Abstract
1. Experiments were performed to determine the relative contribution of the blood plasma and of the cerebrospinal fluid (c.s.f.) to the ionic environment of neurones and glial cells within the brain of the amphibian Necturus maculosus.
2. The concentrations in the blood plasma of untreated control animals were 99 ± 2 mM for Na+ and 2·0 ± 0·1 mM for K+. In the c.s.f. the corresponding values were 112 ± 2 mM-Na+ and 1·9 ± 0·1 mM-K+.
3. By keeping animals in K+-rich water it was possible to raise chronically the concentrations of K+ in the blood plasma up to almost 5 times the normal value, close to 9 mM, while the c.s.f. concentration of K+ was only doubled, to about 4 mM. This behaviour of Necturus, tending to keep the K+ in the c.s.f. low, resembles that of mammals.
4. The membrane potential of glial cells in the optic nerve can be used as an accurate indicator for determining the K+ concentration in the intercellular spaces. Such determinations were made in vivo, and it was shown that the glial cells adjust their membrane potential to the changes of K+ concentrations in the c.s.f. and not to those of the blood plasma. In contrast, the membrane potential of skeletal muscle fibres changes according to the K+ concentration in the blood plasma.
5. It is concluded that the cells within the optic nerve are surrounded by an ionic environment which corresponds to that of the c.s.f. and not to that of the blood plasma. The intercellular spaces are open and ions diffuse freely into them from the c.s.f. A homeostatic mechanism operates, keeping the ion concentrations around neurones and glia within a narrow range and relatively independent of large