Abstract
1. The opercular epithelium lining the inside of the gill chamber of the killifish, Fundulus heteroclitus, contains Cl- cells, identical in fine structure to gill Cl- cells, at the high density of 4 × 105 cells/cm2. This epithelium can be isolated, mounted in a Lucite chamber, and its ion transport properties studied with the short-circuit current technique.
2. The isolated opercular epithelia of seawater-adapted fish, when bathed on both sides with Ringer and gassed with 100% O2, displayed a mean short-circuit current of 136.5 ± 11.1 μA/cm2, a mean transepithelial potential difference of 18.7 ± 1.2 mV (blood side positive), and a mean transepithelial d.c. resistance of 173.7 ± 12.1 Ω.cm2 (mean ± S.E. of mean; n = 64).
3. The transepithelial potential difference across the opercular epithelia of seawater-adapted fish was dependent on both Na+ and Cl- in the bathing solutions and increased linearly with increasing Cl- concentrations with a slope of 28.3 ± 2.1 mV/tenfold concentration change. The short-circuit current was Na+ dependent and increased linearly with increasing Cl- concentrations with no evidence of saturation kinetics below 142.5 m-equiv/l.
4. When the short-circuited epithelia of seawater-adapted fish, bathed on both sides with Ringer, was gassed with 100% O2 the mean Cl- blood side to seawater side flux was 211.7 ± 27.1 μA/cm2 and the mean Cl- seawater side to blood side flux was 48.9 ± 10.0 μA/cm2. This resulted in a