Abstract
1. Human red cells treated with lactose solution and loaded with NaCl and BCl subsequently exchange cation with a nutrient BCl medium. B is the same in cells and medium, and is either K, Rb, Cs or Li. In these circumstances Na always moves outwards with the concentration gradient, but the efflux is largely active.
2. With suspensions in media containing Ca2+, the total Na efflux depends on the amount of Na in the cells and on the nature of cation B. Thus for any given value of mean cell Na (Nam) in excess of 30 m-equiv/l. cells, the effect of B on the amount of Na efflux is K > Cs > Rb > Li, while with Nam between 0·7 and 5 m-equiv/l. cells, the sequence is Cs > Li > K, Rb. With Nam between 5 and 30 m-equiv/l. cells, intermediate sequences may be demonstrated for the effects of B on Na efflux. This applies both to the efflux itself and to the flux: concentration ratio, FCR.
3. FCR for passive Na efflux in these circumstances is determined by adding strophanthin G to the medium. It varies inversely with the duration of exposure to Ca2+ in the exchange and nutrient media, but not with the nature of cation B. FCR for passive efflux is probably little affected by the value of Nam.
4. By deducting passive from total Na efflux, active Na efflux is obtained, and variations in the latter with cell Na content, and with B, result in FCR curves similar to those obtained with total Na efflux.