changes in the blood plasma. This may provide relative stability for the signalling system. Similarities between the optic nerve and other parts of the central nervous system in respect to their relation to c.s.f. and blood are discussed. It seems likely that the mechanisms which control the electrolyte concentrations are similar in Necturus and in mammals.
Full text
PDF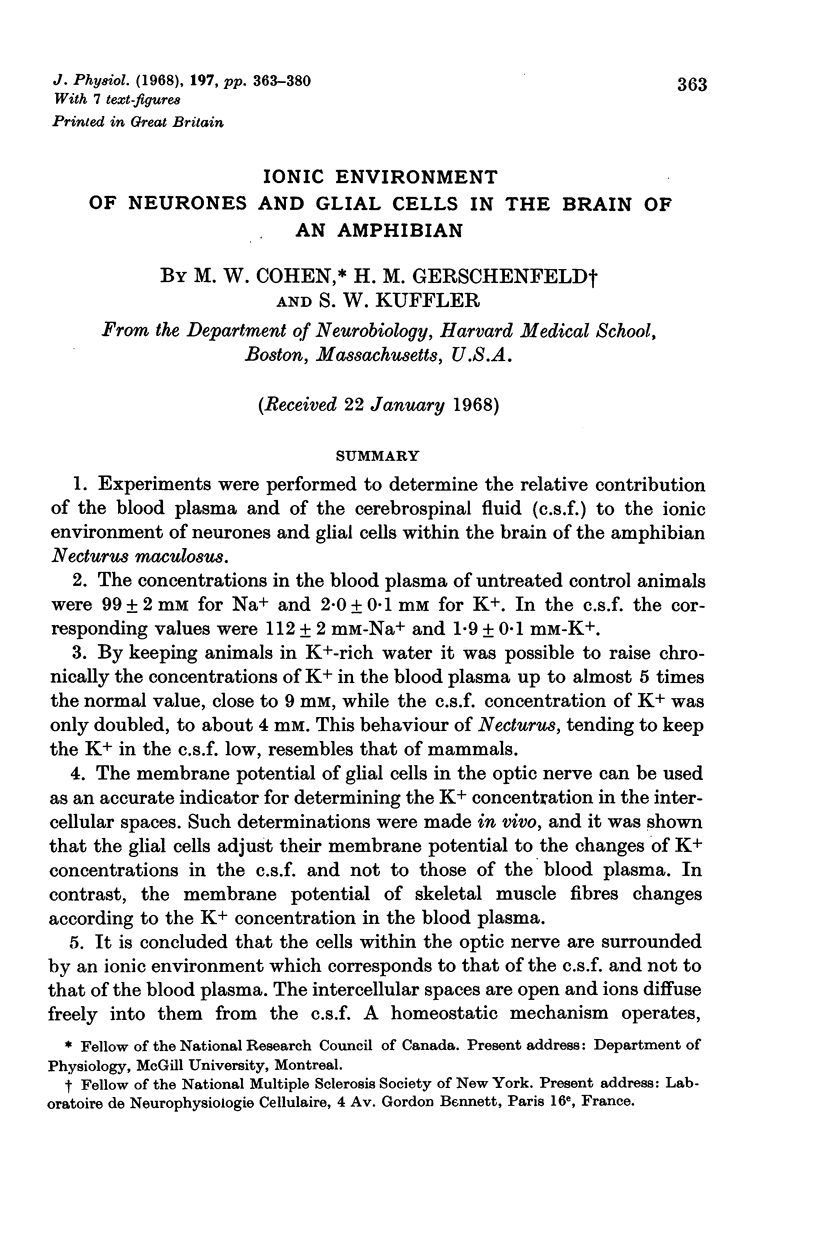