net Cl- blood side to seawater side flux of 162.8 μA/cm2 which was not statistically different (P > 0.70) from the mean short-circuit current of 158.6 ± 16.3 μA/cm2 for these flux studies. The mean Na+ blood side to seawater side flux was 32.2 ± 3.3 μA/cm2 and the mean Na+ seawater side to blood side flux was 34.8 ± 4.1 μA/cm2, resulting in no significant (P > 0.20) net flux of this cation. Similar results were obtained with short-circuited epithelia of seawater-adapted fish when bathed on both sides with Ringer and gassed with 95% O2/5% CO2.
5. Ouabain (10-5 M), furosemide (10-3 M), thiocyanate (10-2 M), adrenaline (10-6 M), and anoxia (100% N2) decreased the short-circuit current 92.7, 85.0, 45.3, 62.6, and 83.3% respectively. Theophylline (10-4 M) stimulated the short-circuit current 54.9%. Increasing the HCO3- concentration in the bathing solutions had a stimulatory effect on the short-circuit current and the potential difference across epithelia from seawater-adapted fish.
6. The opercular epithelia of freshwater-adapted F. heteroclitus, when bathed on both sides with Ringer, displayed a mean short-circuit current of 94.1 ± 10.4 μA/cm2, a mean transepithelial potential difference of 14.8 ± 1.9 mV (blood side positive), and a mean d.c. resistance of 169.0 ± 14.0 Ω.cm2 (mean ± S.E. of mean; n = 20). Isotope flux studies across these short-circuited epithelia revealed a net Cl- blood side to freshwater side flux of 95.2 ± 16.1 μA/cm2 and no significant net flux of Na+.