5. Total and passive Na efflux have also been measured in Ca-free media. Here the passive efflux is considerable, and with Na + K cells in KCl media FCR increases with Nam, but in other systems this change is not significant. However, the rate of passive efflux into LiCl media is less than that for KCl or CsCl media. Owing to the magnitude of passive flux in Ca-free systems, the total Na efflux is also increased, but FCR for active Na efflux is quantitatively and qualitatively similar to that occurring in systems containing Ca2+.
6. The effects of B and Nam on Na efflux give a series of sequences for B which recall some of those obtainable when chemically modified glass membranes separate solutions of salts, and which are attributable to the charge on the membrane and the hydration of the cations involved. However, certain sequences obtained with red cells do not occur with glass membranes. This difficulty is resolved if it be assumed that throughout the range of Nam (0-80 m-equiv/l. cells) active B influx at the external cell face modifies linked Na efflux according to the series K > Rb, Cs > Li, while with Nam between 0 and 30 m-equiv/l. cells (and high complementary B), cations escaping passively compete with active efflux of Na inhibiting the latter according to the series K, Rb > Li > Cs. Both these series could theoretically be explained in terms of surface charges and hydration of cations.
7. Li-loaded cells in nutrient KCl or other media failed to show active Li efflux.
Full text
PDF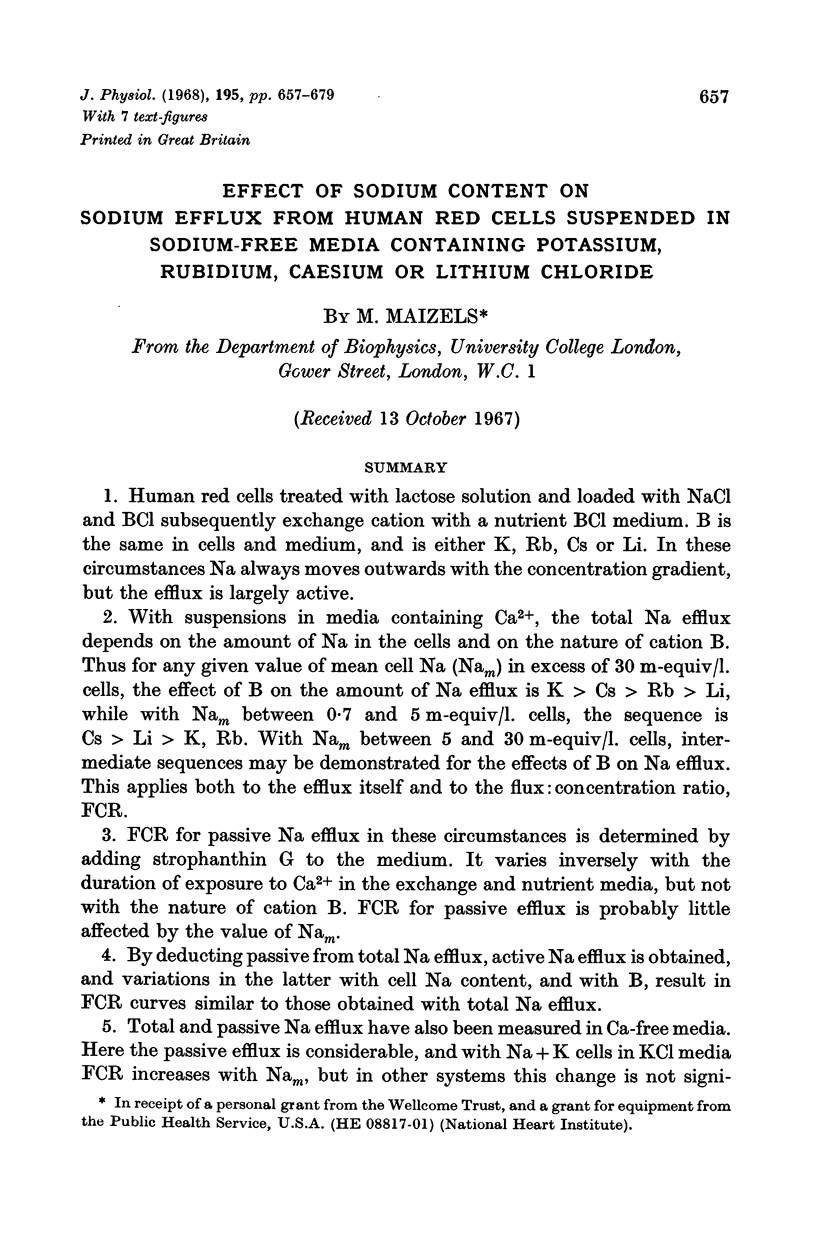
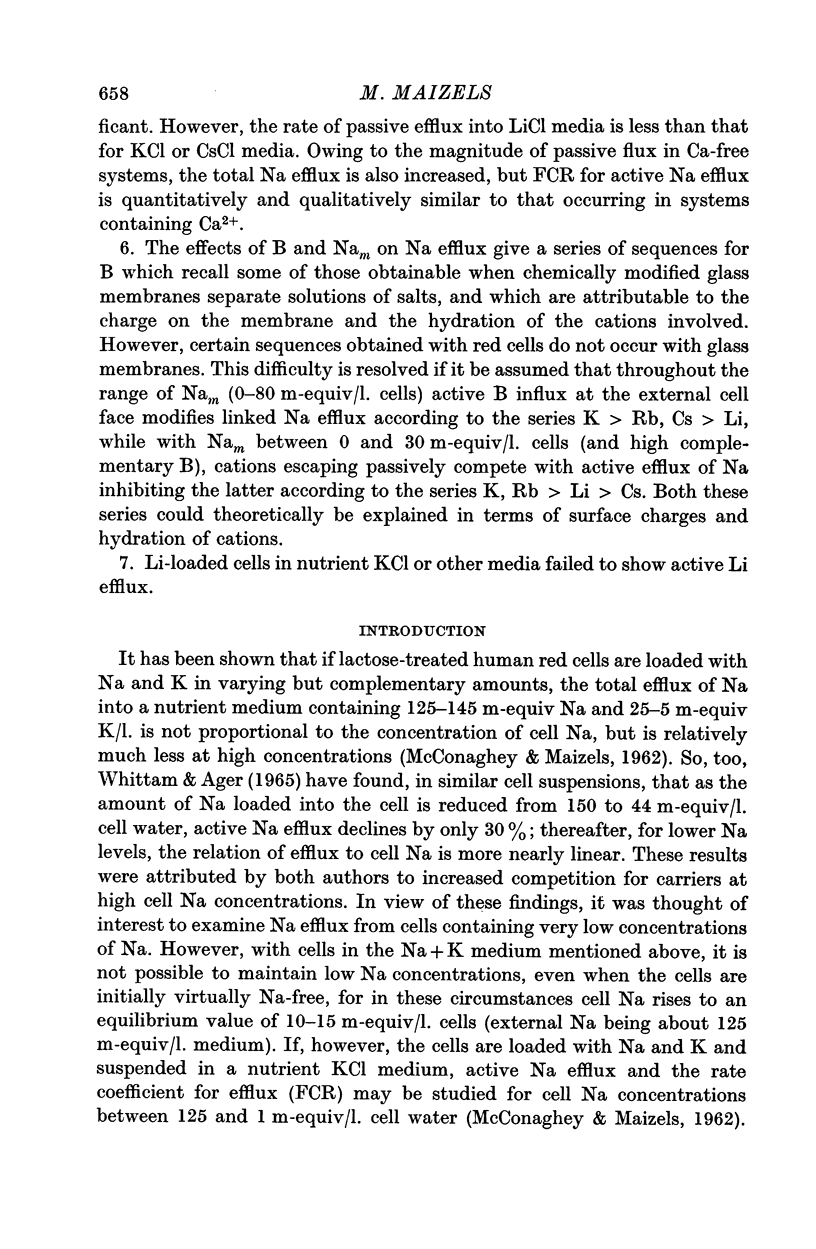
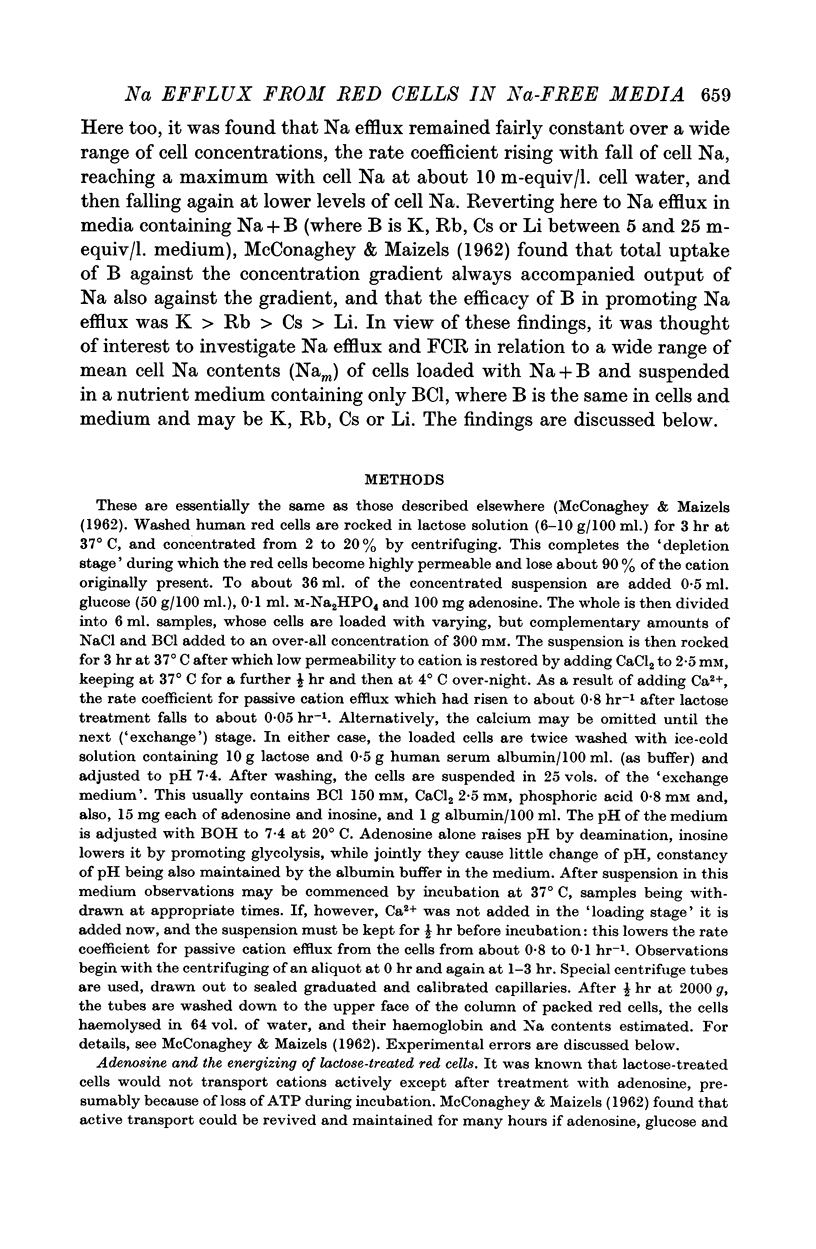
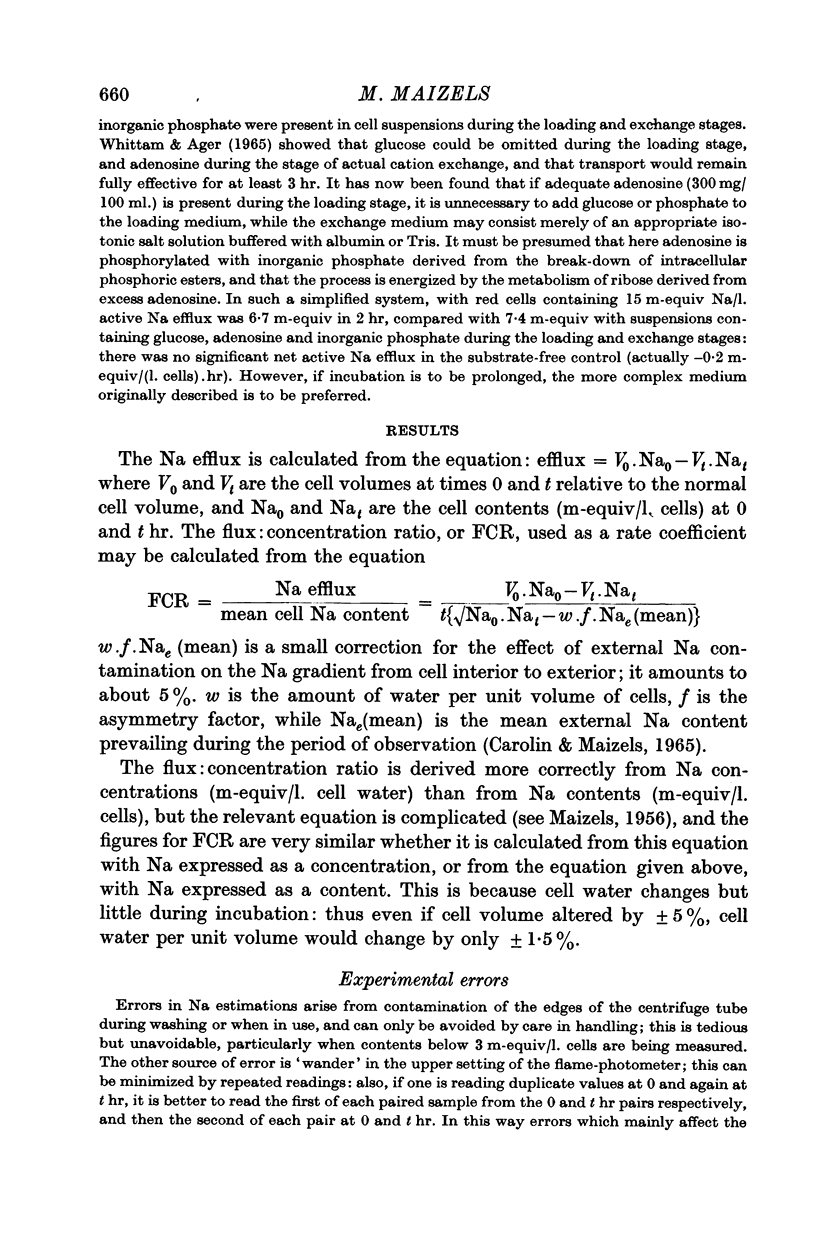
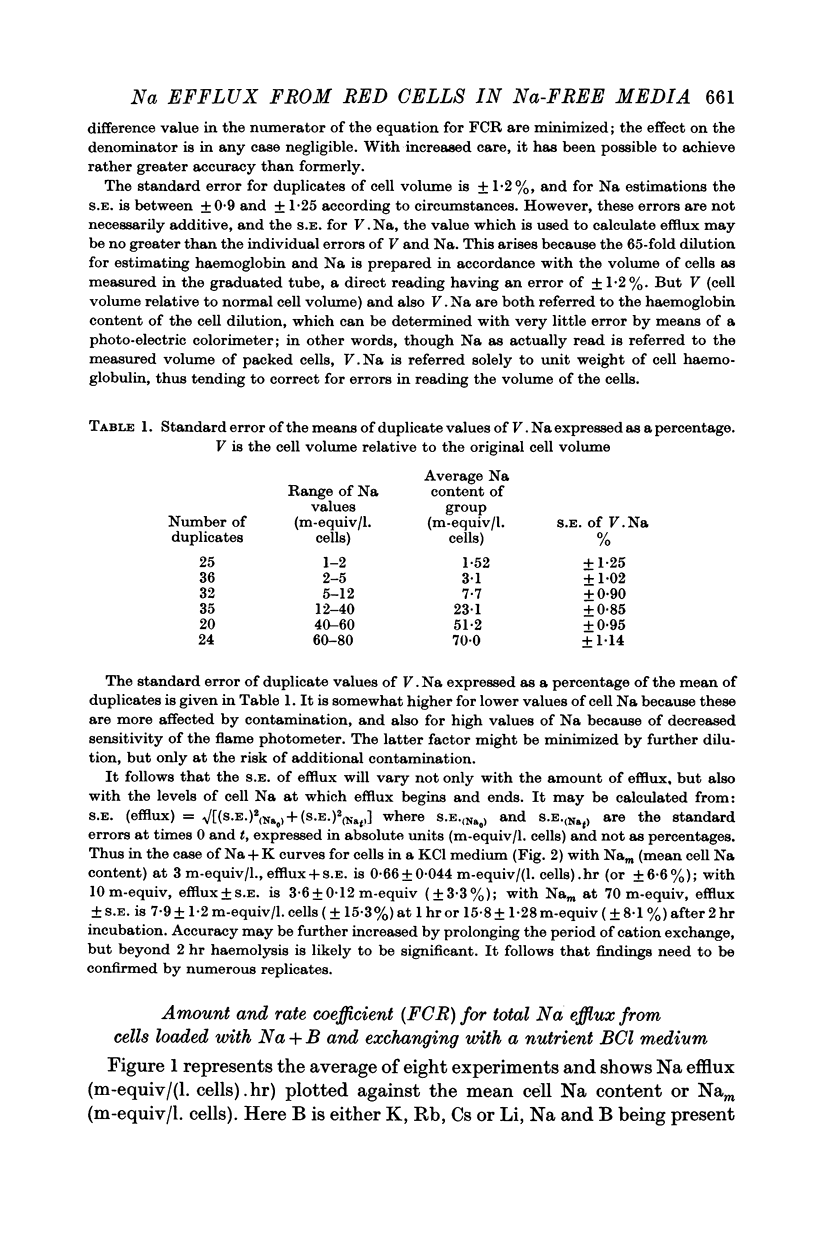
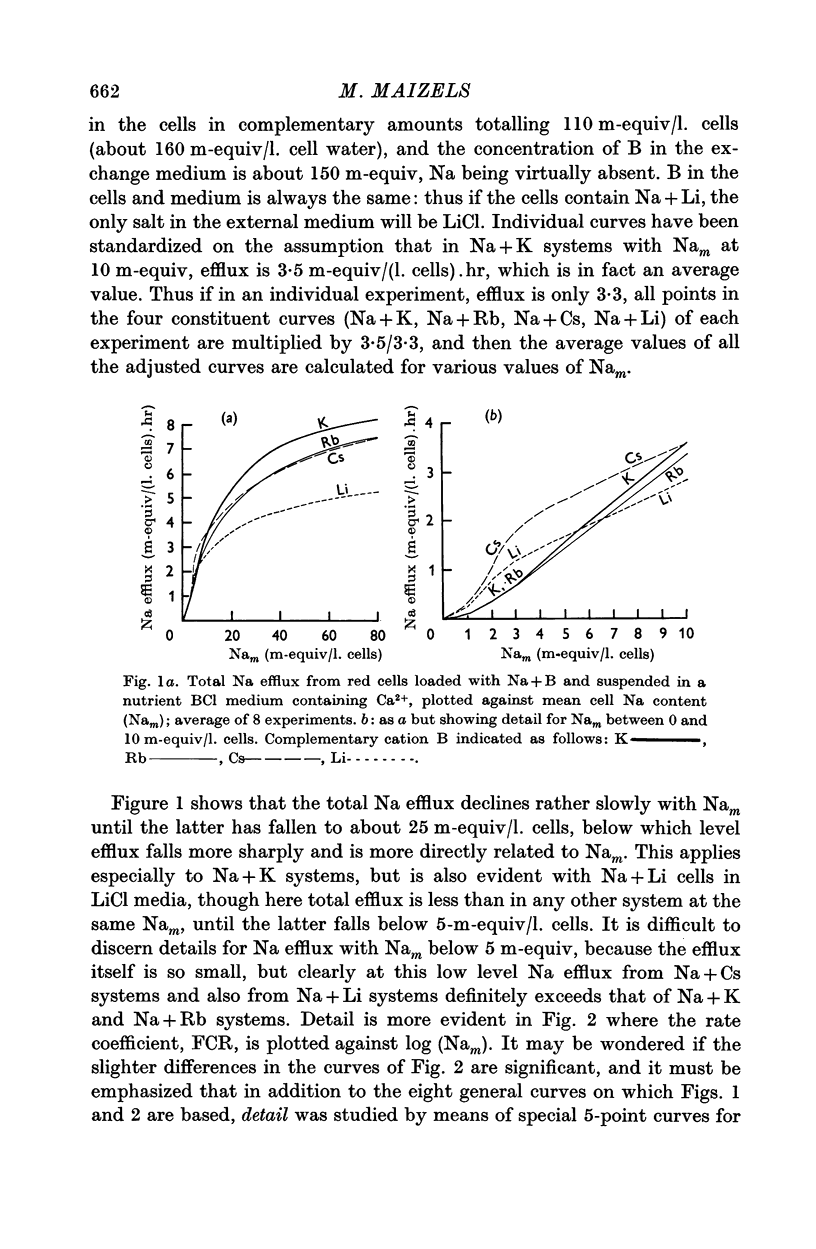
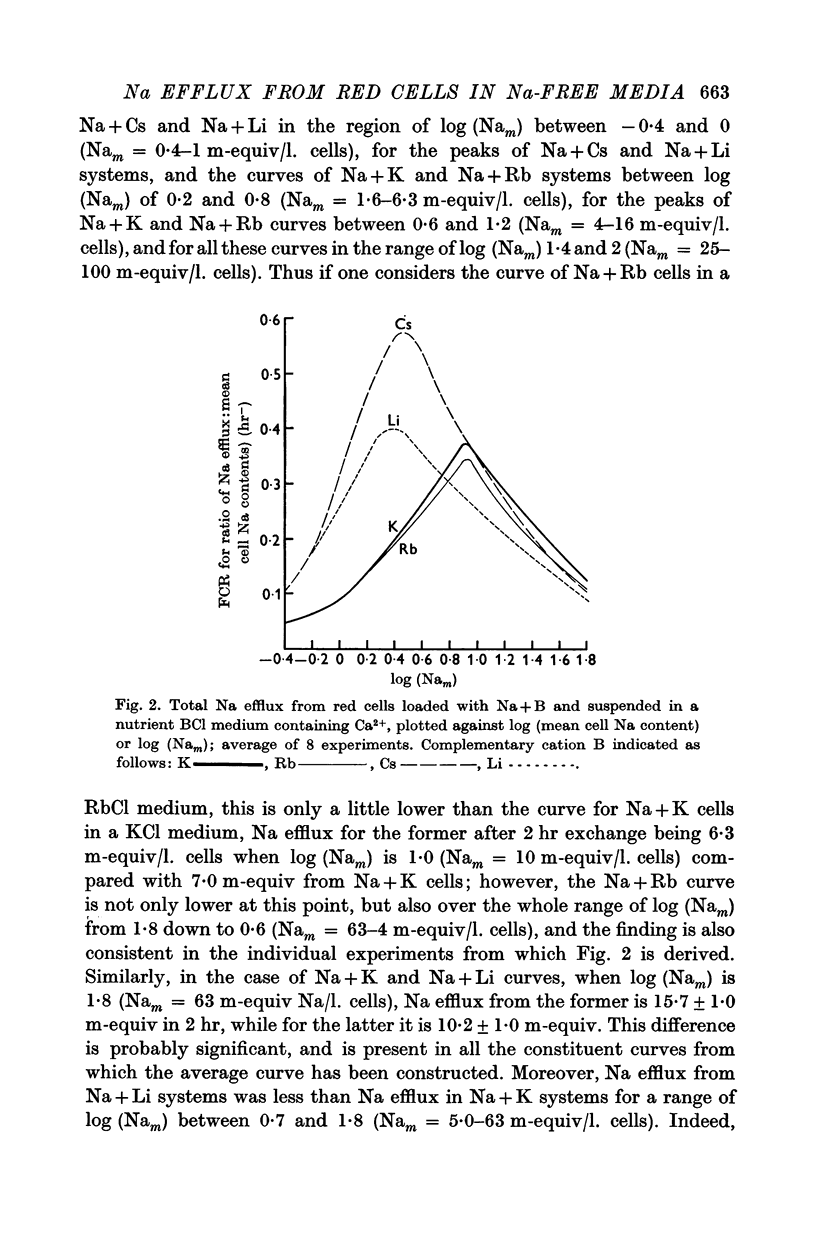
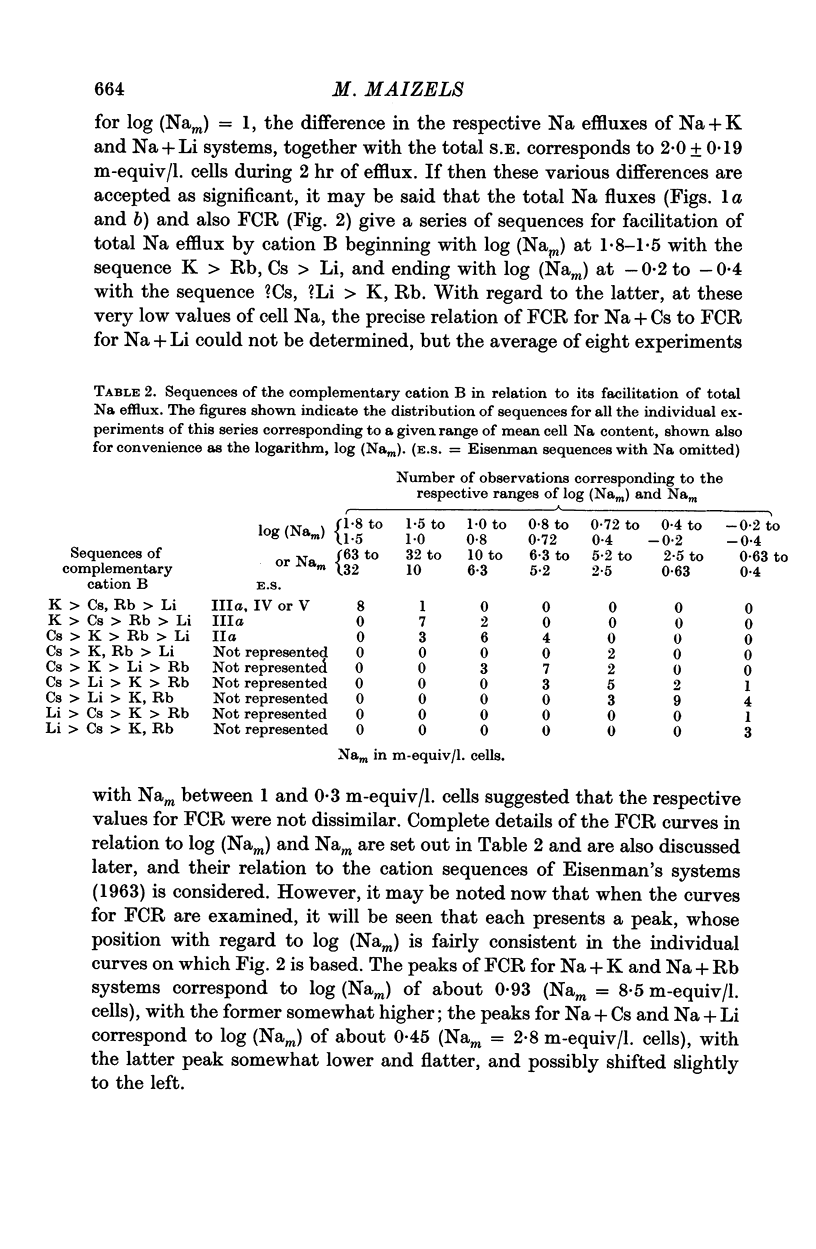
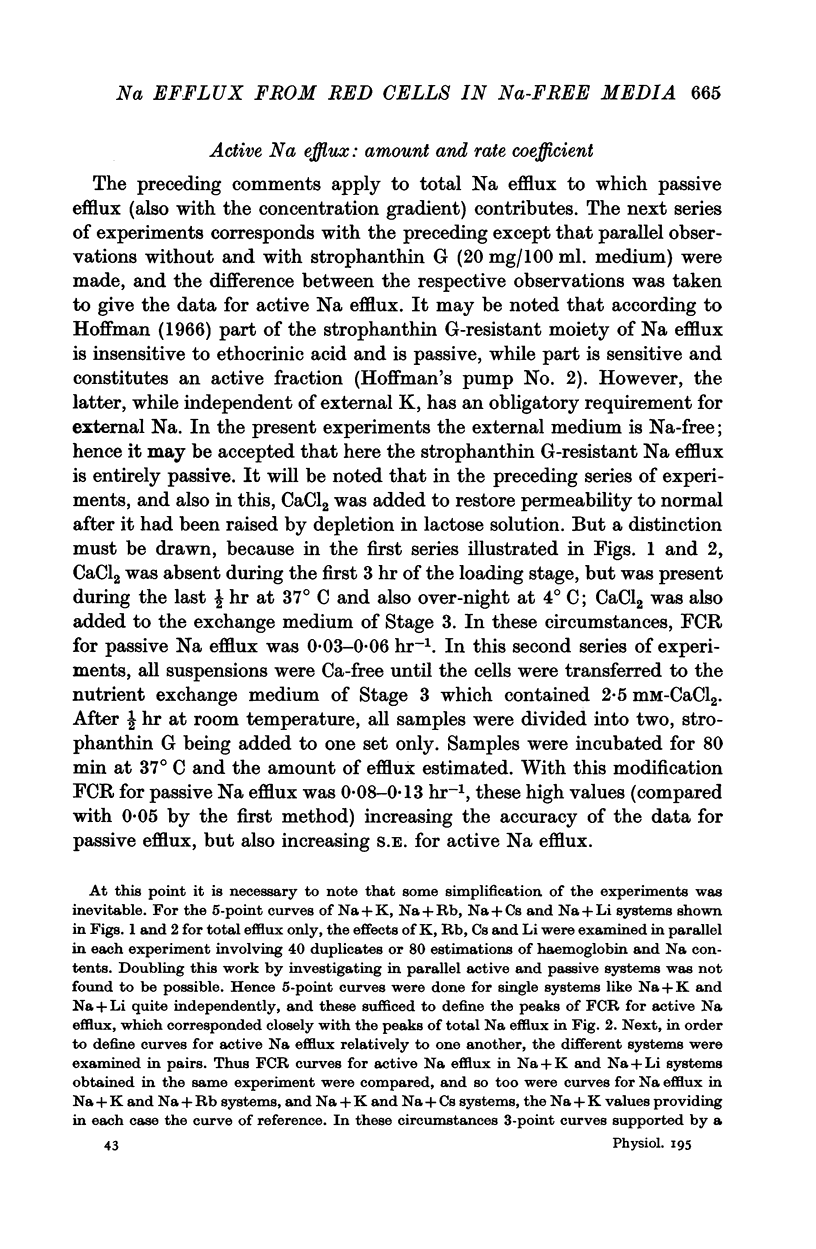
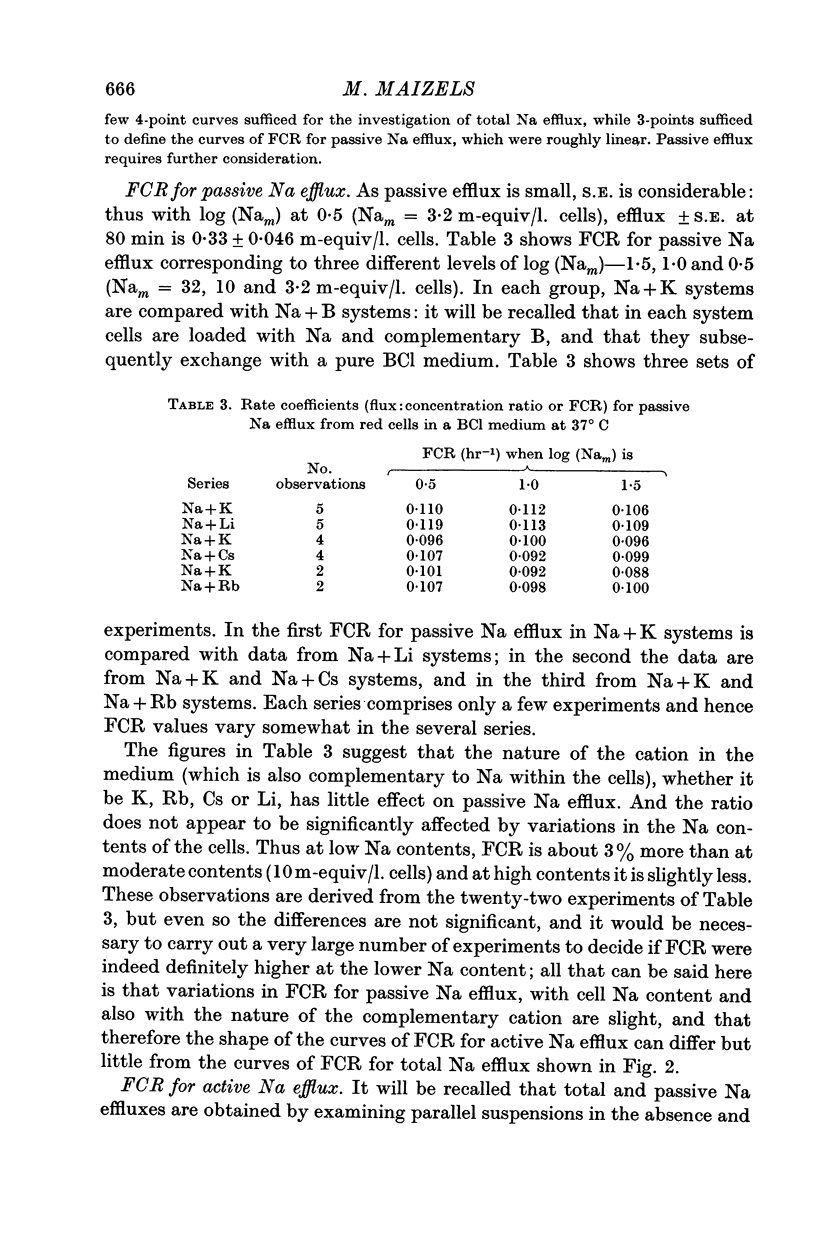
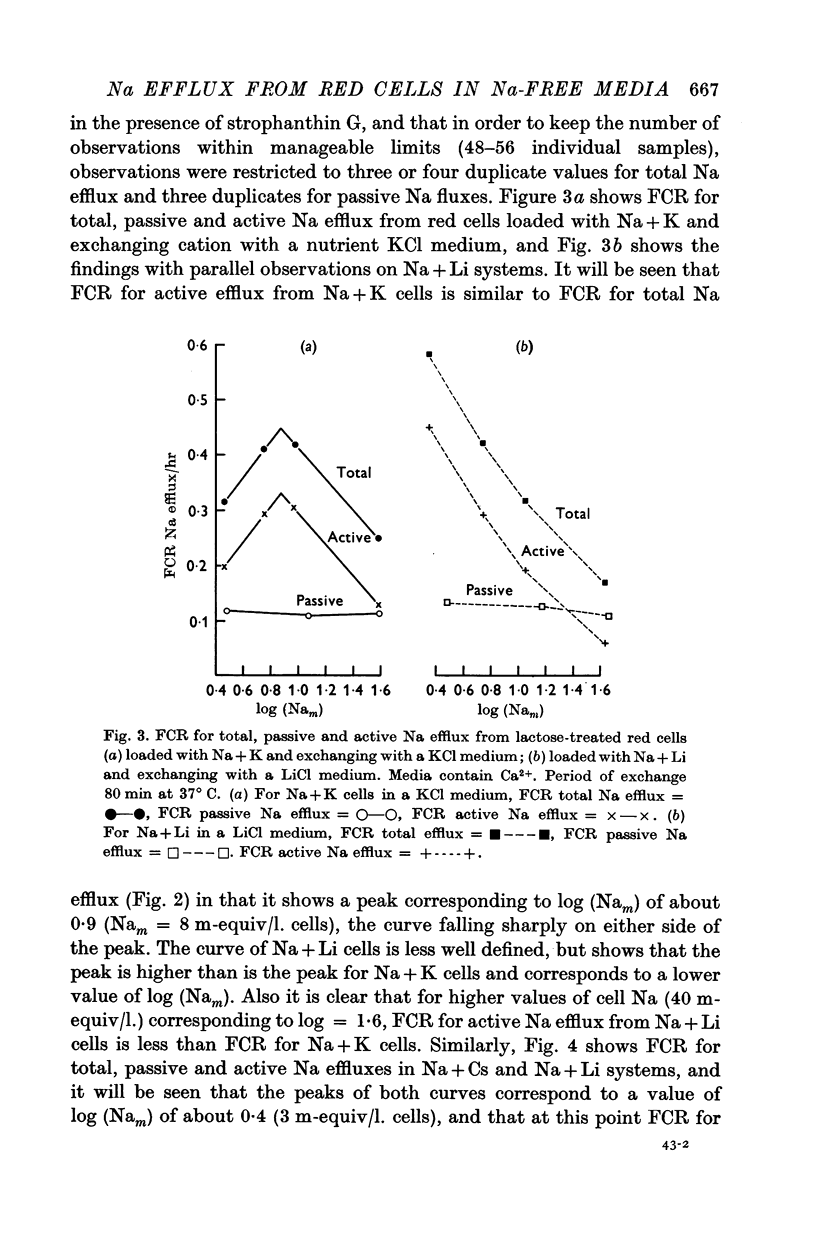
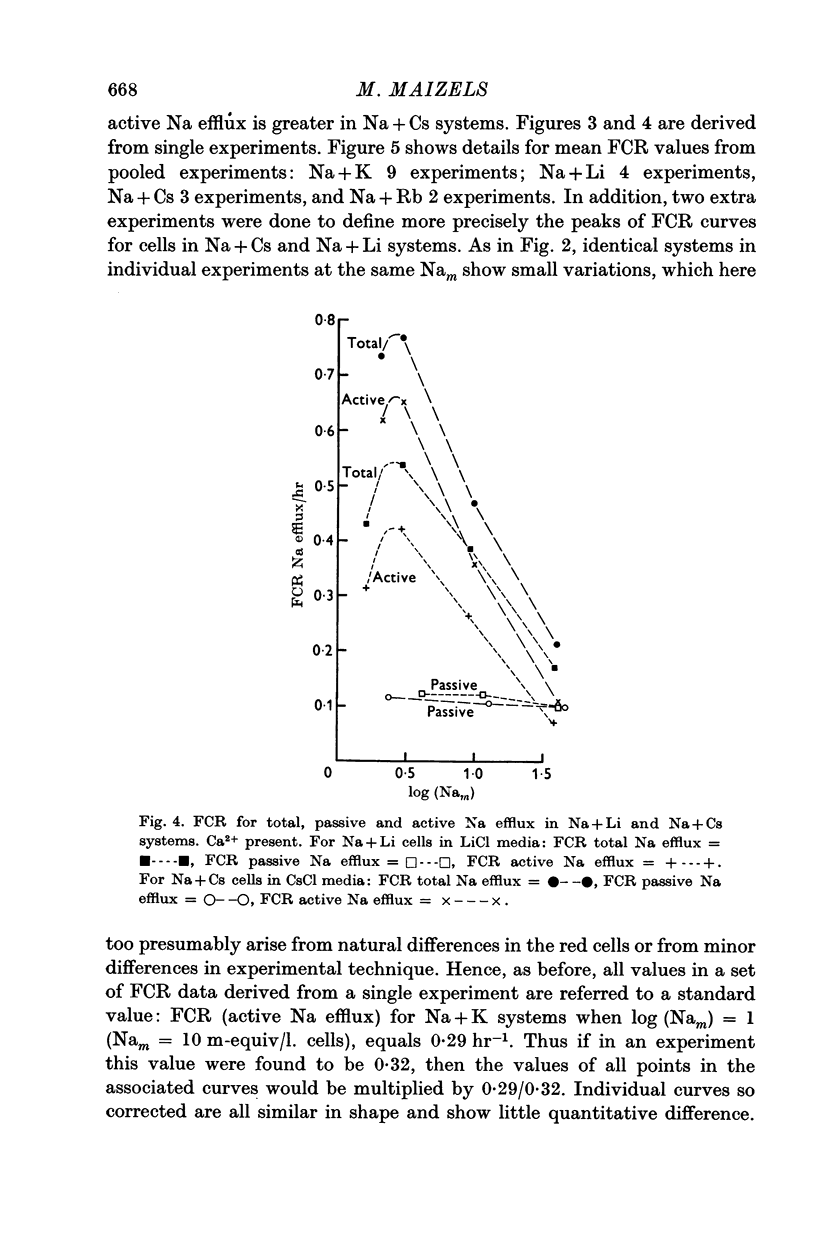
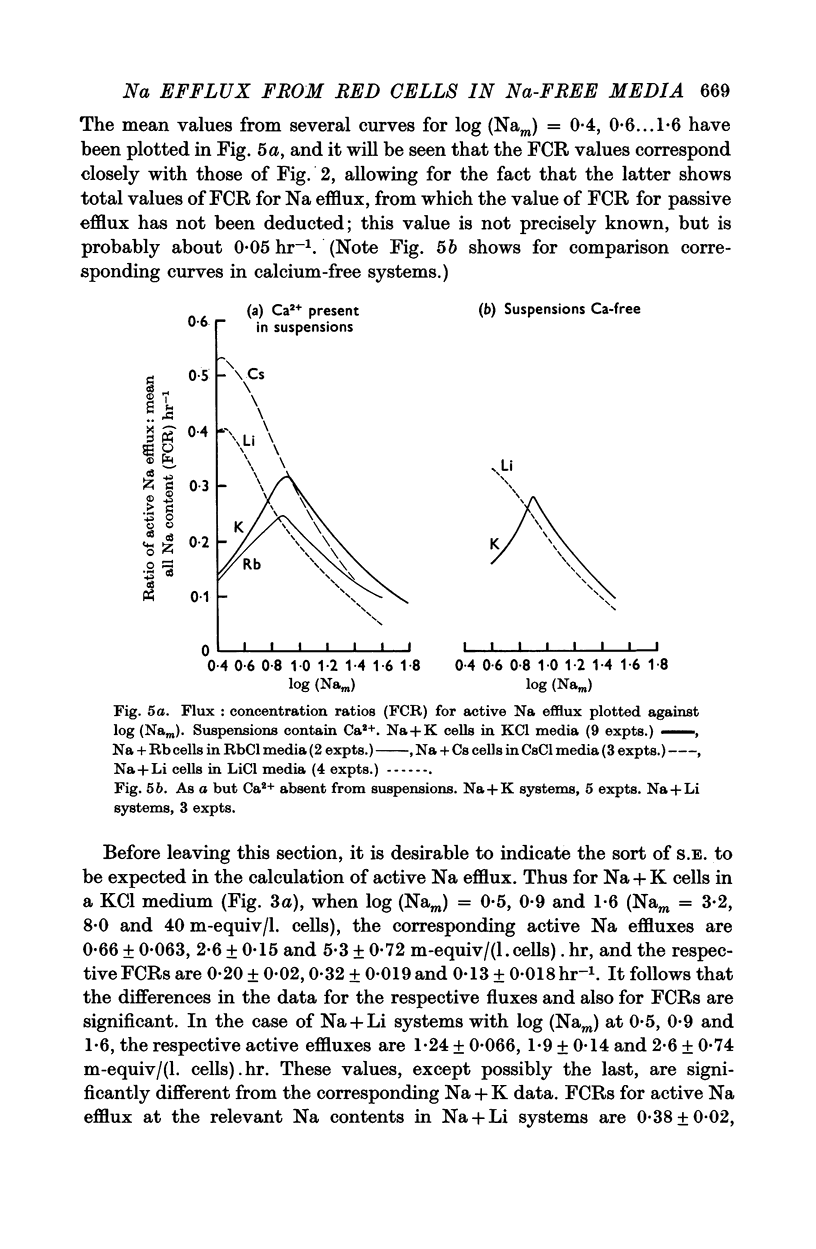
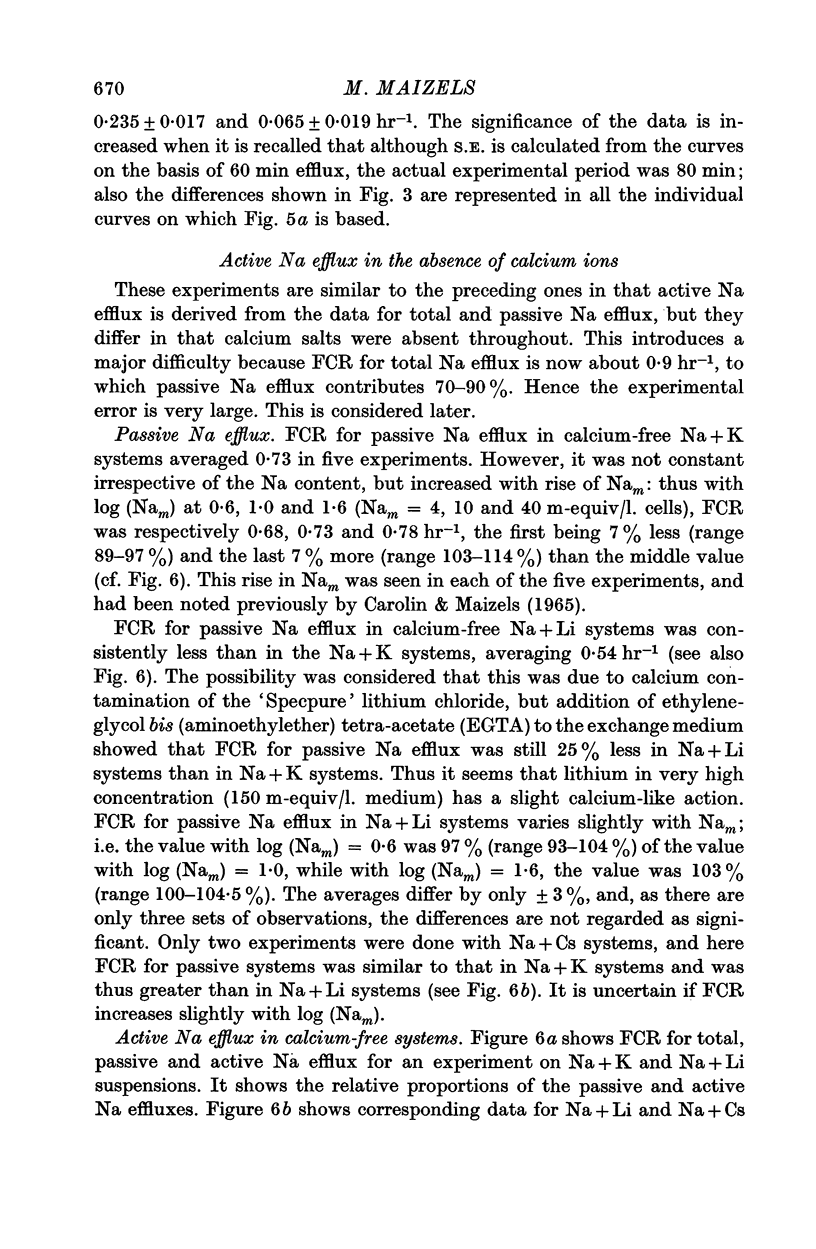
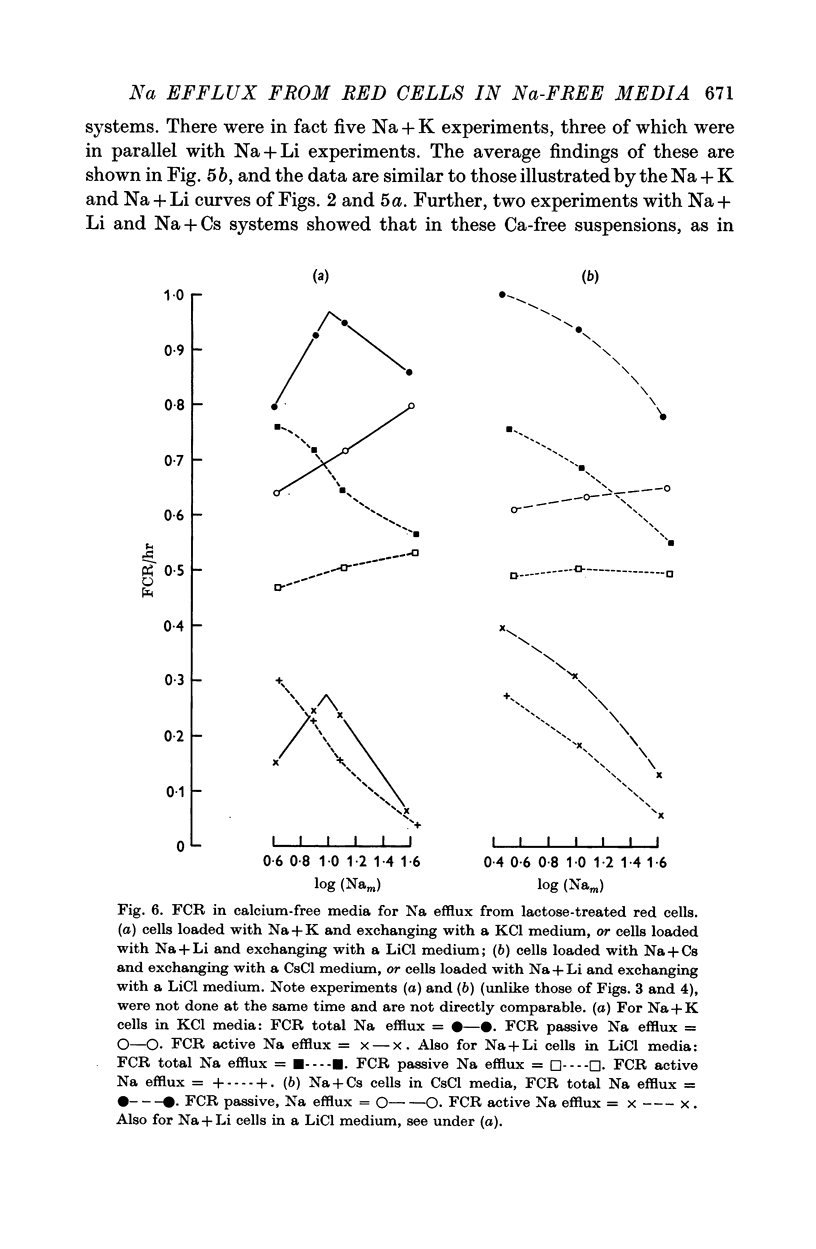
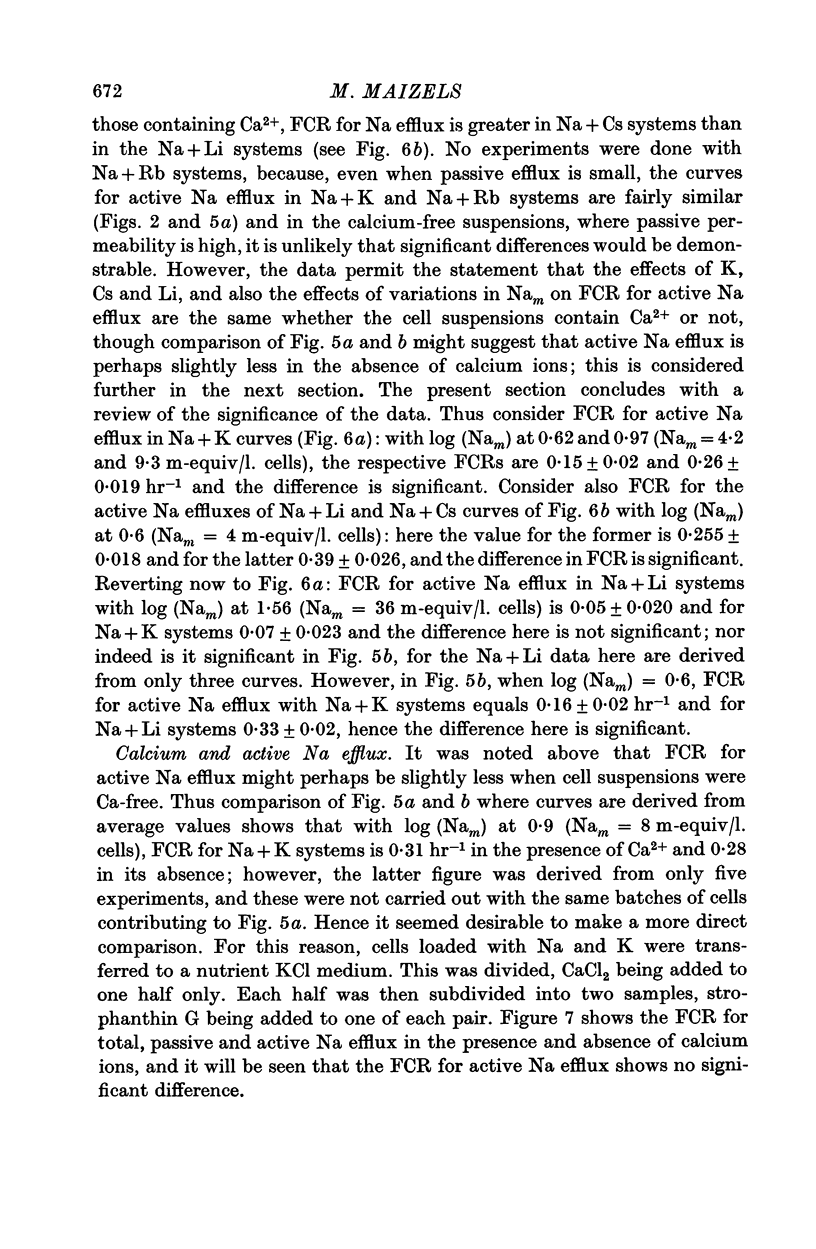
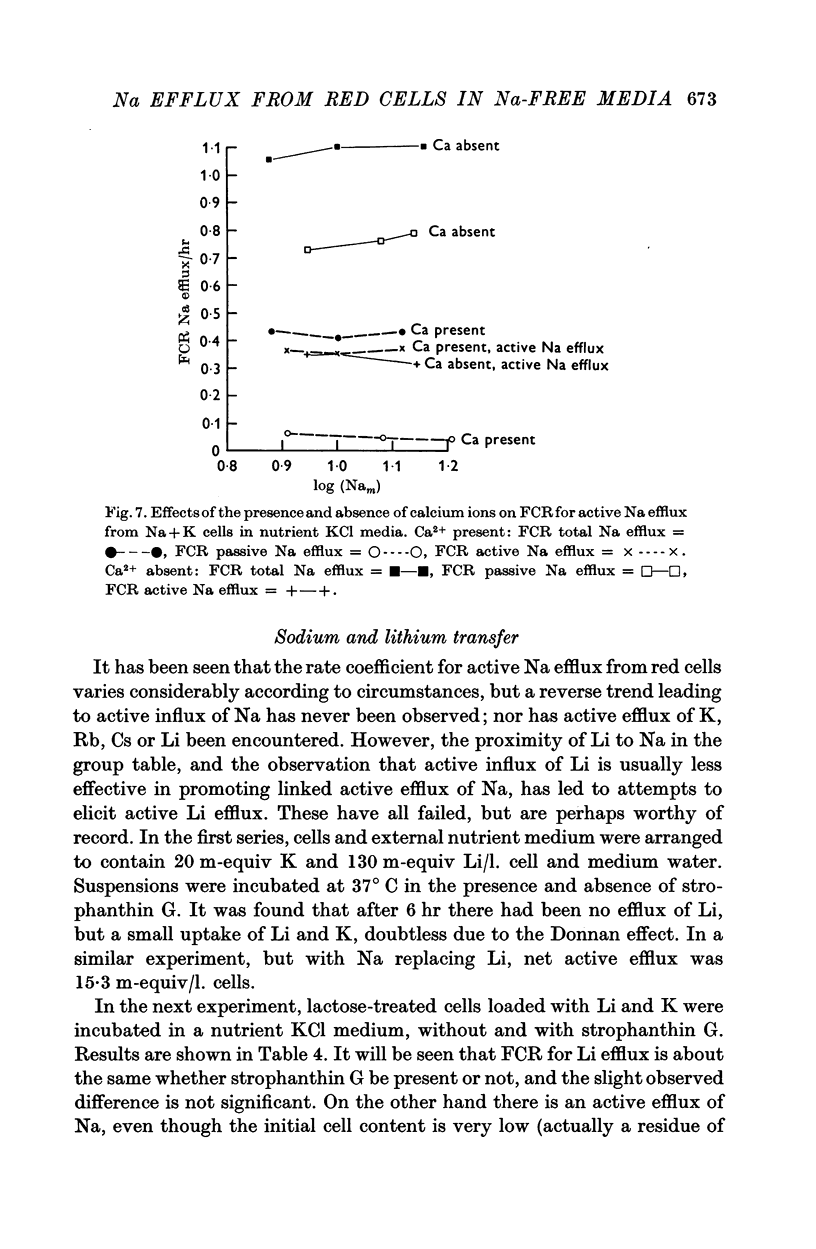
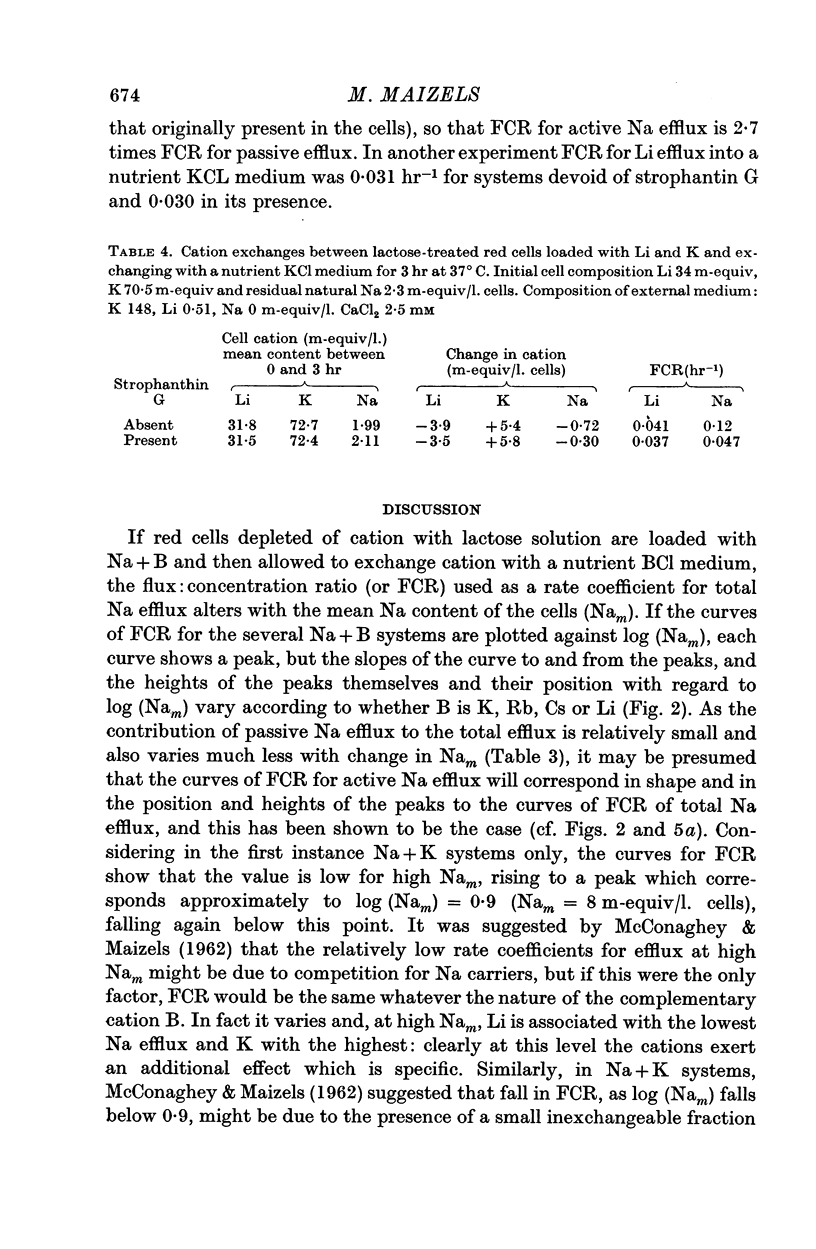
