Abstract
1. The concentrations of sodium, potassium and chloride in frog and bovine lenses showed a normal intracellular ion distribution with the sum of the internal cations approximately equal to the external sum. In the cephalopod lens, however, the sum inside was much lower than that outside.
2. The membrane potentials of frog, Sepiola and bovine lenses were -63, -63 and -23 mV respectively. A comparison of the electrical data with the Nernst potentials predicted from ion concentration data indicated that sodium and chloride ions as well as potassium contributed to the membrane potential in frog and bovine. In contrast, the membrane and Nernst potentials for potassium were equal in Sepiola.
3. Substituting potassium for sodium in the external medium depolarized lens potentials in all three species. Estimates of the relative permeabilities of sodium, potassium and chloride were obtained by fitting the Goldman-Hodgkin-Katz equation to the potential data.
4. The potassium permeability was determined directly by 42K efflux measurements and values of 2·99, 9·83 and 3·13 (× -8 m sec-1) were obtained for frog, Sepiola and bovine lenses respectively.
5. The effect of raising external potassium on the efflux rate constant was determined and there was reasonable agreement between experiment and theory (Kimizuka-Koketsu) in frog and bovine lenses, but the Sepiola data indicated that the potassium permeability decreased by a factor of 2·6 when the external potassium was raised from 10 to 120 mM-K+.
