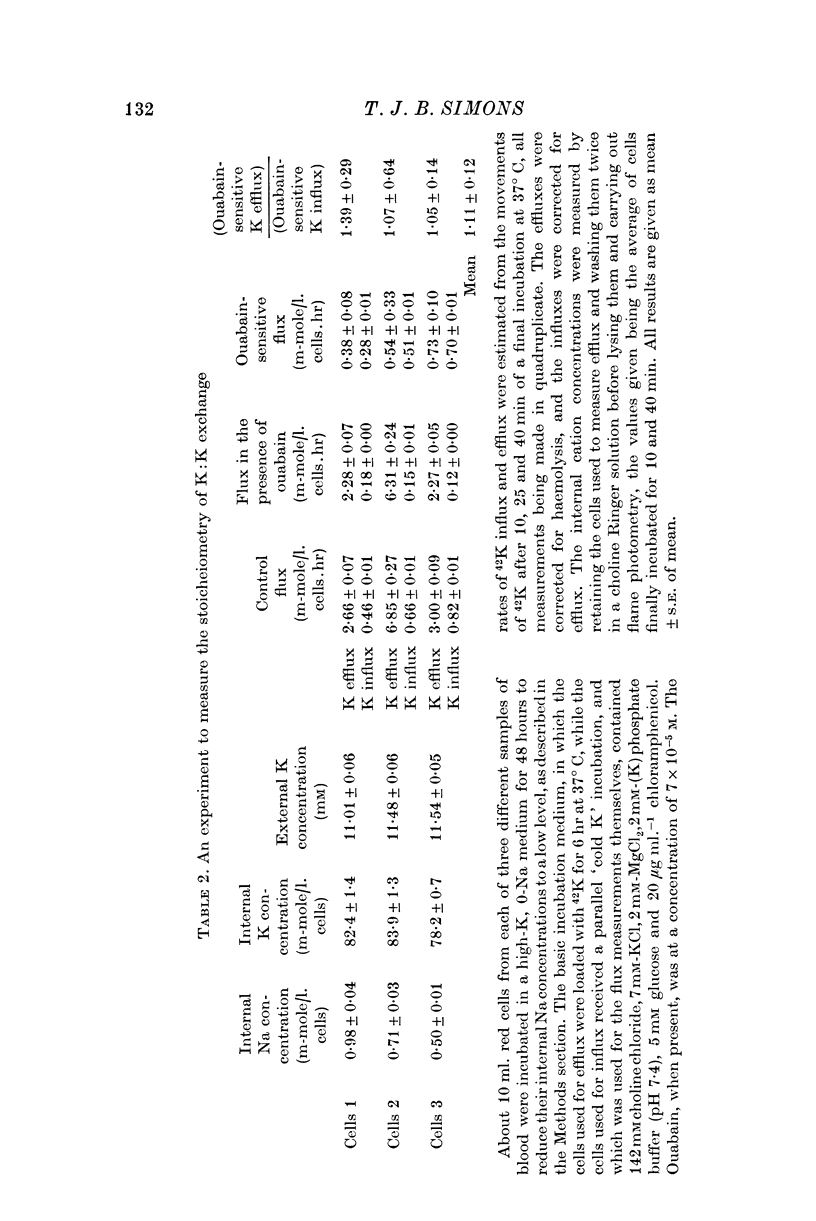
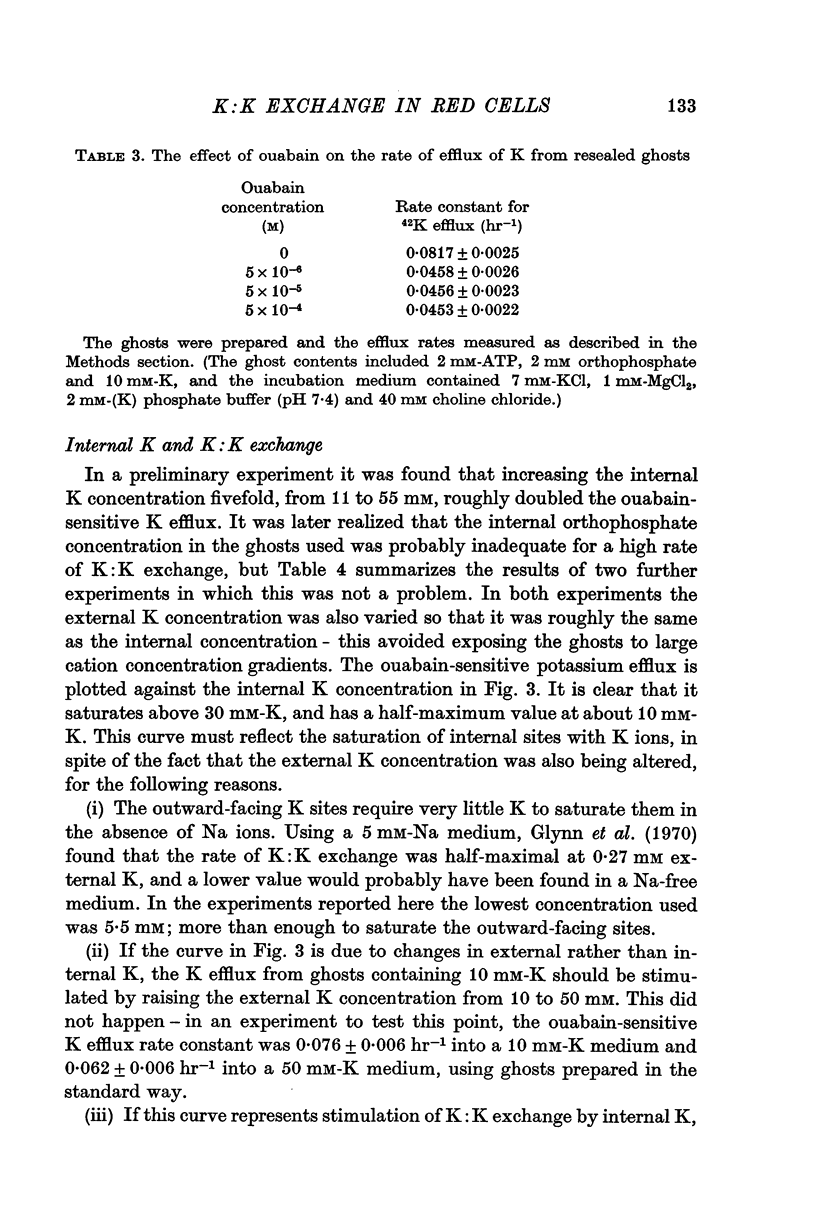
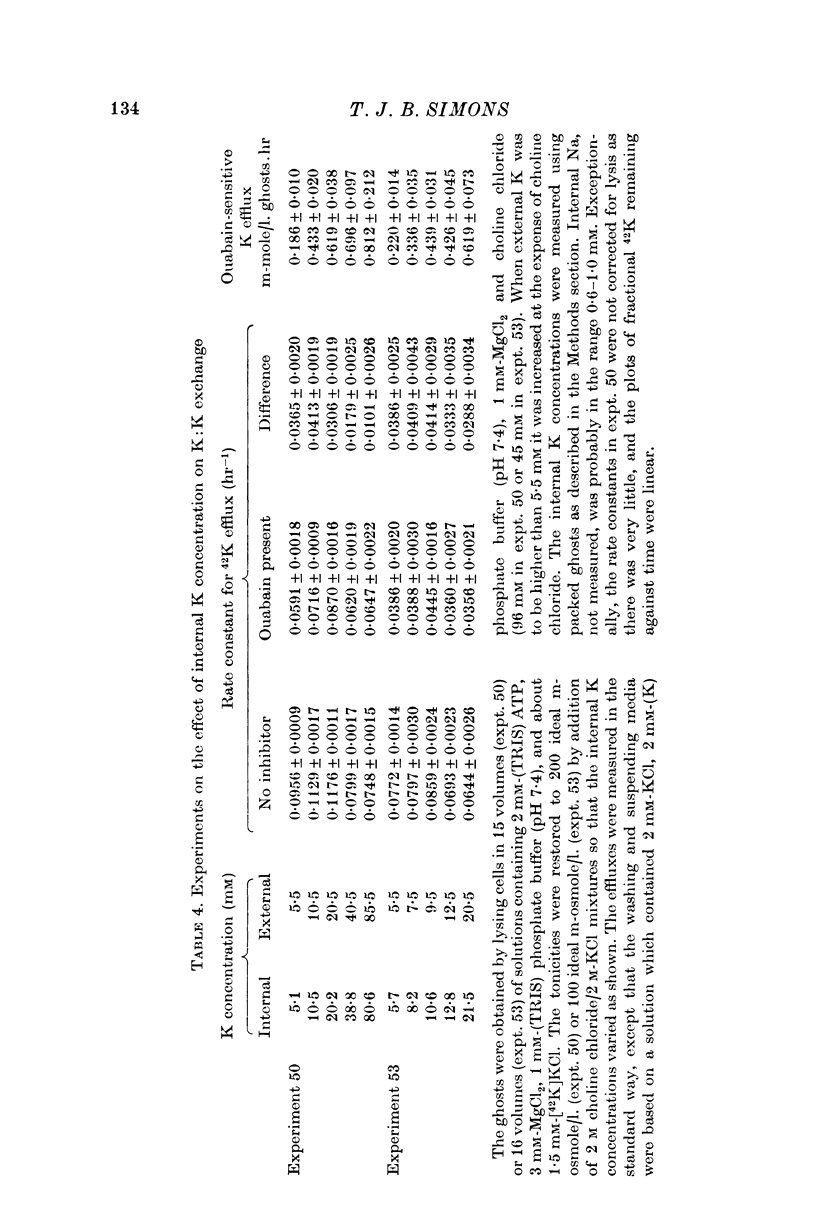
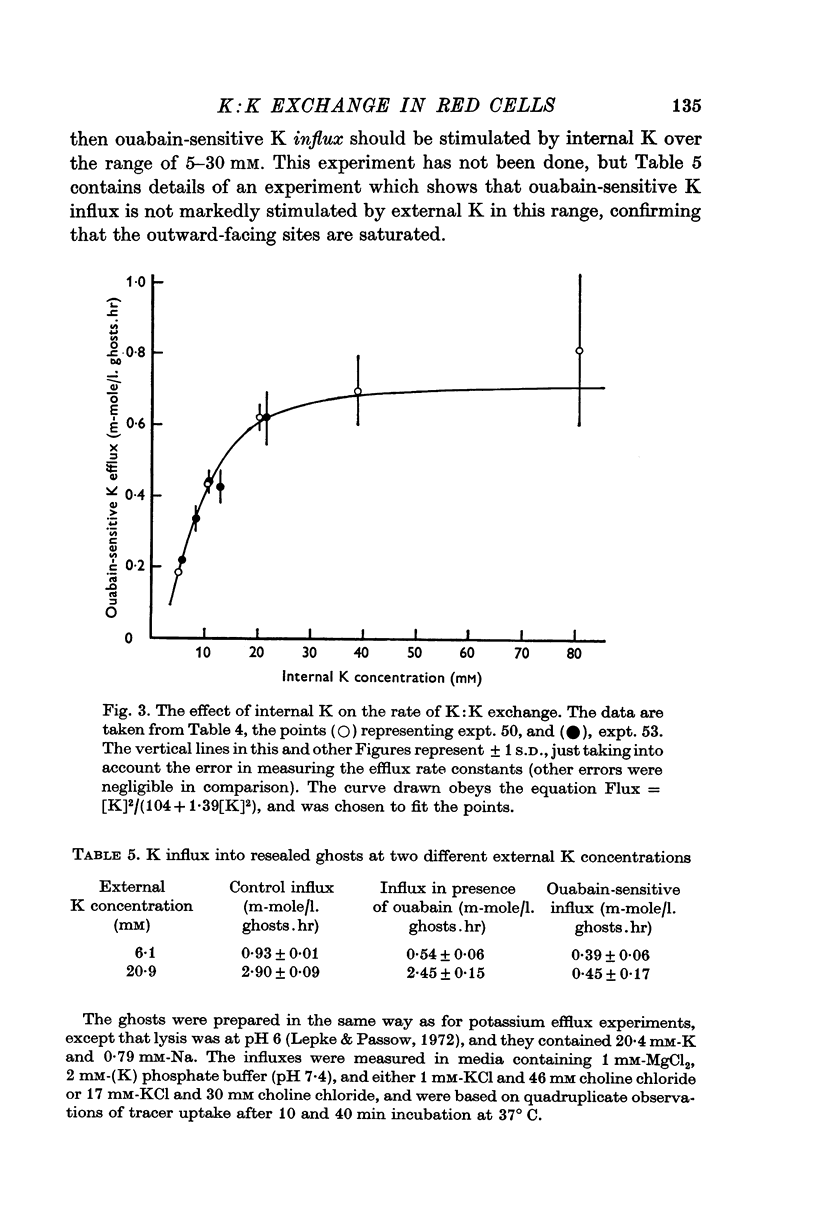