7. The opercular epithelia of 200% seawater-adapted F. heteroclitus, when bathed on both sides with Ringer, displayed a mean short-circuit current of 33.5 ± 8.5 μA/cm2, a mean transepithelial potential difference of 10.5 ± 2.5 mV (blood side positive), and a mean transepithelial d.c. resistance of 440.7 ± 62.6 Ω.cm2 (mean ± S.E. of mean n = 18). Isotope flux studies across these short-circuited epithelia revealed a net Cl- blood side to seawater side flux of 96.2 ± 51.5 μA/cm2 and a net Na+ blood side to seawater side flux of 65.3 ± 28.6 μA/cm2.
Full text
PDF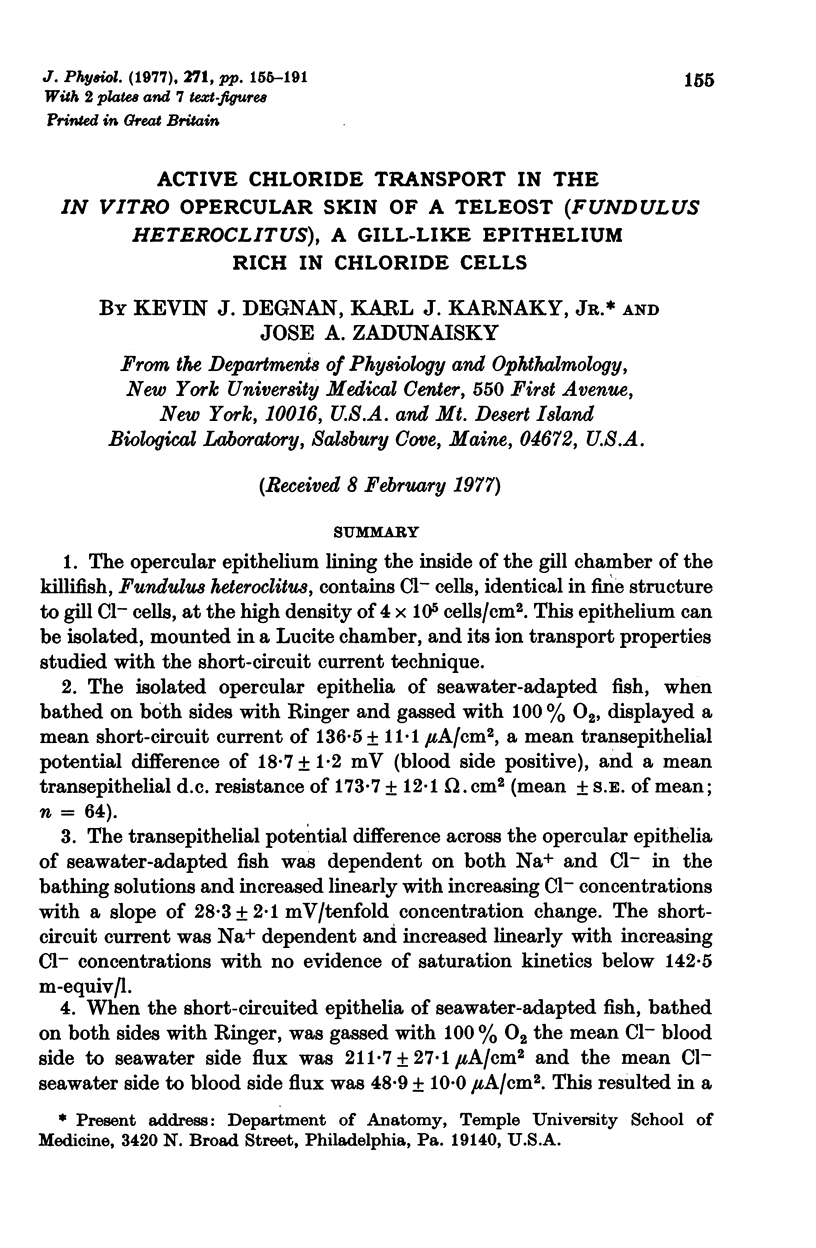
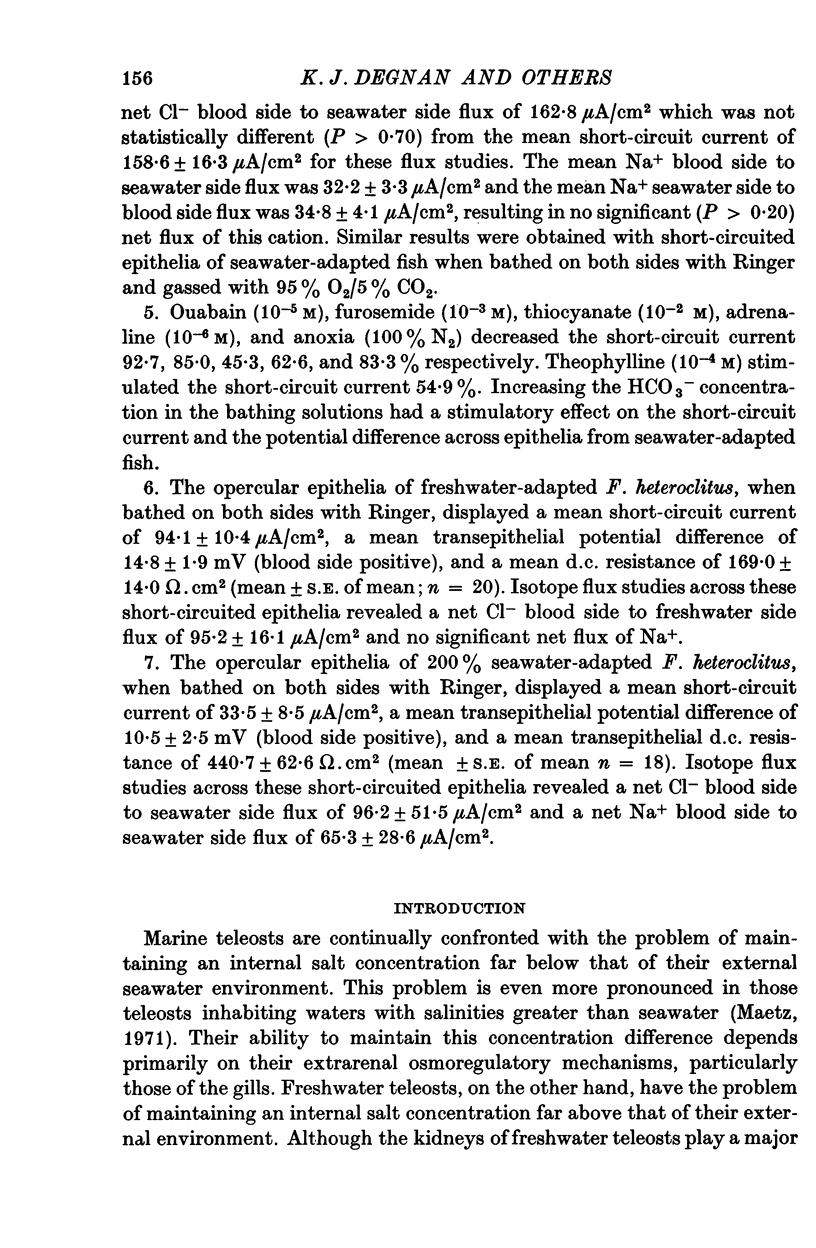
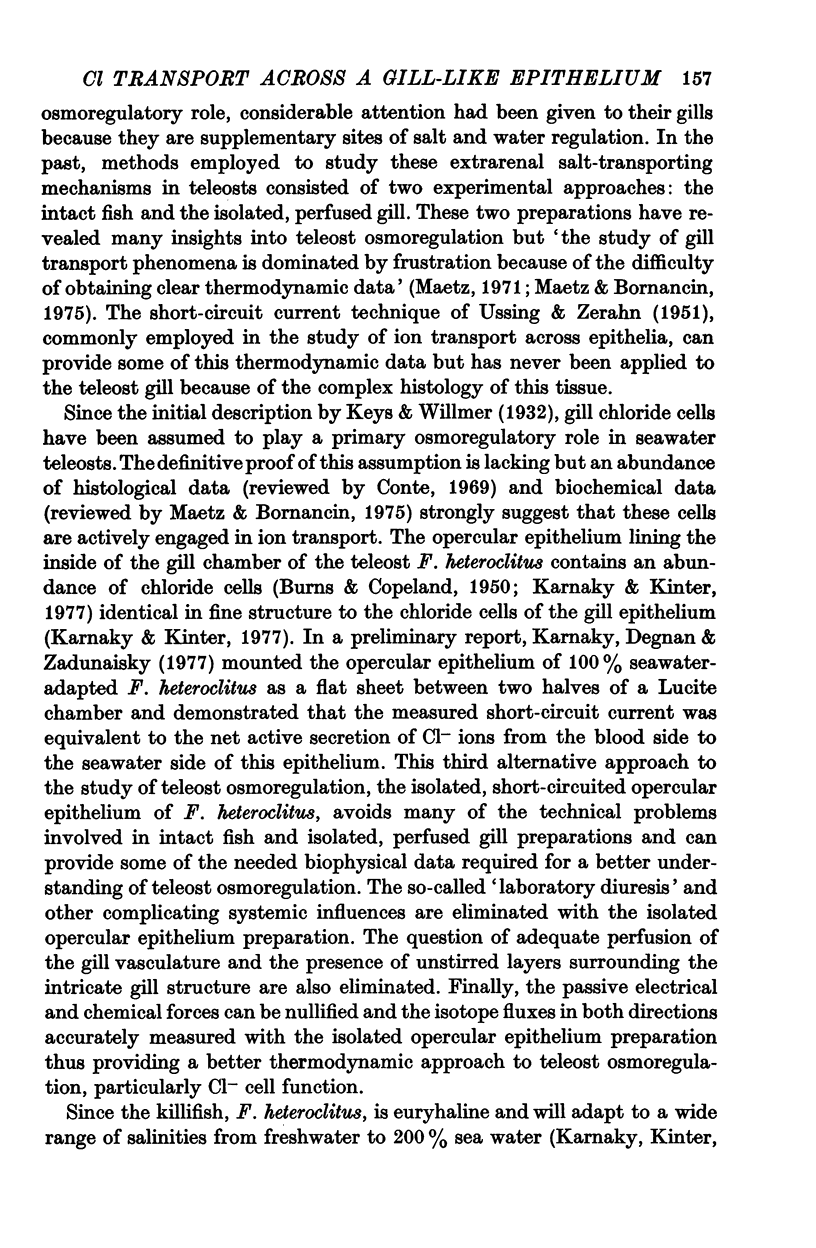
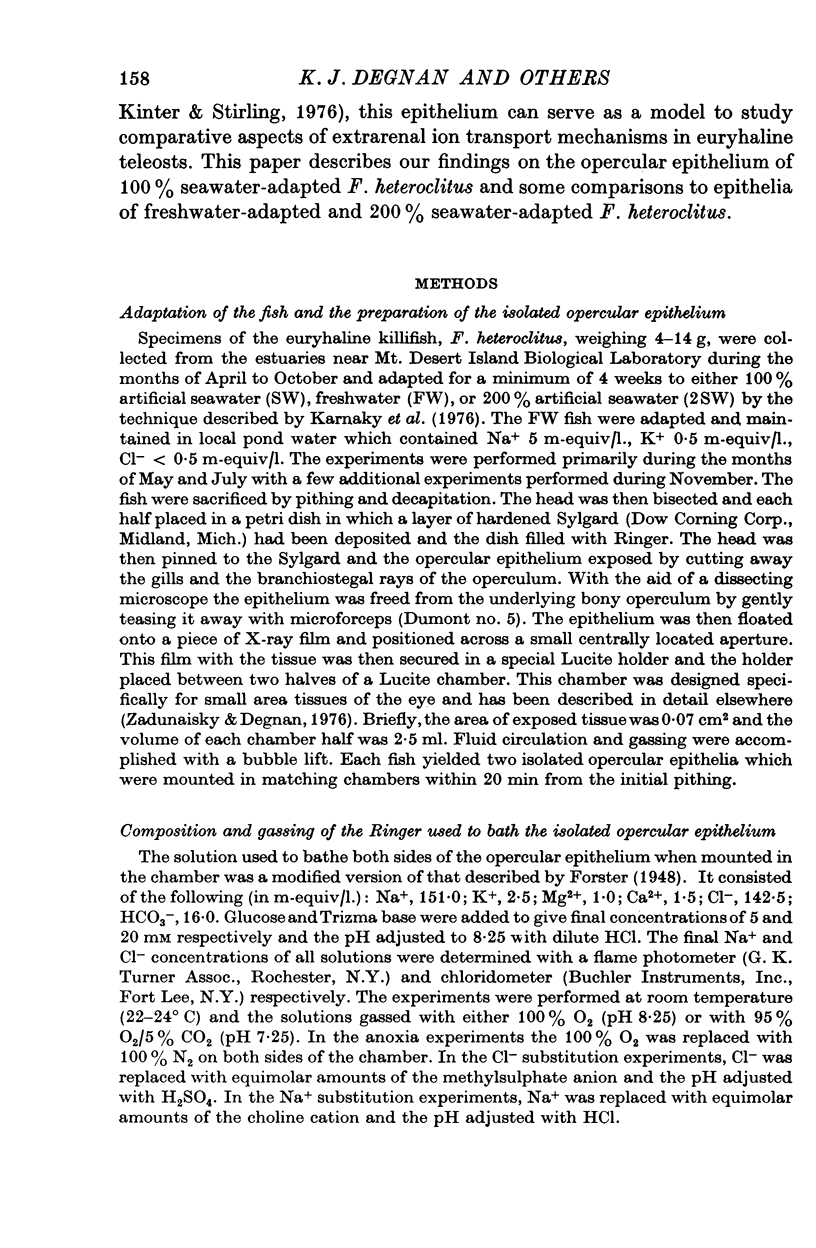
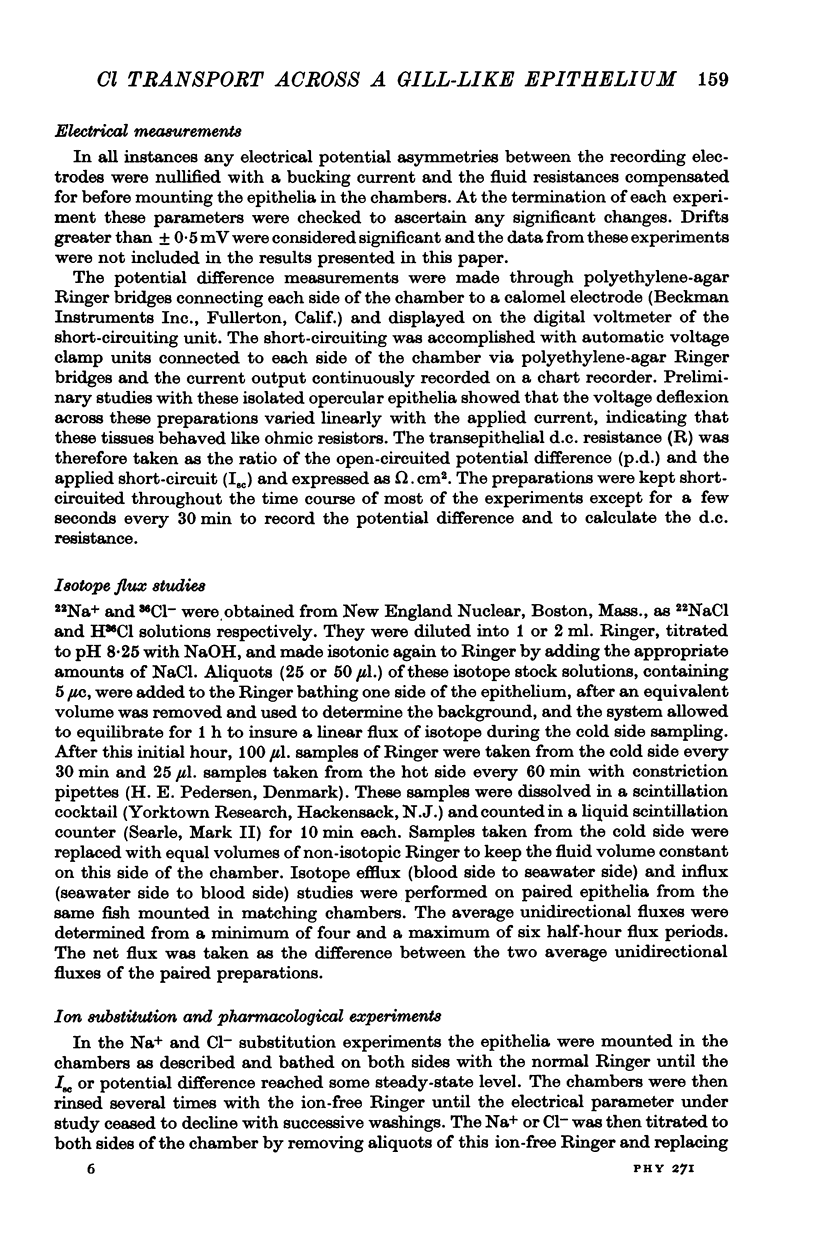
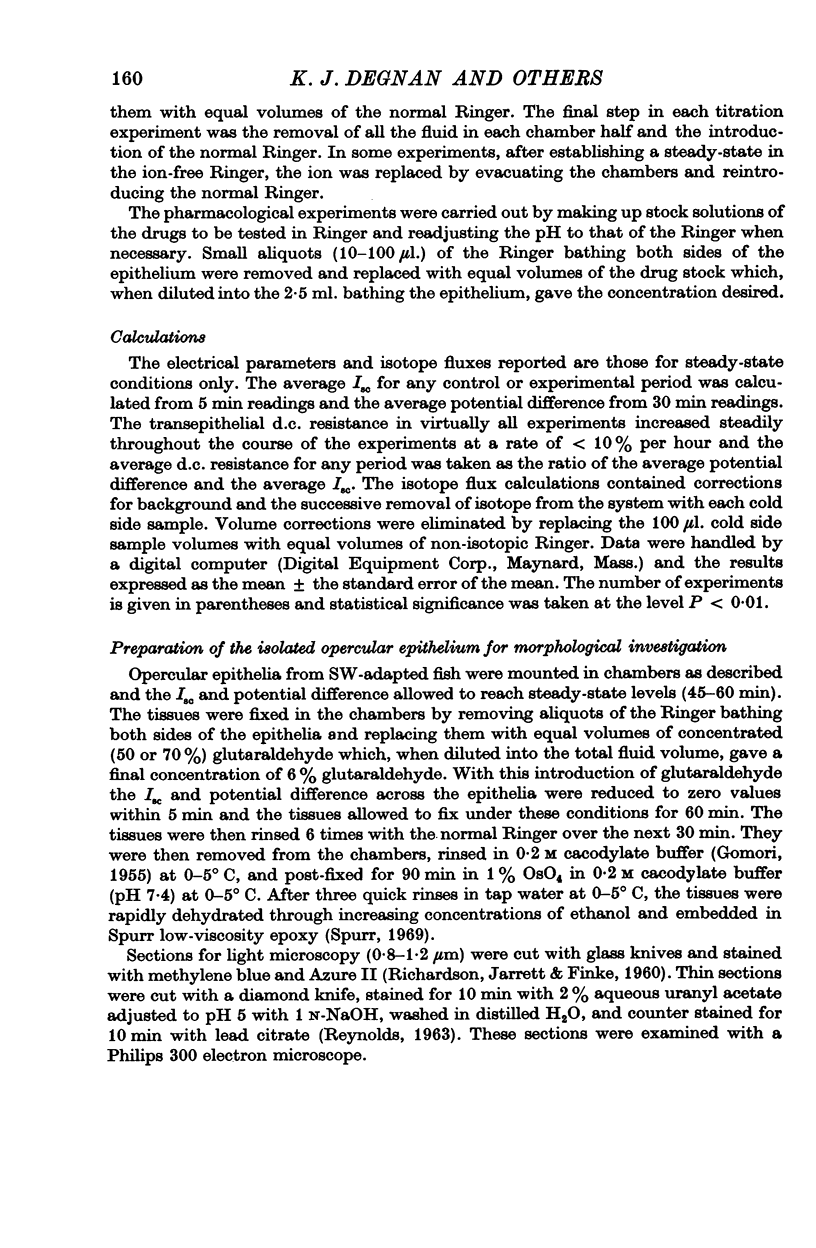
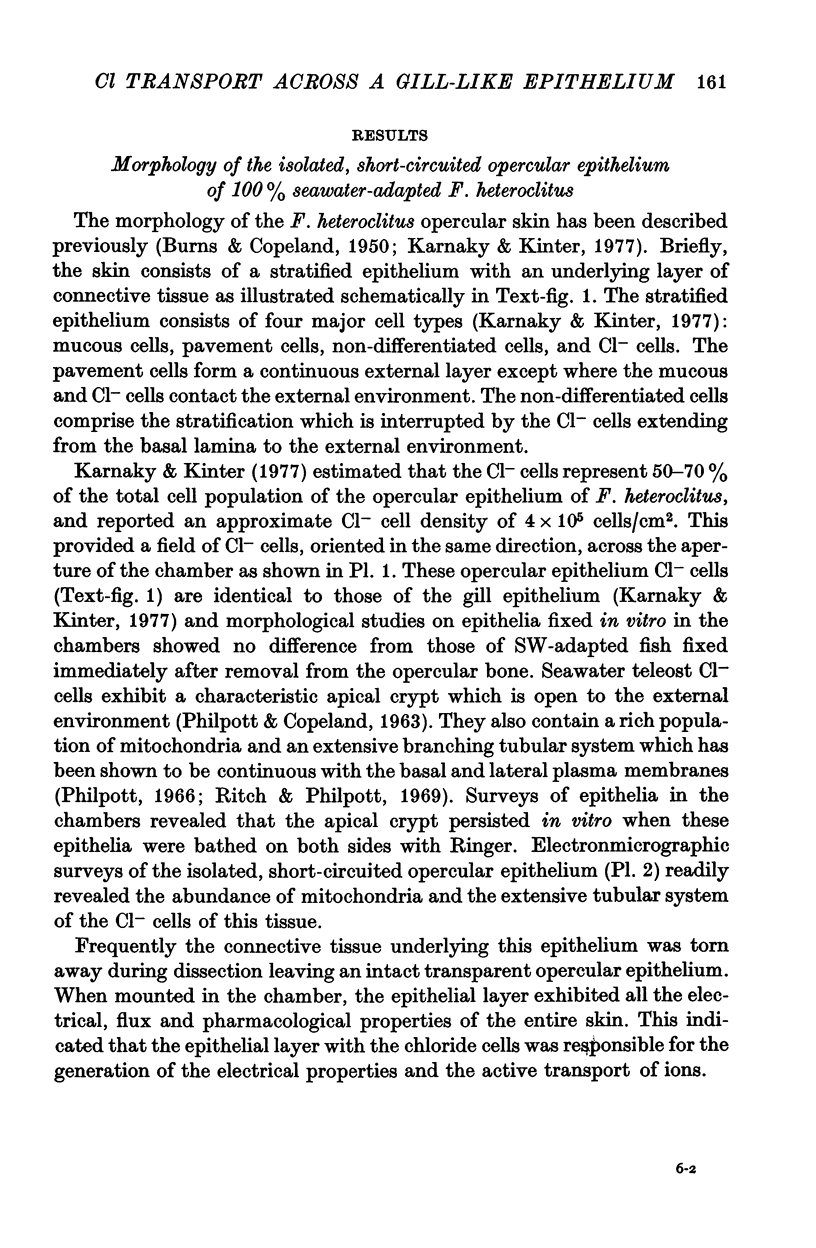
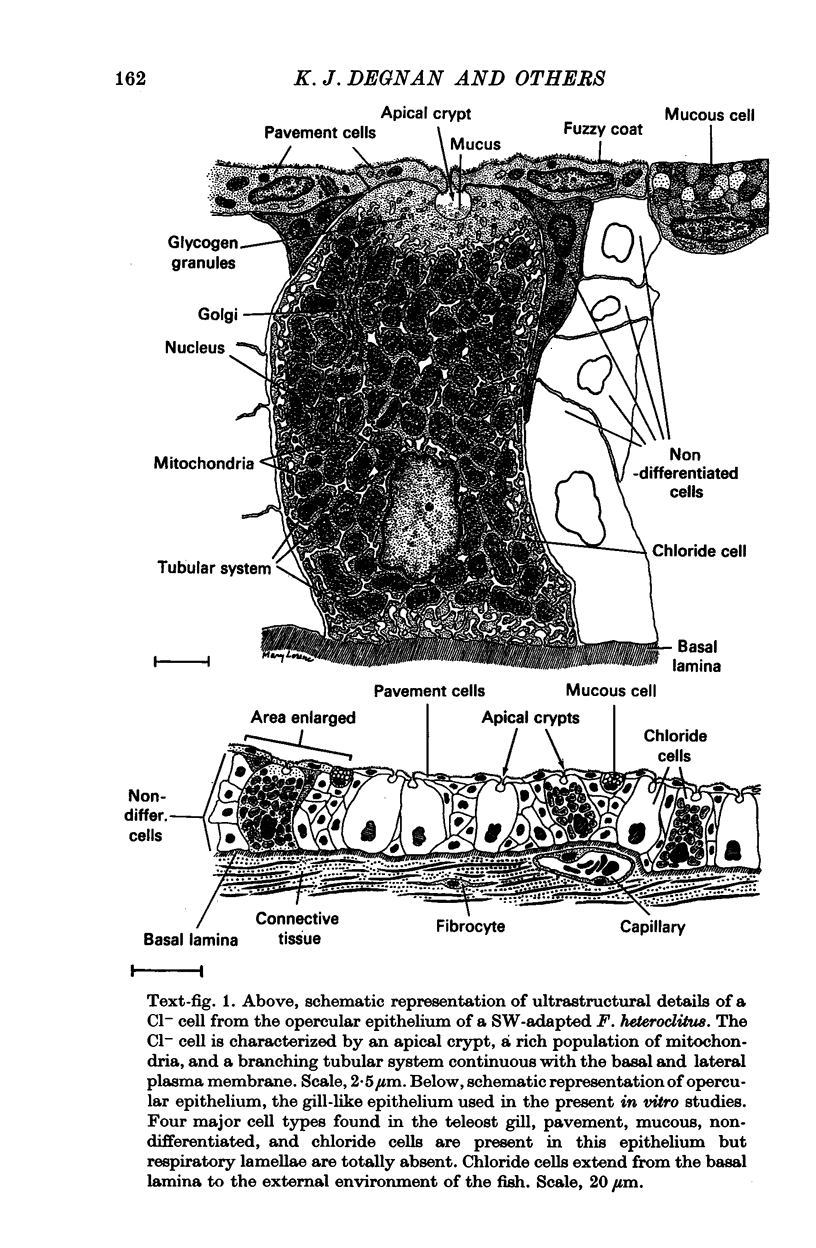
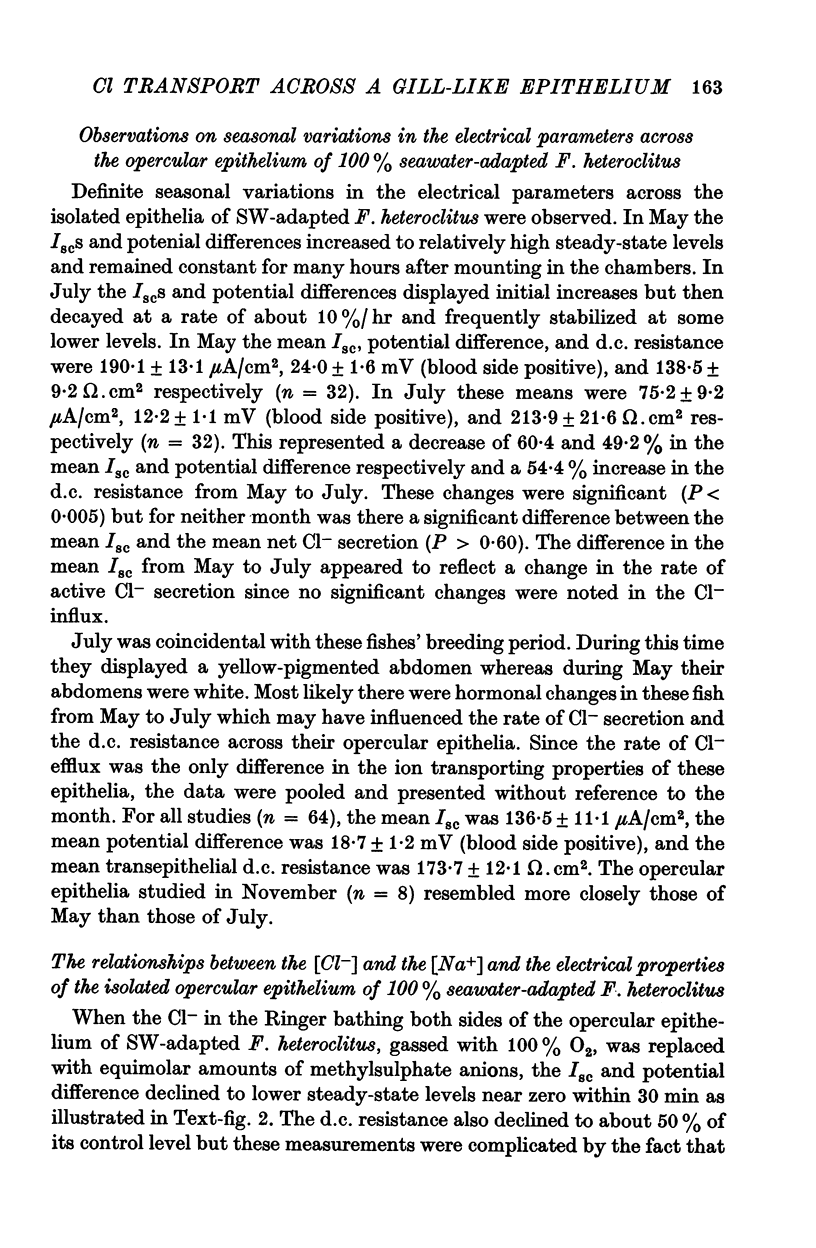
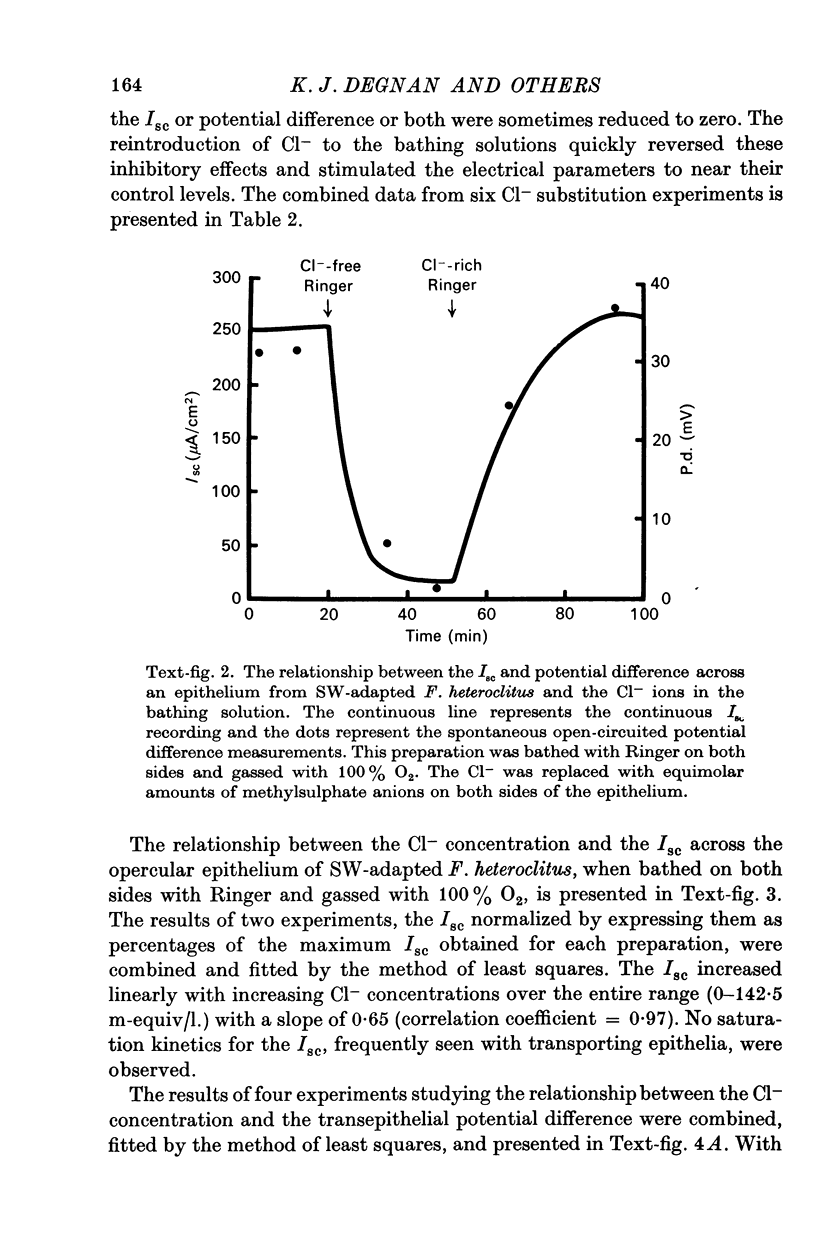
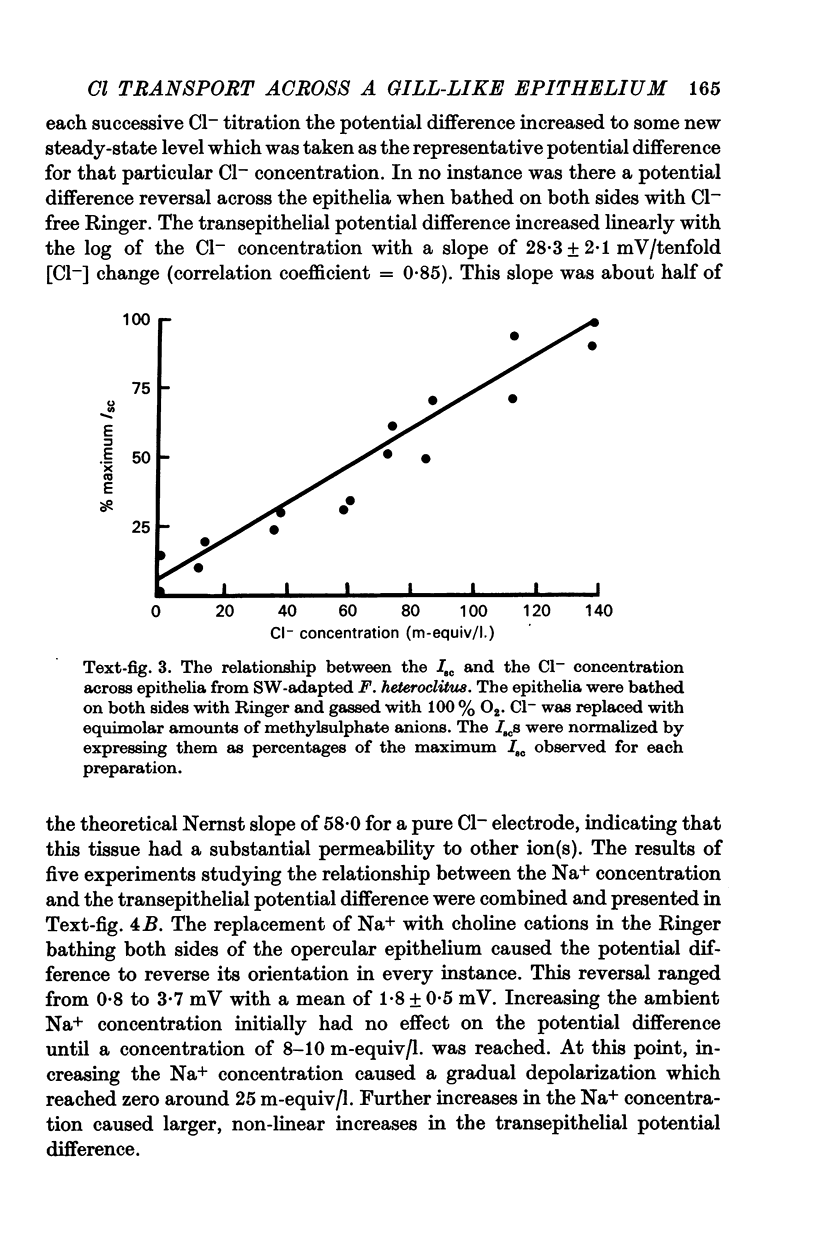
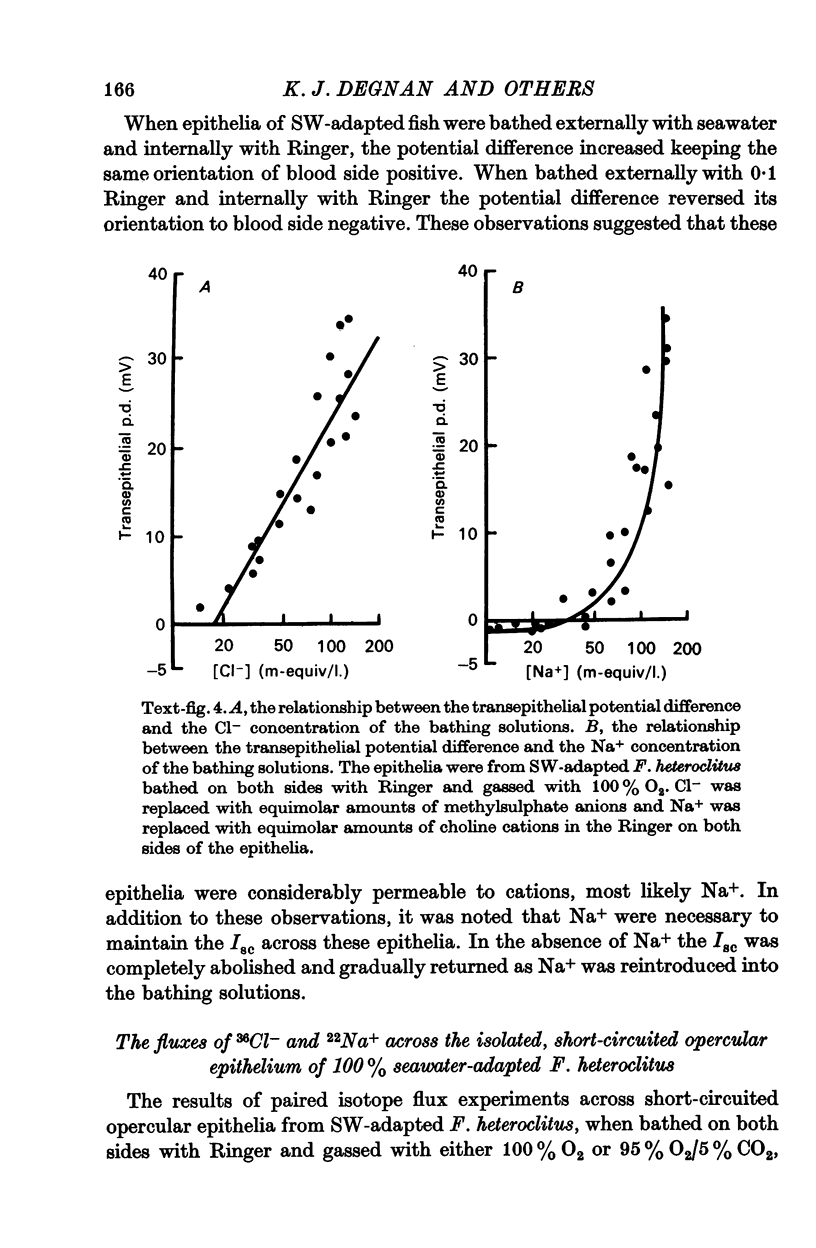
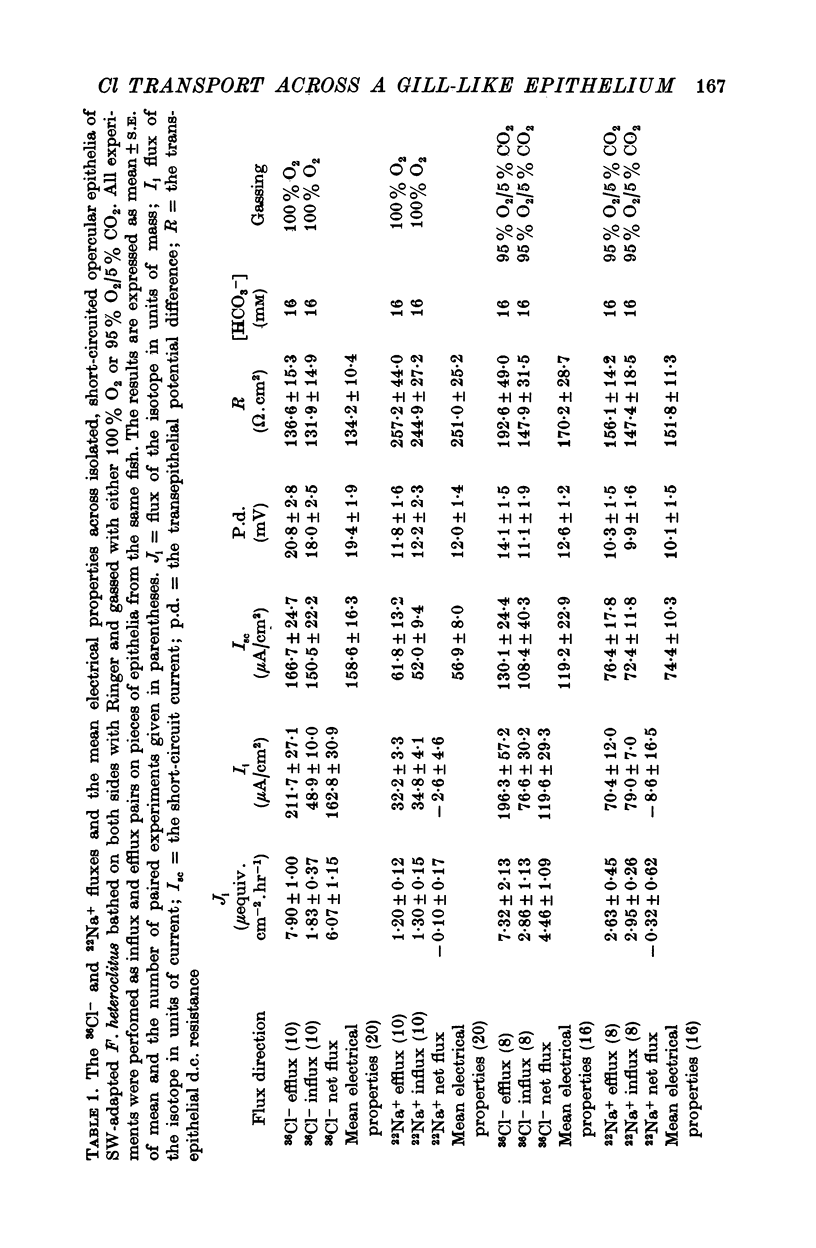
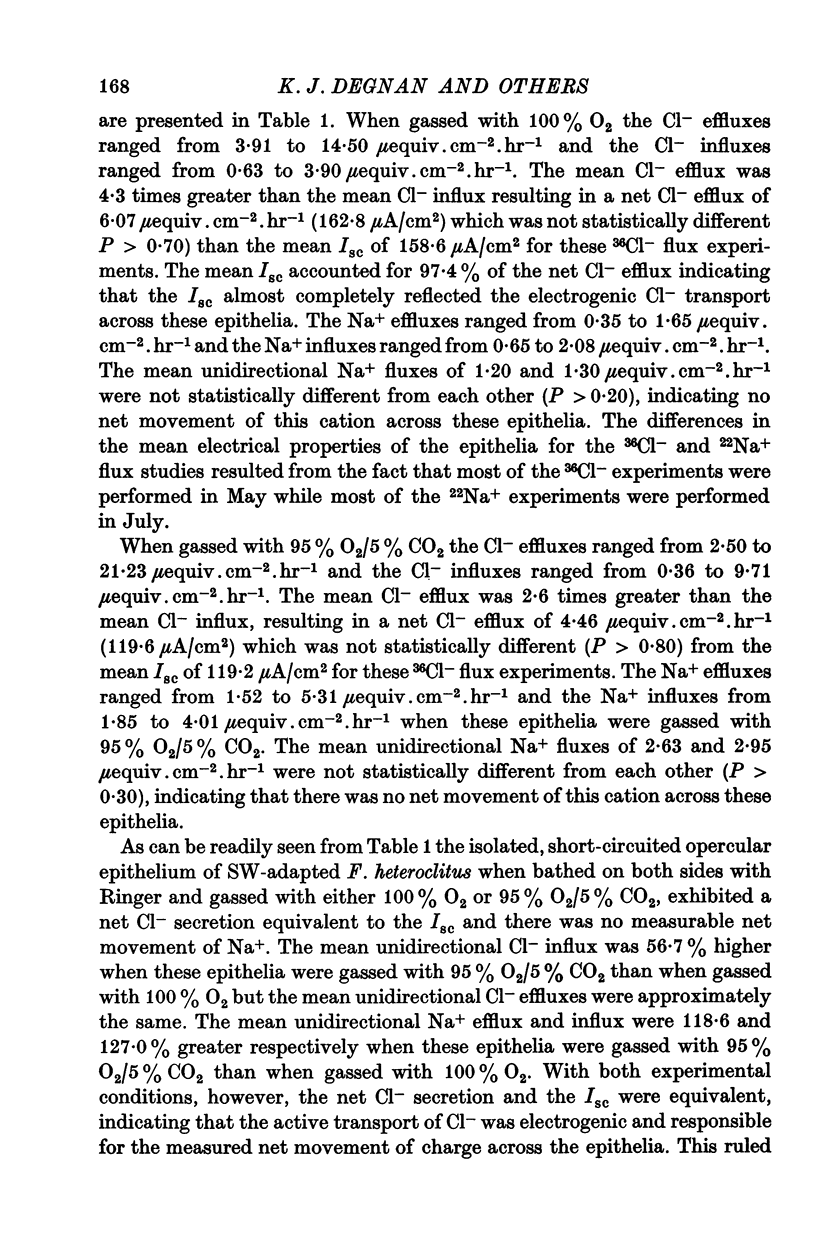
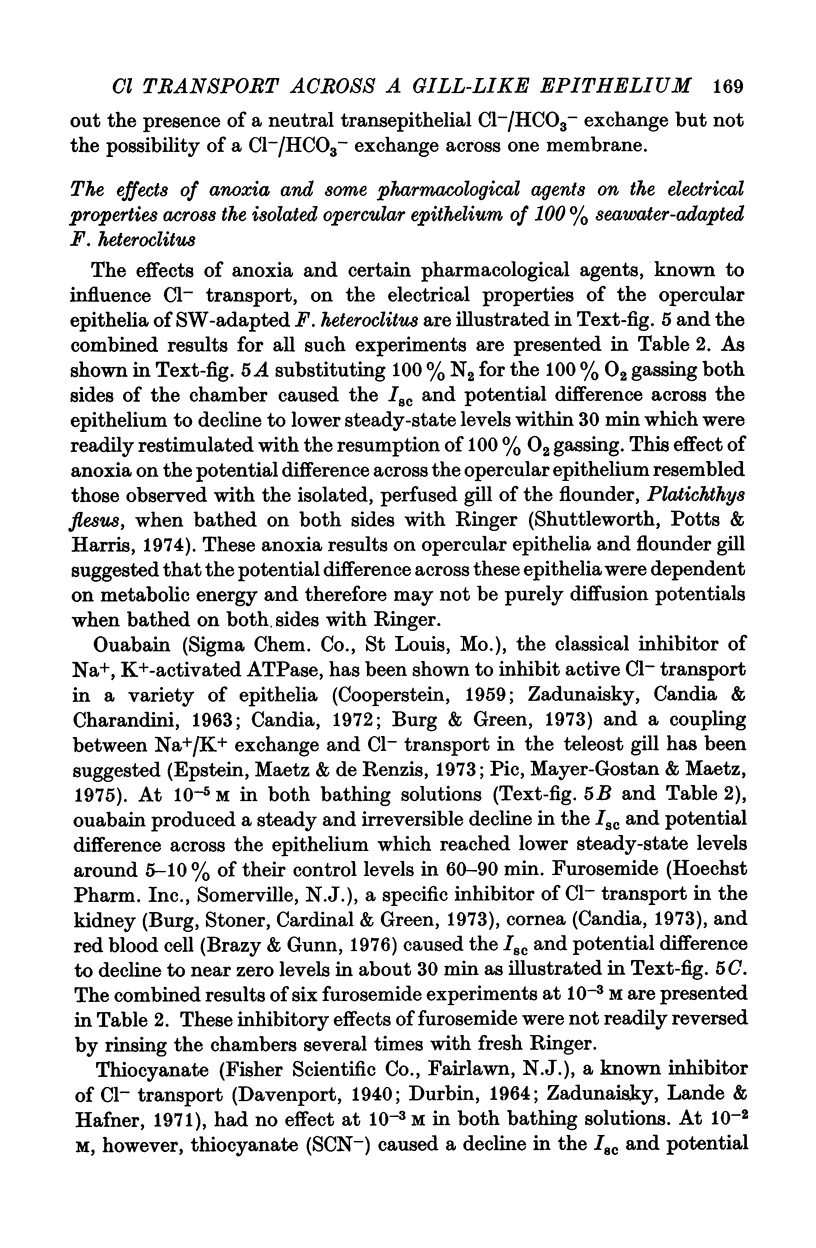
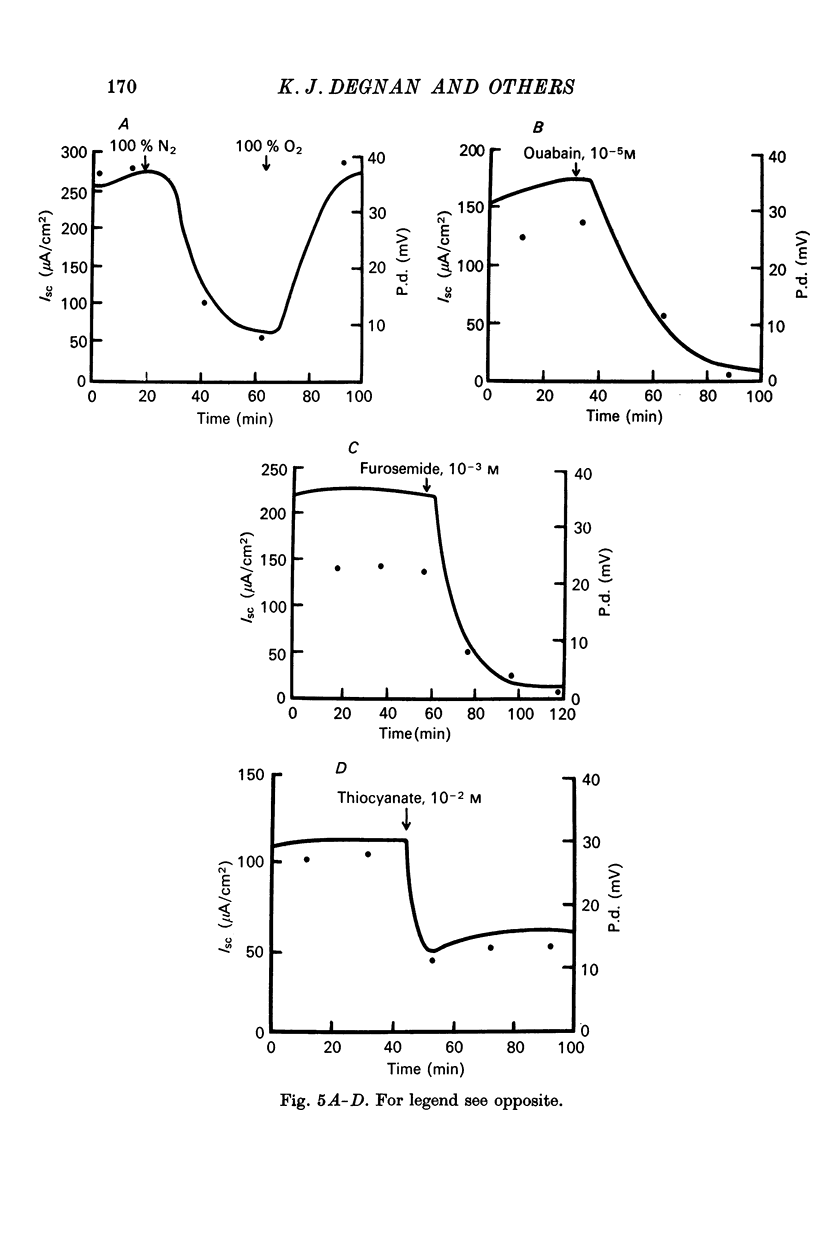
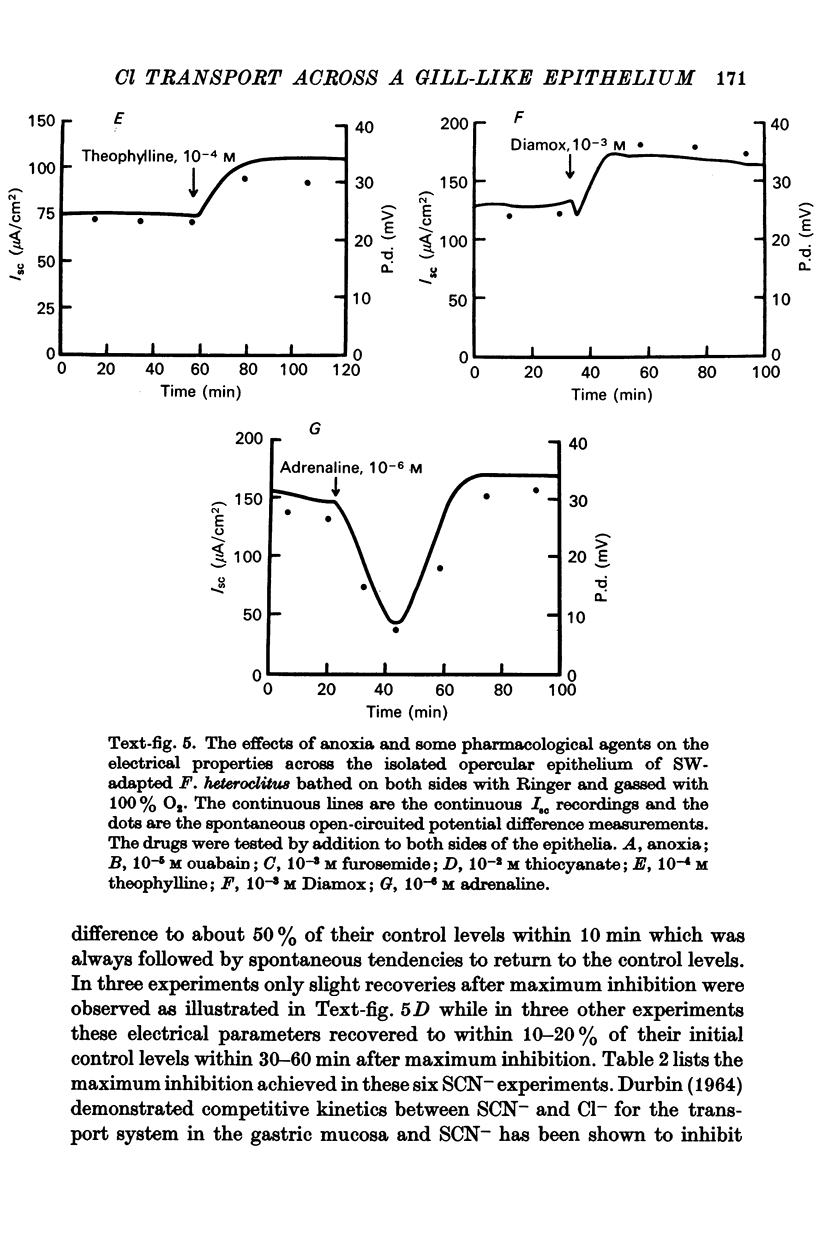
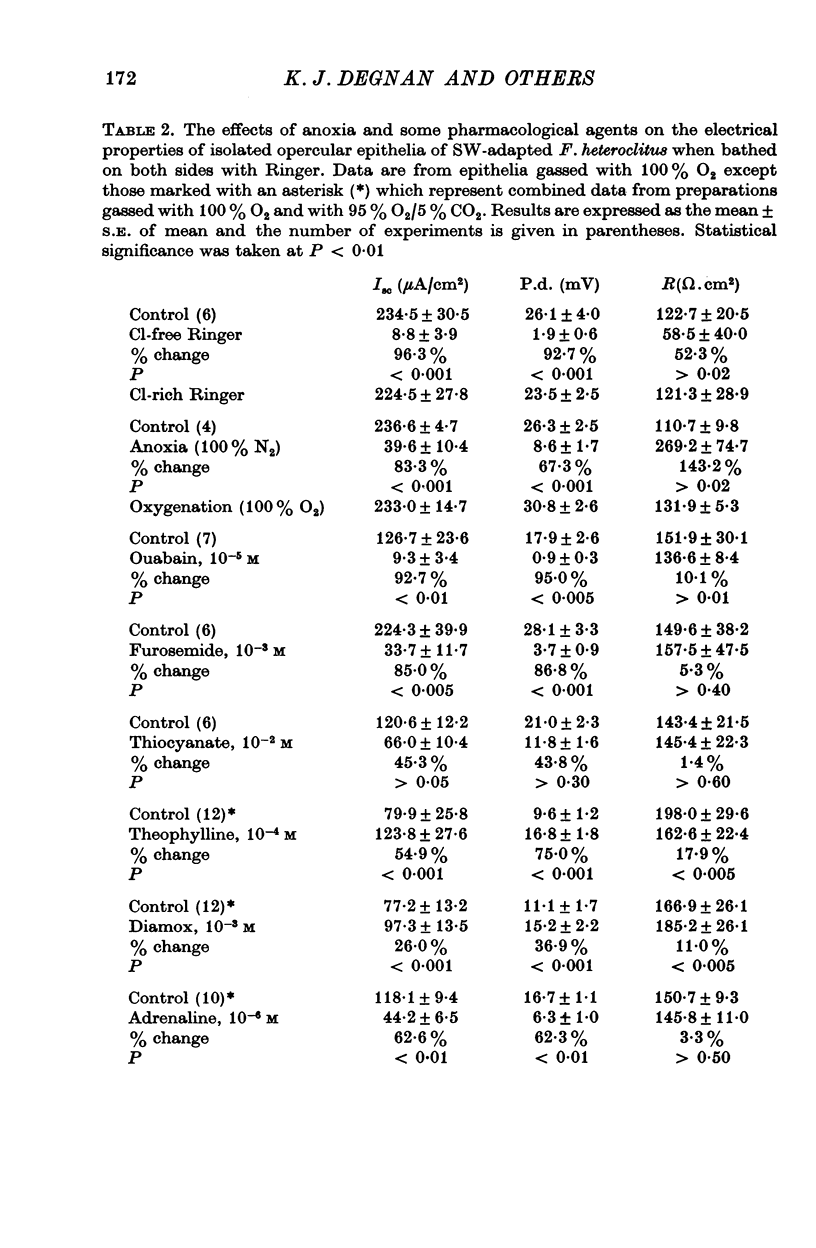
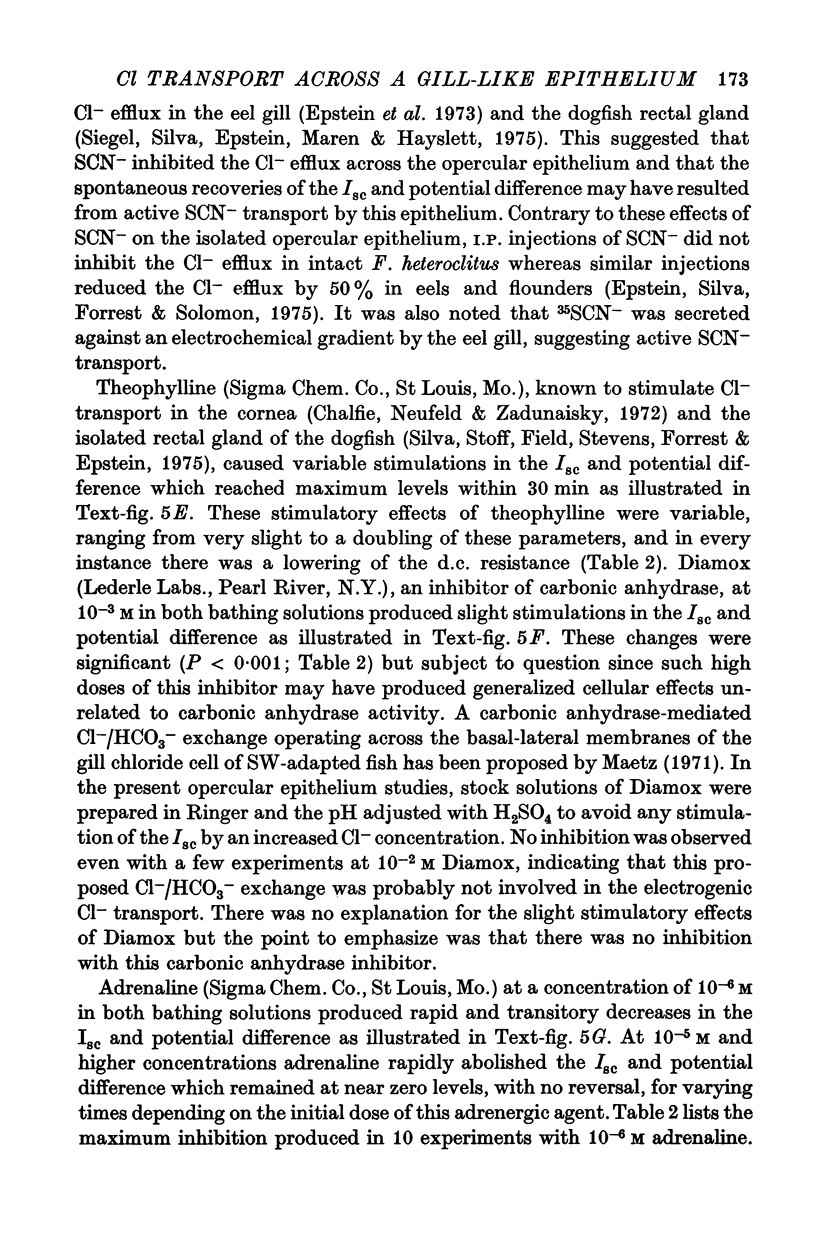
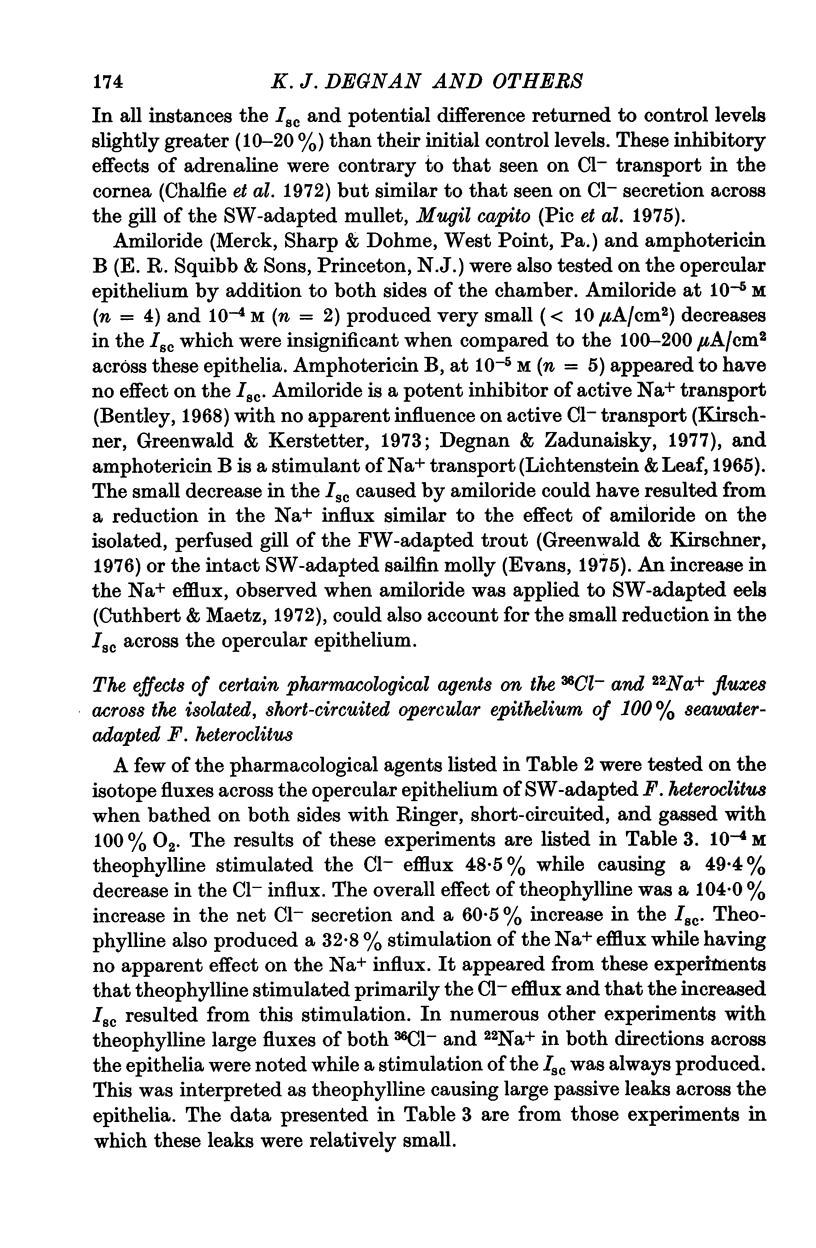
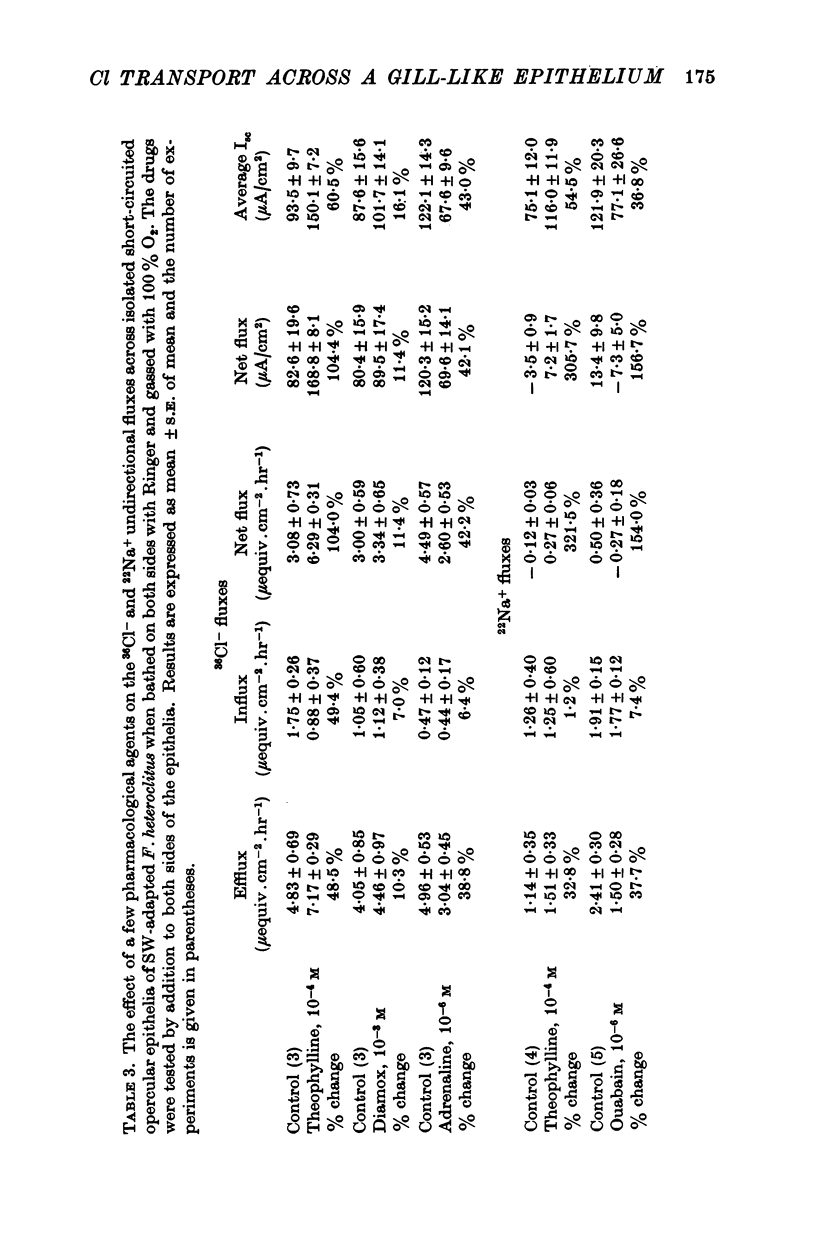