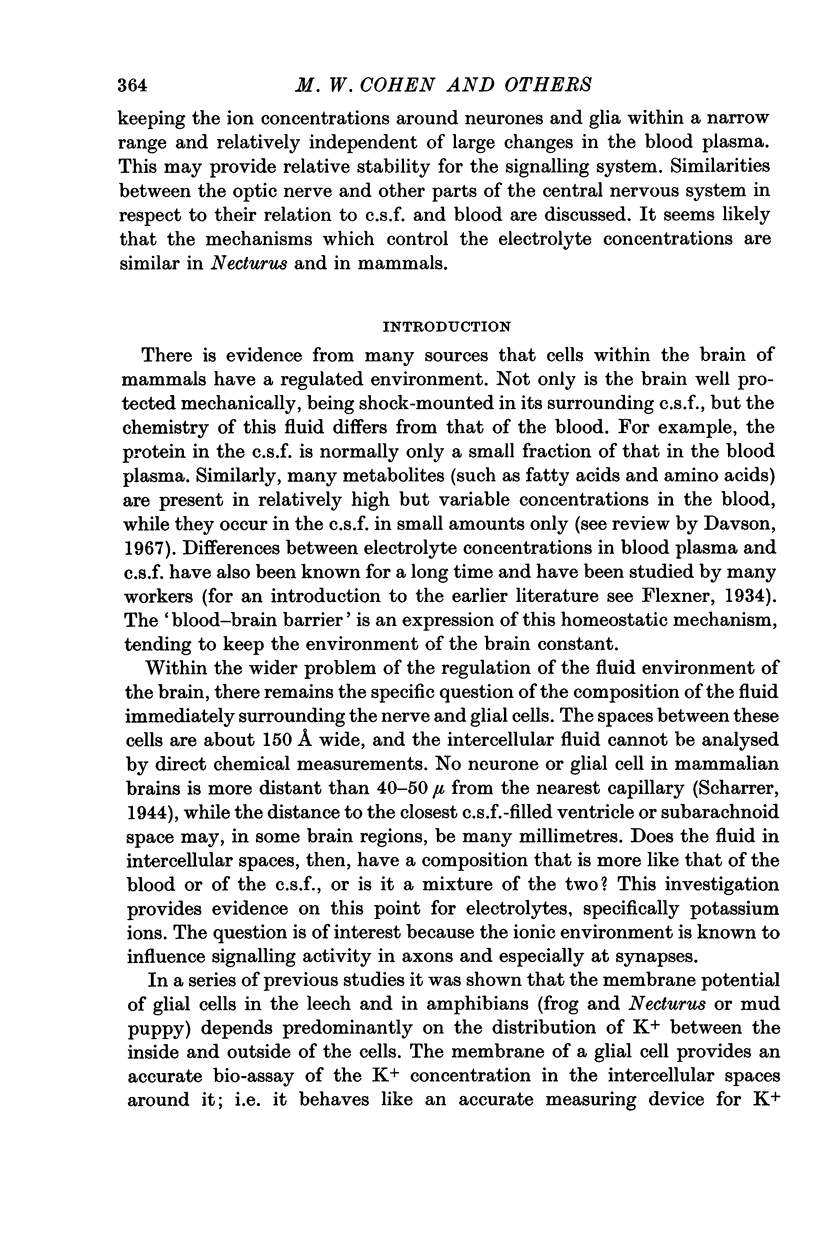
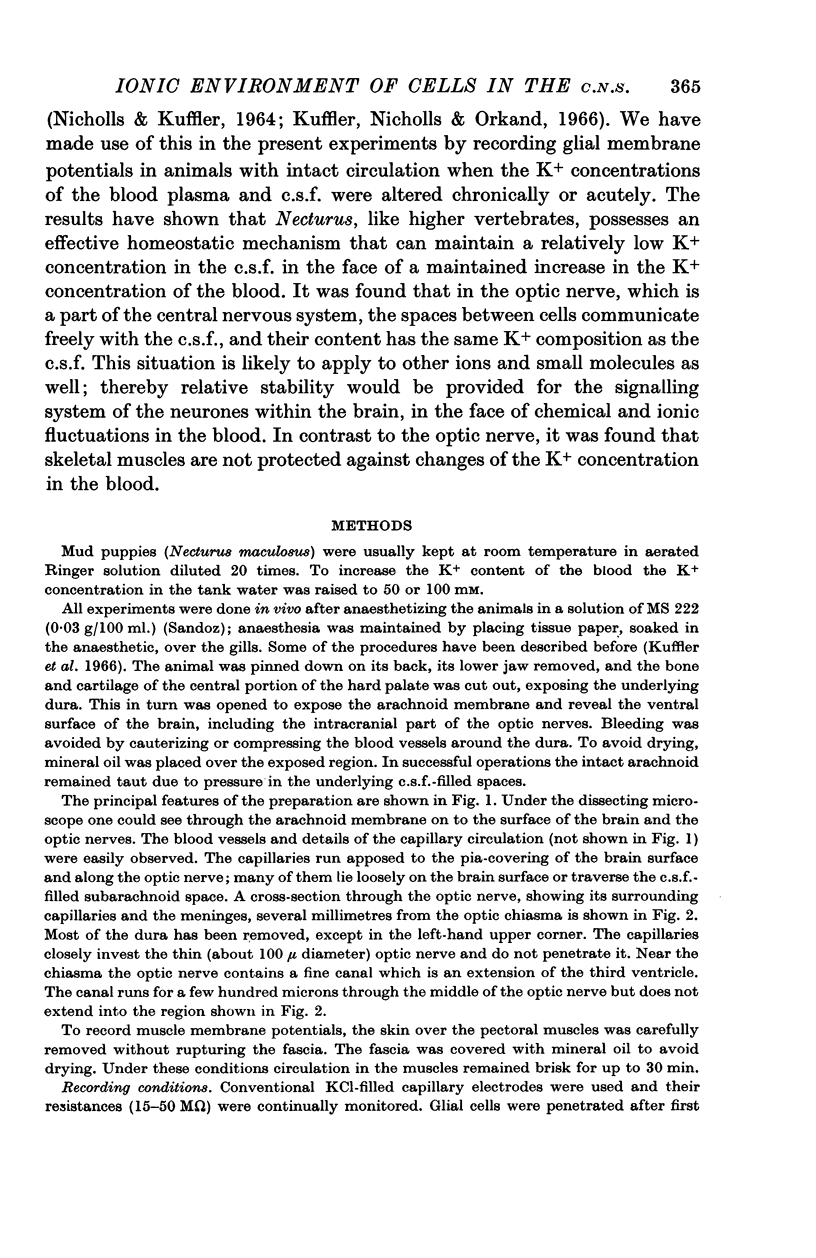
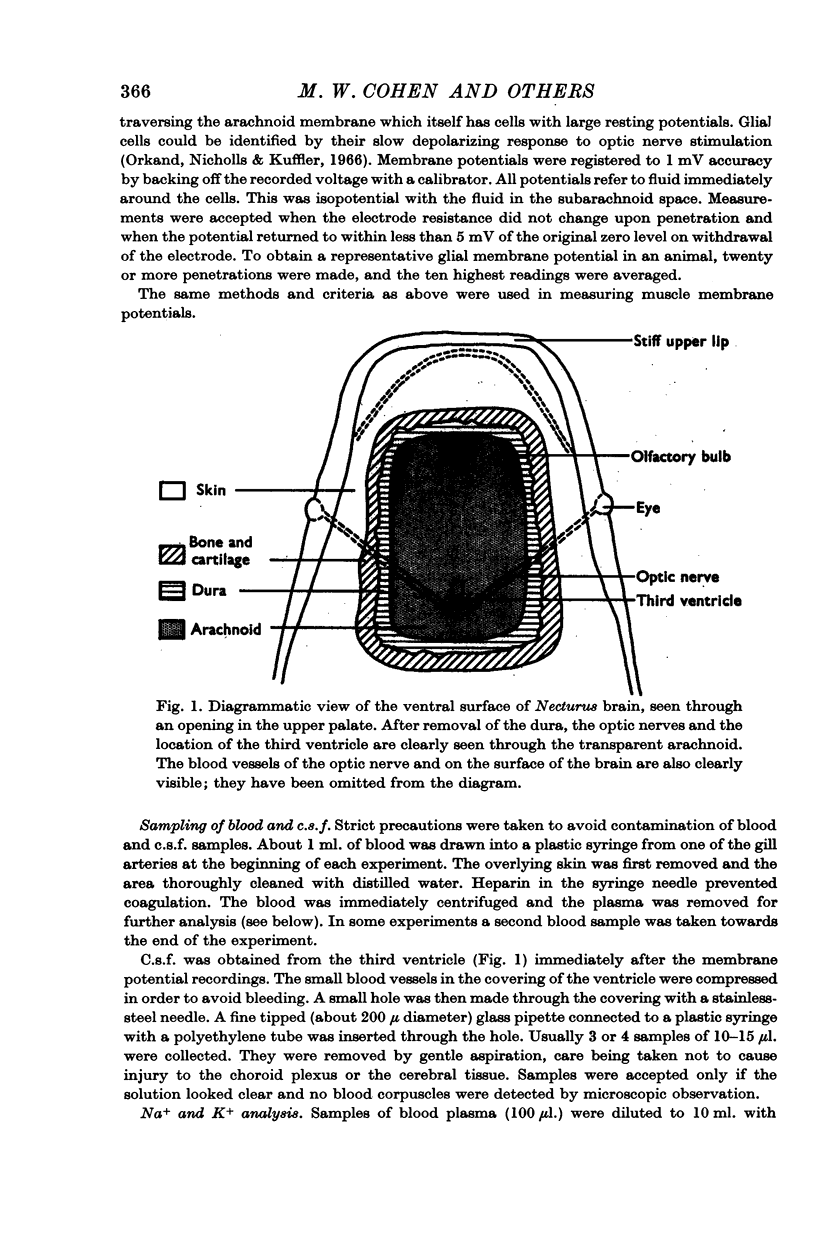
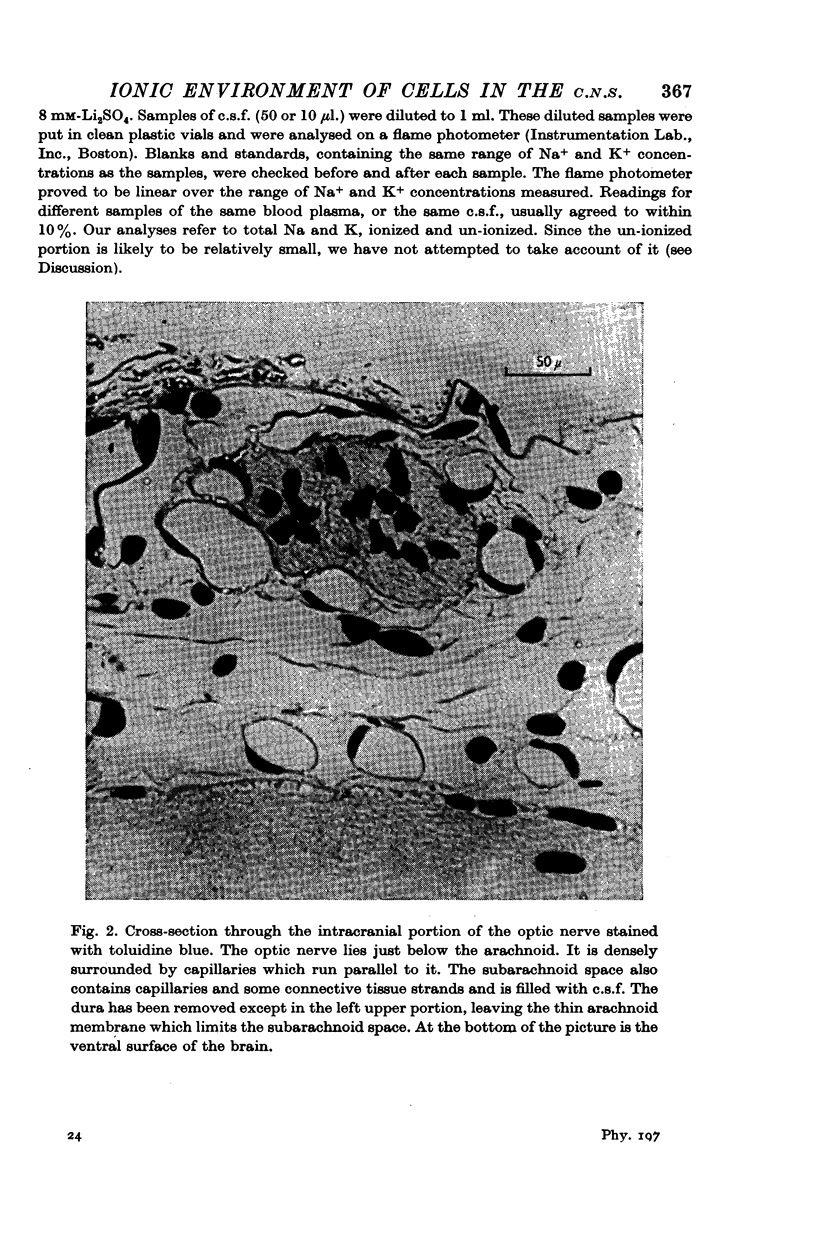
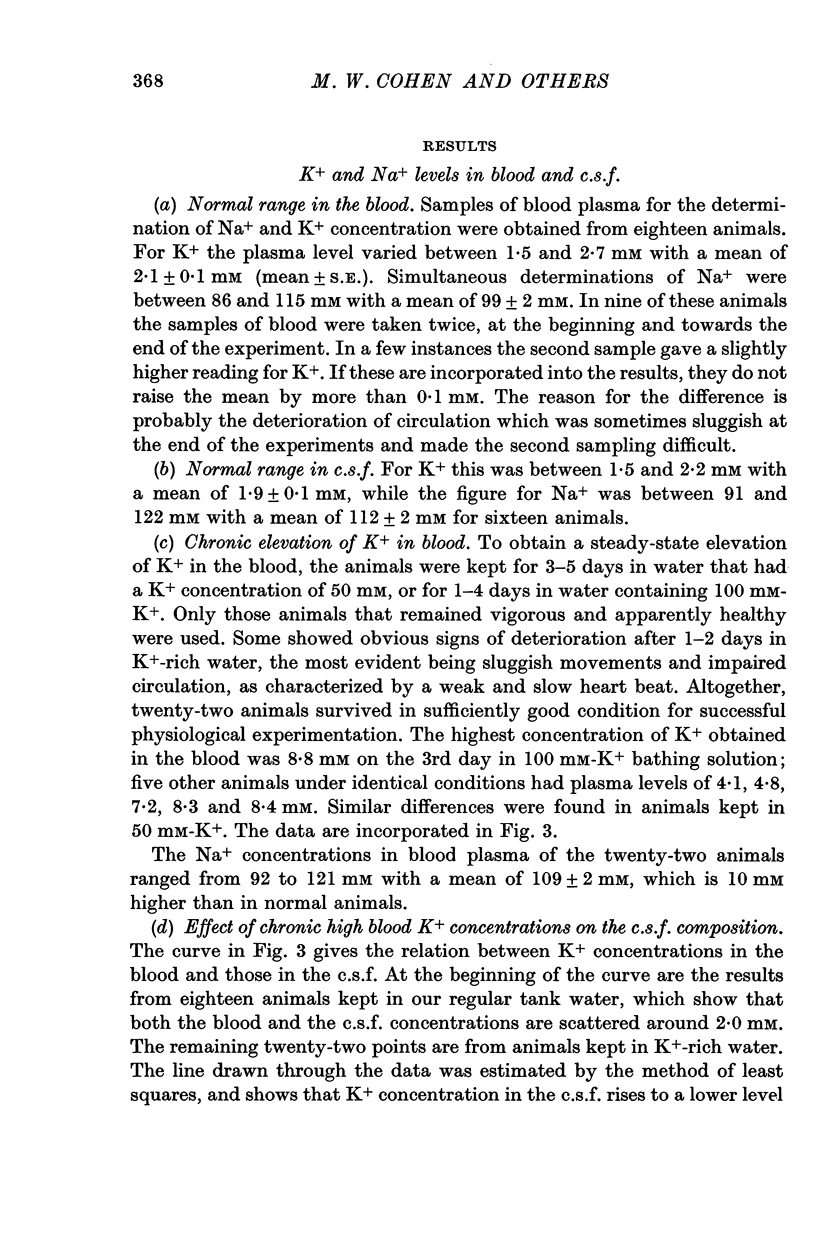
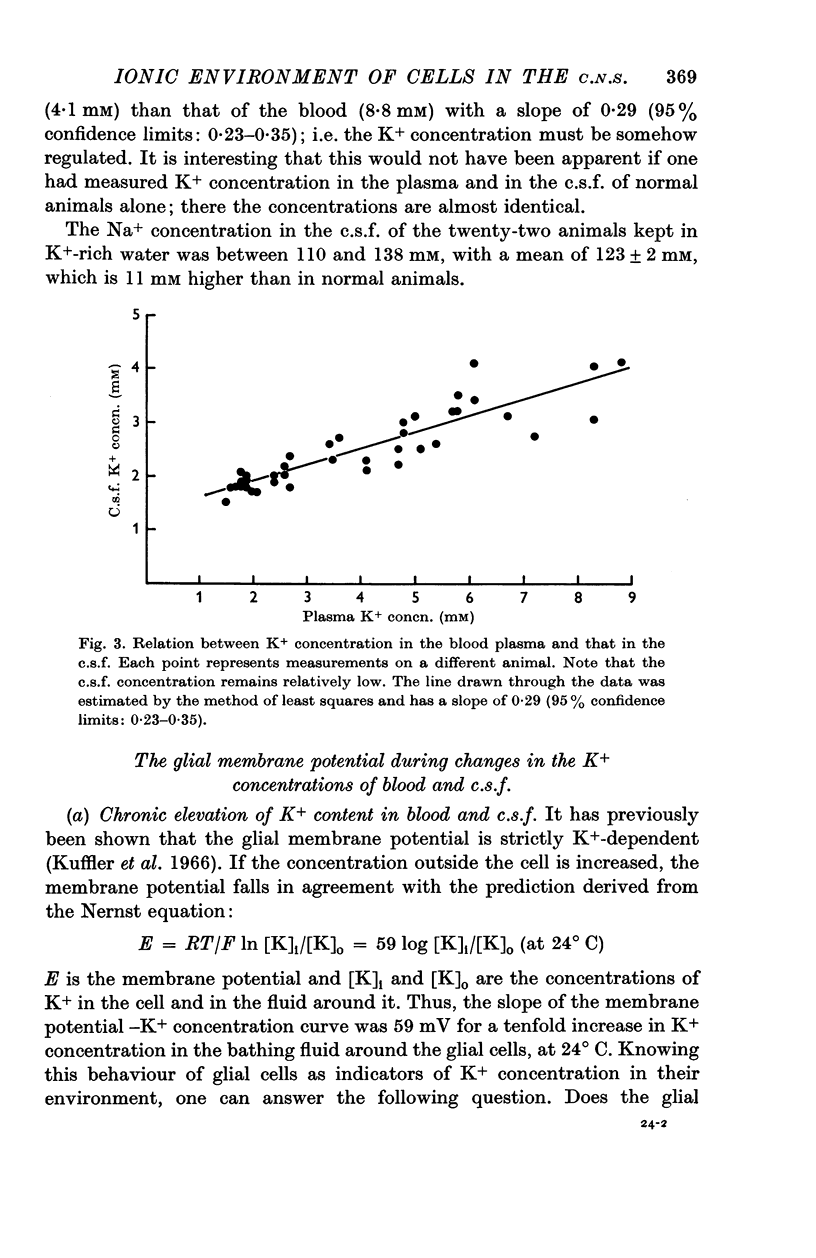
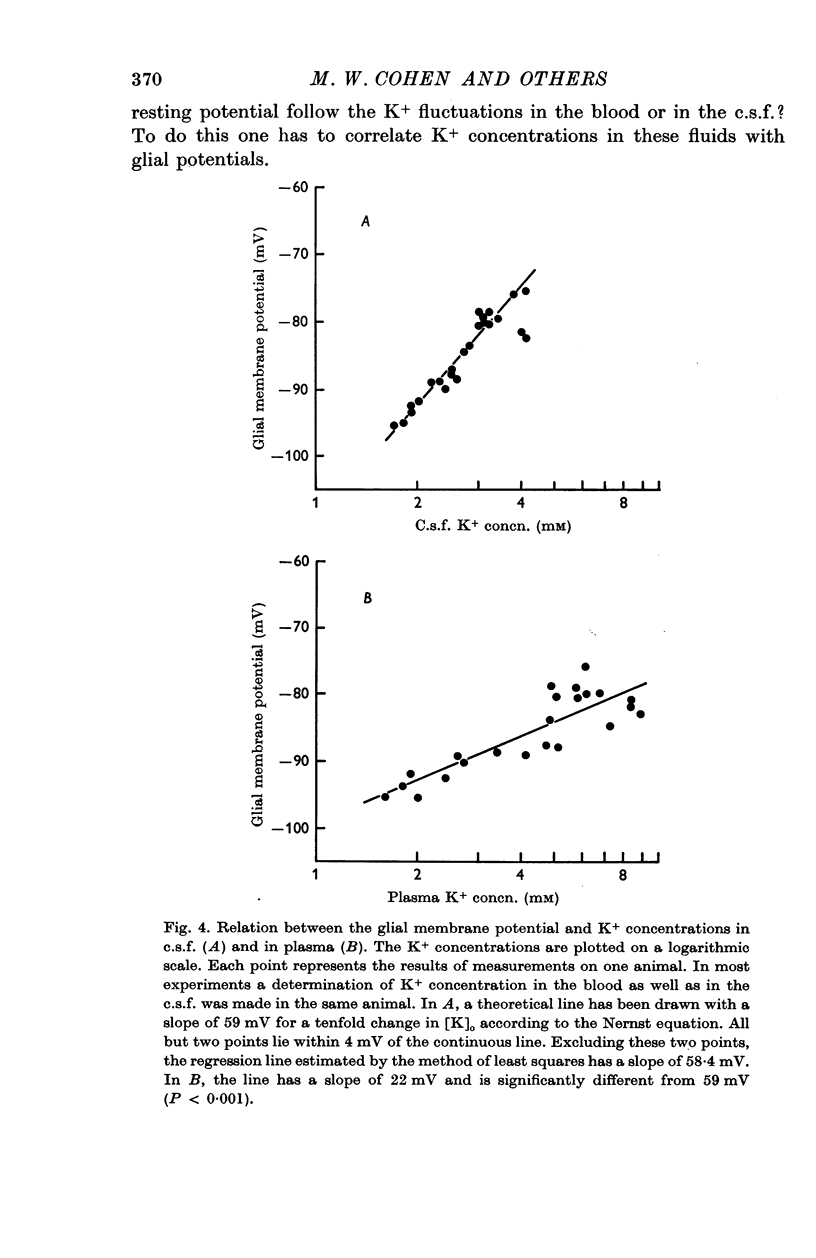
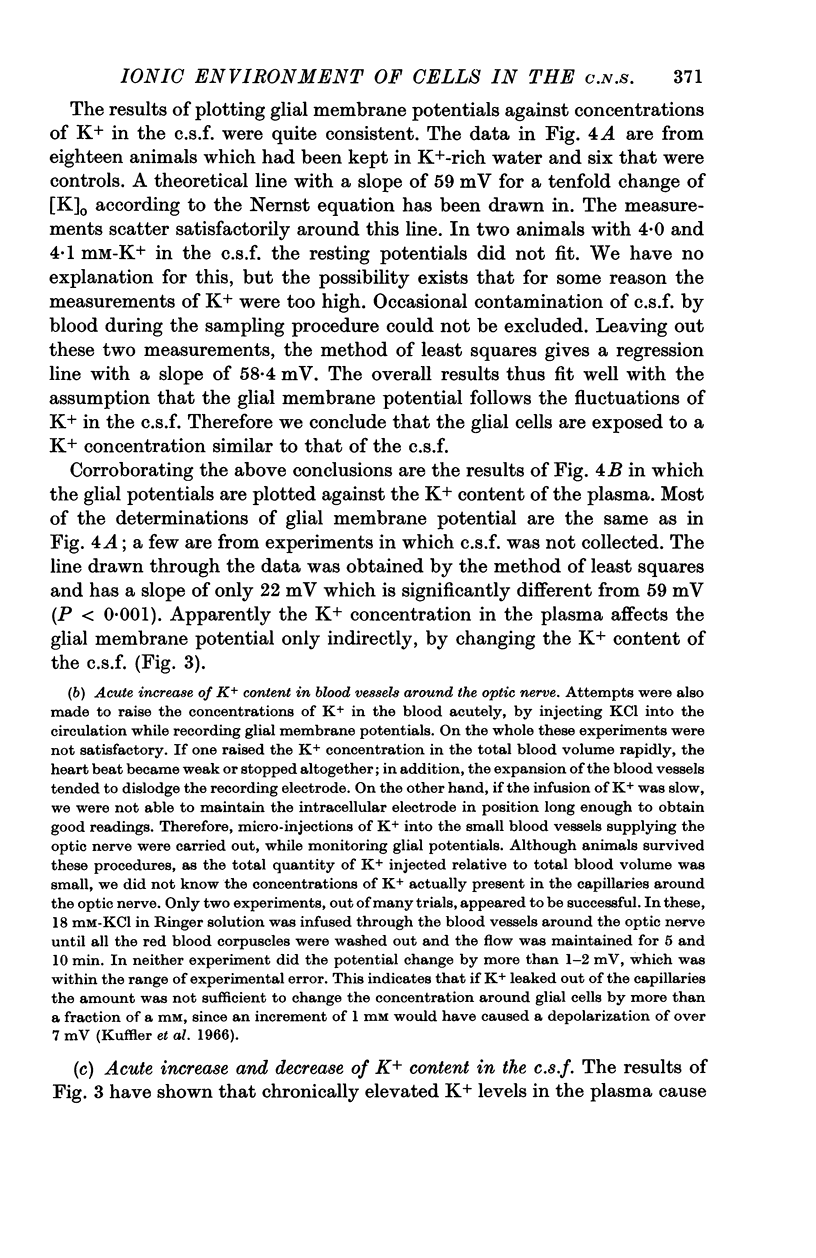
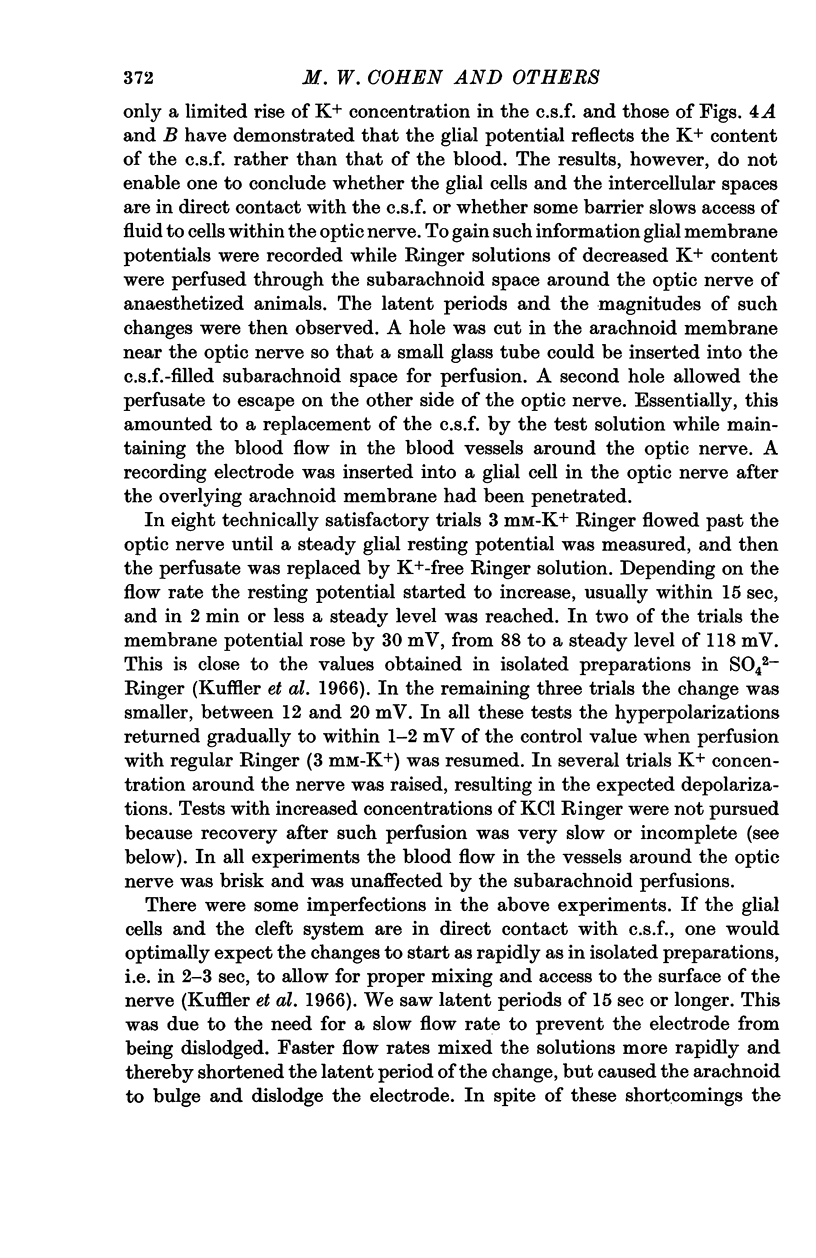
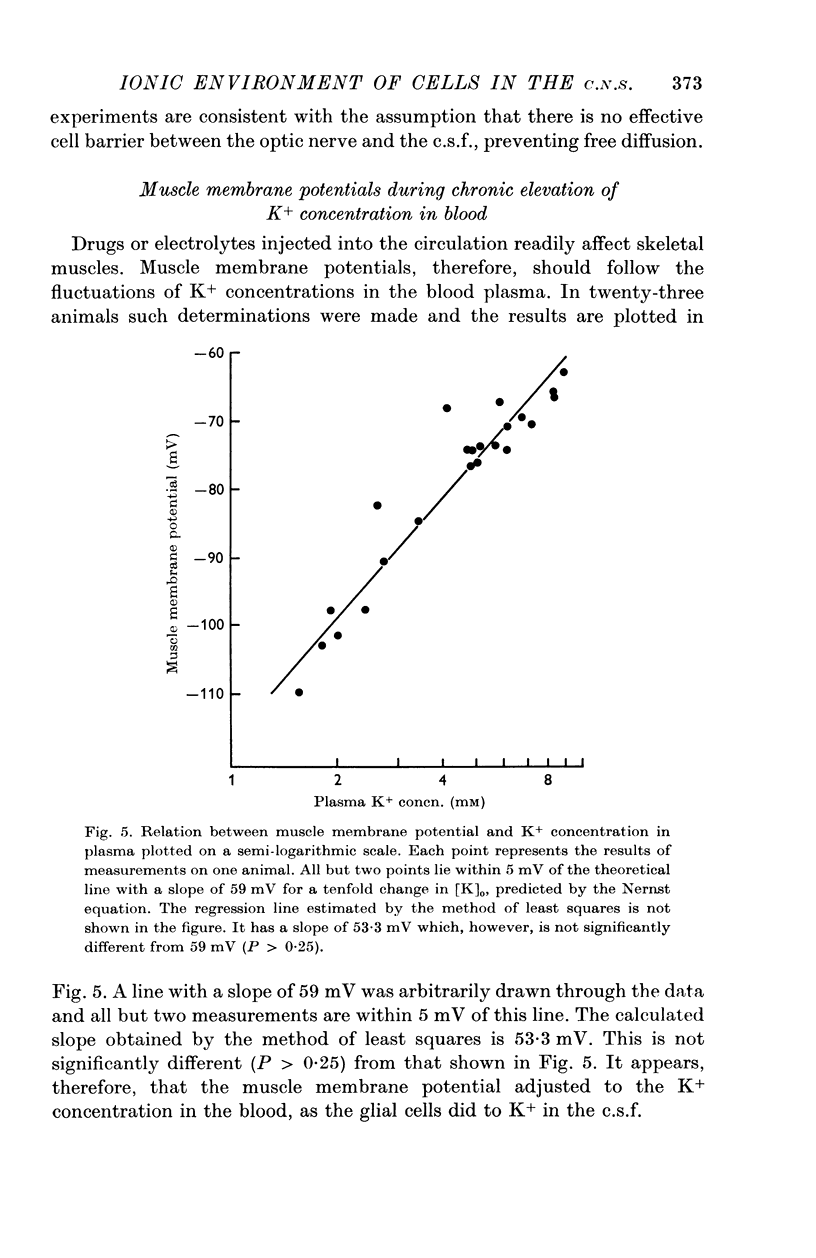