6. The measured specific conductances, obtained using two internal micro-electrodes, were 7·7, 15·9 and 9·9 (Sm-2) for frog, cephalopod and bovine lenses respectively. These data compare with computed values (Kimizuka-Koketsu theory) of 7·5, 14·1 and 17·2 (Sm-2).
7. The effect of increasing external potassium on the conductance was also tested and there was good agreement between experiment and theory (assuming constant permeabilities) only in the amphibian lens. However, when the cephalopod data were corrected assuming a 2·6-fold decrease in PK for a twelvefold increase in potassium, then there was excellent agreement between experiment and theory.
8. The bovine measured conductances were much lower than the theoretical values throughout the range of external potassium concentrations and several explanations were proposed to account for the discrepancies.
Full text
PDF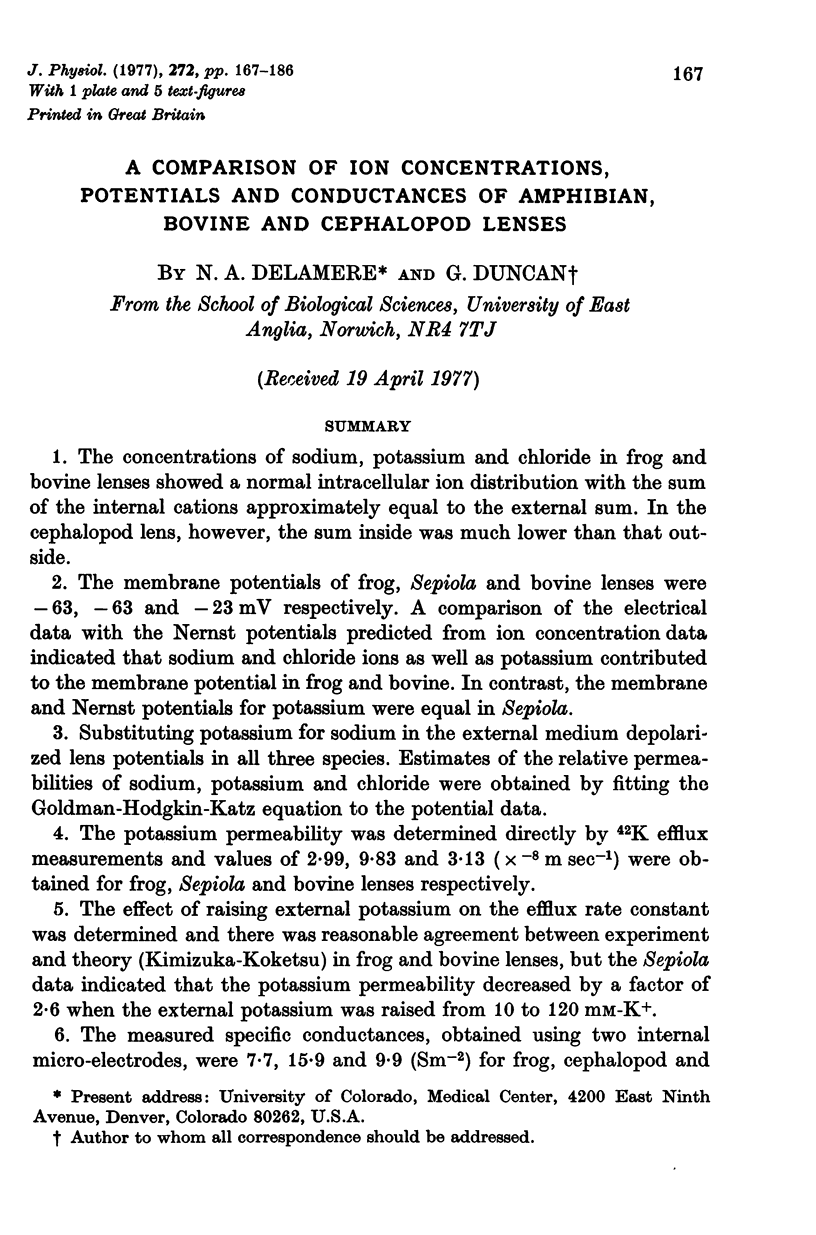