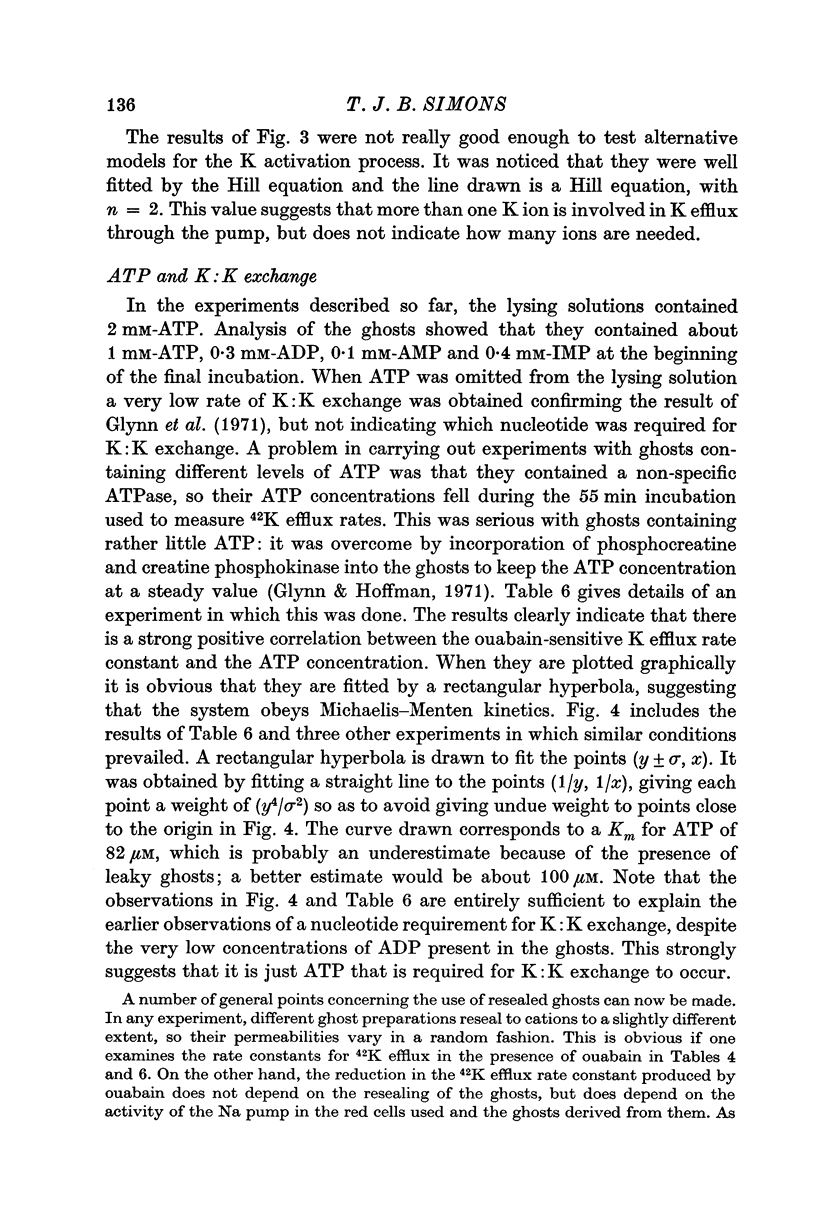
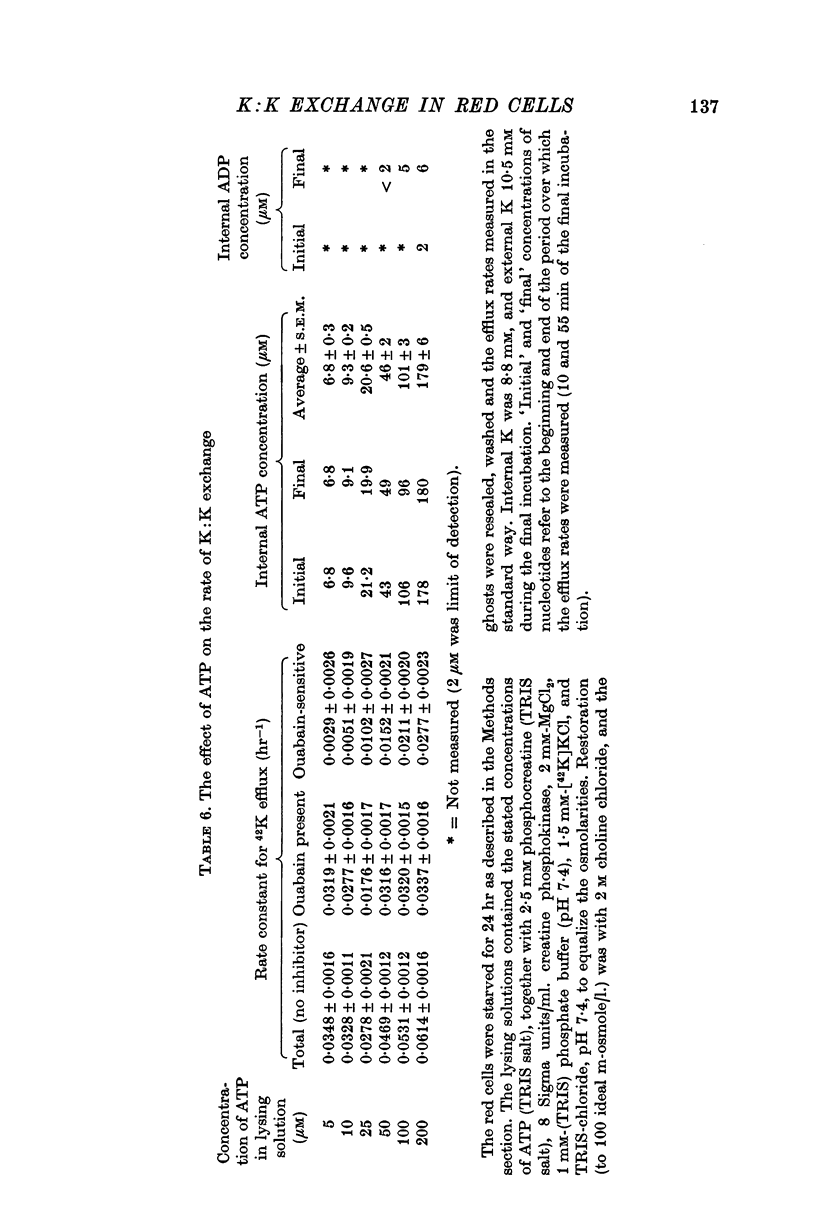
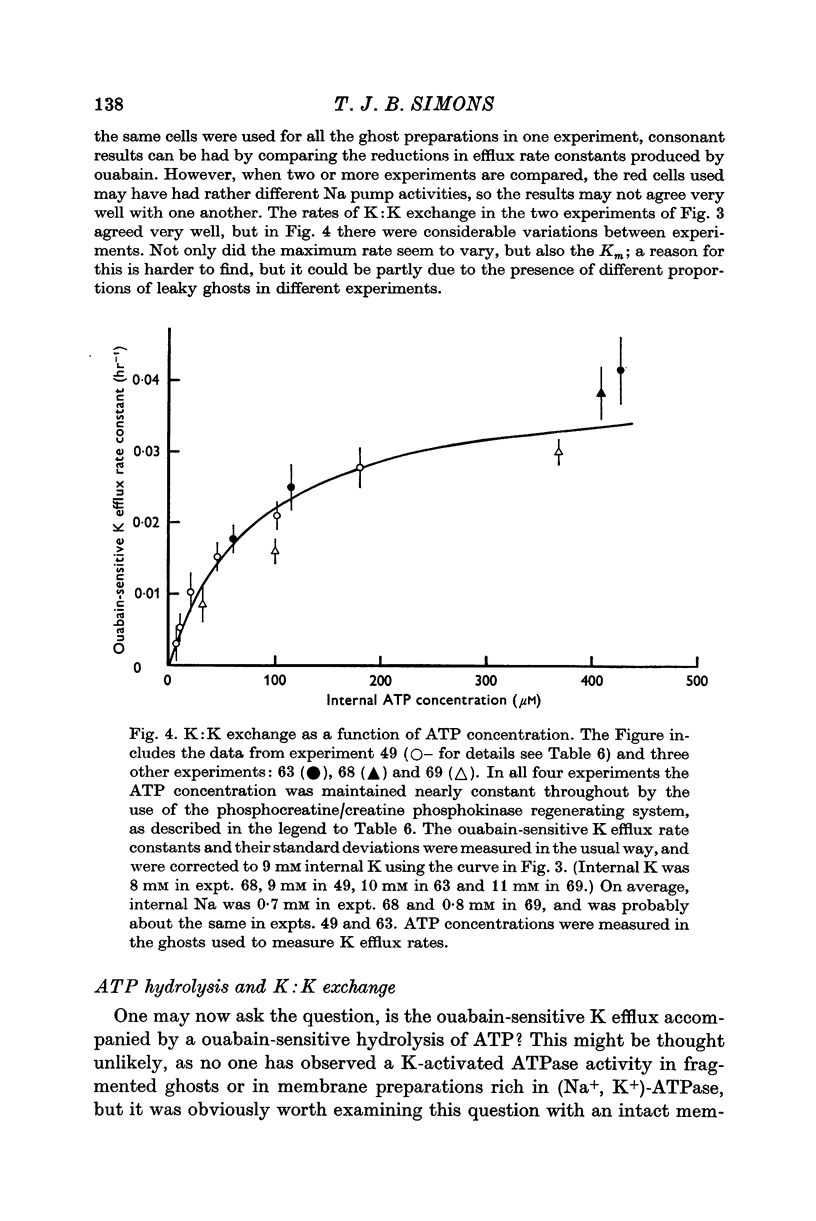
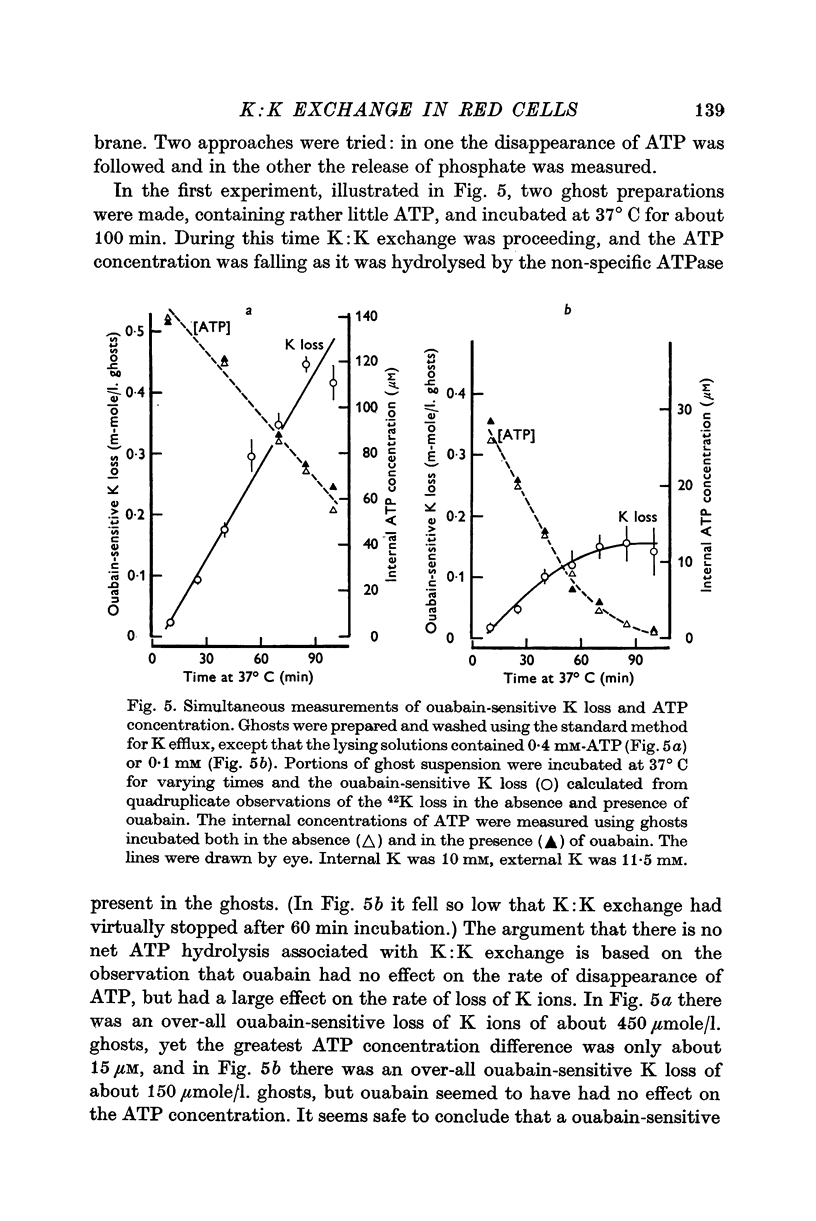