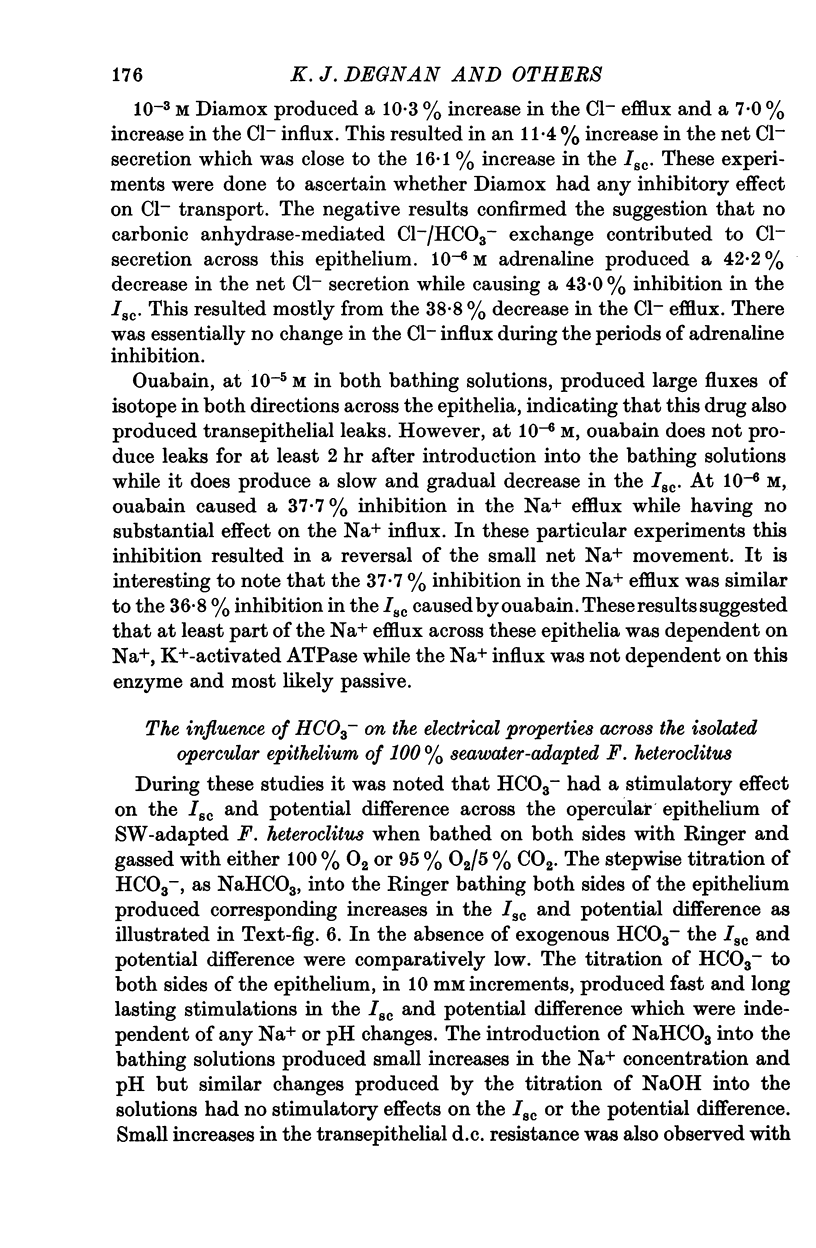
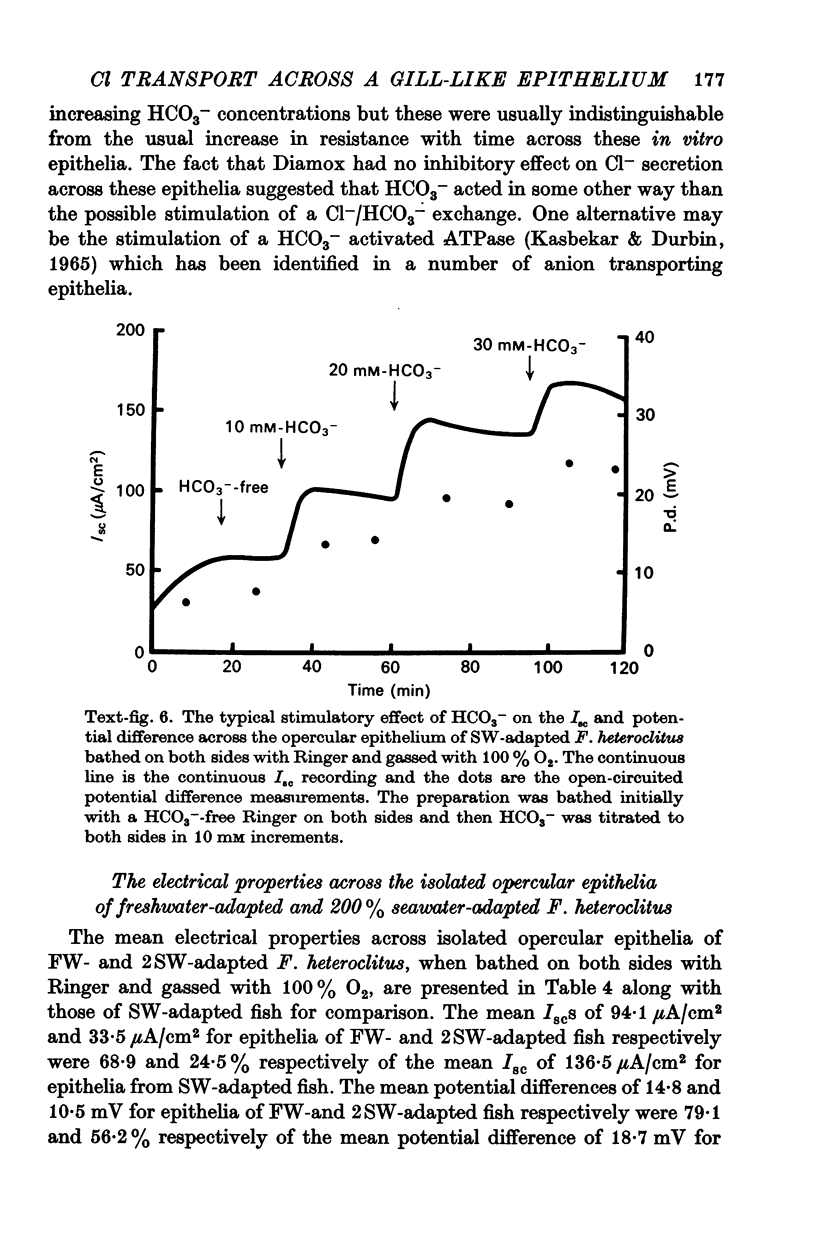
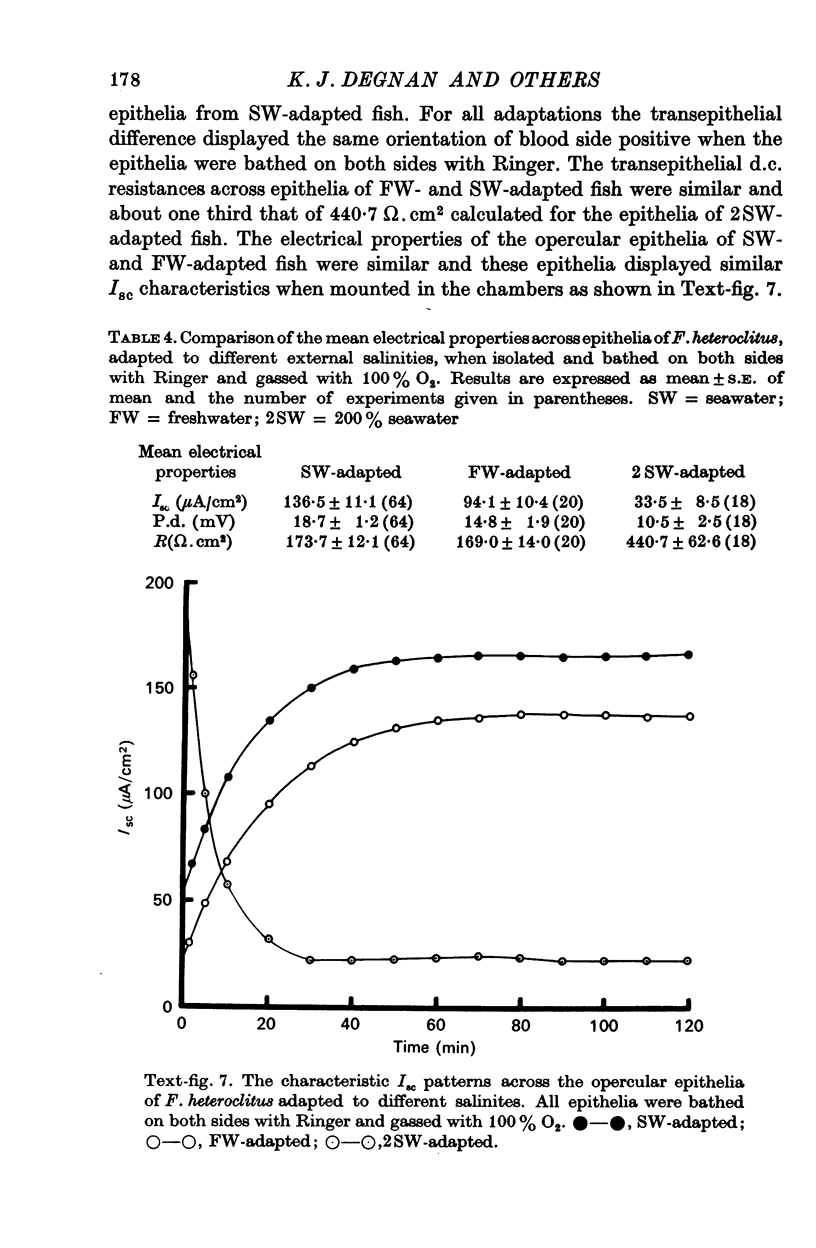
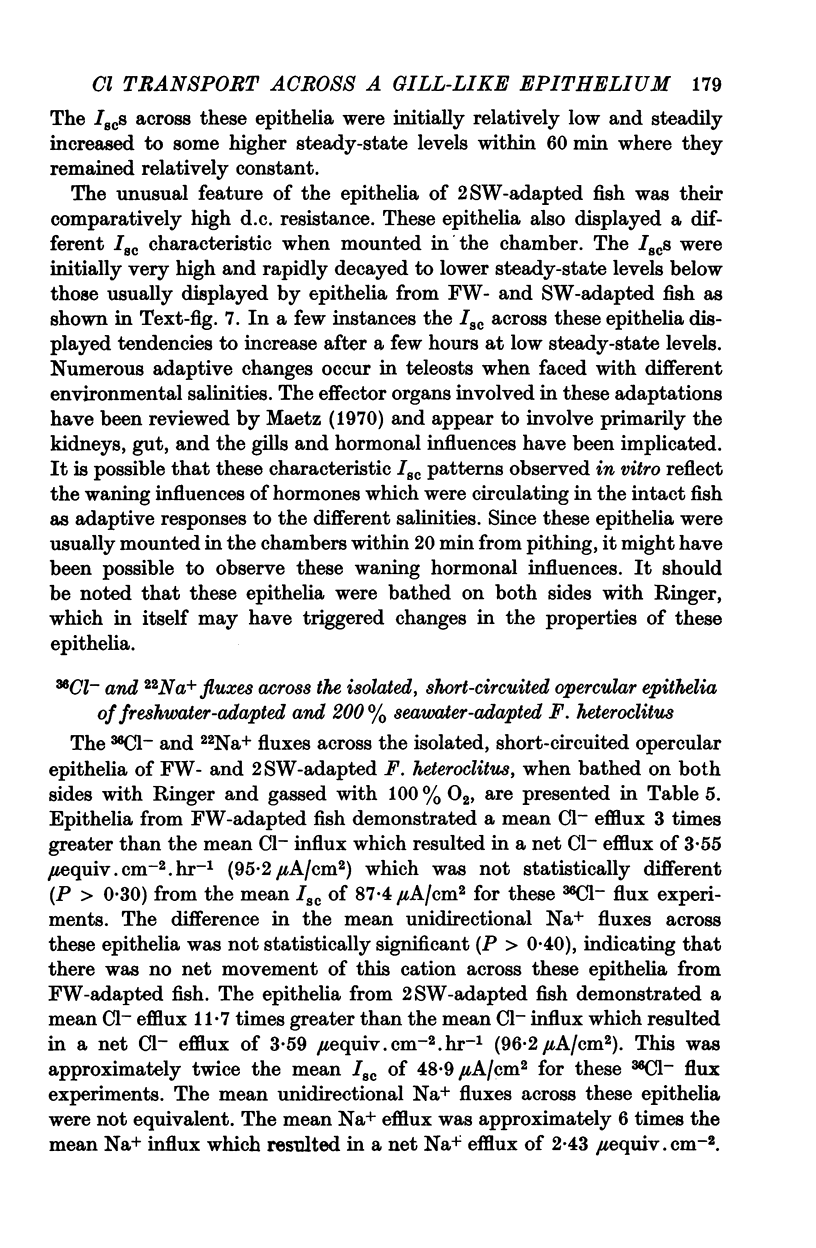
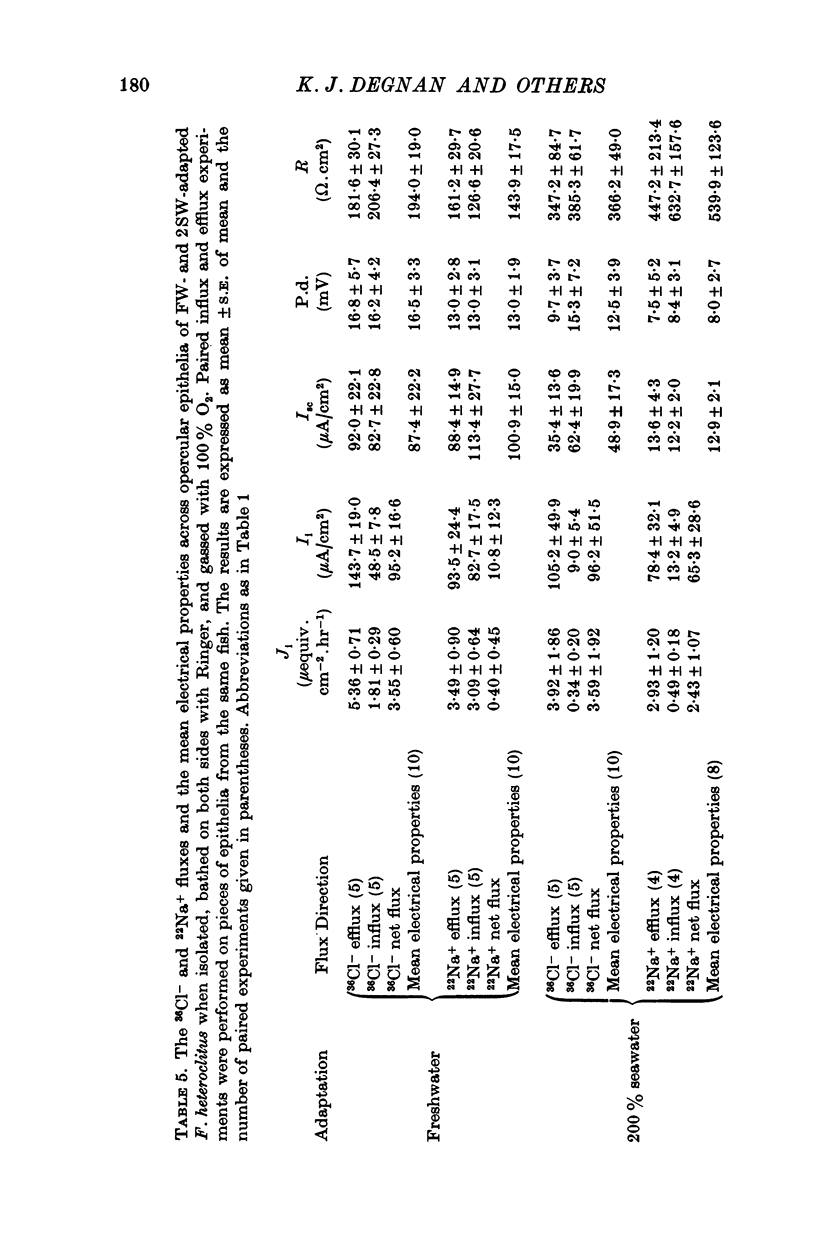
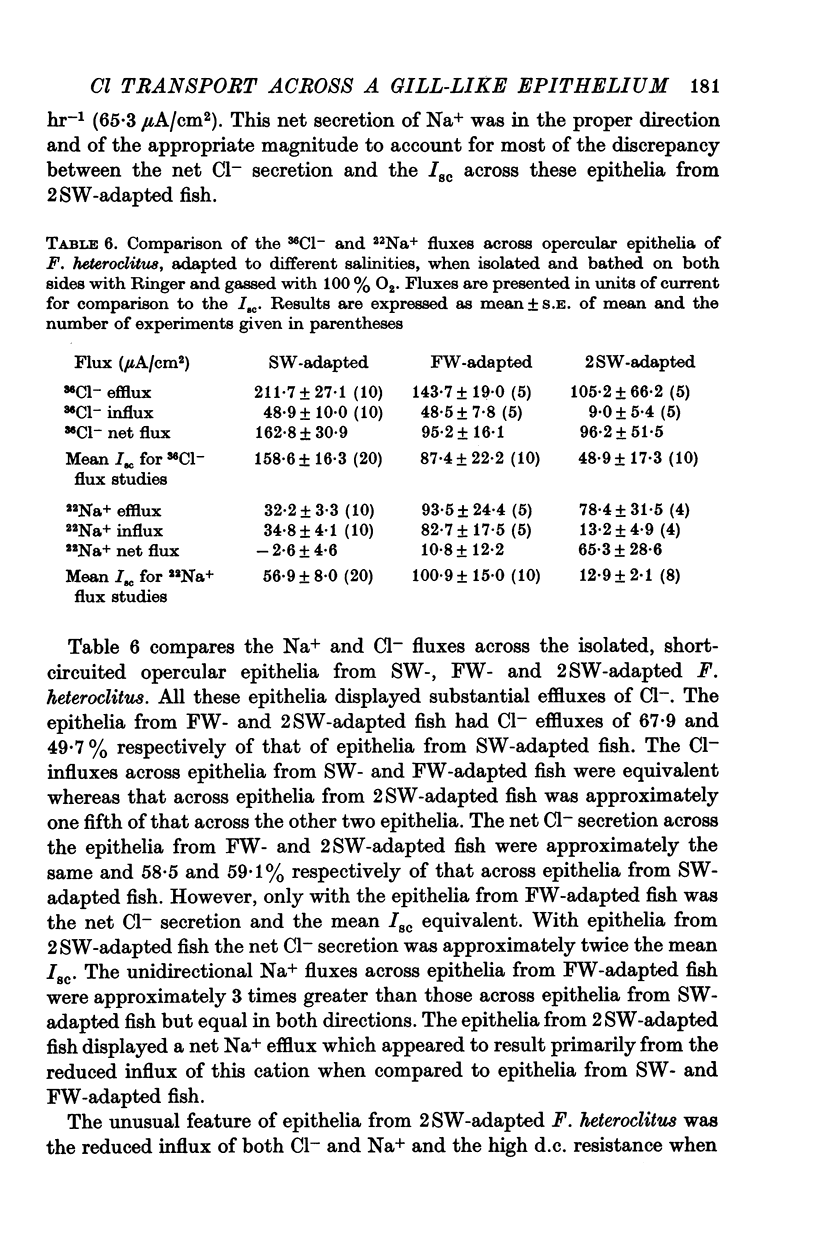
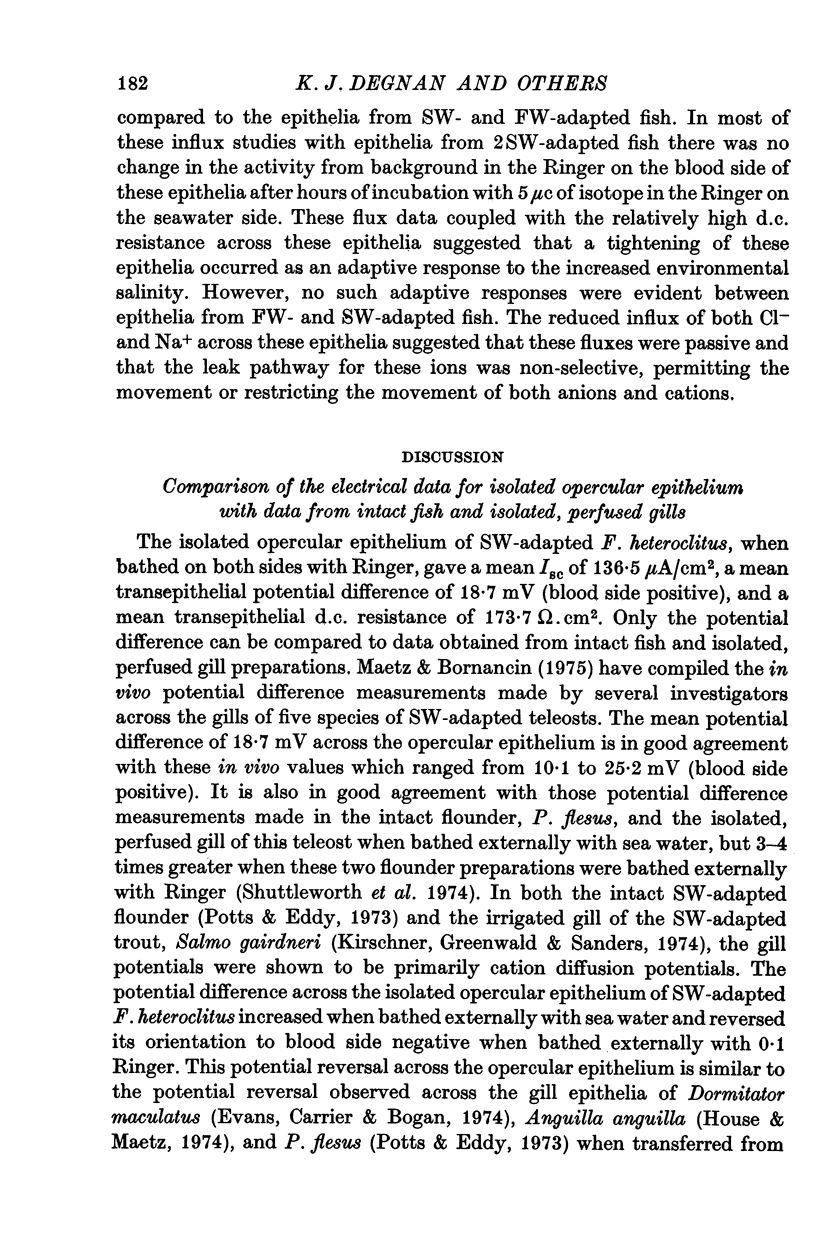
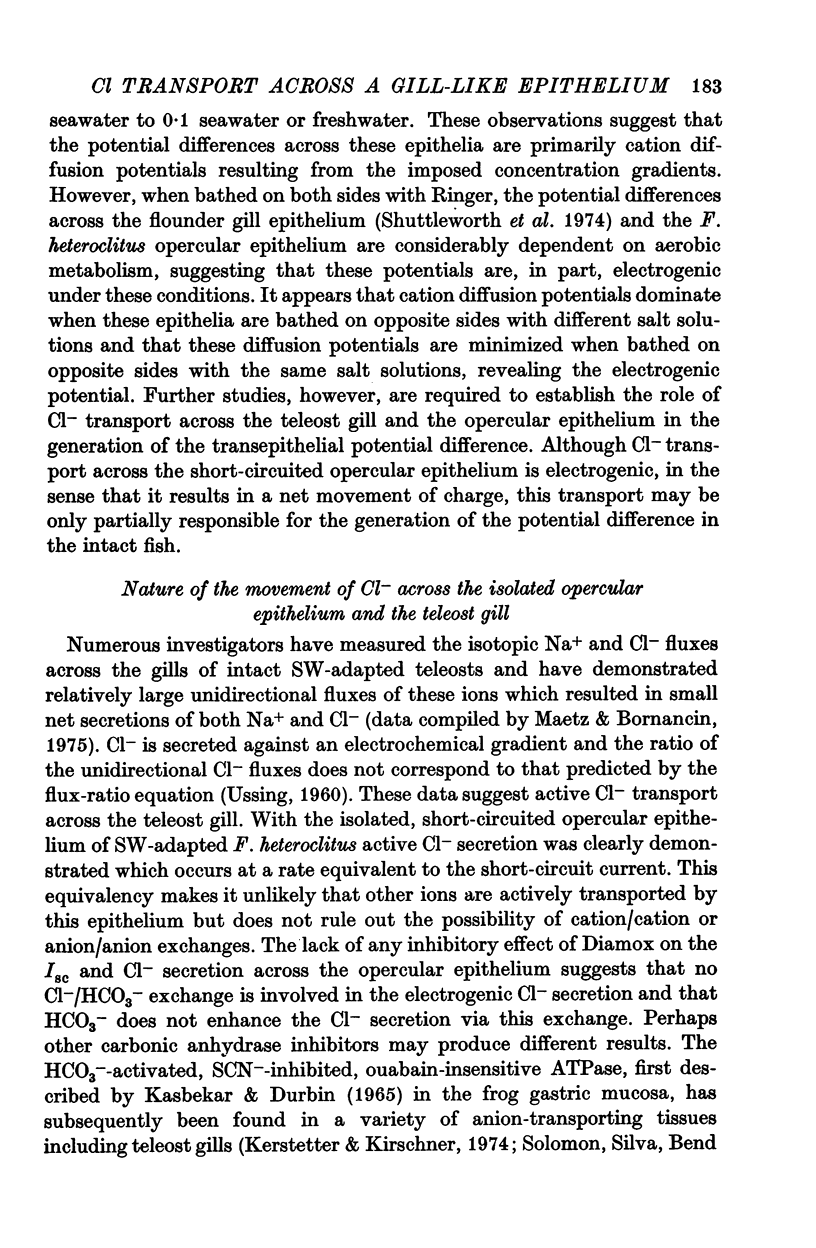
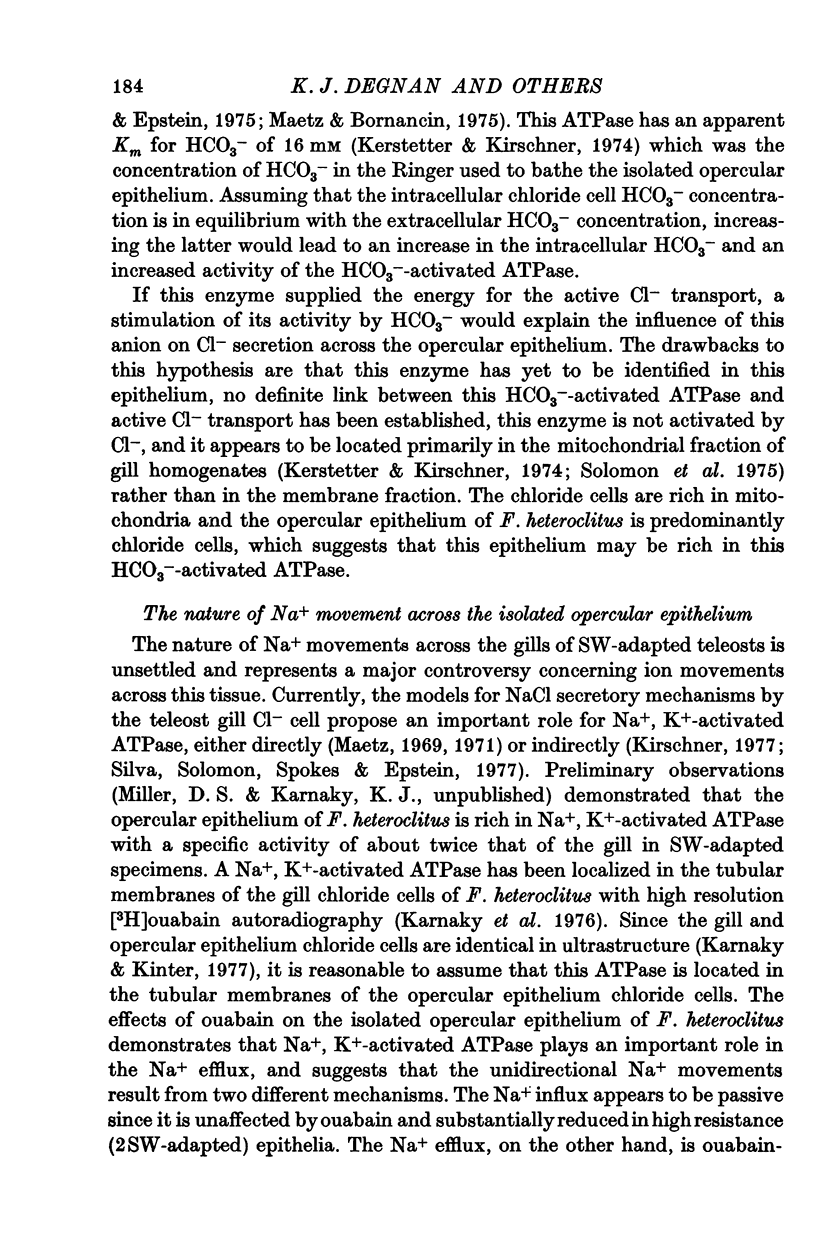
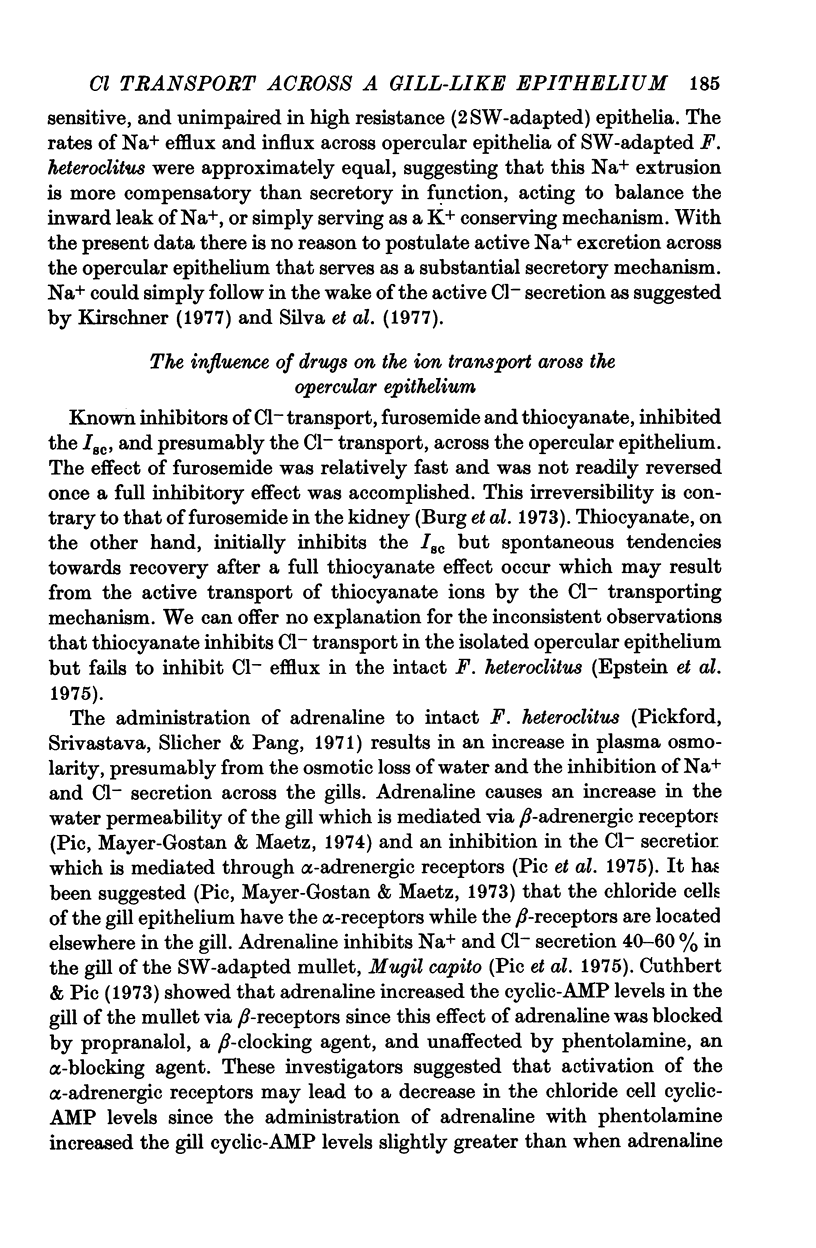
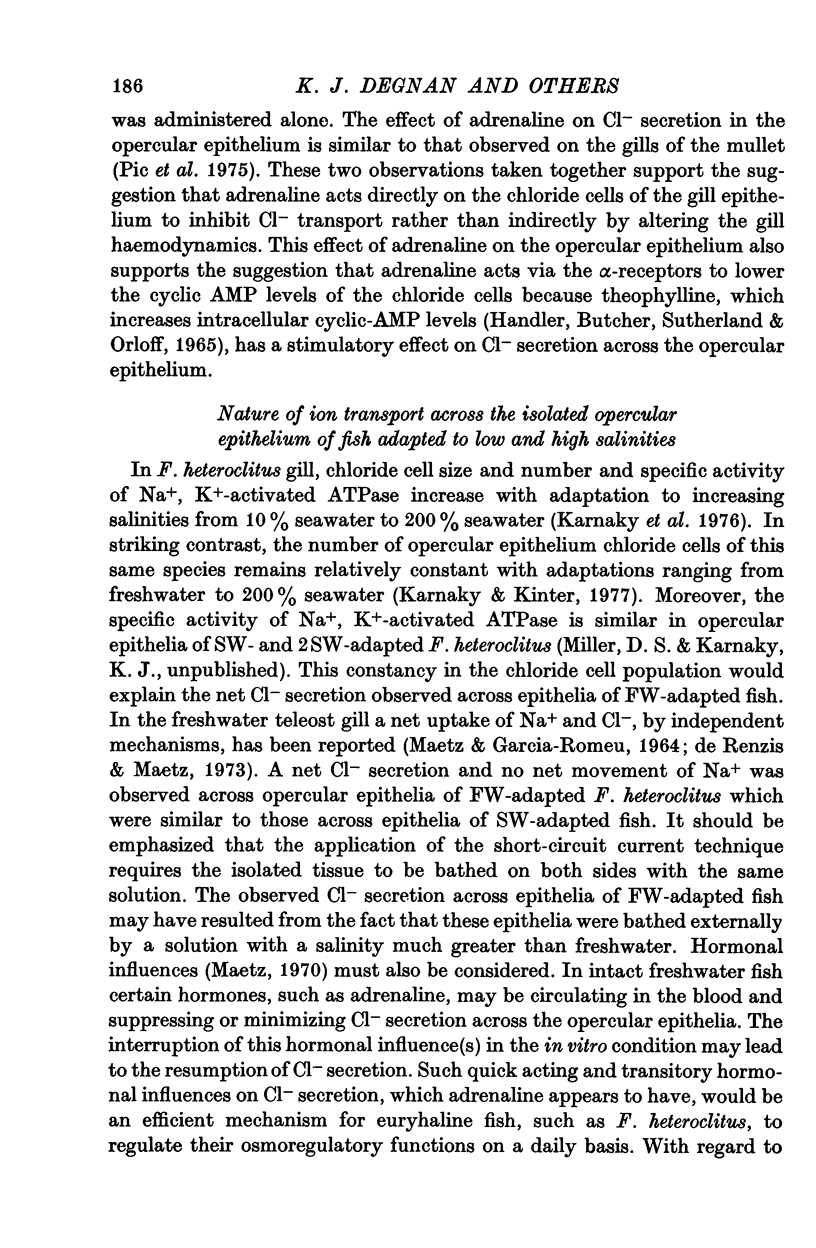
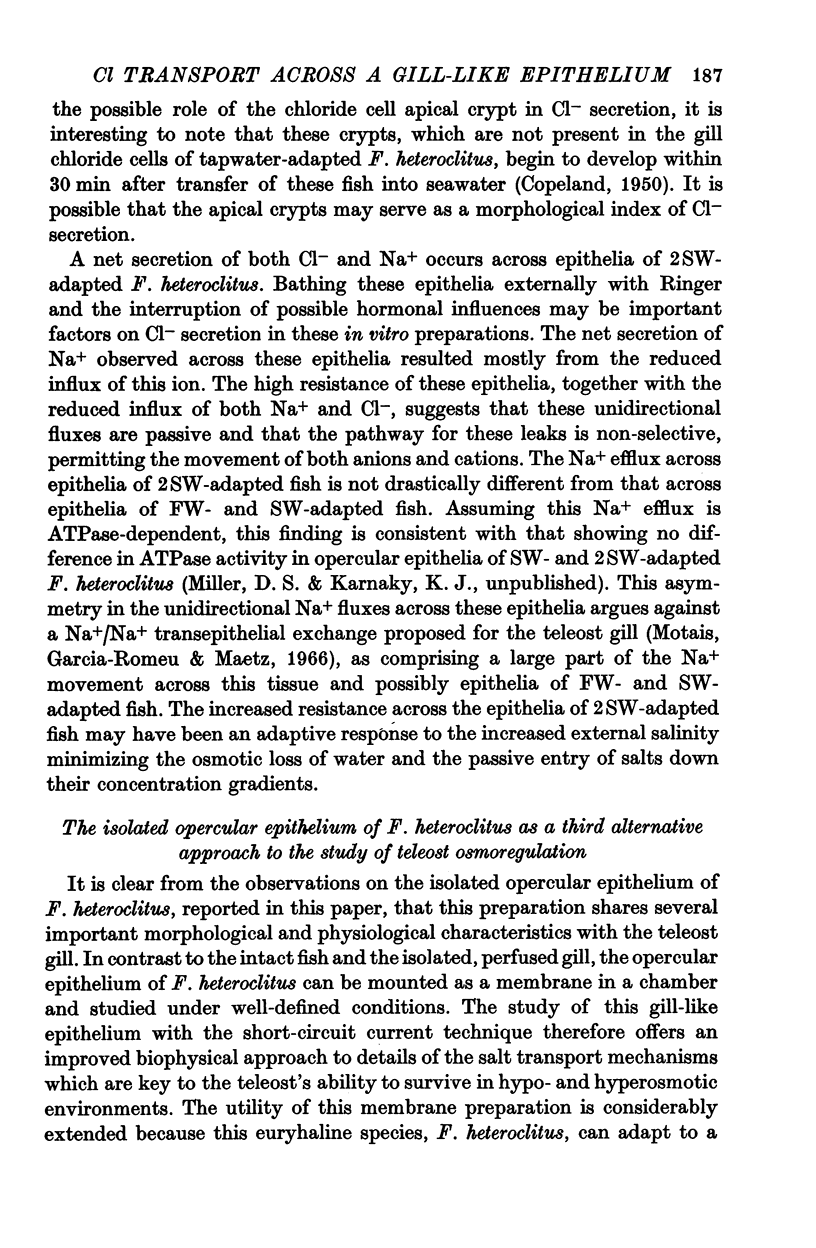
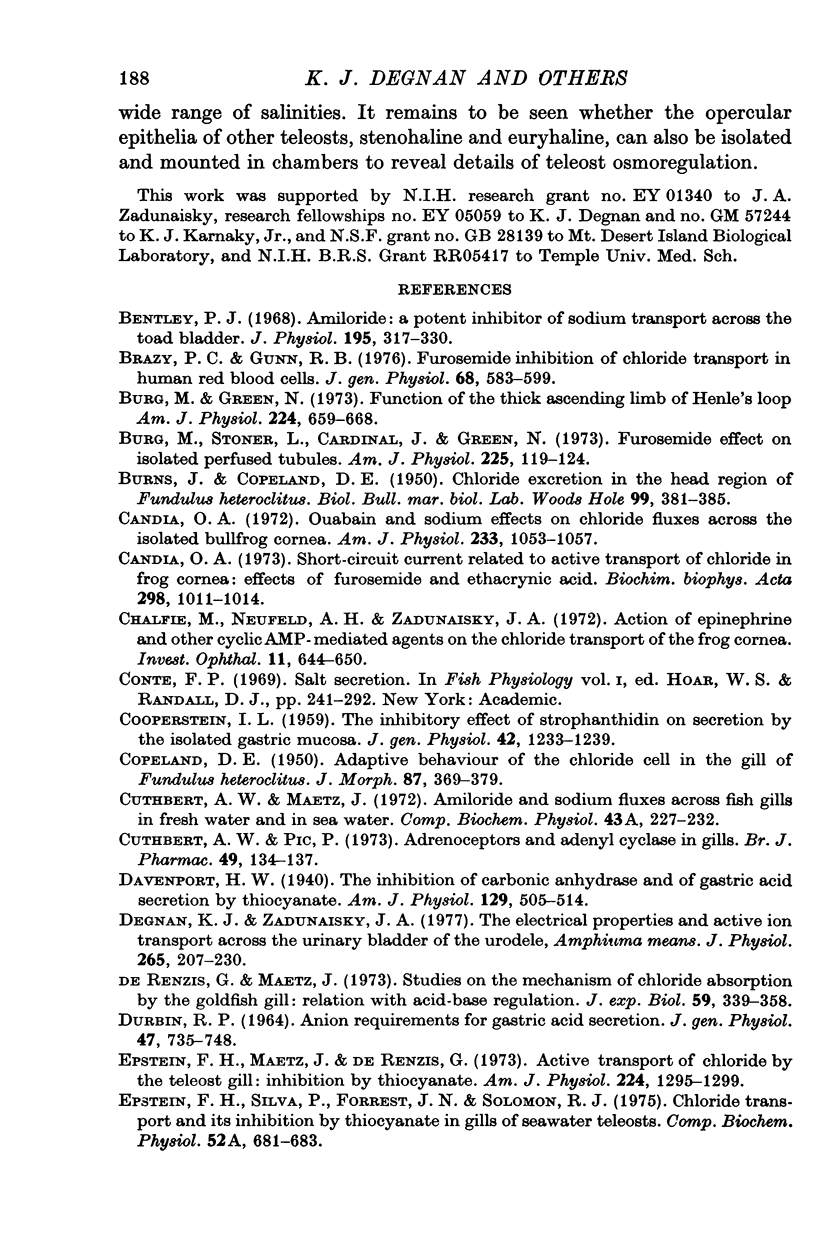
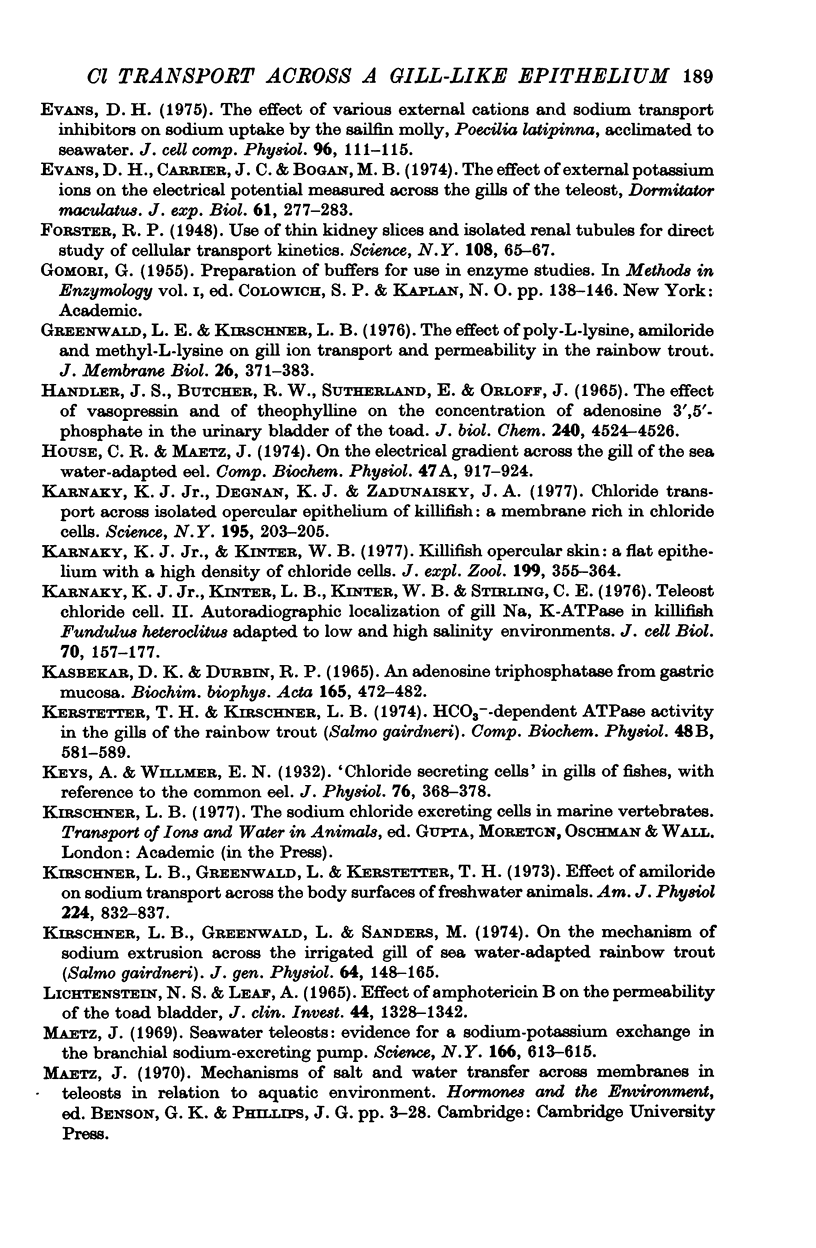
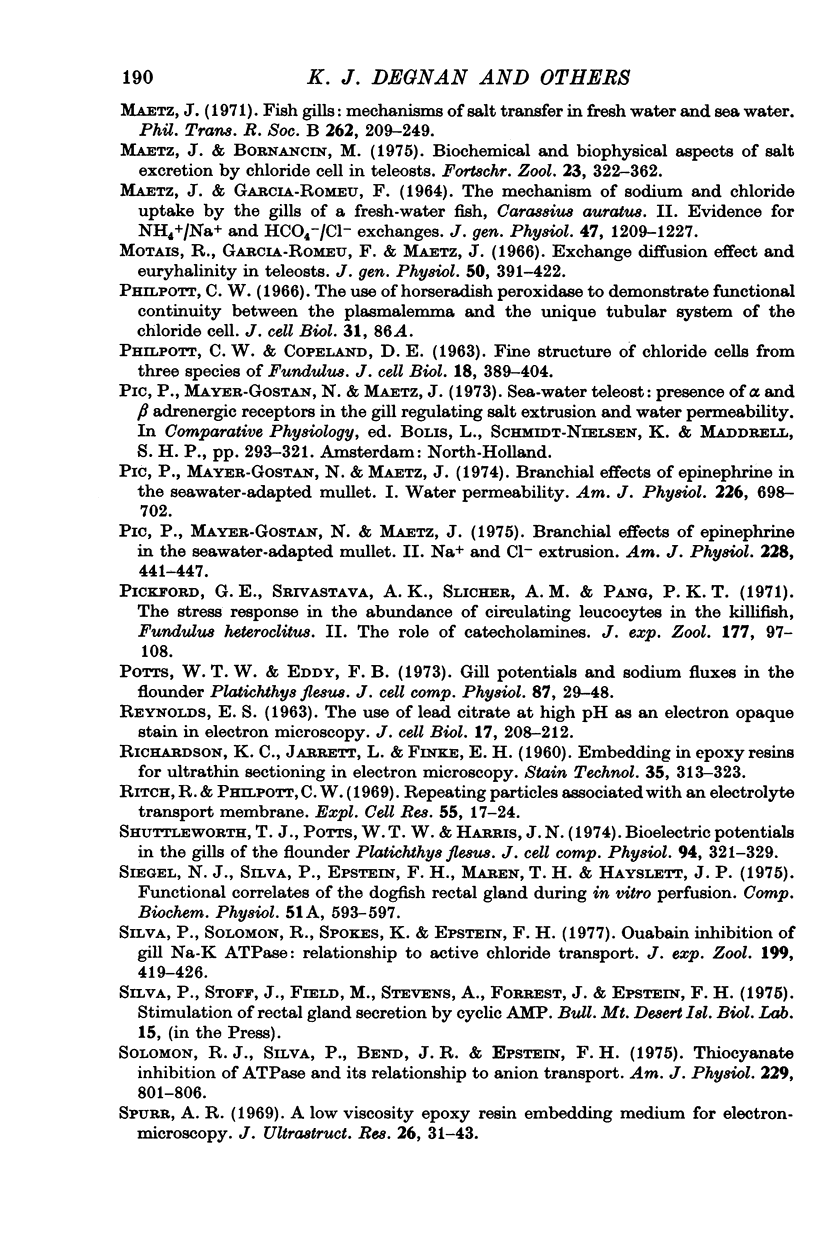
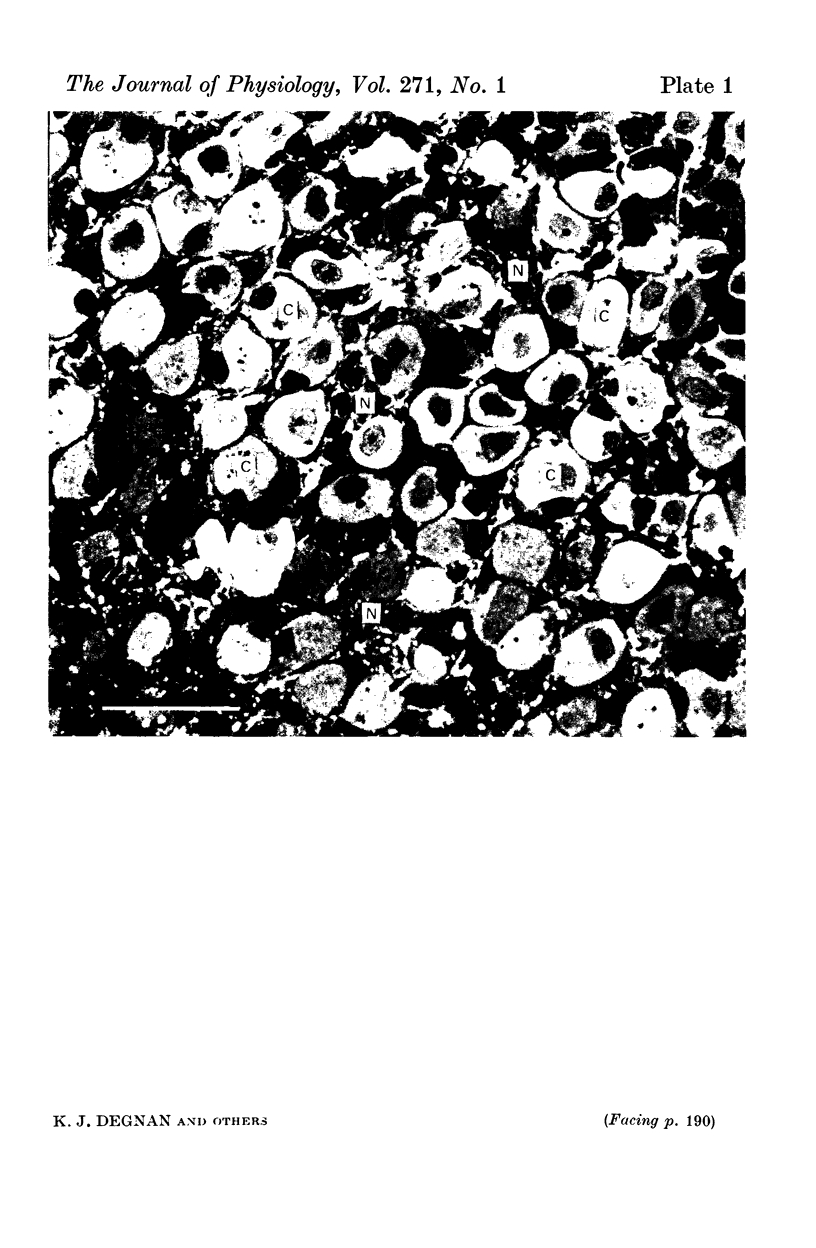
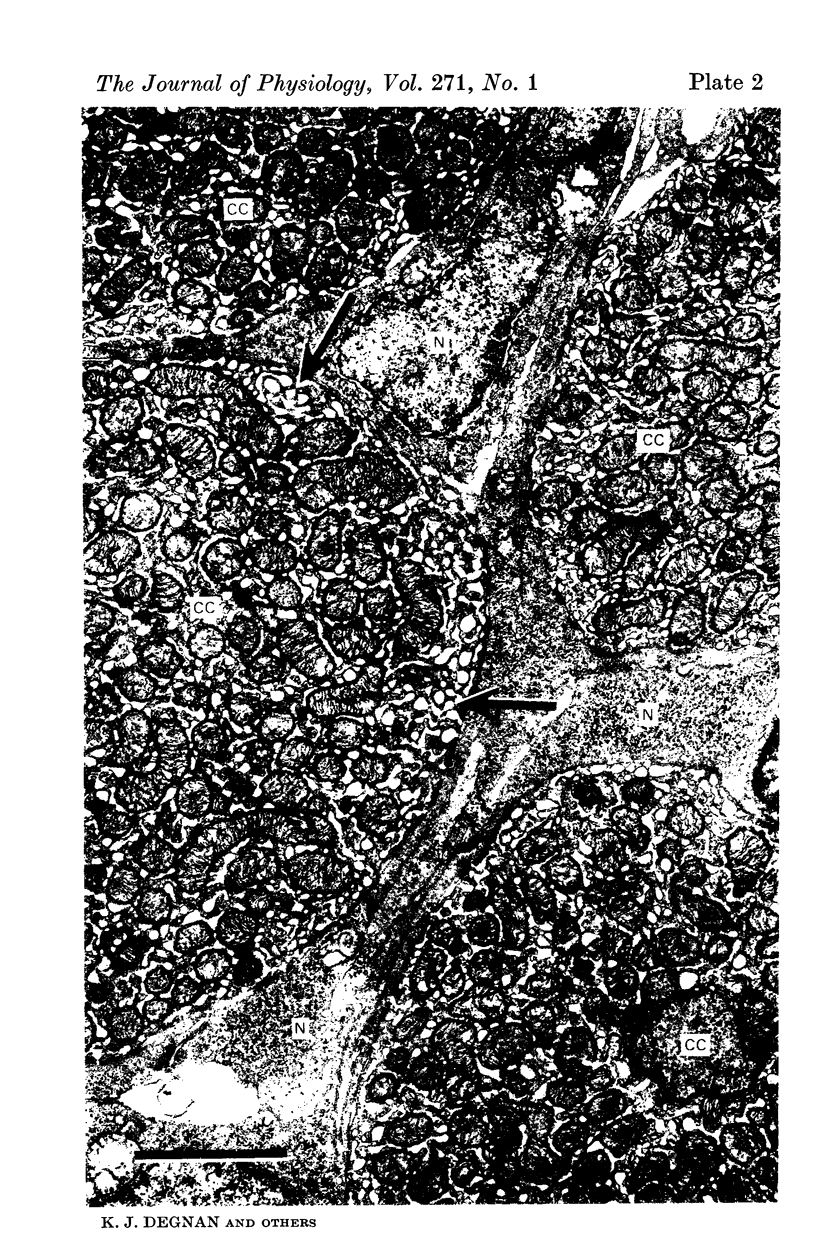
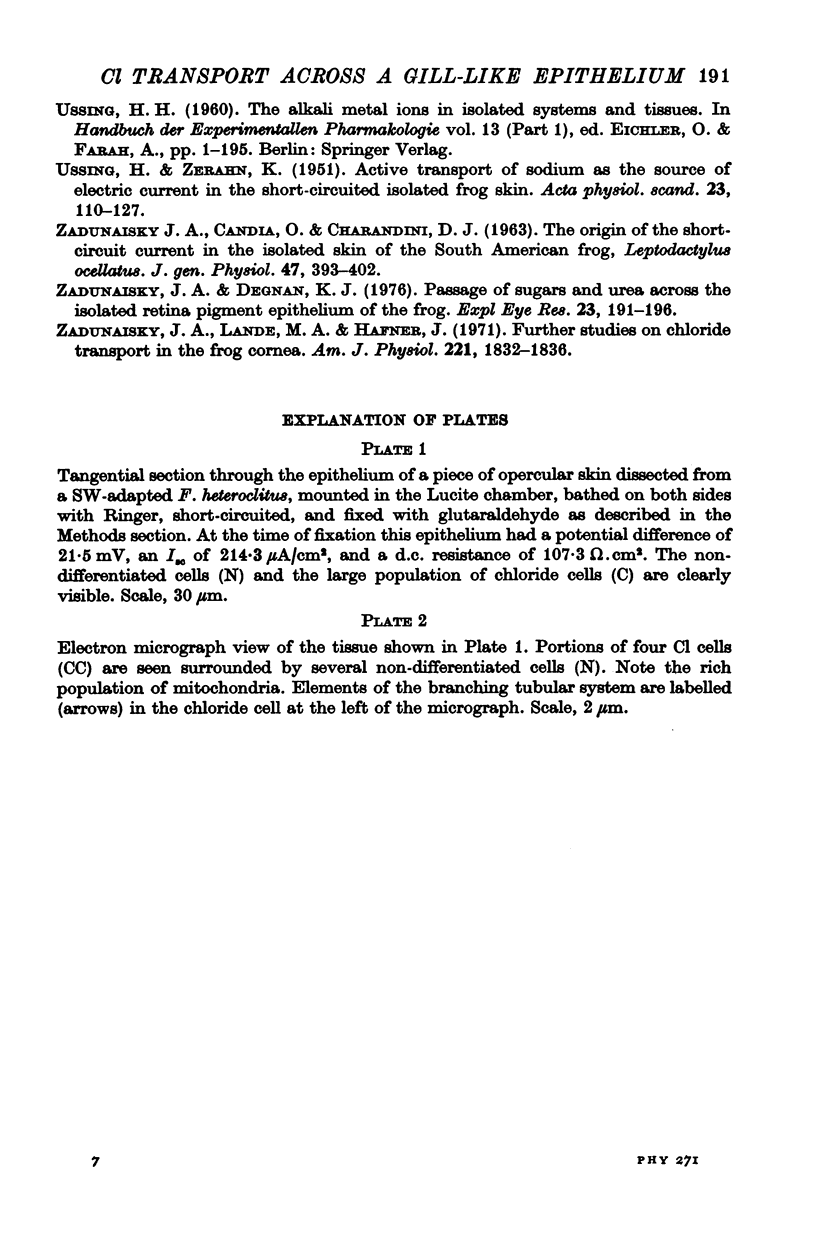
Images in this article
Selected References
These references are in PubMed. This may not be the complete list of references from this article.
- BURNS J., COPELAND D. E. Chloride excretion in the head region of fundulus heteroclitus. Biol Bull. 1950 Dec;99(3):381–385. doi: 10.2307/1538468. [DOI] [PubMed] [Google Scholar]
- Bentley P. J. Amiloride: a potent inhibitor of sodium transport across the toad bladder. J Physiol. 1968 Mar;195(2):317–330. doi: 10.1113/jphysiol.1968.sp008460. [DOI] [PMC free article] [PubMed] [Google Scholar]
- Brazy P. C., Gunn R. B. Furosemide inhibition of chloride transport in human red blood cells. J Gen Physiol. 1976 Dec;68(6):583–599. doi: 10.1085/jgp.68.6.583. [DOI] [PMC free article] [PubMed] [Google Scholar]