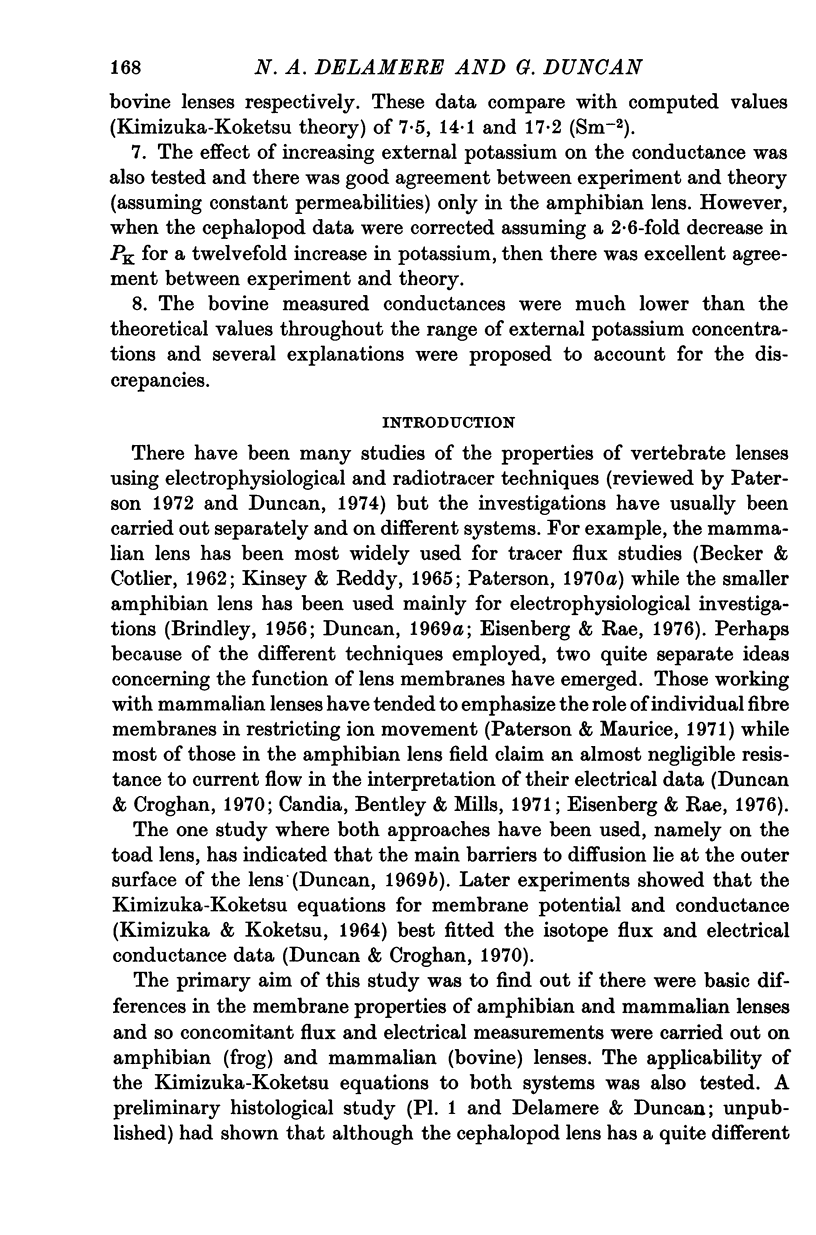
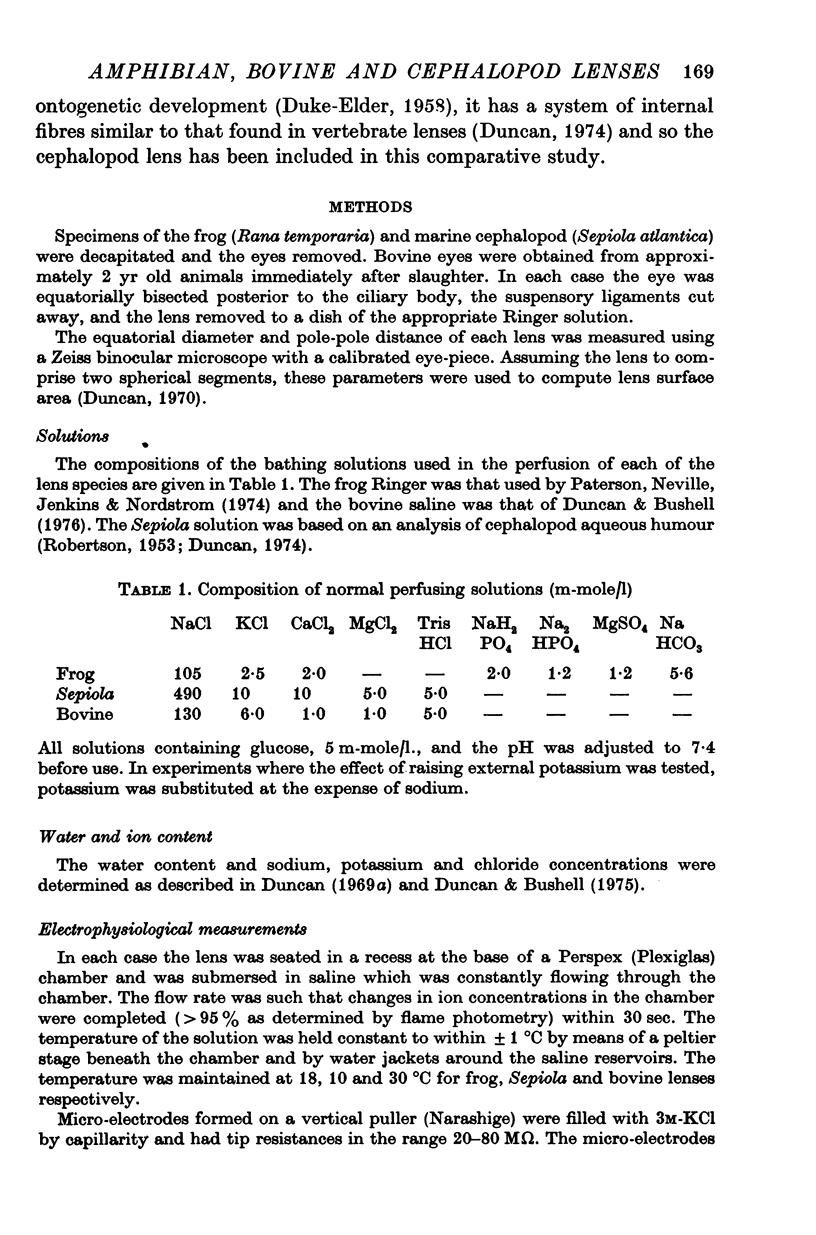
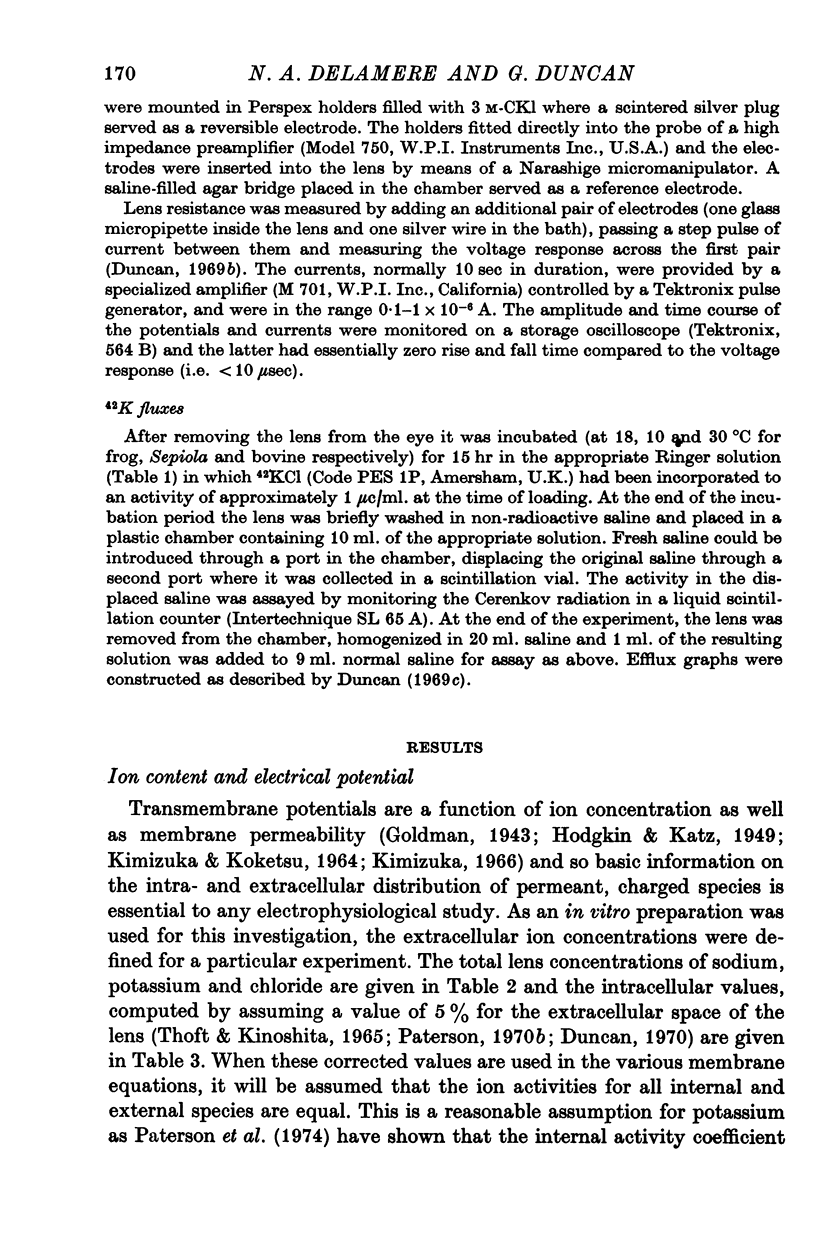
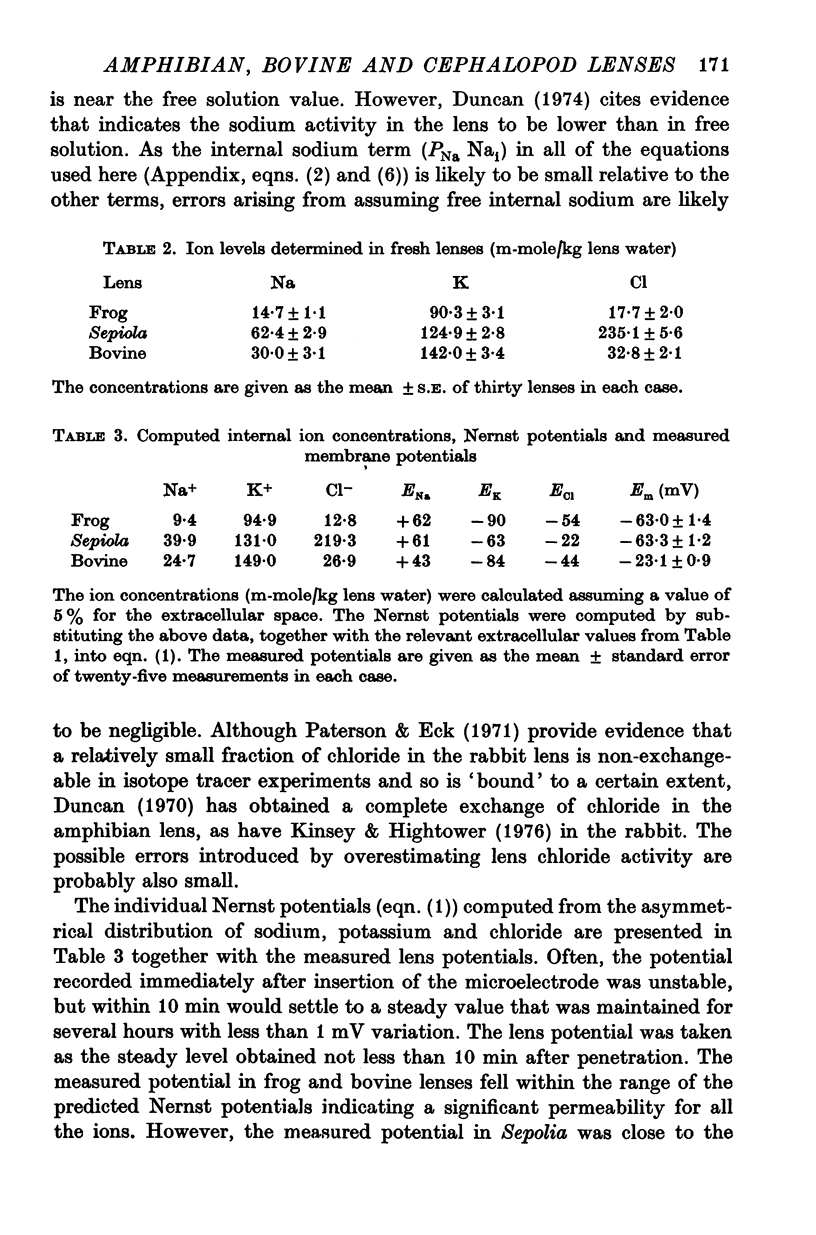
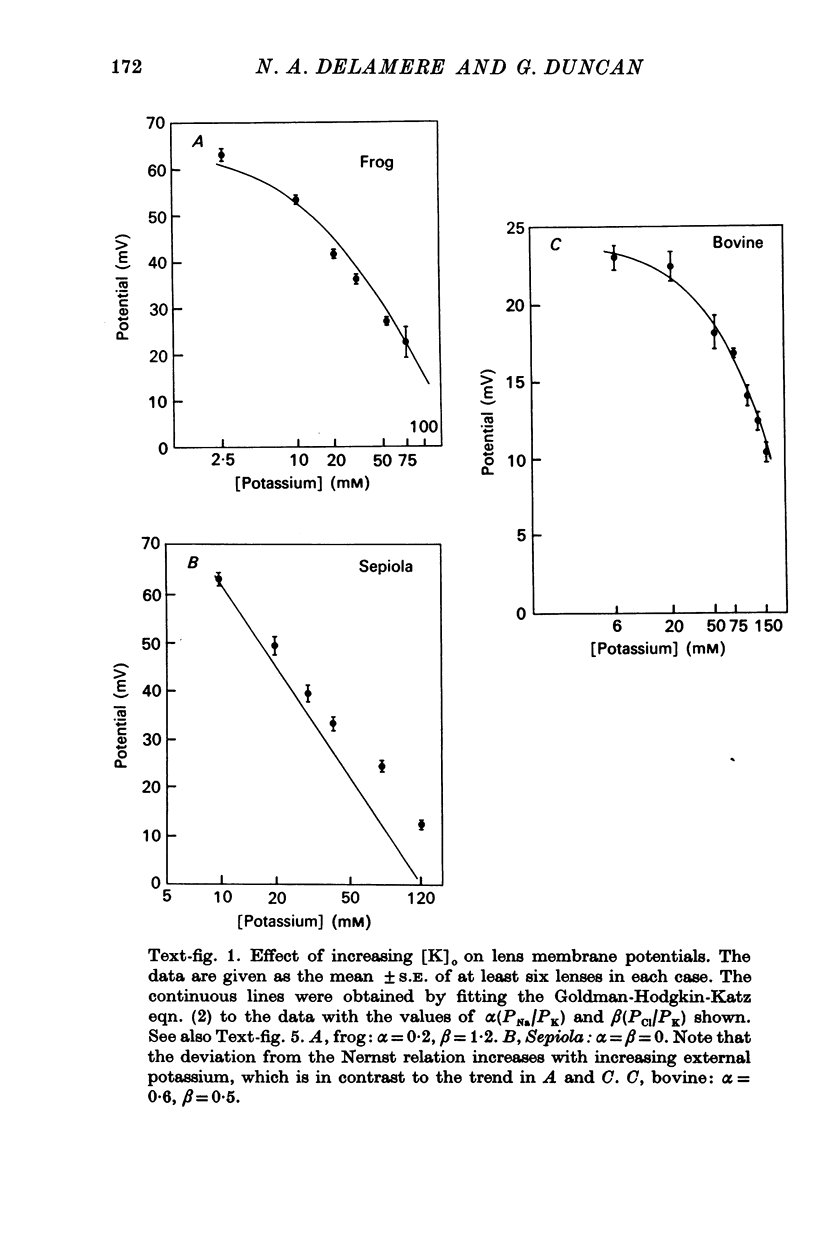
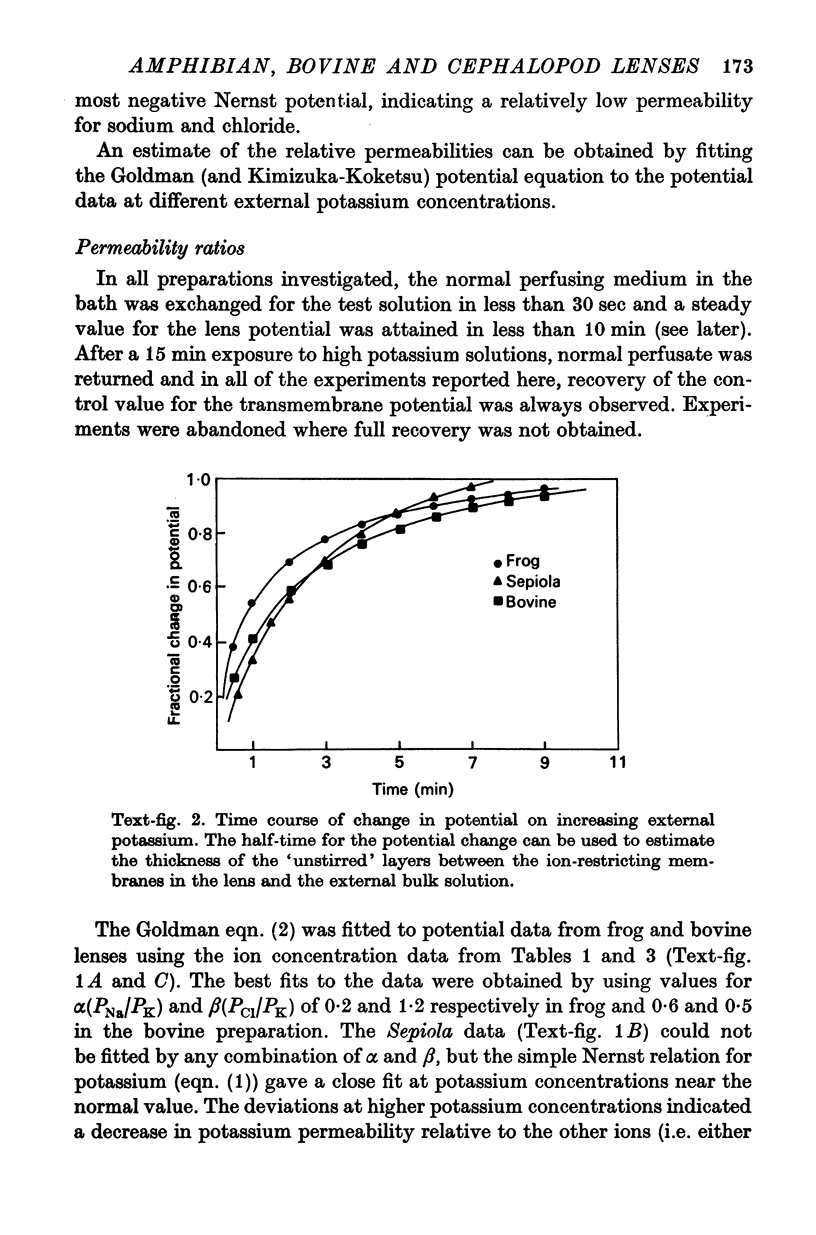
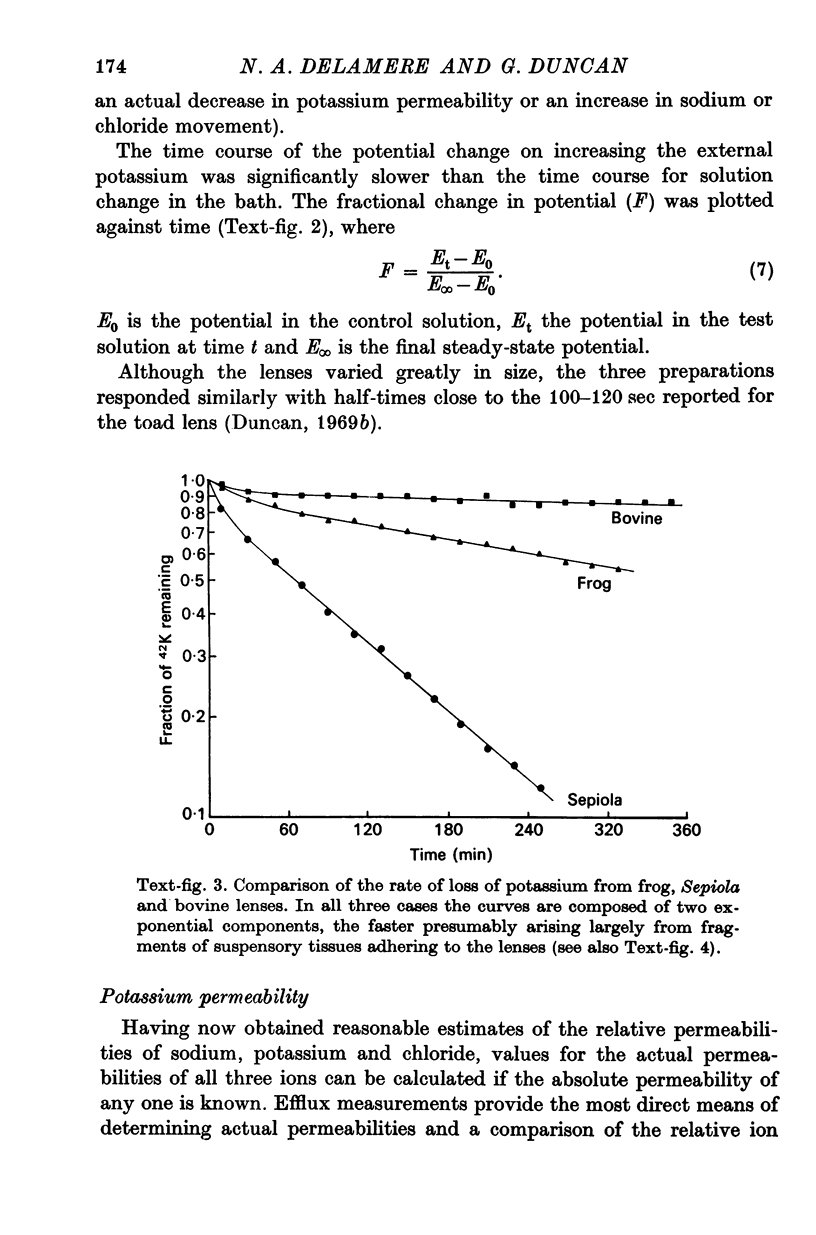
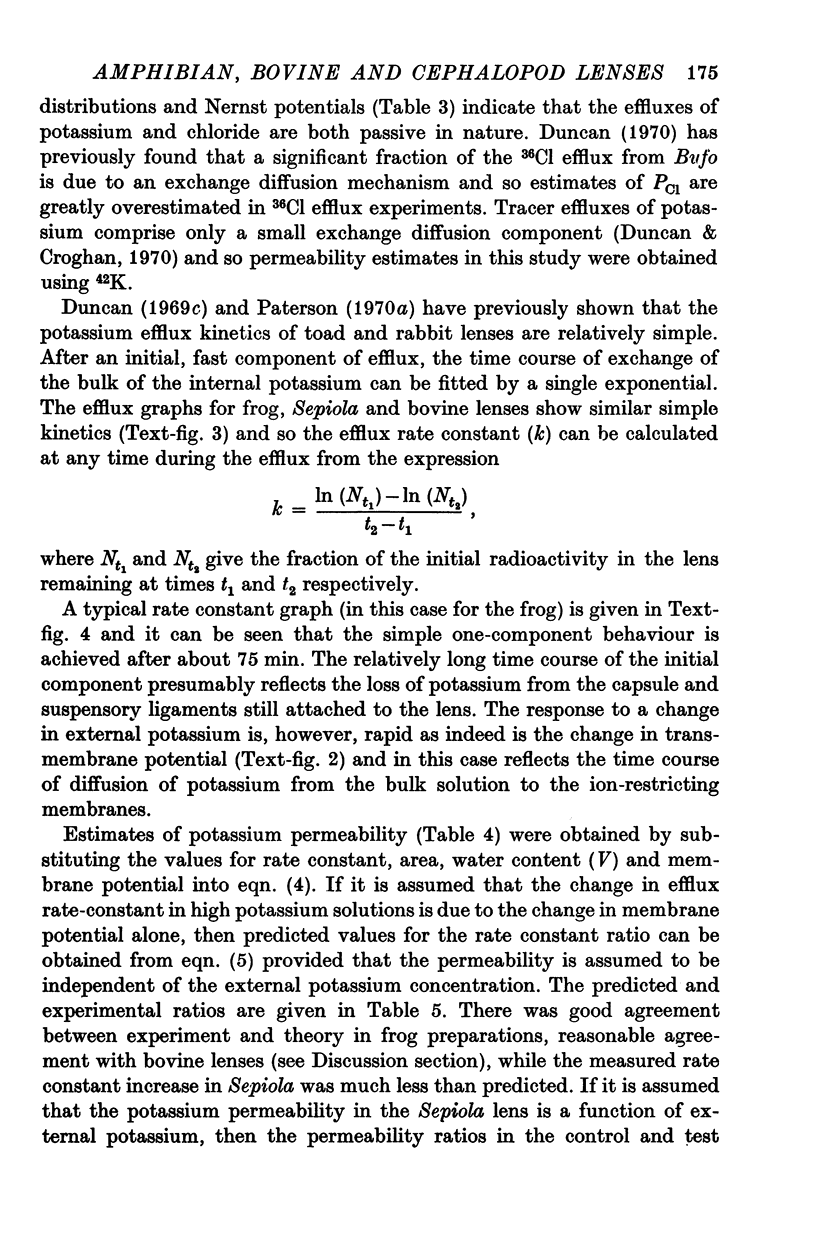
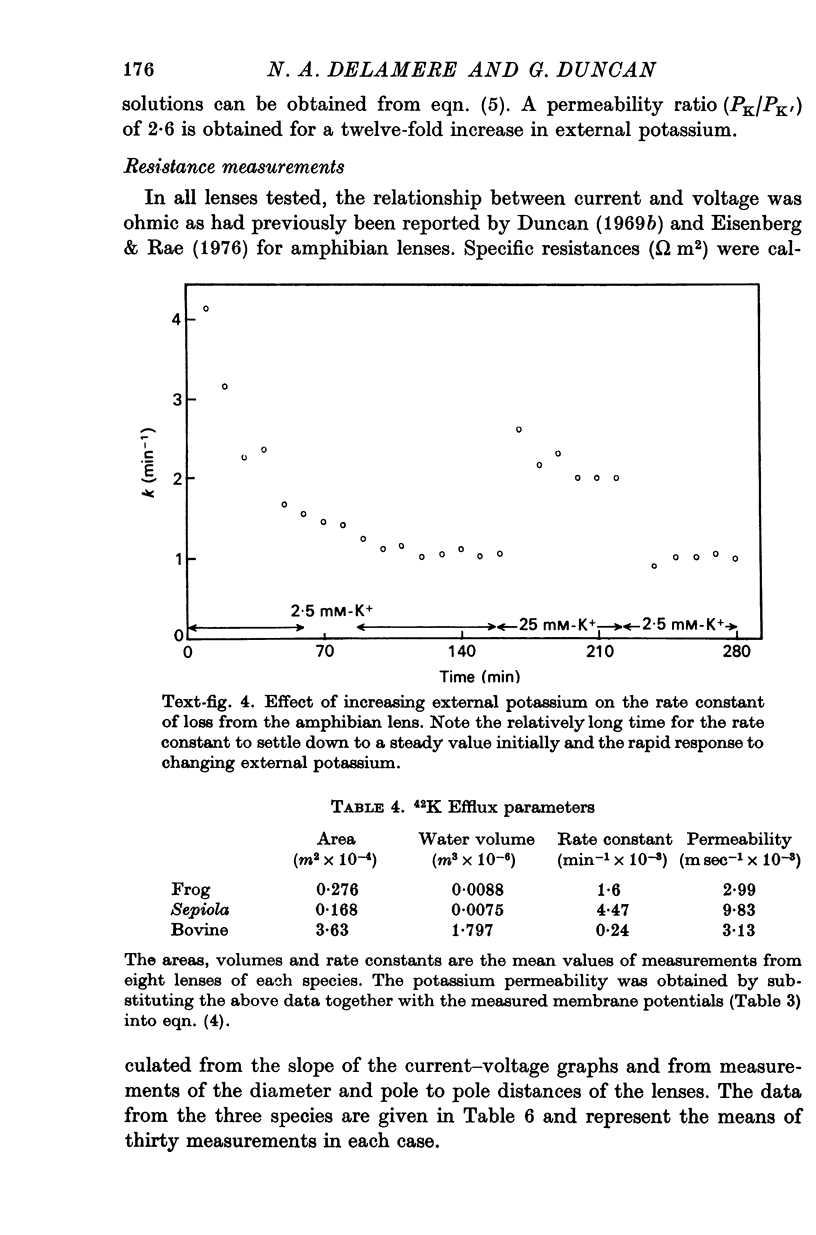
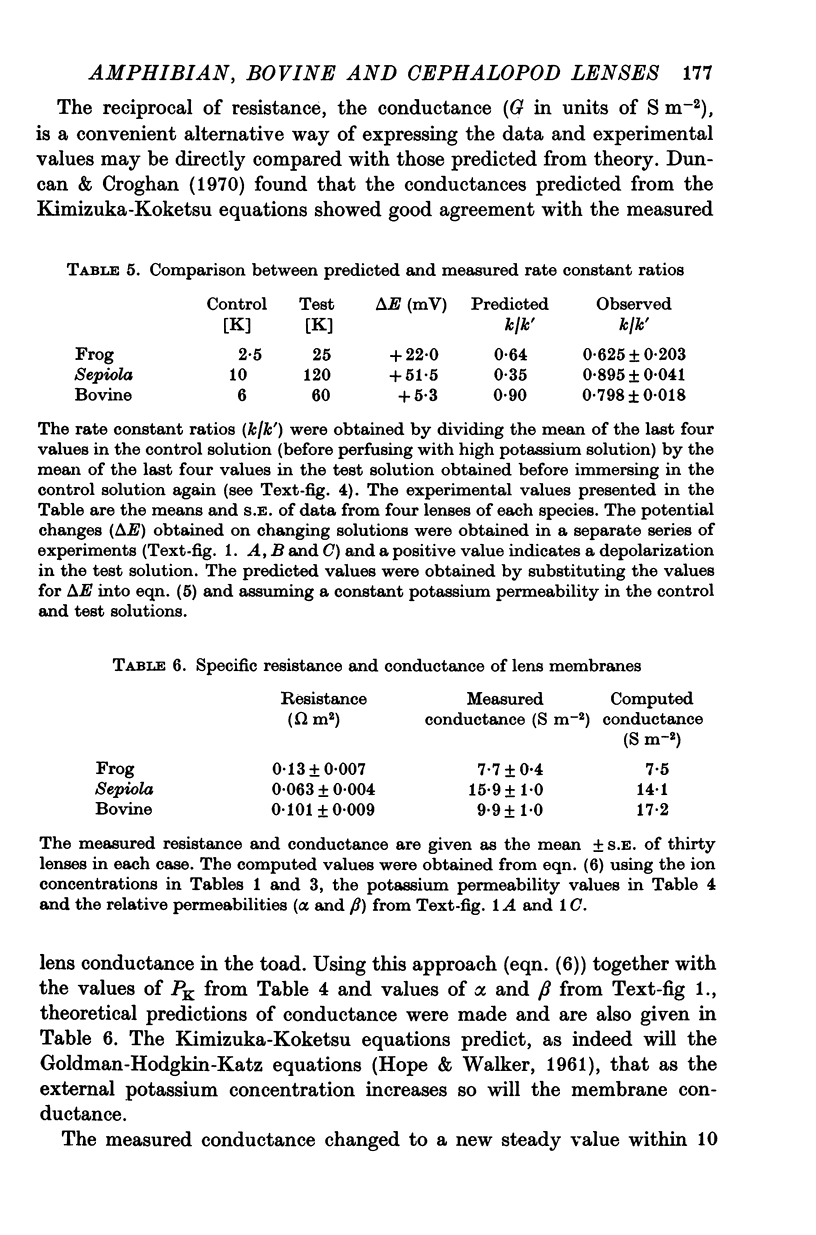
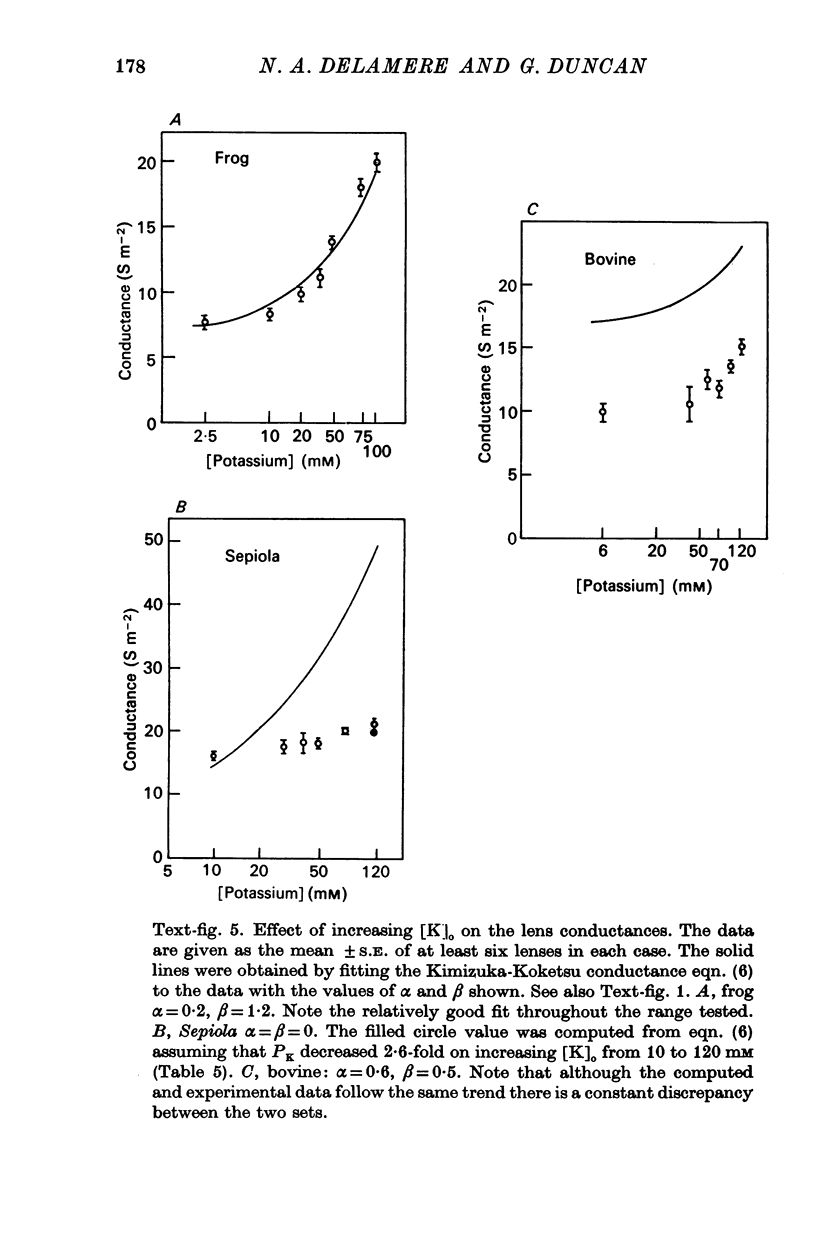
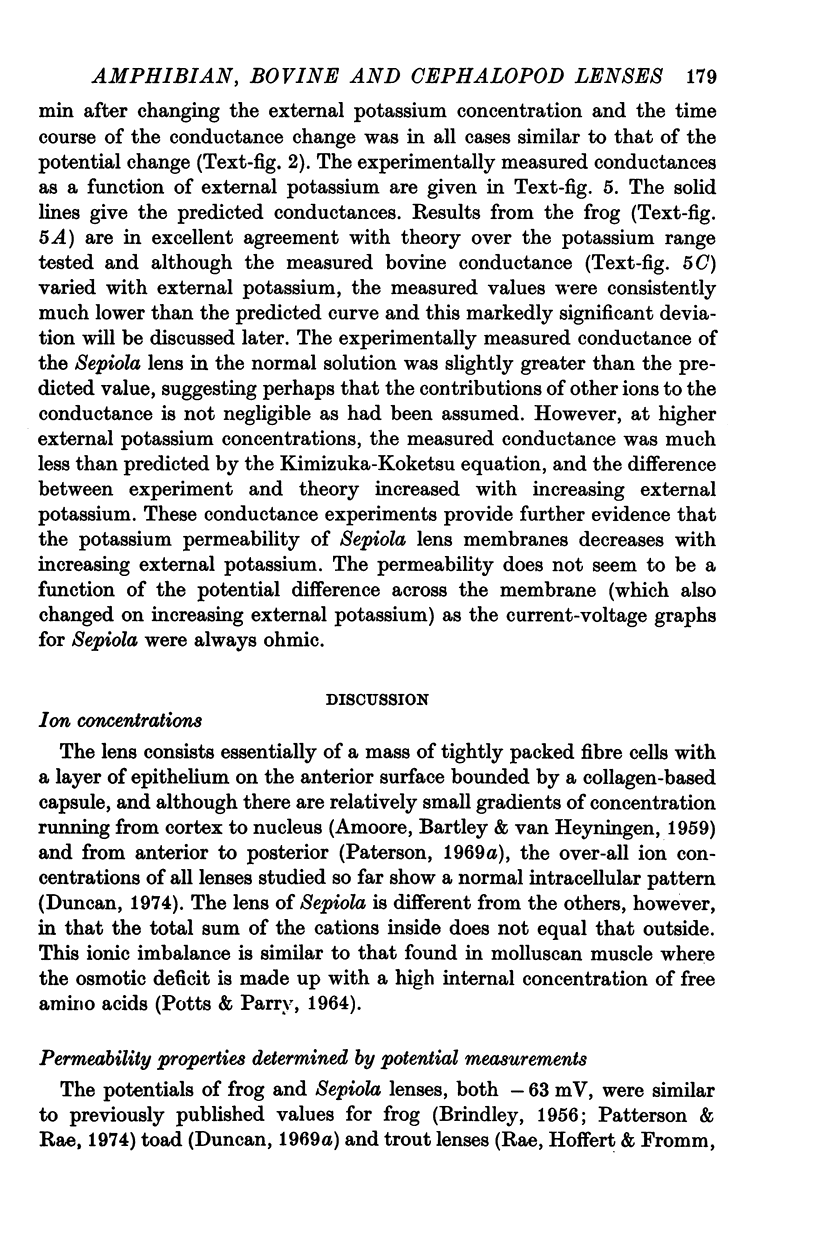