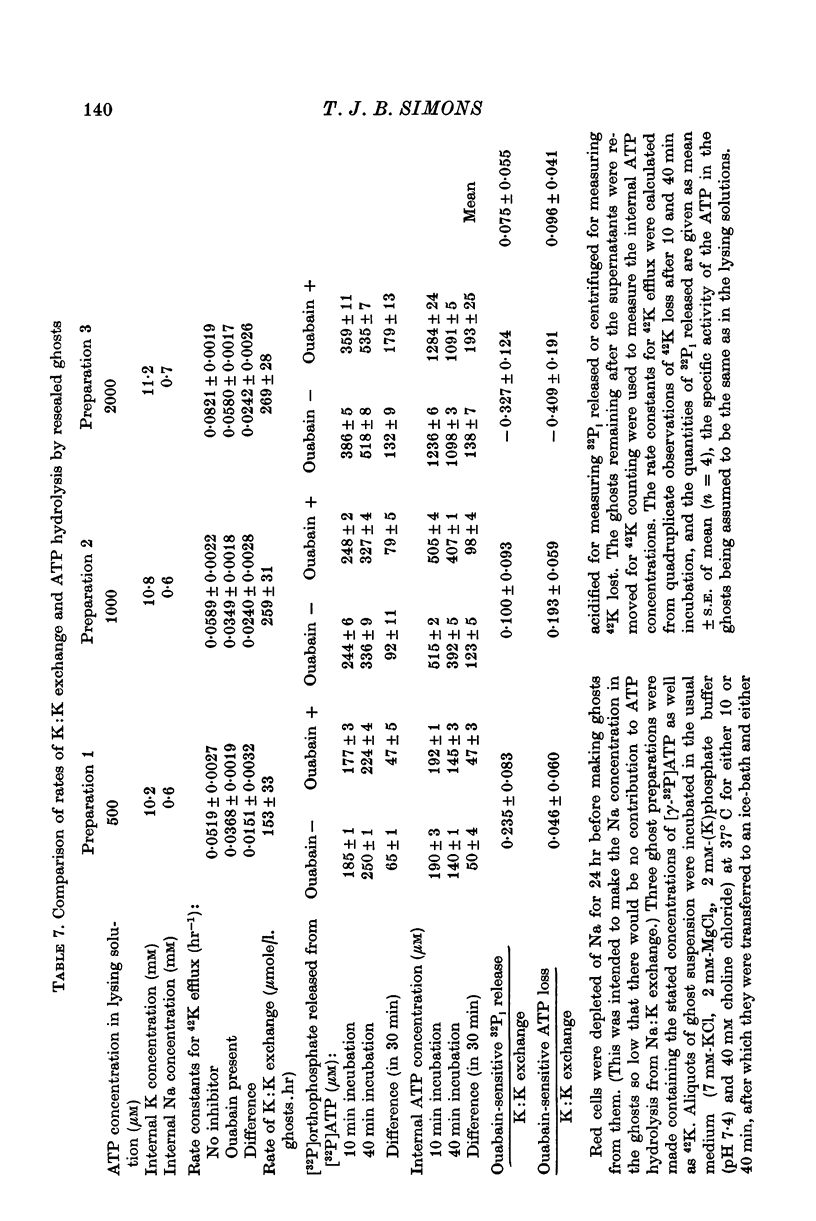
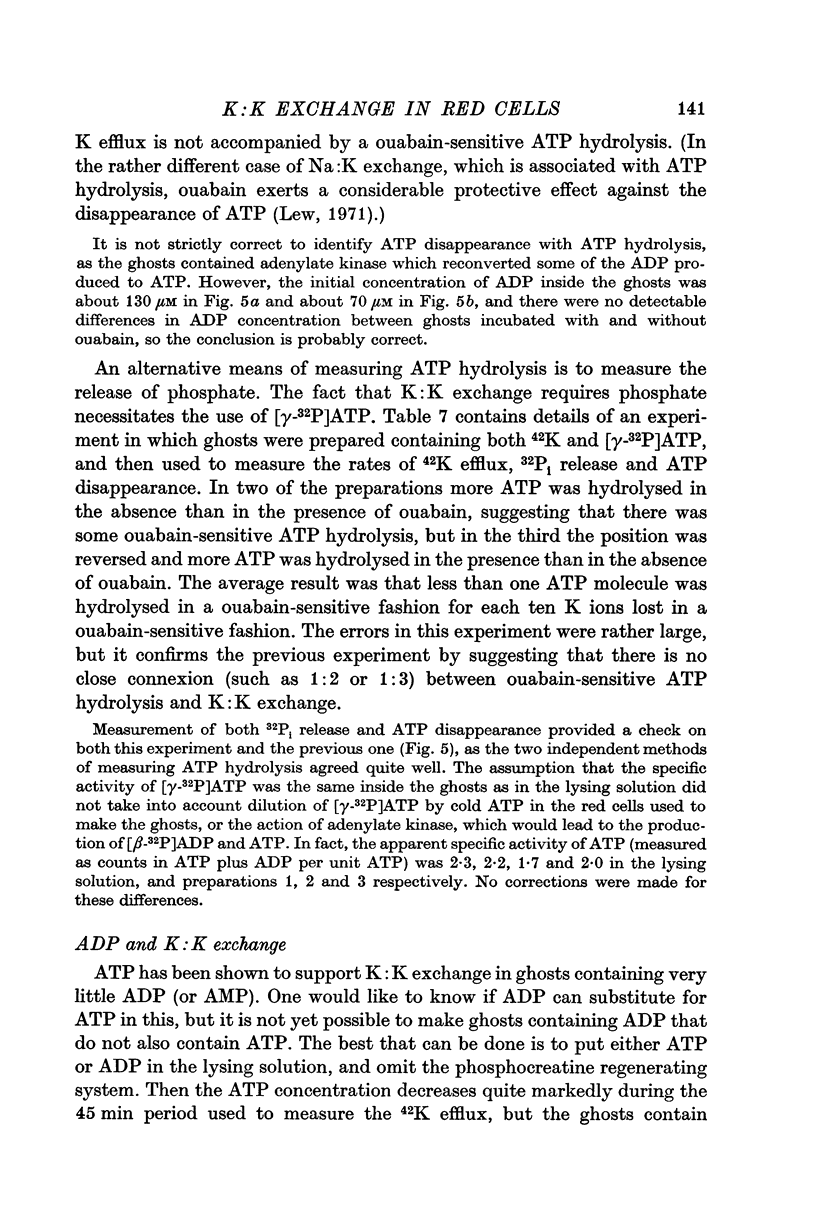
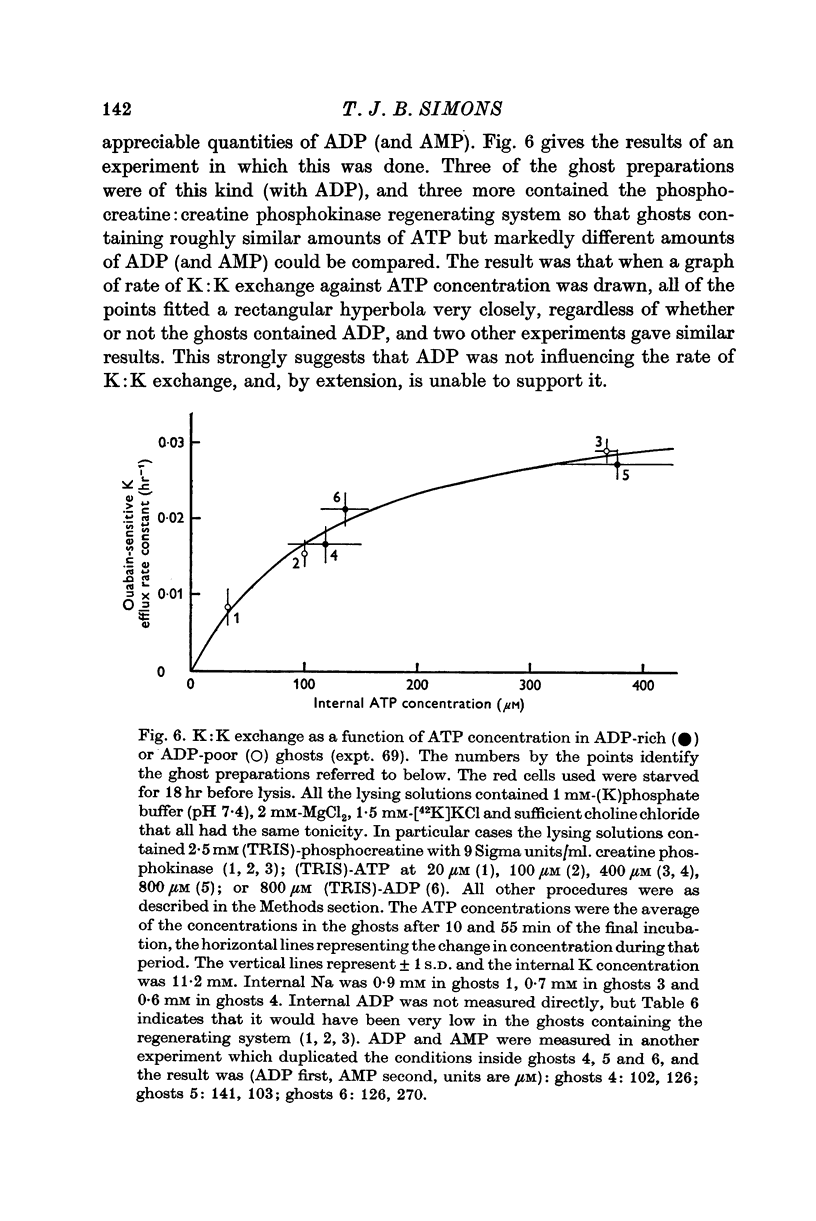
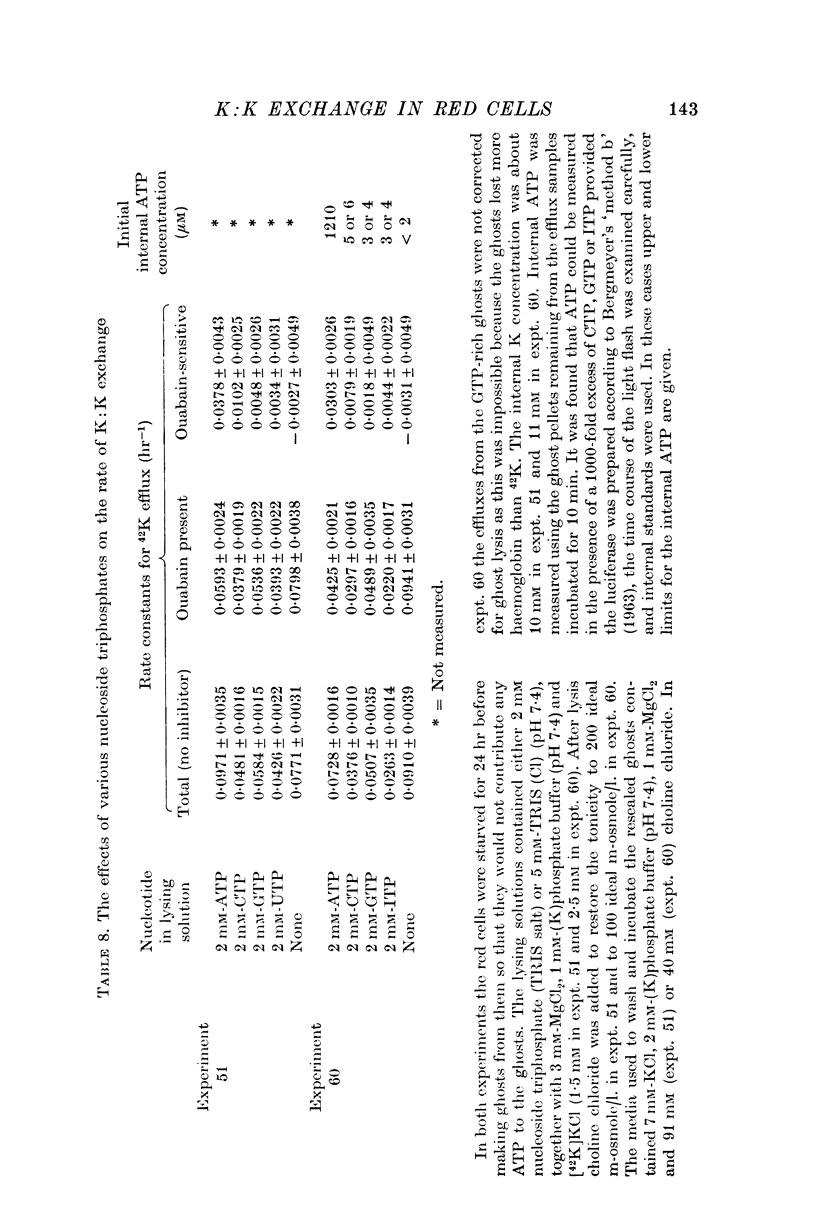