- Burg M. B., Green N. Function of the thick ascending limb of Henle's loop. Am J Physiol. 1973 Mar;224(3):659–668. doi: 10.1152/ajplegacy.1973.224.3.659. [DOI] [PubMed] [Google Scholar]
- Burg M., Stoner L., Cardinal J., Green N. Furosemide effect on isolated perfused tubules. Am J Physiol. 1973 Jul;225(1):119–124. doi: 10.1152/ajplegacy.1973.225.1.119. [DOI] [PubMed] [Google Scholar]
- Candia O. A. Ouabain and sodium effects on chloride fluxes across the isolated bullfrog cornea. Am J Physiol. 1972 Nov;223(5):1053–1057. doi: 10.1152/ajplegacy.1972.223.5.1053. [DOI] [PubMed] [Google Scholar]
- Candia O. A. Short-circuit current related to active transport of chloride in frog cornea: effects of furosemide and ethacrynic acid. Biochim Biophys Acta. 1973 Apr 16;298(4):1011–1014. doi: 10.1016/0005-2736(73)90407-0. [DOI] [PubMed] [Google Scholar]
- Chalfie M., Neufeld A. H., Zadunaisky J. A. Action of epinephrine and other cyclic AMP-mediated agents on the chloride transport of the frog cornea. Invest Ophthalmol. 1972 Aug;11(8):644–650. [PubMed] [Google Scholar]
- Cuthbert A. W., Maetz J. Amiloride and sodium fluxes across fish gills in fresh water and in sea water. Comp Biochem Physiol A Comp Physiol. 1972 Sep 1;43(1):227–232. doi: 10.1016/0300-9629(72)90487-2. [DOI] [PubMed] [Google Scholar]
- Cuthbert A. W., Pic P. Adrenoceptors and adenyl cyclase in gills. Br J Pharmacol. 1973 Sep;49(1):134–137. doi: 10.1111/j.1476-5381.1973.tb08277.x. [DOI] [PMC free article] [PubMed] [Google Scholar]