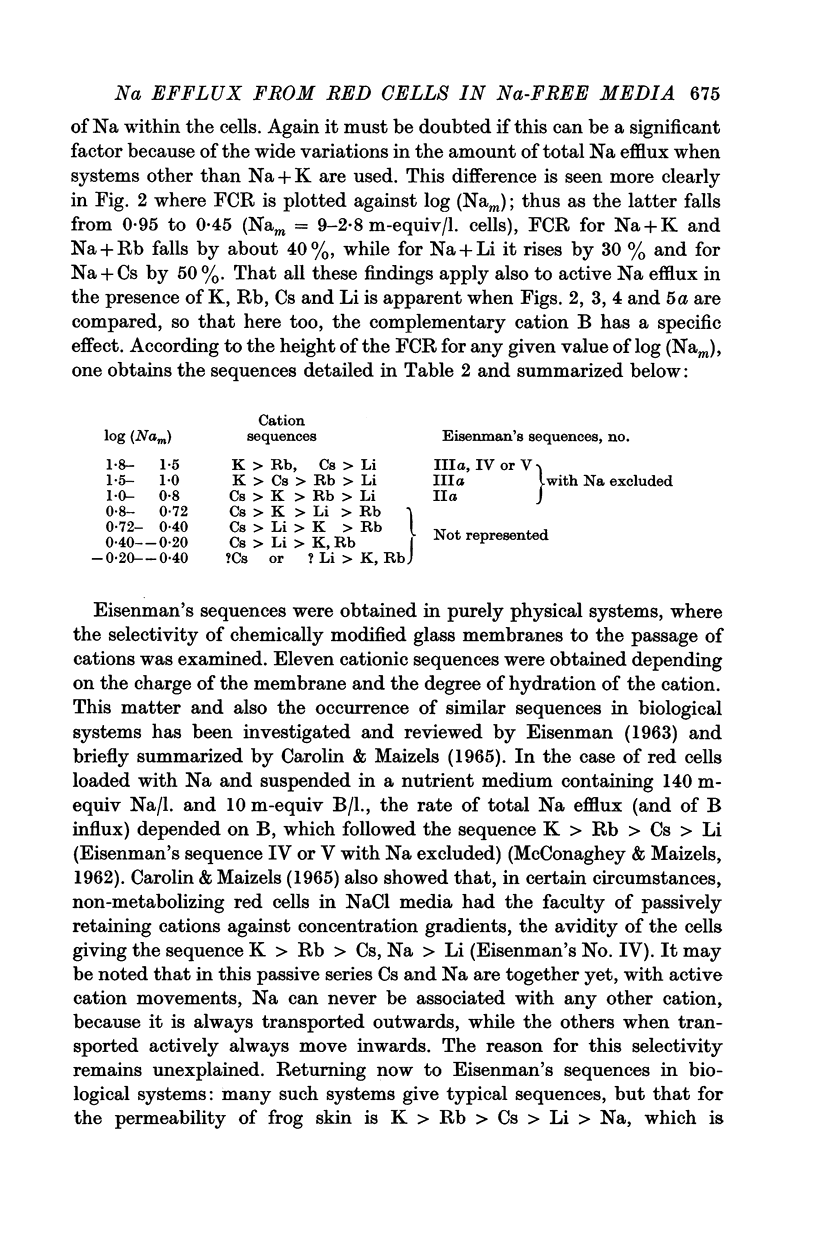
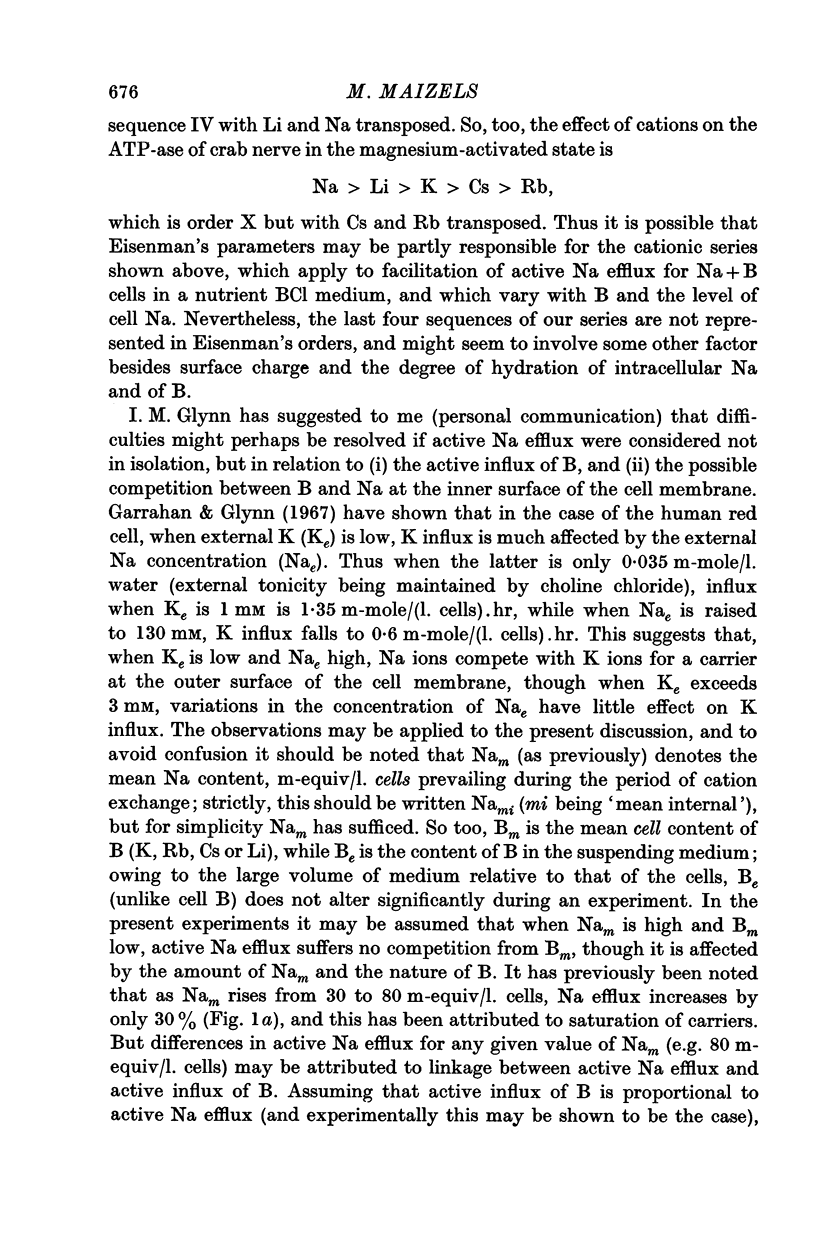
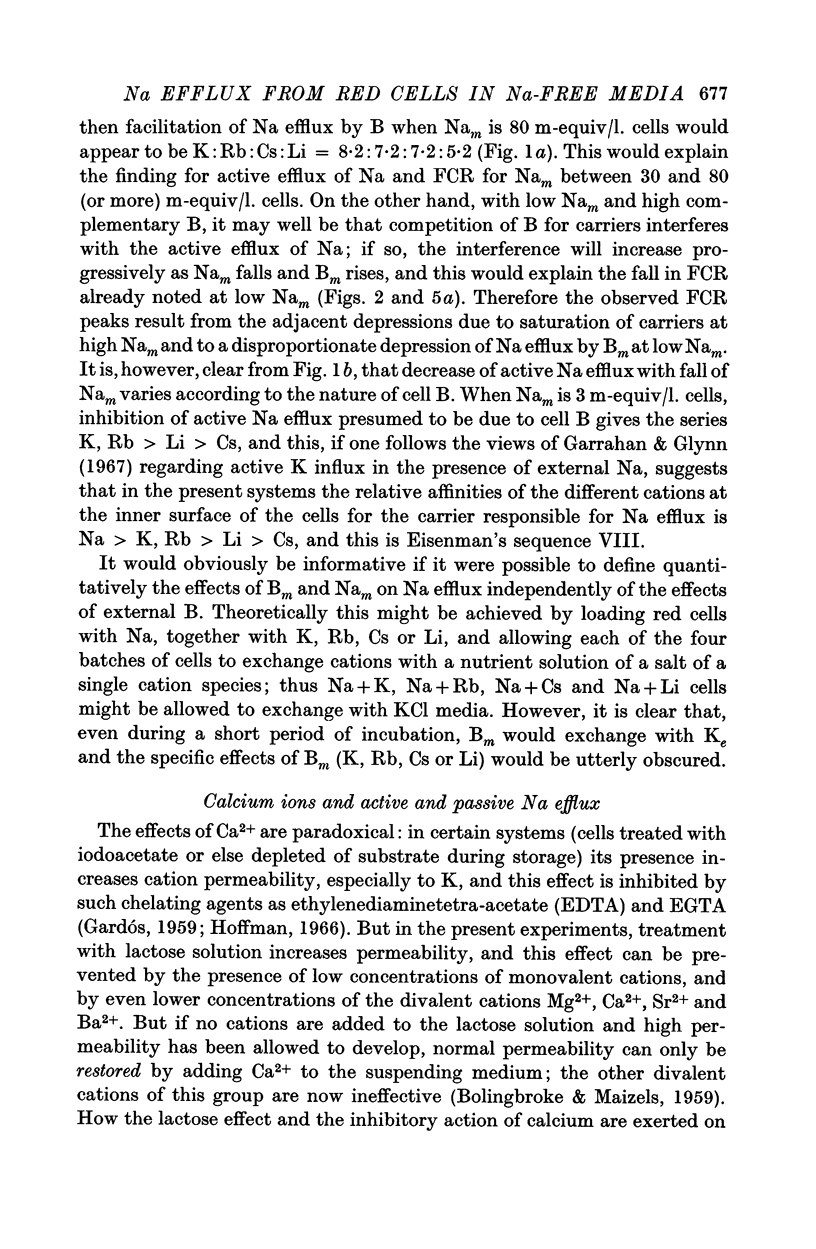
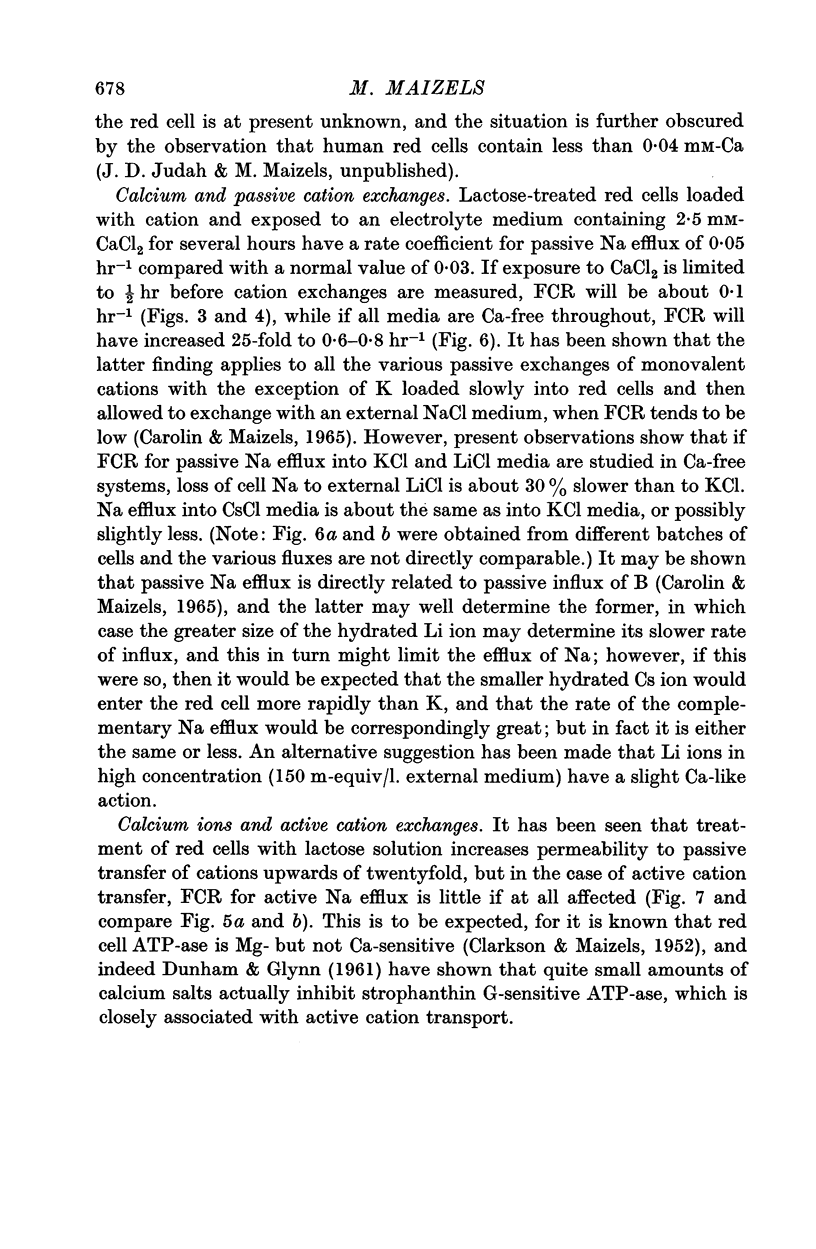
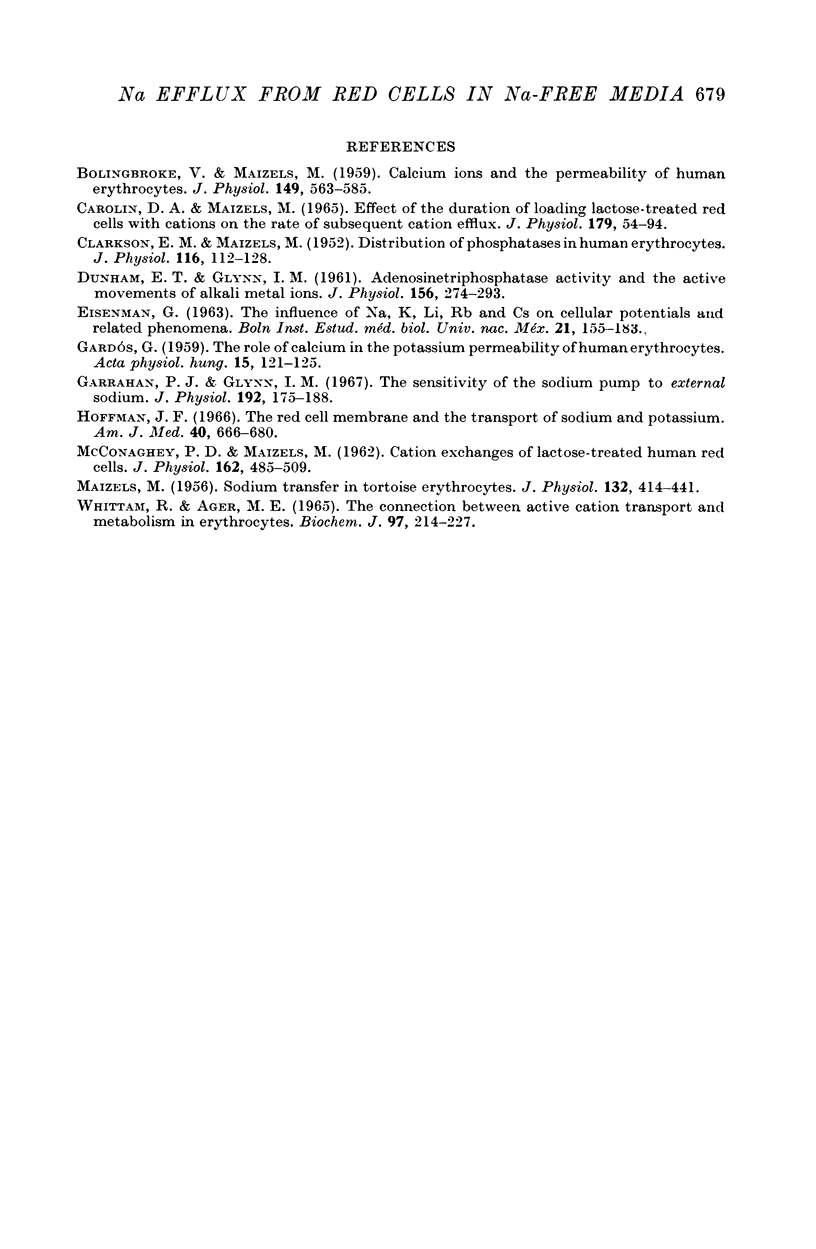
Selected References
These references are in PubMed. This may not be the complete list of references from this article.
- BOLINGBROKE V., MAIZELS M. Calcium ions and the permeability of human erythrocytes. J Physiol. 1959 Dec;149:563–585. doi: 10.1113/jphysiol.1959.sp006361. [DOI] [PMC free article] [PubMed] [Google Scholar]
- CLARKSON E. M., MAIZELS M. Distribution of phosphatases in human erythrocytes. J Physiol. 1952 Jan 28;116(1):112–128. doi: 10.1113/jphysiol.1952.sp004693. [DOI] [PMC free article] [PubMed] [Google Scholar]