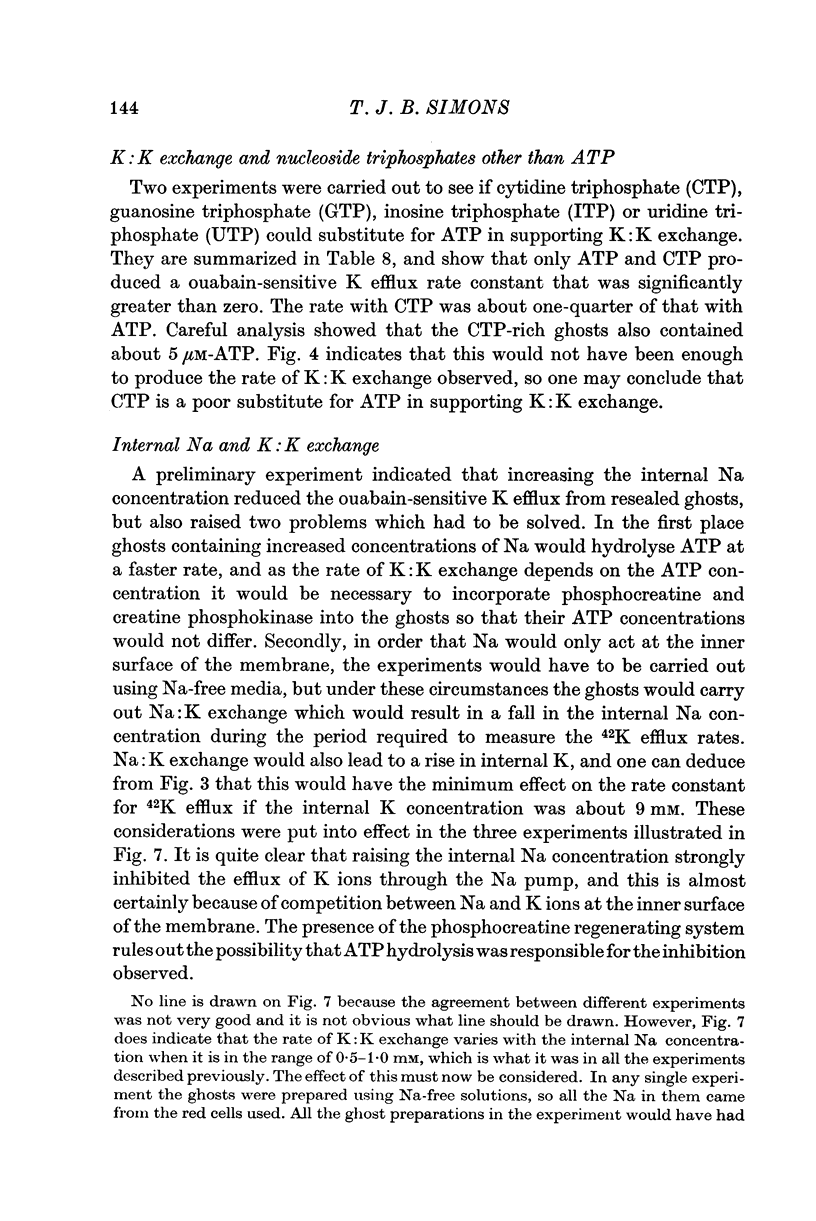
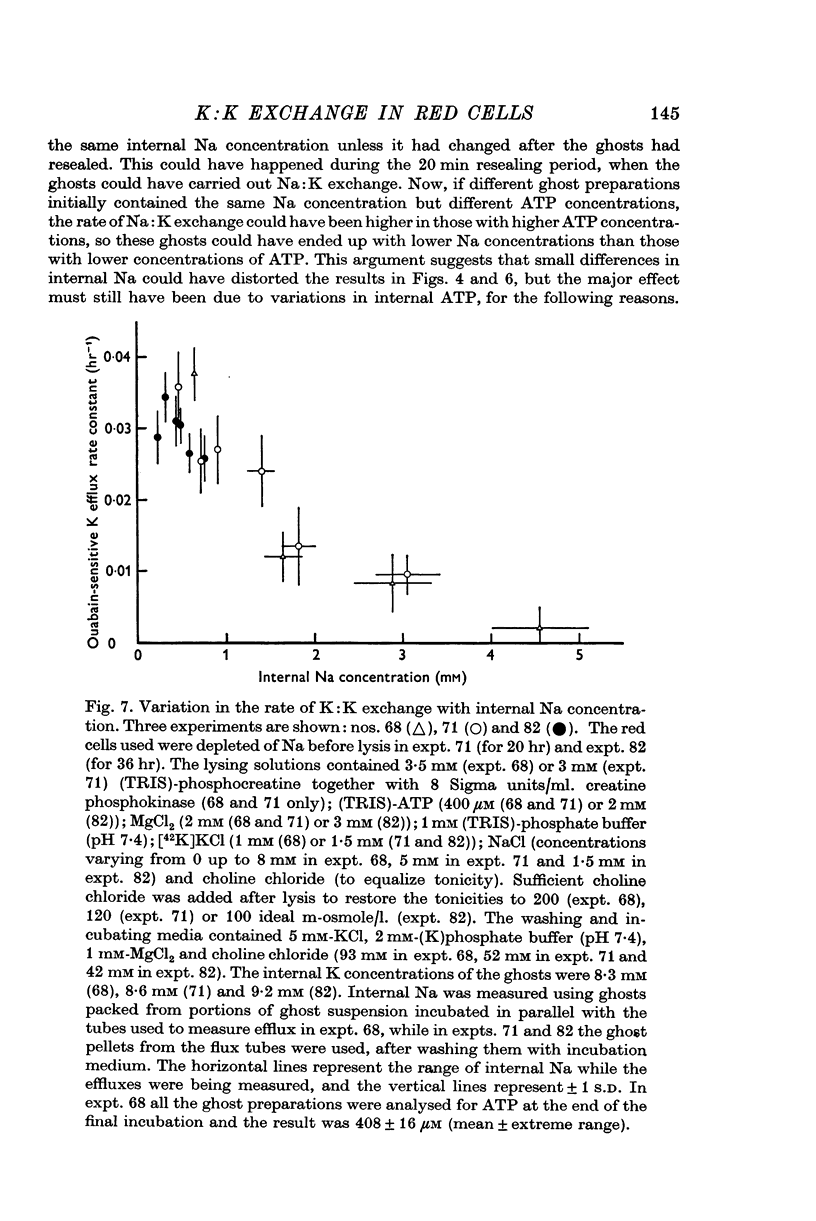
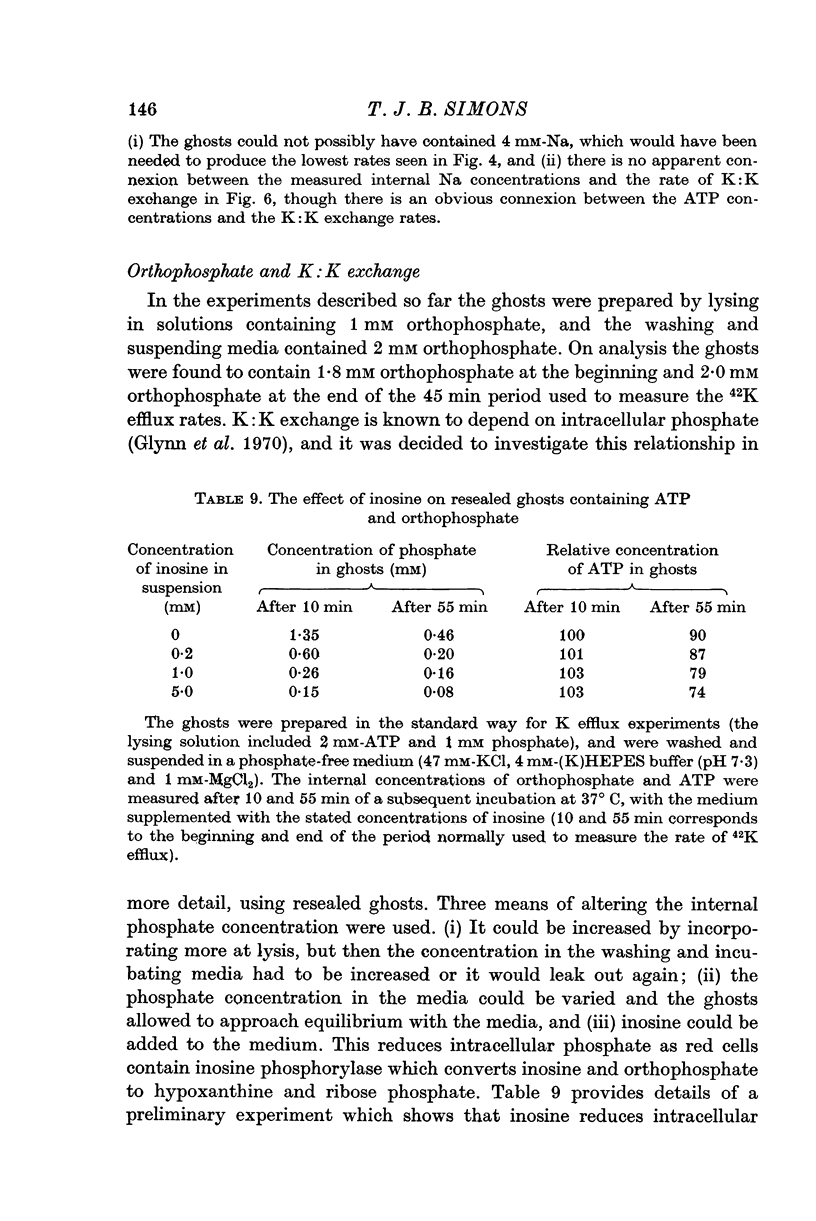