- DURBIN R. P. ANION REQUIREMENTS FOR GASTRIC ACID SECRETION. J Gen Physiol. 1964 Mar;47:735–748. doi: 10.1085/jgp.47.4.735. [DOI] [PMC free article] [PubMed] [Google Scholar]
- Degnan K. J., Zadunaisky J. A. The electrical properties and active ion transport across the urinary bladder of the urodele, Amphiuma means. J Physiol. 1977 Feb;265(1):207–230. doi: 10.1113/jphysiol.1977.sp011713. [DOI] [PMC free article] [PubMed] [Google Scholar]
- Epstein F. H., Maetz J., de Renzis G. Active transport of chloride by the teleost gill: inhibition by thiocyanate. Am J Physiol. 1973 Jun;224(6):1295–1299. doi: 10.1152/ajplegacy.1973.224.6.1295. [DOI] [PubMed] [Google Scholar]
- Epstein F. H., Silva P., Forrest J. N., Solomon R. J. Chloride transport and its inhibition by thiocyanate in gills of seawater teleosts. Comp Biochem Physiol A Comp Physiol. 1975 Dec 1;52(4):681–683. doi: 10.1016/s0300-9629(75)80023-5. [DOI] [PubMed] [Google Scholar]
- Evans D. H., Carrier J. C., Bogan M. B. The effect of external potassium ions on the electrical potential measured across the gills of the teleost, Dormitator maculatus. J Exp Biol. 1974 Oct;61(2):277–283. doi: 10.1242/jeb.61.2.277. [DOI] [PubMed] [Google Scholar]
- Forster R. P. Use of Thin Kidney Slices and Isolated Renal Tubules for Direct Study of Cellular Transport Kinetics. Science. 1948 Jul 16;108(2794):65–67. doi: 10.1126/science.108.2794.65-a. [DOI] [PubMed] [Google Scholar]