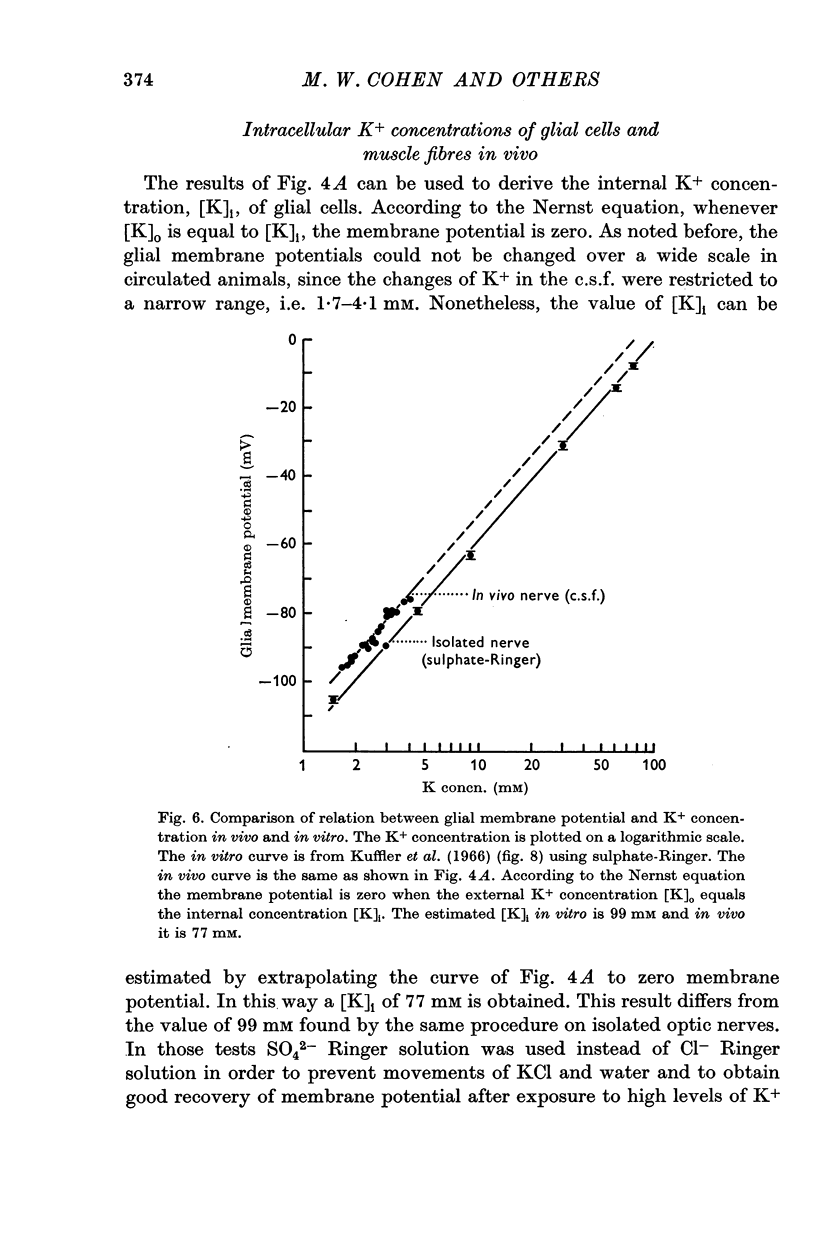
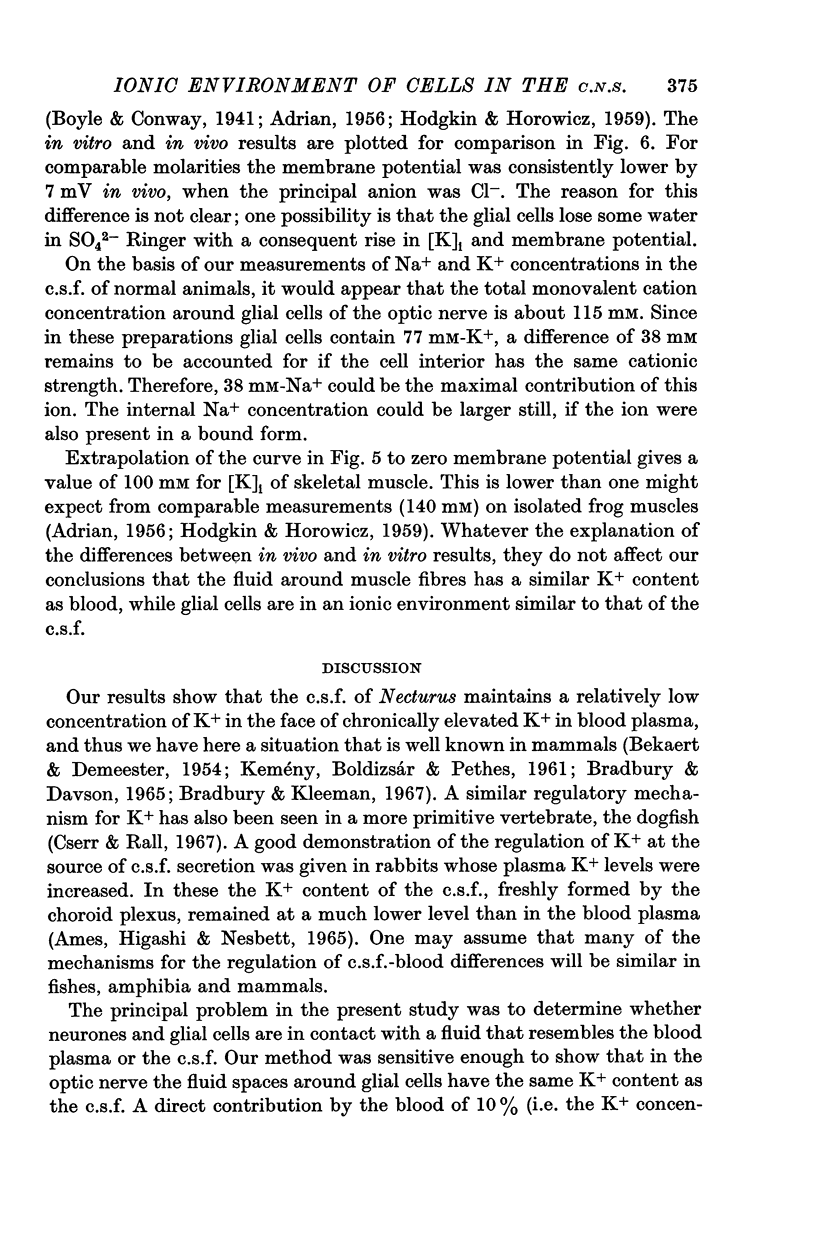
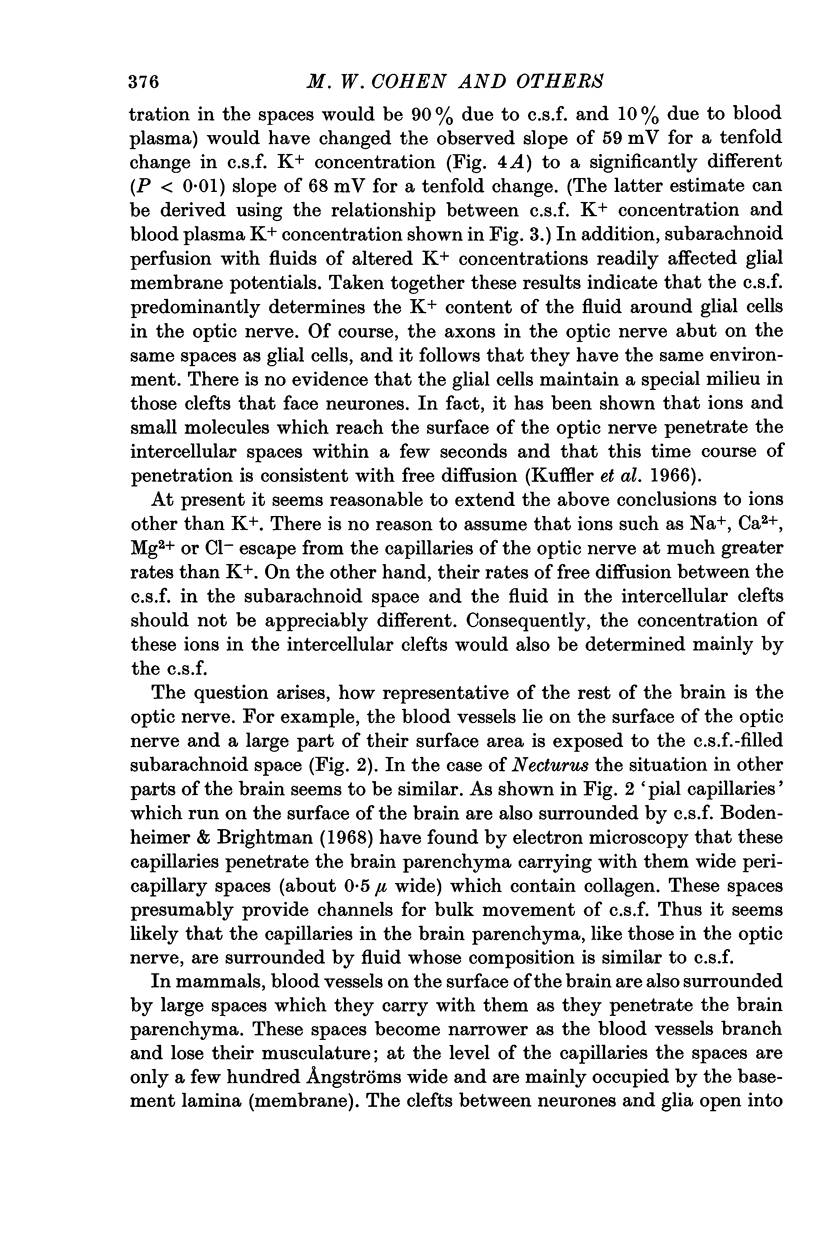
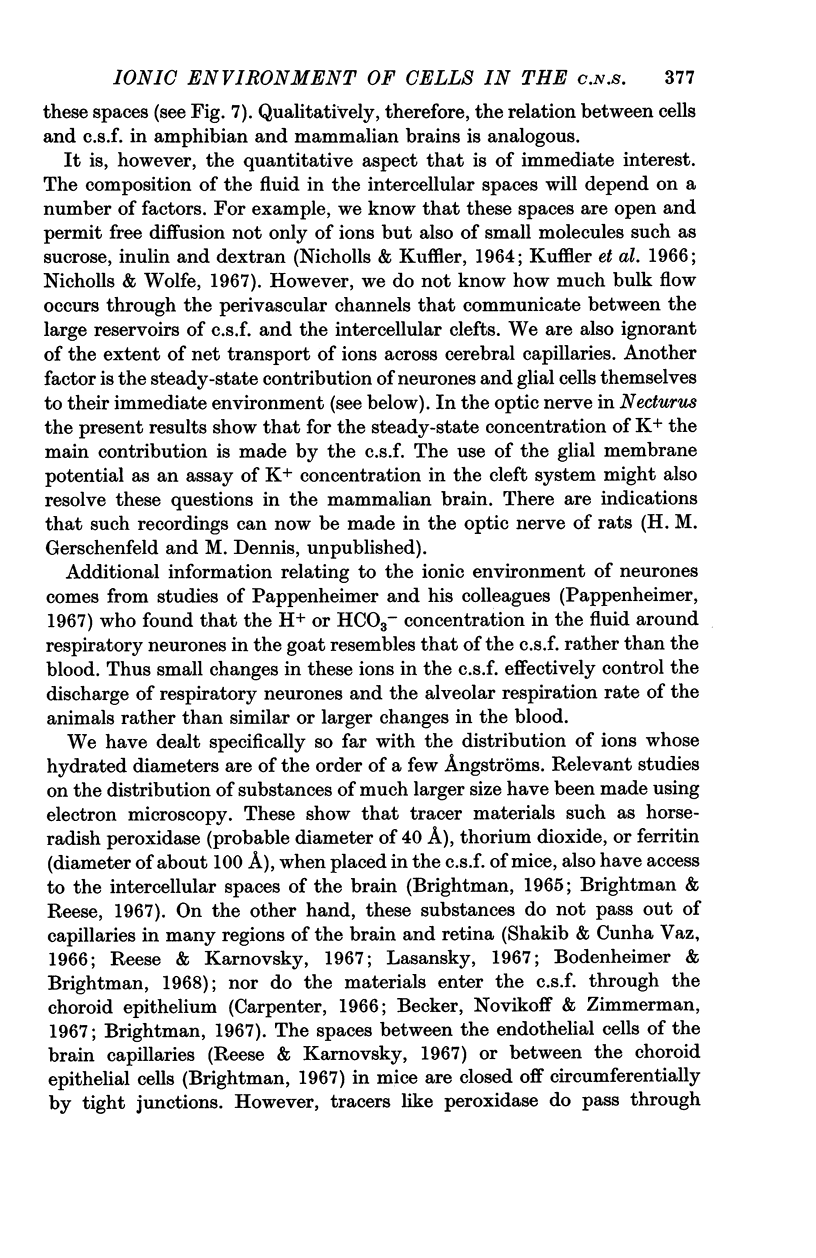
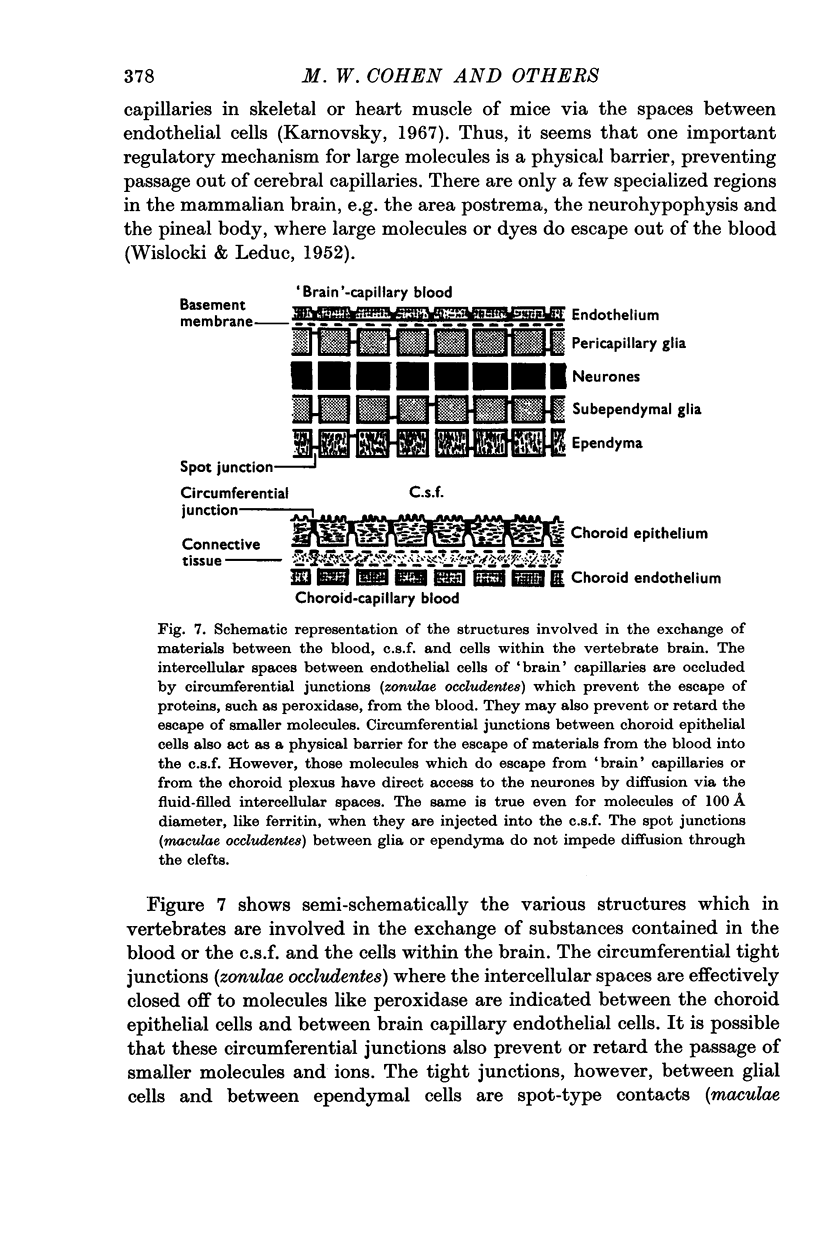
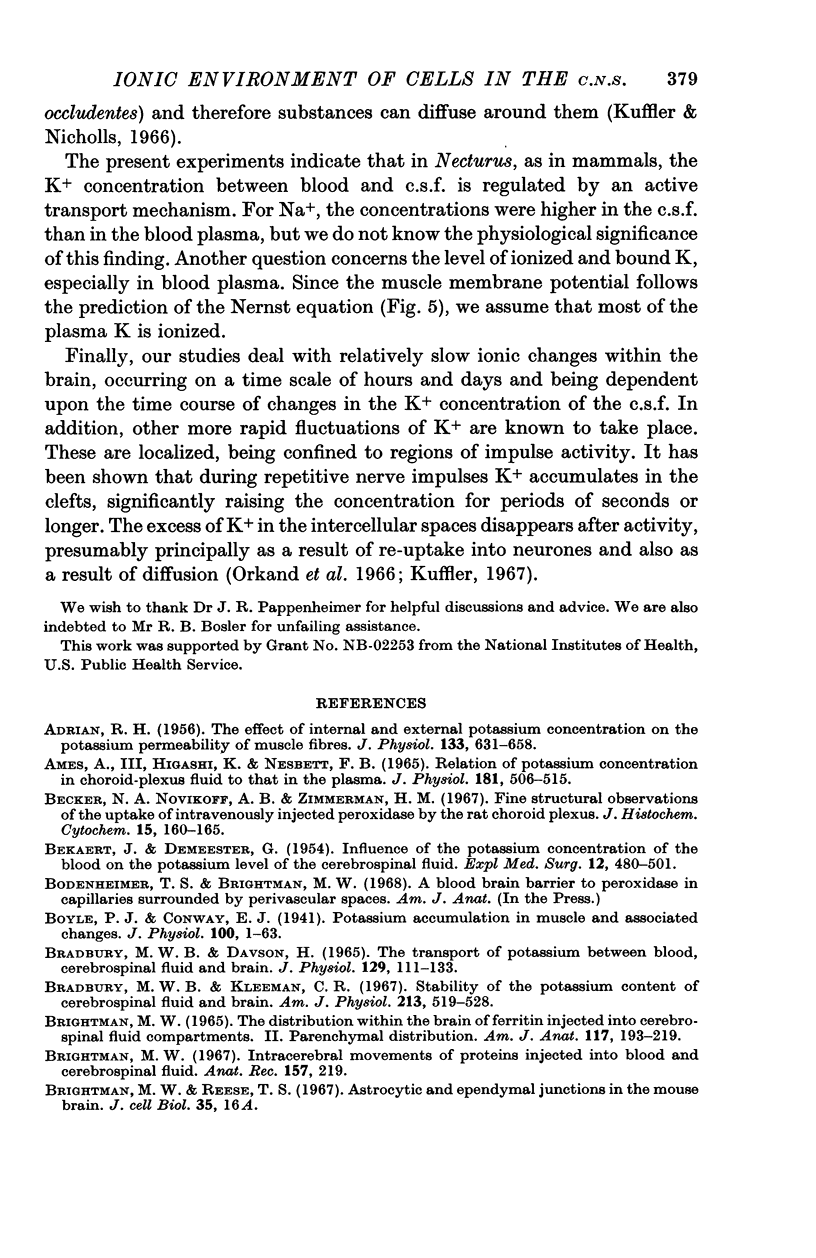
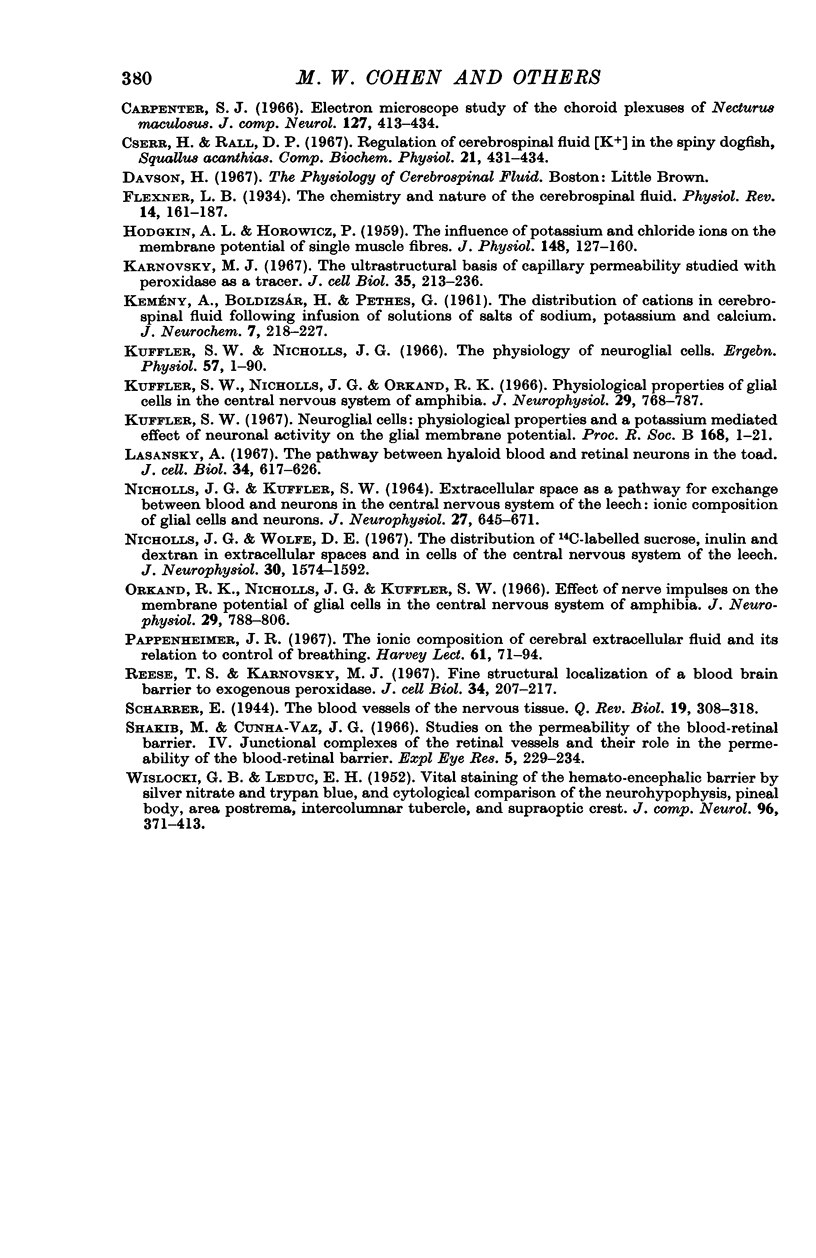
Images in this article
Selected References
These references are in PubMed. This may not be the complete list of references from this article.