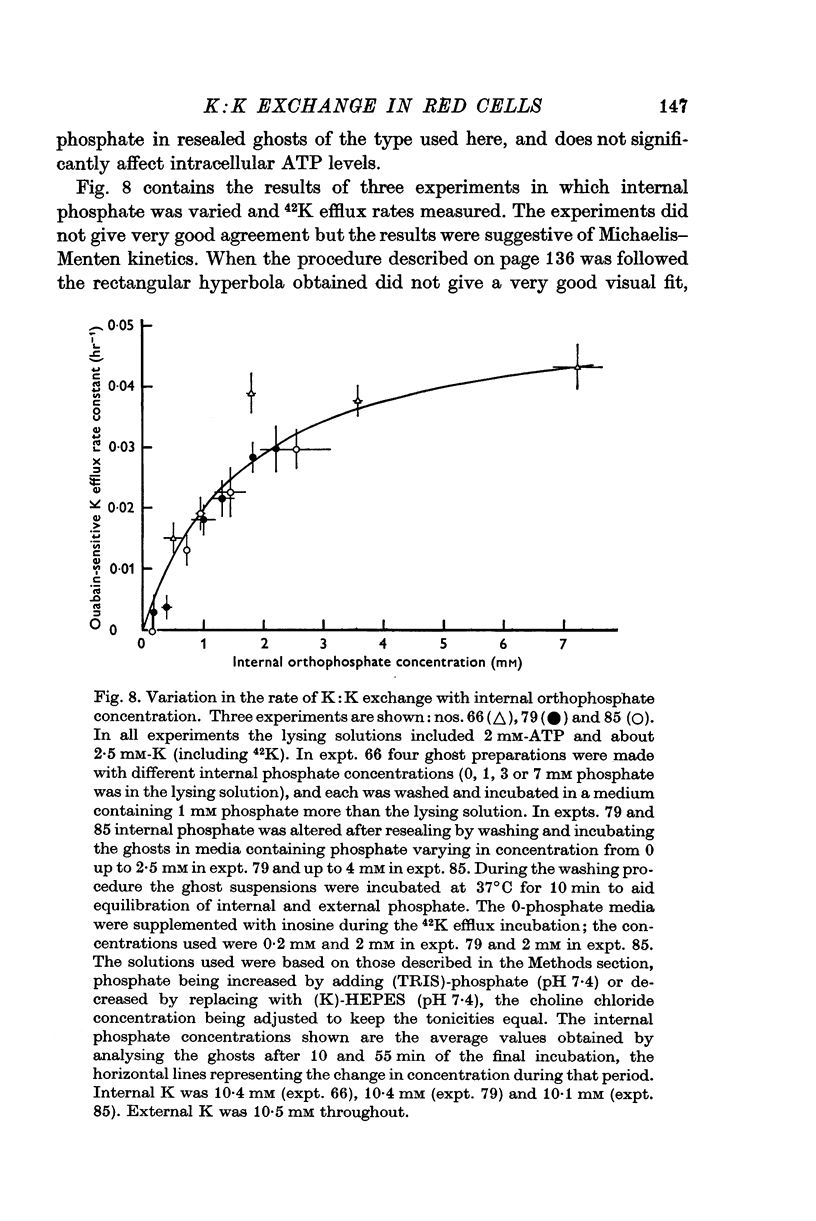
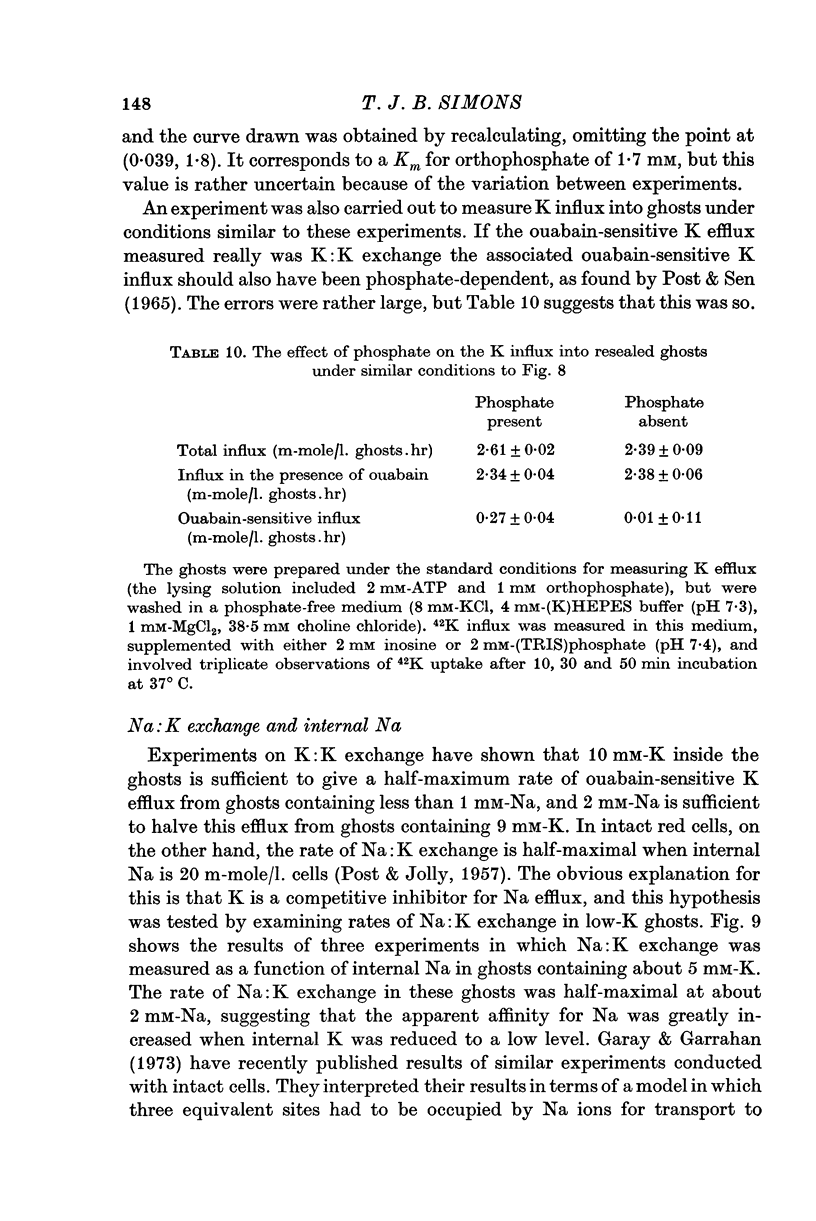
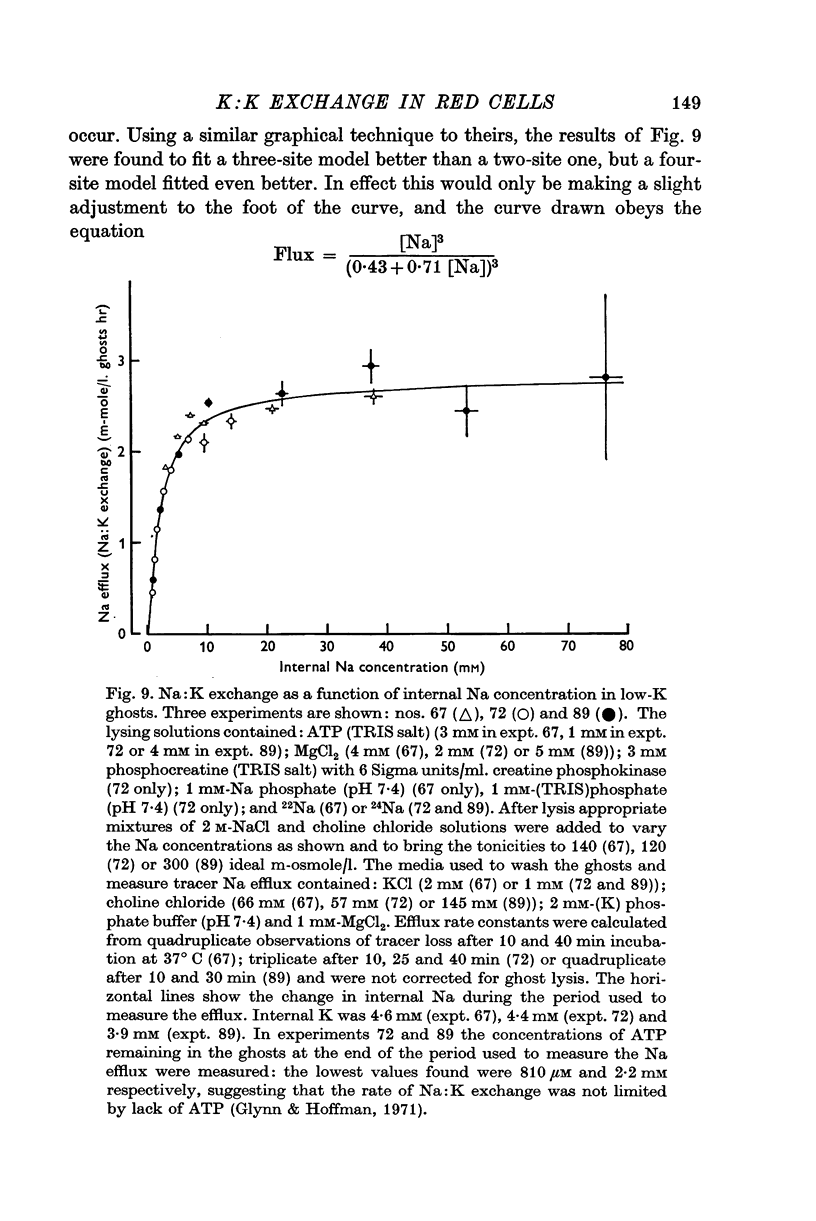
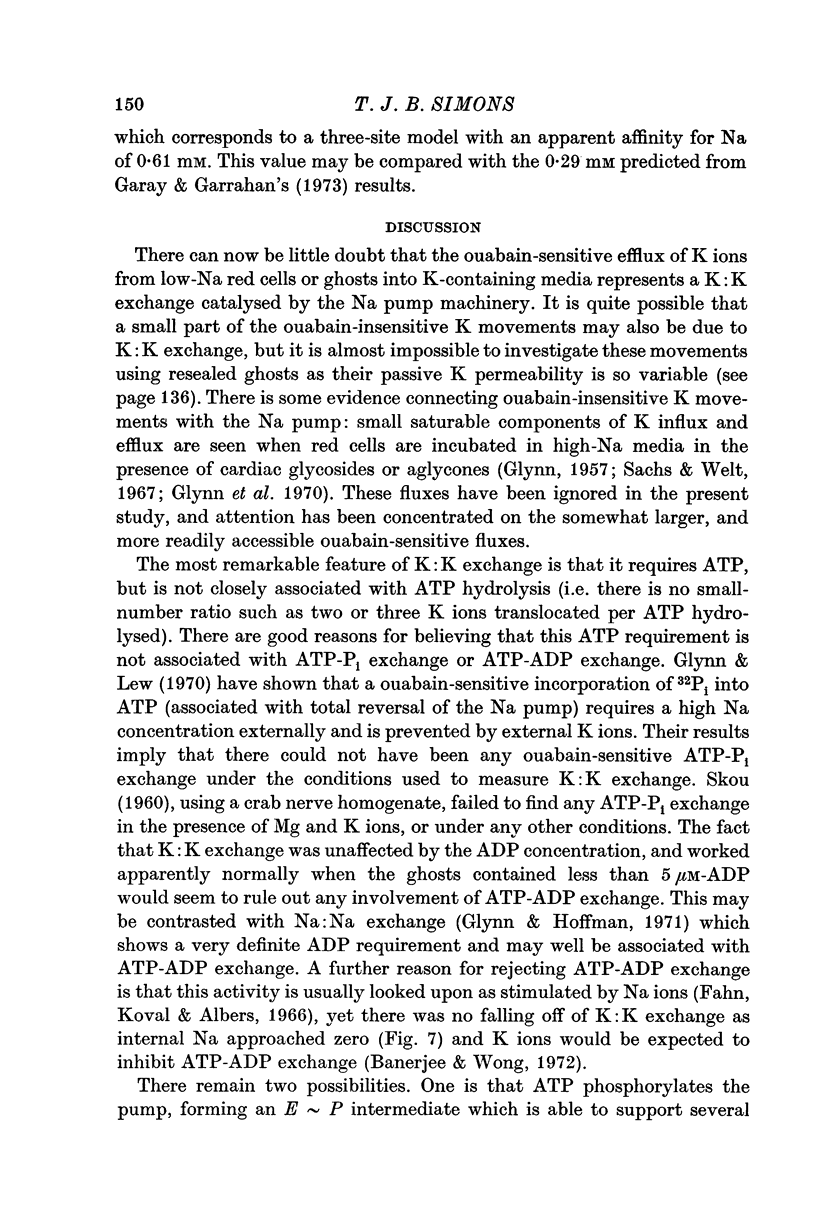