- Greenwald L. E., Kirschner L. B. The effect of poly-L-lysine, amiloride and methyl-L-lysine on gill ion transport and permeability in the rainbow trout. J Membr Biol. 1976 May;26(4):371–383. doi: 10.1007/BF01868884. [DOI] [PubMed] [Google Scholar]
- Handler J. S., Butcher R. W., Sutherland E. W., Orloff J. The effect of vasopressin and of theophylline on the concentration of adenosine 3',5'-phosphate in the urinary bladder of the toad. J Biol Chem. 1965 Nov;240(11):4524–4526. [PubMed] [Google Scholar]
- House C. R., Maetz J. On the electrical gradient across the gill of the sea water-adapted eel. Comp Biochem Physiol A Comp Physiol. 1974 Mar 1;47(3):917–924. doi: 10.1016/0300-9629(74)90466-6. [DOI] [PubMed] [Google Scholar]
- Karnaky K. G., Jr, Kinter W. B. Killifish opercular skin: a flat epithelium with a high density of chloride cells. J Exp Zool. 1977 Mar;199(3):355–364. doi: 10.1002/jez.1401990309. [DOI] [PubMed] [Google Scholar]
- Karnaky K. J., Jr, Degnan K. J., Zadunaisky J. A. Chloride transport across isolated opercular epithelium of killifish: a membrane rich in chloride cells. Science. 1977 Jan 14;195(4274):203–205. doi: 10.1126/science.831273. [DOI] [PubMed] [Google Scholar]
- Karnaky K. J., Jr, Kinter L. B., Kinter W. B., Stirling C. E. Teleost chloride cell. II. Autoradiographic localization of gill Na,K-ATPase in killifish Fundulus heteroclitus adapted to low and high salinity environments. J Cell Biol. 1976 Jul;70(1):157–177. doi: 10.1083/jcb.70.1.157. [DOI] [PMC free article] [PubMed] [Google Scholar]