- ADRIAN R. H. The effect of internal and external potassium concentration on the membrane potential of frog muscle. J Physiol. 1956 Sep 27;133(3):631–658. doi: 10.1113/jphysiol.1956.sp005615. [DOI] [PMC free article] [PubMed] [Google Scholar]
- Ames A., 3rd, Higashi K., Nesbett F. B. Relation of potassium concentration in choroidplexus fluid to that in plasma. J Physiol. 1965 Dec;181(3):506–515. doi: 10.1113/jphysiol.1965.sp007779. [DOI] [PMC free article] [PubMed] [Google Scholar]
- BEKAERT J., DEMEESTER G. Influence of the potassium concentration of the blood on the potassium level of the cerebrospinal fluid. Exp Med Surg. 1954;12(4):480–501. [PubMed] [Google Scholar]
- Becker N. H., Novikoff A. B., Zimmerman H. M. Fine structure observations of the uptake of intravenously injected peroxidase by the rat choroid plexus. J Histochem Cytochem. 1967 Mar;15(3):160–165. doi: 10.1177/15.3.160. [DOI] [PubMed] [Google Scholar]
- Boyle P. J., Conway E. J. Potassium accumulation in muscle and associated changes. J Physiol. 1941 Aug 11;100(1):1–63. doi: 10.1113/jphysiol.1941.sp003922. [DOI] [PMC free article] [PubMed] [Google Scholar]
- Bradbury M. W., Kleeman C. R. Stability of the potassium content of cerebrospinal fluid and brain. Am J Physiol. 1967 Aug;213(2):519–528. doi: 10.1152/ajplegacy.1967.213.2.519. [DOI] [PubMed] [Google Scholar]
- Brightman M. W. The distribution within the brain of ferritin injected into cerebrospinal fluid compartments. II. Parenchymal distribution. Am J Anat. 1965 Sep;117(2):193–219. doi: 10.1002/aja.1001170204. [DOI] [PubMed] [Google Scholar]
- Carpenter S. J. An electron microscopic study of the choroid plexuses of Necturus maculosus. J Comp Neurol. 1966 Jul;127(3):413–434. doi: 10.1002/cne.901270309. [DOI] [PubMed] [Google Scholar]
- Cserr H., Rall D. P. Regulation of cerebrospinal fluid [K+] in the spiny dogfish, Squalus acanthias. Comp Biochem Physiol. 1967 May;21(2):431–434. doi: 10.1016/0010-406x(67)90805-5. [DOI] [PubMed] [Google Scholar]
- HODGKIN A. L., HOROWICZ P. The influence of potassium and chloride ions on the membrane potential of single muscle fibres. J Physiol. 1959 Oct;148:127–160. doi: 10.1113/jphysiol.1959.sp006278. [DOI] [PMC free article] [PubMed] [Google Scholar]
- KEMENY A., BOLDIZSAR H., PETHES G. The distribution of cations in plasma and cerebrospinal fluid following infusion of solution of salts of sodium, potassium magnesium and calcium. J Neurochem. 1961 Jul;7:218–227. doi: 10.1111/j.1471-4159.1961.tb13506.x. [DOI] [PubMed] [Google Scholar]
- Karnovsky M. J. The ultrastructural basis of capillary permeability studied with peroxidase as a tracer. J Cell Biol. 1967 Oct;35(1):213–236. doi: 10.1083/jcb.35.1.213. [DOI] [PMC free article] [PubMed] [Google Scholar]
- Kuffler S. W. Neuroglial cells: physiological properties and a potassium mediated effect of neuronal activity on the glial membrane potential. Proc R Soc Lond B Biol Sci. 1967 Jun 6;168(1010):1–21. doi: 10.1098/rspb.1967.0047. [DOI] [PubMed] [Google Scholar]
- Kuffler S. W., Nicholls J. G., Orkand R. K. Physiological properties of glial cells in the central nervous system of amphibia. J Neurophysiol. 1966 Jul;29(4):768–787. doi: 10.1152/jn.1966.29.4.768. [DOI] [PubMed] [Google Scholar]
- Kuffler S. W., Nicholls J. G. The physiology of neuroglial cells. Ergeb Physiol. 1966;57:1–90. [PubMed] [Google Scholar]
- Lasansky A. The pathway between hyaloid blood and retinal neurons in the toad. Structural observations and permeability to tracer substances. J Cell Biol. 1967 Aug;34(2):617–626. doi: 10.1083/jcb.34.2.617. [DOI] [PMC free article] [PubMed] [Google Scholar]
- NICHOLLS J. G., KUFFLER S. W. EXTRACELLULAR SPACE AS A PATHWAY FOR EXCHANGE BETWEEN BLOOD AND NEURONS IN THE CENTRAL NERVOUS SYSTEM OF THE LEECH: IONIC COMPOSITION OF GLIAL CELLS AND NEURONS. J Neurophysiol. 1964 Jul;27:645–671. doi: 10.1152/jn.1964.27.4.645. [DOI] [PubMed] [Google Scholar]
- Nicholls J. G., Wolfe D. E. Distribution of 14C-labeled sucrose, inulin, and dextran in extracellular spaces and in cells of the leech central nervous system. J Neurophysiol. 1967 Nov;30(6):1574–1592. doi: 10.1152/jn.1967.30.6.1574. [DOI] [PubMed] [Google Scholar]
- Orkand R. K., Nicholls J. G., Kuffler S. W. Effect of nerve impulses on the membrane potential of glial cells in the central nervous system of amphibia. J Neurophysiol. 1966 Jul;29(4):788–806. doi: 10.1152/jn.1966.29.4.788. [DOI] [PubMed] [Google Scholar]
- Pappenheimer J. R. The ionic composition of cerebral extracellular fluid and its relation to control of breathing. Harvey Lect. 1967;61:71–94. [PubMed] [Google Scholar]
- Reese T. S., Karnovsky M. J. Fine structural localization of a blood-brain barrier to exogenous peroxidase. J Cell Biol. 1967 Jul;34(1):207–217. doi: 10.1083/jcb.34.1.207. [DOI] [PMC free article] [PubMed] [Google Scholar]
- Shakib M., Cunha-Vaz J. G. Studies on the permeability of the blood-retinal barrier. IV. Junctional complexes of the retinal vessels and their role in the permeability of the blood-retinal barrier. Exp Eye Res. 1966 Jul;5(3):229–234. doi: 10.1016/s0014-4835(66)80011-8. [DOI] [PubMed] [Google Scholar]
- WISLOCKI G. B., LEDUC E. H. Vital staining of the hematoencephalic barrier by silver nitrate and trypan blue, and cytological comparisons of the neurohypophysis, pineal body, area postrema, intercolumnar tubercle and supraoptic crest. J Comp Neurol. 1952 Jun;96(3):371–413. doi: 10.1002/cne.900960302. [DOI] [PubMed] [Google Scholar]