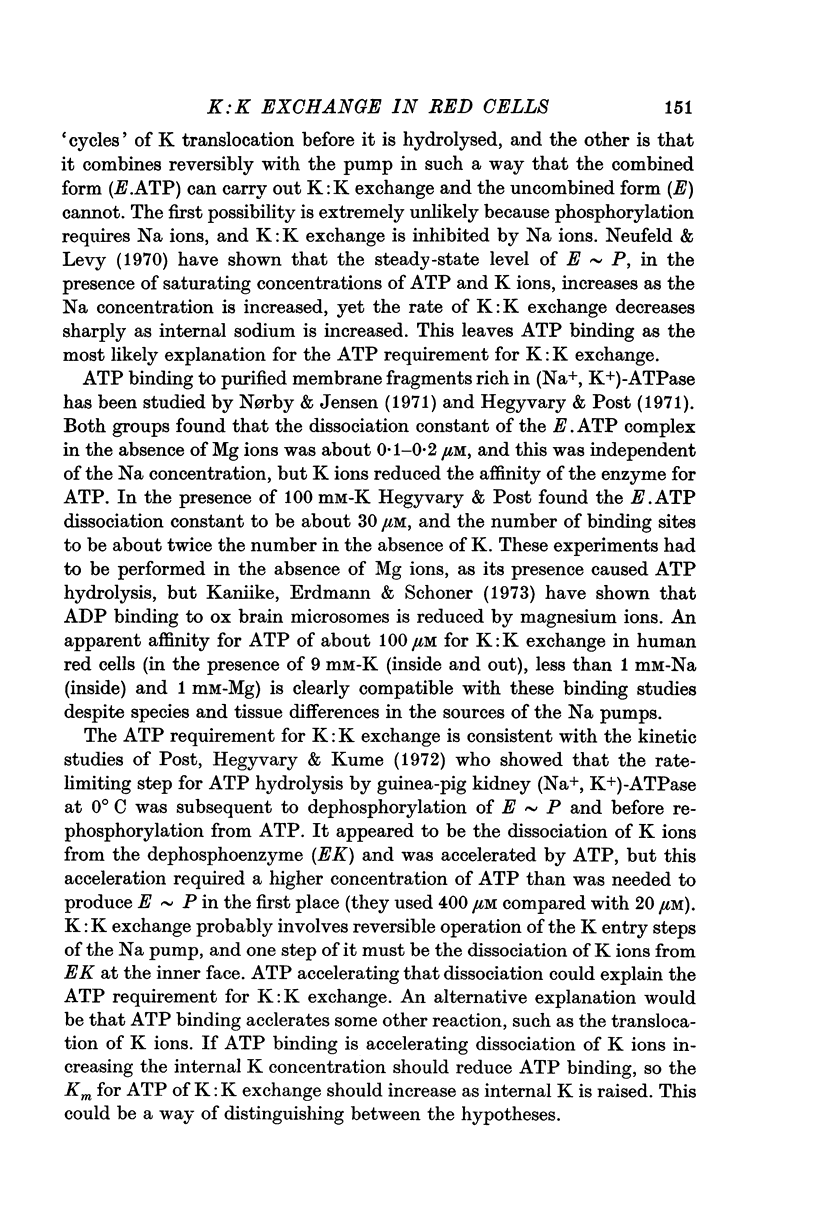
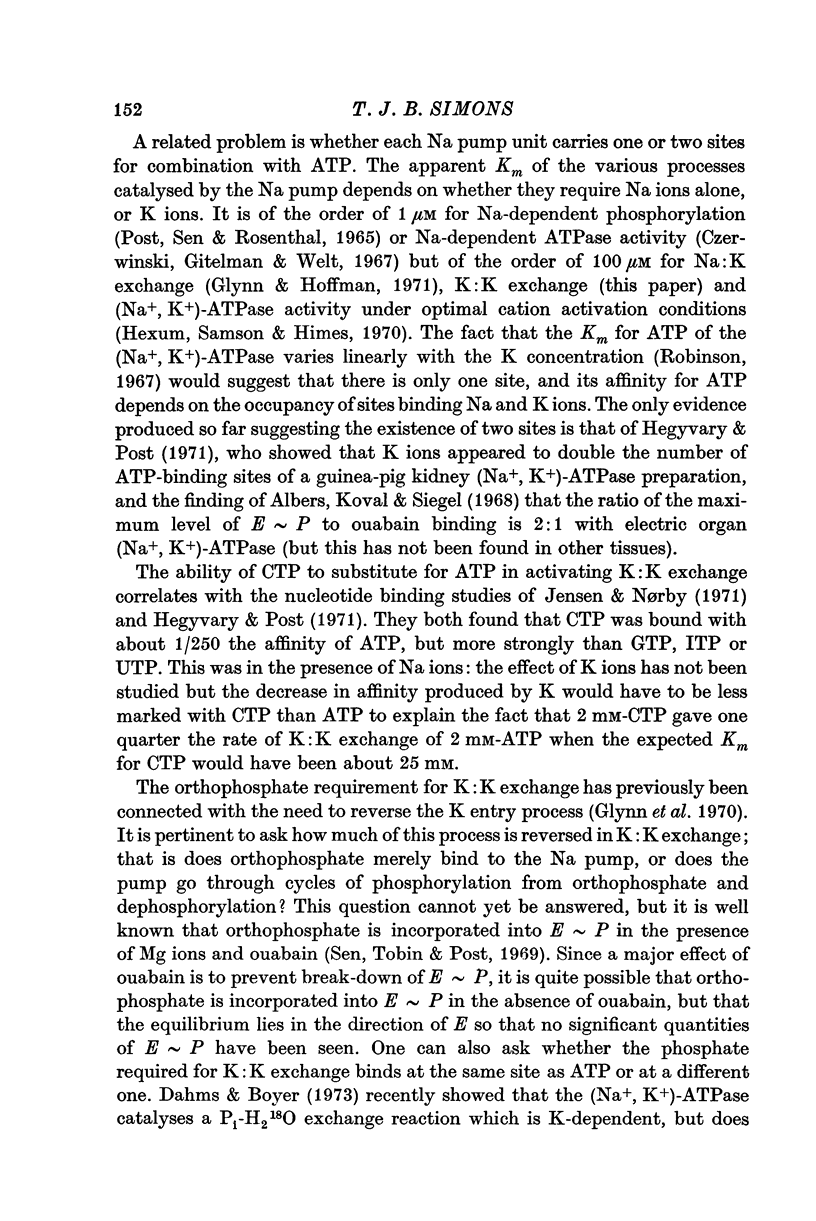
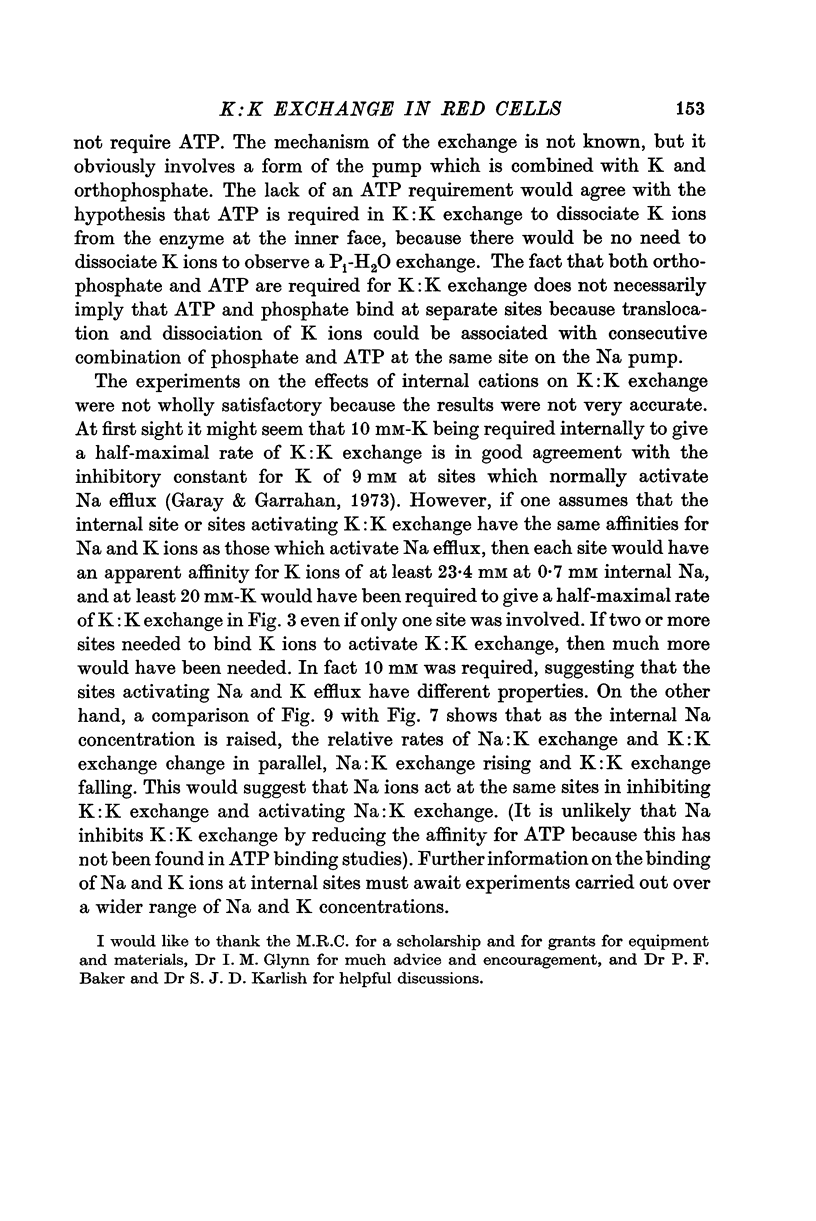
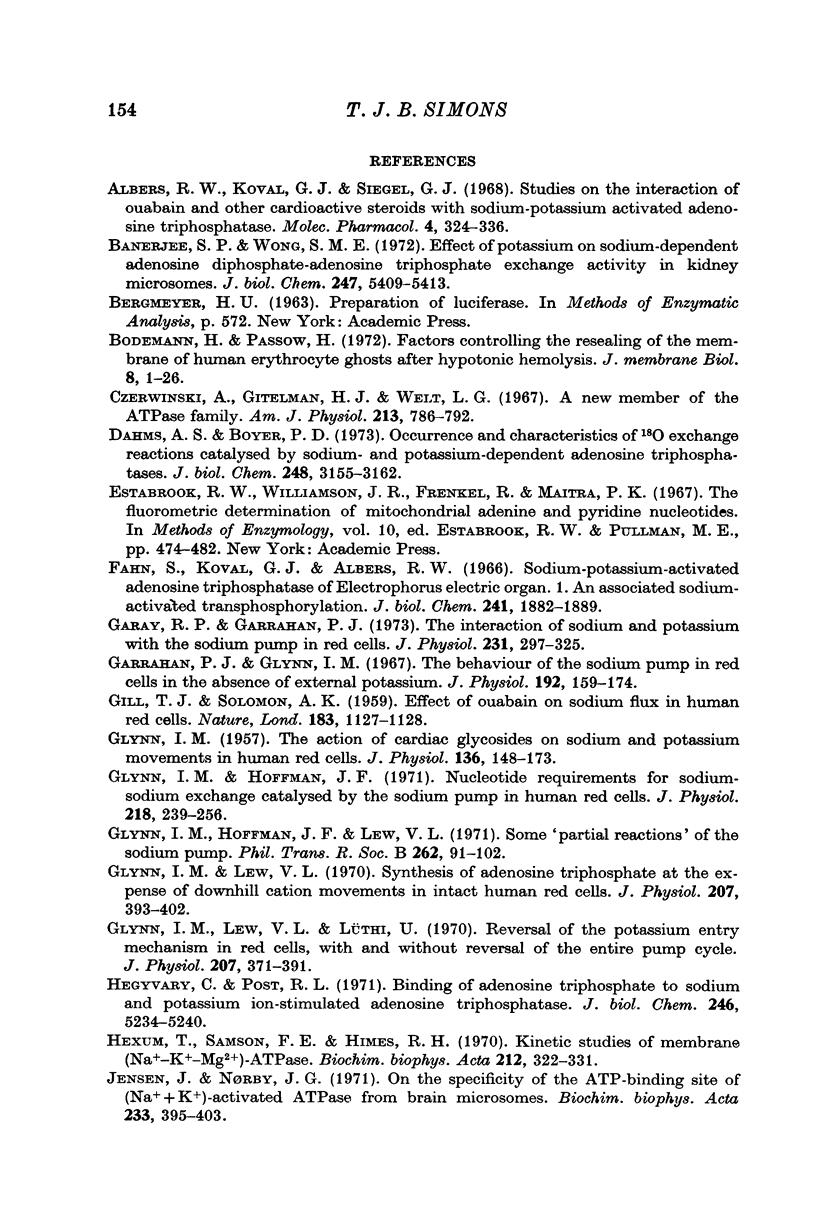