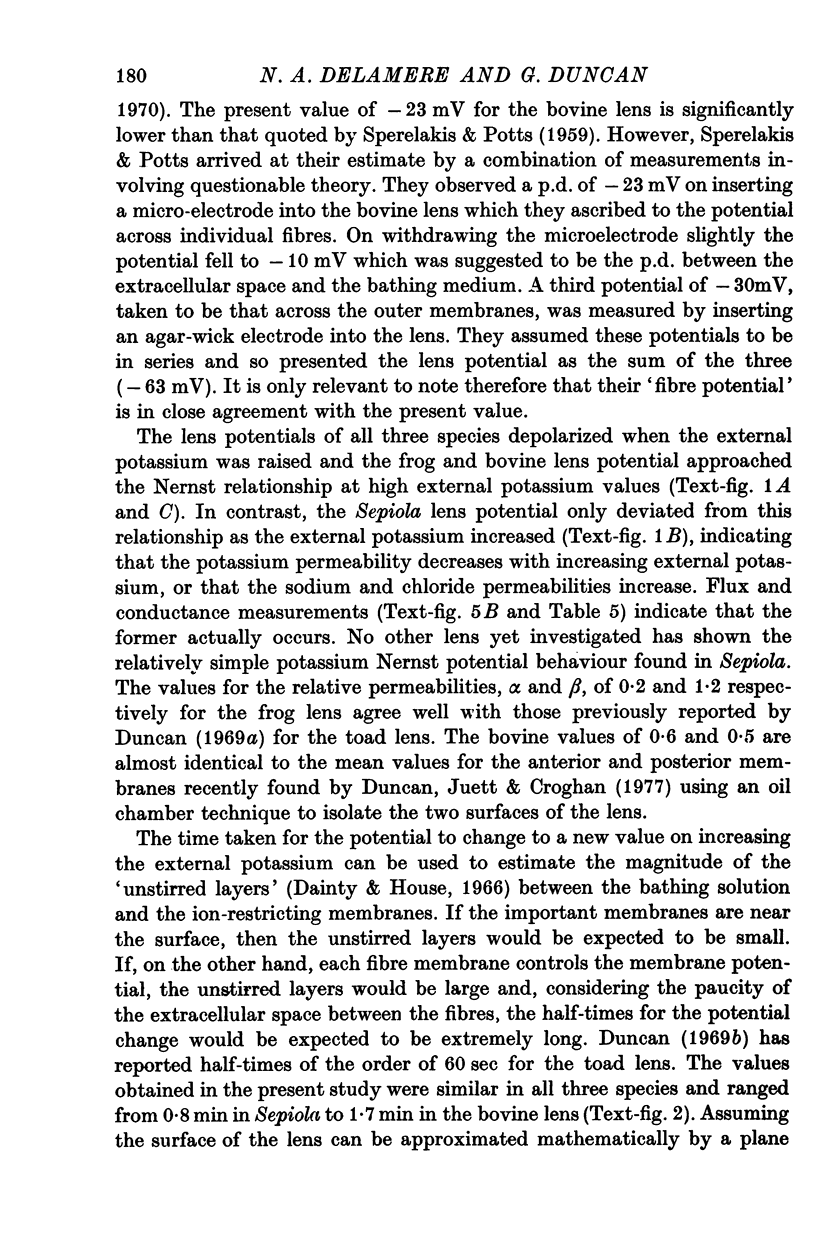
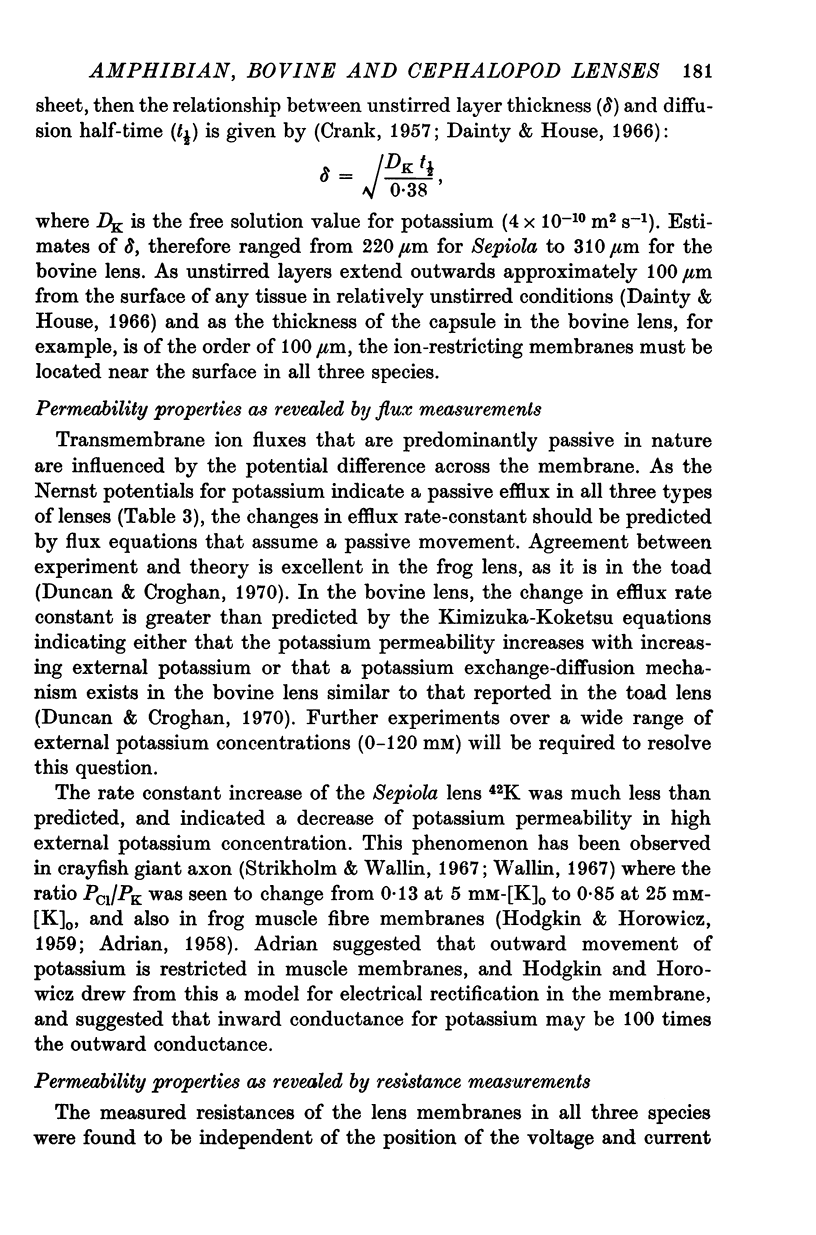
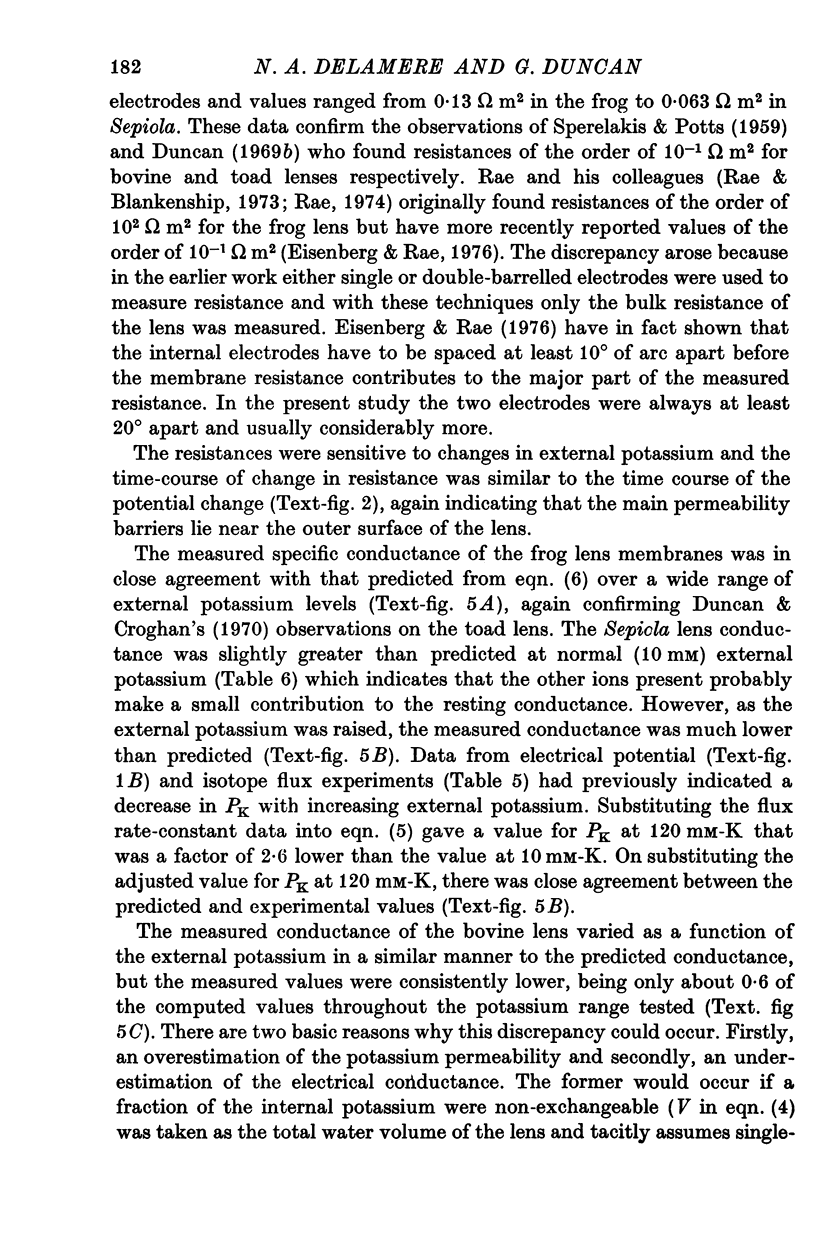
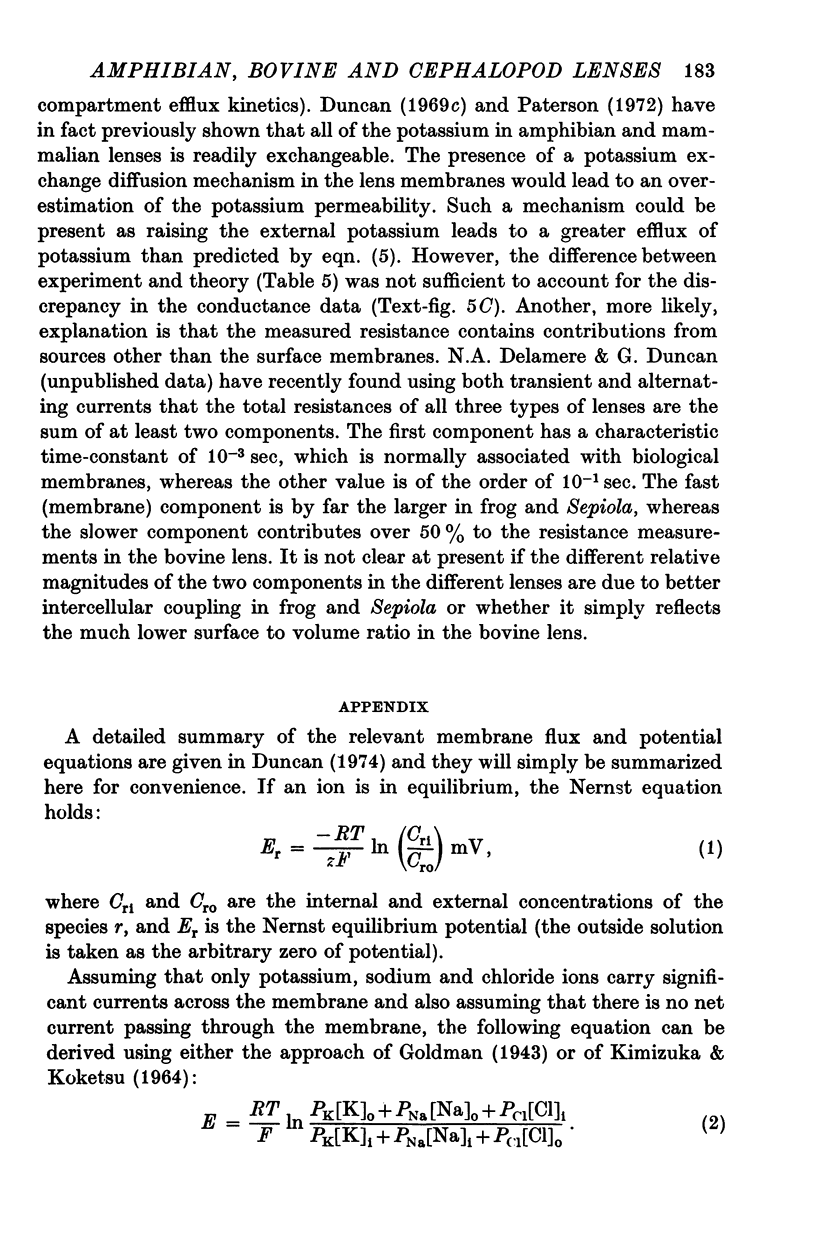
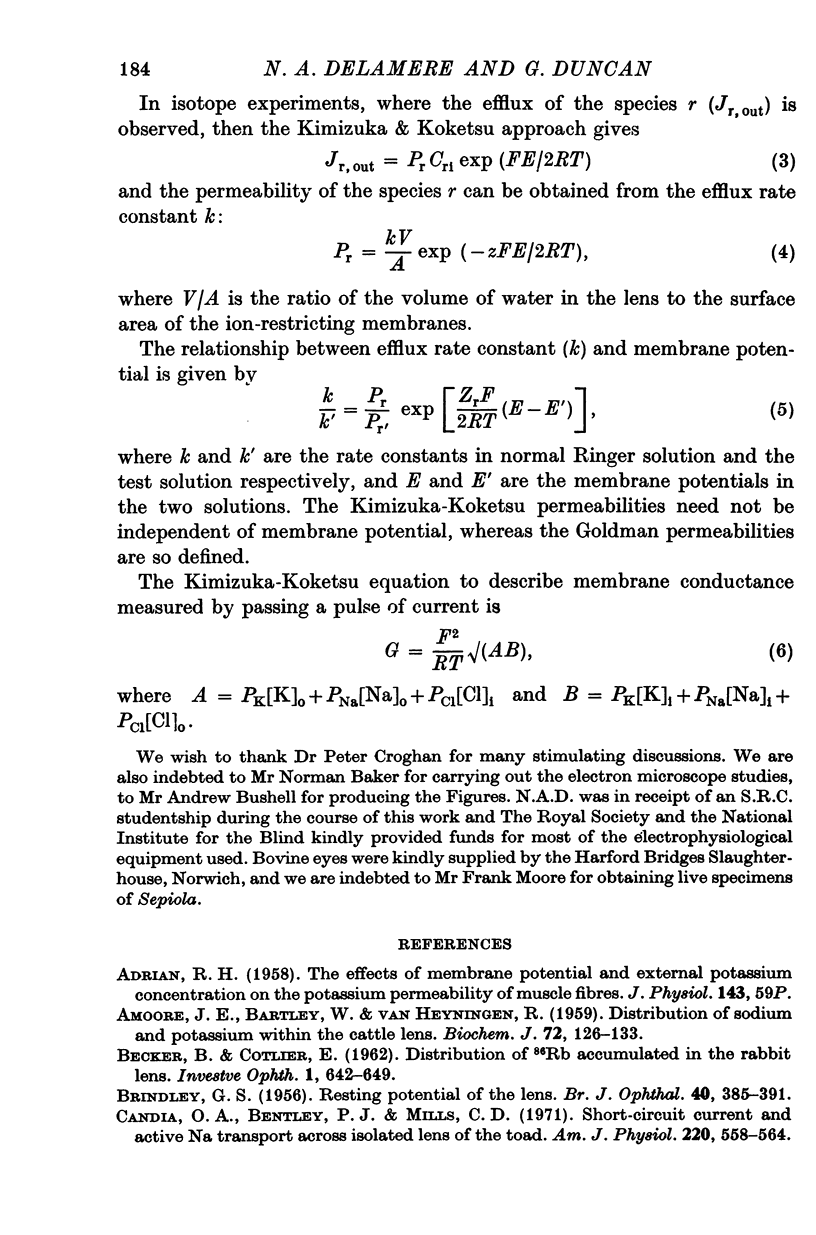
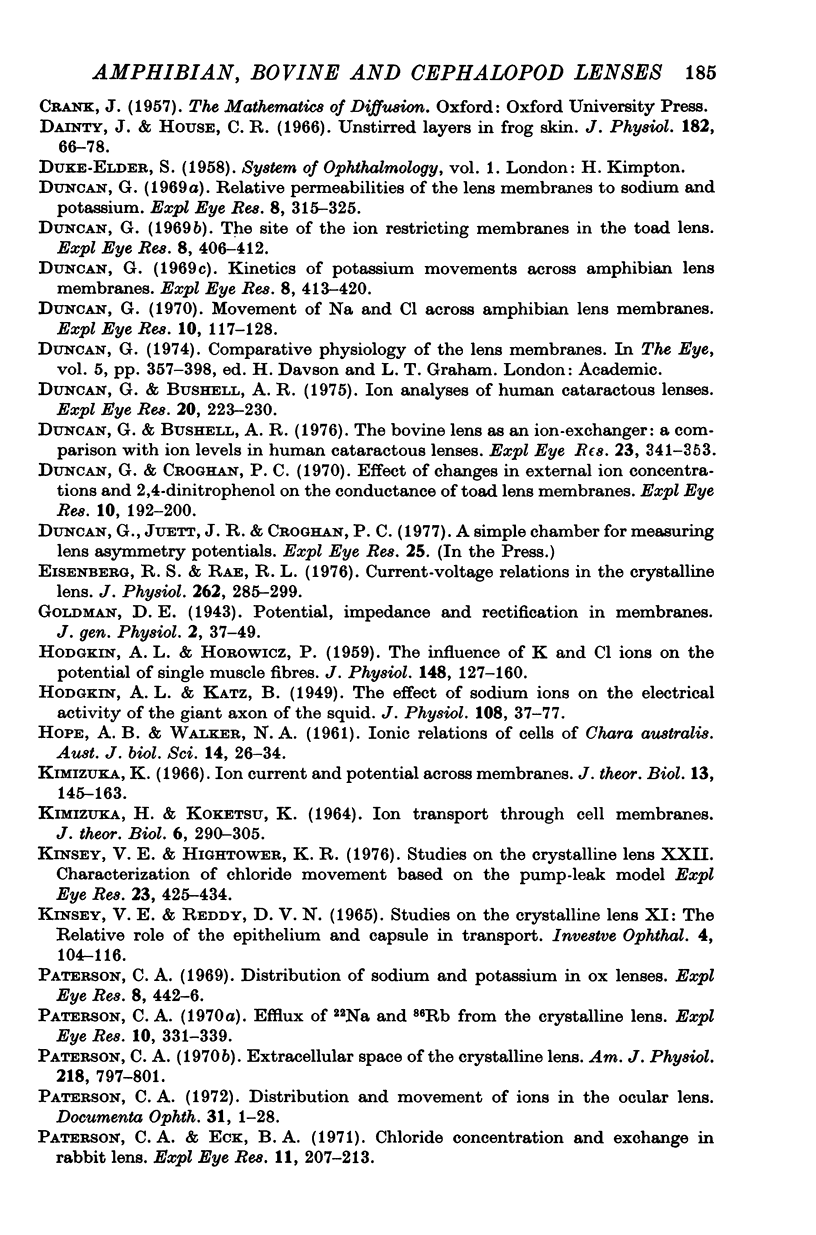
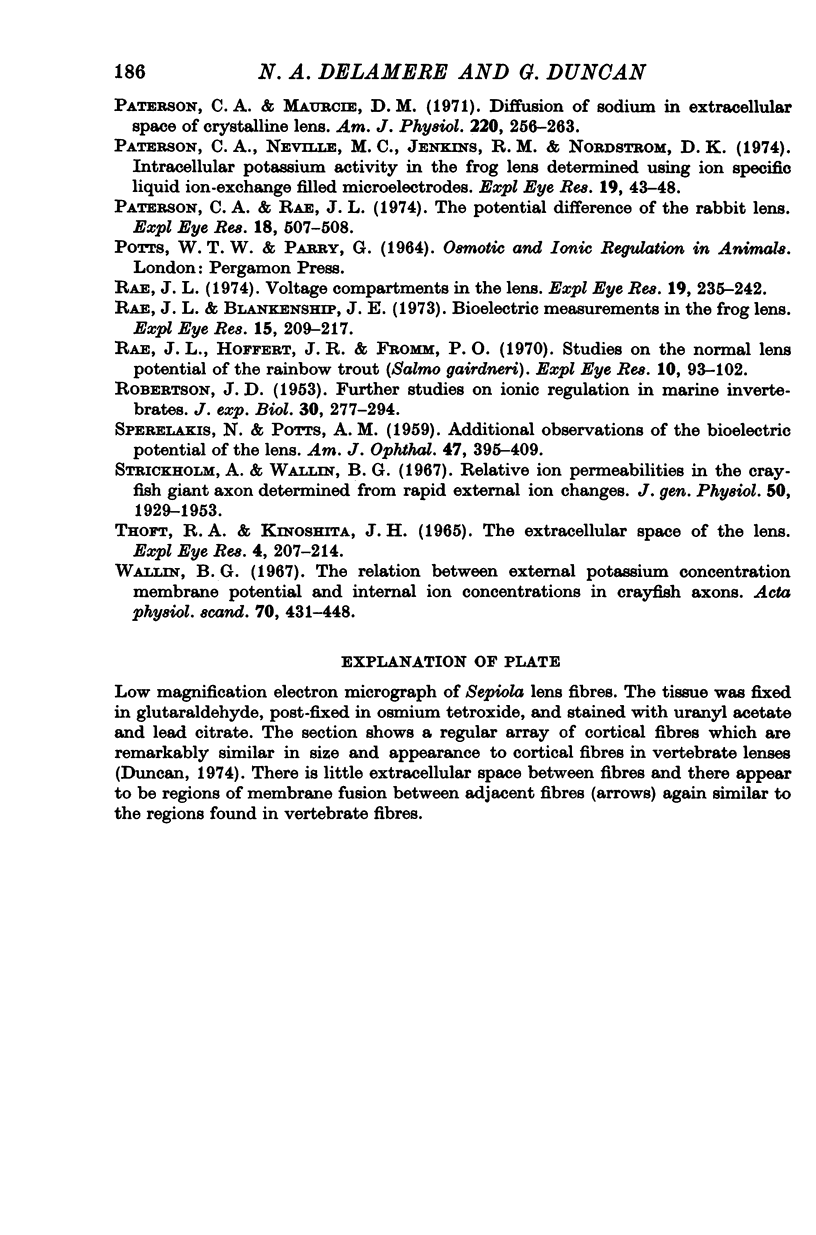
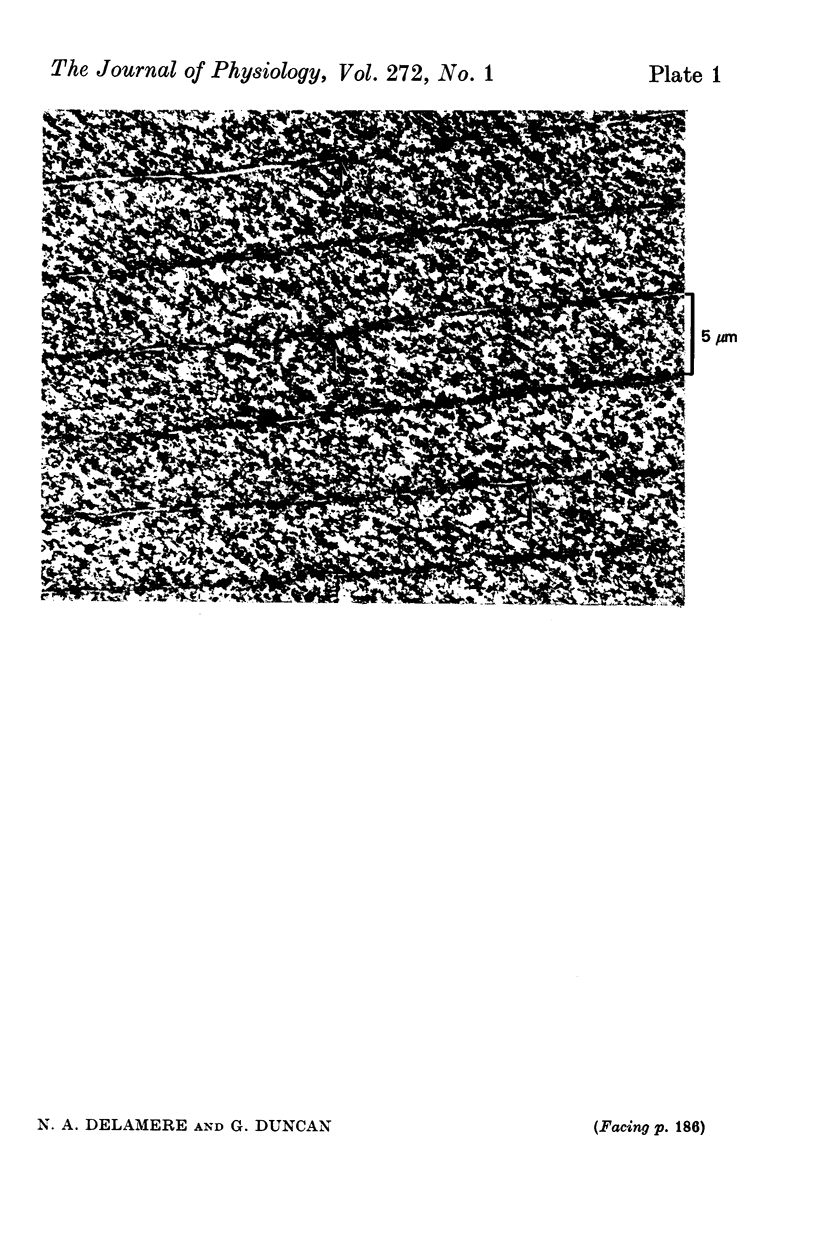
Images in this article
Selected References
These references are in PubMed. This may not be the complete list of references from this article.