- Carolin D. A., Maizels M. Effect of the duration of loading lactose-treated red cells with cations on the rate of subsequent cation efflux. J Physiol. 1965 Jul;179(1):54–94. doi: 10.1113/jphysiol.1965.sp007649. [DOI] [PMC free article] [PubMed] [Google Scholar]
- DUNHAM E. T., GLYNN I. M. Adenosinetriphosphatase activity and the active movements of alkali metal ions. J Physiol. 1961 Apr;156:274–293. doi: 10.1113/jphysiol.1961.sp006675. [DOI] [PMC free article] [PubMed] [Google Scholar]
- Garrahan P. J., Glynn I. M. The sensitivity of the sodium pump to external sodium. J Physiol. 1967 Sep;192(1):175–188. doi: 10.1113/jphysiol.1967.sp008295. [DOI] [PMC free article] [PubMed] [Google Scholar]
- Hoffman J. F. The red cell membrane and the transport of sodium and potassium. Am J Med. 1966 Nov;41(5):666–680. doi: 10.1016/0002-9343(66)90029-5. [DOI] [PubMed] [Google Scholar]
- MAIZELS M. Sodium transfer in tortoise erythrocytes. J Physiol. 1956 May 28;132(2):414–441. doi: 10.1113/jphysiol.1956.sp005535. [DOI] [PMC free article] [PubMed] [Google Scholar]
- McConaghey P. D., Maizels M. Cation exchanges of lactose-treated human red cells. J Physiol. 1962 Aug;162(3):485–509. doi: 10.1113/jphysiol.1962.sp006946. [DOI] [PMC free article] [PubMed] [Google Scholar]
- Whittam R., Ager M. E. The connexion between active cation transport and metabolism in erythrocytes. Biochem J. 1965 Oct;97(1):214–227. doi: 10.1042/bj0970214. [DOI] [PMC free article] [PubMed] [Google Scholar]