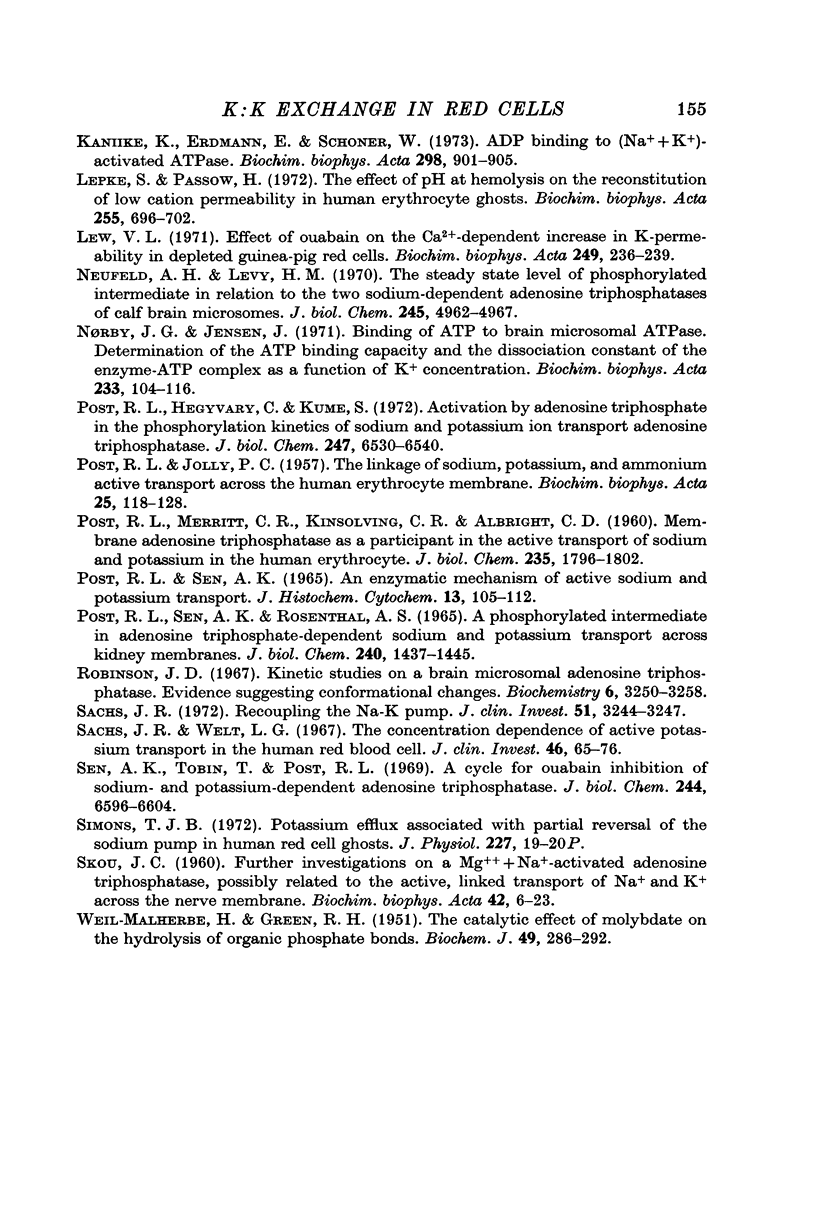
Selected References
These references are in PubMed. This may not be the complete list of references from this article.