- AMOORE J. E., BARTLEY W., VAN HEYNINGEN R. Distribution of sodium and potassium within cattle lens. Biochem J. 1959 May;72(1):126–133. doi: 10.1042/bj0720126. [DOI] [PMC free article] [PubMed] [Google Scholar]
- BECKER B., COTLIER E. Distribution of rubidium-86 accumulated in the rabbit lens. Invest Ophthalmol. 1962 Oct;1:642–645. [PubMed] [Google Scholar]
- BRINDLEY G. S. Resting potential of the lens. Br J Ophthalmol. 1956 Jul;40(7):385–391. doi: 10.1136/bjo.40.7.385. [DOI] [PMC free article] [PubMed] [Google Scholar]
- Candia O. A., Bentley P. J., Mills C. D. Short-circuit current and active Na transport across isolated lens of the toad. Am J Physiol. 1971 Feb;220(2):558–564. doi: 10.1152/ajplegacy.1971.220.2.558. [DOI] [PubMed] [Google Scholar]
- Dainty J., House C. R. Unstirred layers in frog skin. J Physiol. 1966 Jan;182(1):66–78. doi: 10.1113/jphysiol.1966.sp007809. [DOI] [PMC free article] [PubMed] [Google Scholar]
- Duncan G., Bushell A. R. Ion analyses of human cataractous lenses. Exp Eye Res. 1975 Mar;20(3):223–230. doi: 10.1016/0014-4835(75)90136-0. [DOI] [PubMed] [Google Scholar]
- Duncan G., Bushell A. R. The bovine lens as an ion-exchanger: a comparison with ion levels in human cataractous lenses. Exp Eye Res. 1976 Sep;23(3):341–353. doi: 10.1016/0014-4835(76)90133-0. [DOI] [PubMed] [Google Scholar]
- Duncan G., Croghan P. C. Effect of changes in external ion concentrations and 2,4-dinitrophenol on the conductance of toad lens membranes. Exp Eye Res. 1970 Oct;10(2):192–200. doi: 10.1016/s0014-4835(70)80027-6. [DOI] [PubMed] [Google Scholar]
- Duncan G. Kinetics of potassium movement across amphibian lens membranes. Exp Eye Res. 1969 Oct;8(4):413–420. doi: 10.1016/s0014-4835(69)80007-2. [DOI] [PubMed] [Google Scholar]
- Duncan G. Movement of sodium and chloride across amphibian lens membranes. Exp Eye Res. 1970 Jul;10(1):117–128. doi: 10.1016/s0014-4835(70)80017-3. [DOI] [PubMed] [Google Scholar]
- Duncan G. Relative permeabilities of the lens membranes to sodium and potassium. Exp Eye Res. 1969 Jul;8(3):315–325. doi: 10.1016/s0014-4835(69)80045-x. [DOI] [PubMed] [Google Scholar]
- Duncan G. The site of the ion restricting membranes in the toad lens. Exp Eye Res. 1969 Oct;8(4):406–412. doi: 10.1016/s0014-4835(69)80006-0. [DOI] [PubMed] [Google Scholar]
- Eisenberg R. S., Rae J. L. Current-voltage relationships in the crystalline lens. J Physiol. 1976 Nov;262(2):285–300. doi: 10.1113/jphysiol.1976.sp011596. [DOI] [PMC free article] [PubMed] [Google Scholar]
- Goldman D. E. POTENTIAL, IMPEDANCE, AND RECTIFICATION IN MEMBRANES. J Gen Physiol. 1943 Sep 20;27(1):37–60. doi: 10.1085/jgp.27.1.37. [DOI] [PMC free article] [PubMed] [Google Scholar]
- HODGKIN A. L., HOROWICZ P. The influence of potassium and chloride ions on the membrane potential of single muscle fibres. J Physiol. 1959 Oct;148:127–160. doi: 10.1113/jphysiol.1959.sp006278. [DOI] [PMC free article] [PubMed] [Google Scholar]
- HODGKIN A. L., KATZ B. The effect of sodium ions on the electrical activity of giant axon of the squid. J Physiol. 1949 Mar 1;108(1):37–77. doi: 10.1113/jphysiol.1949.sp004310. [DOI] [PMC free article] [PubMed] [Google Scholar]
- KINSEY V. E., REDDY D. V. STUDIES ON THE CRYSTALLINE LENS. XI. THE RELATIVE ROLE OF THE EPITHELIUM AND CAPSULE IN TRANSPORT. Invest Ophthalmol. 1965 Feb;4:104–116. [PubMed] [Google Scholar]
- Kimizuka H., Koketsu K. Ion transport through cell membrane. J Theor Biol. 1964 Mar;6(2):290–305. doi: 10.1016/0022-5193(64)90035-9. [DOI] [PubMed] [Google Scholar]
- Kinsey V. E., Hightower K. R. Studies on the crystalline lens. XXII. Characterization of chloride movement based on the pump-leak model. Exp Eye Res. 1976 Oct;23(4):425–433. doi: 10.1016/0014-4835(76)90171-8. [DOI] [PubMed] [Google Scholar]
- Paterson C. A. Distribution and movement of ions in the ocular lens. Doc Ophthalmol. 1972 Apr 15;31(1):1–28. doi: 10.1007/BF00167360. [DOI] [PubMed] [Google Scholar]
- Paterson C. A. Distribution of sodium and potassium in ox lenses. Exp Eye Res. 1969 Oct;8(4):442–446. doi: 10.1016/s0014-4835(69)80011-4. [DOI] [PubMed] [Google Scholar]
- Paterson C. A., Eck B. A. Chloride concentration and exchange in rabbit lens. Exp Eye Res. 1971 Mar;11(2):207–213. doi: 10.1016/s0014-4835(71)80024-6. [DOI] [PubMed] [Google Scholar]
- Paterson C. A. Efflux of 22Na and 86Rb from the crystalline lens. Exp Eye Res. 1970 Oct;10(2):331–338. doi: 10.1016/s0014-4835(70)80045-8. [DOI] [PubMed] [Google Scholar]
- Paterson C. A. Extracellular space of the crystalline lens. Am J Physiol. 1970 Mar;218(3):797–802. doi: 10.1152/ajplegacy.1970.218.3.797. [DOI] [PubMed] [Google Scholar]
- Paterson C. A., Maurice D. M. Diffusion of sodium in extracellular space of the crystalline lens. Am J Physiol. 1971 Jan;220(1):256–263. doi: 10.1152/ajplegacy.1971.220.1.256. [DOI] [PubMed] [Google Scholar]
- Paterson C. A., Neville M. C., Jenkins R. M., 2nd, Nordstrom D. K. Intracellular potassium activity in frog lens determined using ion specific liquid ion-exchanger filled microelectrodes. Exp Eye Res. 1974 Jul;19(1):43–48. doi: 10.1016/0014-4835(74)90070-0. [DOI] [PubMed] [Google Scholar]
- Paterson C. A., Rae J. L. Letter: The potential difference of the rabbit lens. Exp Eye Res. 1974 May;18(5):507–508. doi: 10.1016/0014-4835(74)90087-6. [DOI] [PubMed] [Google Scholar]
- Rae J. L., Blankenship J. E. Bioelectric measurements in the frog lens. Exp Eye Res. 1973 Feb;15(2):209–217. doi: 10.1016/0014-4835(73)90121-8. [DOI] [PubMed] [Google Scholar]
- Rae J. L., Hoffert J. R., Fromm P. O. Studies on the normal lens potential of the rainbow trout (Salmo gairdneri). Exp Eye Res. 1970 Jul;10(1):93–101. doi: 10.1016/s0014-4835(70)80015-x. [DOI] [PubMed] [Google Scholar]
- Rae J. L. Voltage compartments in the lens. Exp Eye Res. 1974 Sep;19(3):235–242. doi: 10.1016/0014-4835(74)90142-0. [DOI] [PubMed] [Google Scholar]
- SPERELAKIS N., POTTS A. M. Additional observations on the bioelectric potentials of the lens. Am J Ophthalmol. 1959 Jan;47(1 Pt 2):395–409. doi: 10.1016/s0002-9394(14)78046-8. [DOI] [PubMed] [Google Scholar]
- Strickholm A., Wallin B. G. Relative ion permeabilities in the crayfish giant axon determined from rapid external ion changes. J Gen Physiol. 1967 Aug;50(7):1929–1953. doi: 10.1085/jgp.50.7.1929. [DOI] [PMC free article] [PubMed] [Google Scholar]
- Wallin B. G. The relation between external potassium concentration, membrane potential and internal ion concentrations in crayfish axons. Acta Physiol Scand. 1967 Jul-Aug;70(3):431–448. doi: 10.1111/j.1748-1716.1967.tb03641.x. [DOI] [PubMed] [Google Scholar]