- Albers R. W., Koval G. J., Siegel Studies on the interaction of ouabain and other cardio-active steroids with sodium-potassium-activated adenosine triphosphatase. Mol Pharmacol. 1968 Jul;4(4):324–336. [PubMed] [Google Scholar]
- Banerjee S. P., Wong S. M. Effect of potassium on sodium-dependent adenosine diphosphate-adenosine triphosphate exchange activity in kidney microsomes. J Biol Chem. 1972 Sep 10;247(17):5409–5413. [PubMed] [Google Scholar]
- Bodemann H., Passow H. Factors controlling the resealing of the membrane of human erythrocyte ghosts after hypotonic hemolysis. J Membr Biol. 1972;8(1):1–26. doi: 10.1007/BF01868092. [DOI] [PubMed] [Google Scholar]
- Czerwinski A., Gitelman H. J., Welt L. G. A new member of the ATPase family. Am J Physiol. 1967 Sep;213(3):786–792. doi: 10.1152/ajplegacy.1967.213.3.786. [DOI] [PubMed] [Google Scholar]
- Dahms A. S., Boyer P. D. Occurrence and characteristics of 18 O exchange reactions catalyzed by sodium- and potassium-dependent adenosine triphosphatases. J Biol Chem. 1973 May 10;248(9):3155–3162. doi: 10.2172/4485348. [DOI] [PubMed] [Google Scholar]
- Fahn S., Koval G. J., Albers R. W. Sodium-potassium-activated adenosine triphosphatase of Electrophorus electric organ. I. An associated sodium-activated transphosphorylation. J Biol Chem. 1966 Apr 25;241(8):1882–1889. [PubMed] [Google Scholar]
- GILL T. J., SOLOMON A. K. Effect of ouabain on sodium flux in human red cells. Nature. 1959 Apr 18;183(4668):1127–1128. doi: 10.1038/1831127a0. [DOI] [PubMed] [Google Scholar]
- GLYNN I. M. The action of cardiac glycosides on sodium and potassium movements in human red cells. J Physiol. 1957 Apr 3;136(1):148–173. doi: 10.1113/jphysiol.1957.sp005749. [DOI] [PMC free article] [PubMed] [Google Scholar]
- Garay R. P., Garrahan P. J. The interaction of sodium and potassium with the sodium pump in red cells. J Physiol. 1973 Jun;231(2):297–325. doi: 10.1113/jphysiol.1973.sp010234. [DOI] [PMC free article] [PubMed] [Google Scholar]
- Garrahan P. J., Glynn I. M. The behaviour of the sodium pump in red cells in the absence of external potassium. J Physiol. 1967 Sep;192(1):159–174. doi: 10.1113/jphysiol.1967.sp008294. [DOI] [PMC free article] [PubMed] [Google Scholar]
- Glynn I. M., Hoffman J. F., Lew V. L. Some "partial reactions" of the sodium pump. Philos Trans R Soc Lond B Biol Sci. 1971 Aug 20;262(842):91–102. doi: 10.1098/rstb.1971.0080. [DOI] [PubMed] [Google Scholar]
- Glynn I. M., Hoffman J. F. Nucleotide requirements for sodium-sodium exchange catalysed by the sodium pump in human red cells. J Physiol. 1971 Oct;218(1):239–256. doi: 10.1113/jphysiol.1971.sp009612. [DOI] [PMC free article] [PubMed] [Google Scholar]
- Glynn I. M., Lew V. L., Lüthi U. Reversal of the potassium entry mechanism in red cells, with and without reversal of the entire pump cycle. J Physiol. 1970 Apr;207(2):371–391. doi: 10.1113/jphysiol.1970.sp009067. [DOI] [PMC free article] [PubMed] [Google Scholar]
- Glynn I. M., Lew V. L. Synthesis of adenosine triphosphate at the expense of downhill cation movements in intact human red cells. J Physiol. 1970 Apr;207(2):393–402. doi: 10.1113/jphysiol.1970.sp009068. [DOI] [PMC free article] [PubMed] [Google Scholar]
- Hegyvary C., Post R. L. Binding of adenosine triphosphate to sodium and potassium ion-stimulated adenosine triphosphatase. J Biol Chem. 1971 Sep 10;246(17):5234–5240. [PubMed] [Google Scholar]
- Hexum T., Samson F. E., Jr, Himes R. H. Kinetic studies of membrane (Na+-K+-Mg2+)-ATPase. Biochim Biophys Acta. 1970 Aug 15;212(2):322–331. doi: 10.1016/0005-2744(70)90213-5. [DOI] [PubMed] [Google Scholar]
- Jensen J., Norby J. G. On the specificity of the ATP-binding site of (Na+ + K+)-activated ATPase from brain microsomes. Biochim Biophys Acta. 1971 Apr 13;233(2):395–403. doi: 10.1016/0005-2736(71)90336-1. [DOI] [PubMed] [Google Scholar]
- Kaniike K., Erdmann E., Schoner W. ADP binding to (Na++K+)-activated ATPase. Biochim Biophys Acta. 1973 Apr 16;298(4):901–905. doi: 10.1016/0005-2736(73)90394-5. [DOI] [PubMed] [Google Scholar]
- Lepke S., Passow H. The effect of pH at hemolysis on the reconstitution of low cation permeability in human erythrocyte ghosts. Biochim Biophys Acta. 1972 Feb 11;255(2):696–702. doi: 10.1016/0005-2736(72)90174-5. [DOI] [PubMed] [Google Scholar]
- Lew V. L. Effect of ouabain on the Ca 2+ -dependent increase in K + permeability in depleted guinea-pig red cells. Biochim Biophys Acta. 1971 Oct 12;249(1):236–239. doi: 10.1016/0005-2736(71)90100-3. [DOI] [PubMed] [Google Scholar]
- Neufeld A. H., Levy H. M. The steady state level of phosphorylated intermediate in relation to the two sodium-dependent adenosine triphosphatases of calf brain microsomes. J Biol Chem. 1970 Oct 10;245(19):4962–4967. [PubMed] [Google Scholar]
- Norby J. G., Jensen J. Binding of ATP to brain microsomal ATPase. Determination of the ATP-binding capacity and the dissociation constant of the enzyme-ATP complex as a function of K+ concentration. Biochim Biophys Acta. 1971 Mar 9;233(1):104–116. doi: 10.1016/0005-2736(71)90362-2. [DOI] [PubMed] [Google Scholar]
- POST R. L., JOLLY P. C. The linkage of sodium, potassium, and ammonium active transport across the human erythrocyte membrane. Biochim Biophys Acta. 1957 Jul;25(1):118–128. doi: 10.1016/0006-3002(57)90426-2. [DOI] [PubMed] [Google Scholar]
- POST R. L., MERRITT C. R., KINSOLVING C. R., ALBRIGHT C. D. Membrane adenosine triphosphatase as a participant in the active transport of sodium and potassium in the human erythrocyte. J Biol Chem. 1960 Jun;235:1796–1802. [PubMed] [Google Scholar]
- POST R. L., SEN A. K. AN ENZYMATIC MECHANISM OF ACTIVE SODIUM AND POTASSIUM TRANSPORT. J Histochem Cytochem. 1965 Feb;13:105–112. doi: 10.1177/13.2.105. [DOI] [PubMed] [Google Scholar]
- POST R. L., SEN A. K., ROSENTHAL A. S. A PHOSPHORYLATED INTERMEDIATE IN ADENOSINE TRIPHOSPHATE-DEPENDENT SODIUM AND POTASSIUM TRANSPORT ACROSS KIDNEY MEMBRANES. J Biol Chem. 1965 Mar;240:1437–1445. [PubMed] [Google Scholar]
- Post R. L., Hegyvary C., Kume S. Activation by adenosine triphosphate in the phosphorylation kinetics of sodium and potassium ion transport adenosine triphosphatase. J Biol Chem. 1972 Oct 25;247(20):6530–6540. [PubMed] [Google Scholar]
- Robinson J. D. Kinetic studies on a brain microsomal adenosine triphosphatase. Evidence suggesting conformational changes. Biochemistry. 1967 Oct;6(10):3250–3258. doi: 10.1021/bi00862a034. [DOI] [PubMed] [Google Scholar]
- Sachs J. R. Recoupling the Na-K pump. J Clin Invest. 1972 Dec;51(12):3244–3247. doi: 10.1172/JCI107151. [DOI] [PMC free article] [PubMed] [Google Scholar]
- Sachs J. R., Welt L. G. The concentration dependence of active potassium transport in the human red blood cell. J Clin Invest. 1967 Jan;46(1):65–76. doi: 10.1172/JCI105512. [DOI] [PMC free article] [PubMed] [Google Scholar]
- Sen A. K., Tobin T. A cycle for ouabain inhibition of sodium- and potassium-dependent adenosine triphosphatase. J Biol Chem. 1969 Dec 25;244(24):6596–6604. [PubMed] [Google Scholar]
- Simons T. J. Potassium efflux associated with partial reversal of the sodium pump in human red cell ghosts. J Physiol. 1972 Dec;227(2):19P–20P. [PubMed] [Google Scholar]
- WEIL-MALHERBE H., GREEN R. H. The catalytic effect of molybdate on the hydrolysis of organic phosphate bonds. Biochem J. 1951 Aug;49(3):286–292. [PMC free article] [PubMed] [Google Scholar]