- Kasbekar D. K., Durbin R. P. An adenosine triphosphatase from frog gastric mucosa. Biochim Biophys Acta. 1965 Sep 20;105(3):472–482. doi: 10.1016/s0926-6593(65)80232-6. [DOI] [PubMed] [Google Scholar]
- Kerstetter T. H., Kirschner L. B. HCO3- dependent ATPase activity in the gills of rainbow trout (Salmo gairdneri). Comp Biochem Physiol B. 1974 Aug 15;48(4):581–589. doi: 10.1016/0305-0491(74)90138-2. [DOI] [PubMed] [Google Scholar]
- Keys A., Willmer E. N. "Chloride secreting cells" in the gills of fishes, with special reference to the common eel. J Physiol. 1932 Nov 5;76(3):368–378.2. doi: 10.1113/jphysiol.1932.sp002932. [DOI] [PMC free article] [PubMed] [Google Scholar]
- Kirschner L. B., Greenwald L., Kerstetter T. H. Effect of amiloride on sodium transport across body surfaces of freshwater animals. Am J Physiol. 1973 Apr;224(4):832–837. doi: 10.1152/ajplegacy.1973.224.4.832. [DOI] [PubMed] [Google Scholar]
- Kirschner L. B., Greenwald L., Sanders M. On the mechanism of sodium extrusion across the irrigated gill of sea water-adapted rainbow trout (Salmo gairdneri). J Gen Physiol. 1974 Aug;64(2):148–165. [PMC free article] [PubMed] [Google Scholar]
- LICHTENSTEIN N. S., LEAF A. EFFECT OF AMPHOTERICIN B ON THE PERMEABILITY OF THE TOAD BLADDER. J Clin Invest. 1965 Aug;44:1328–1342. doi: 10.1172/JCI105238. [DOI] [PMC free article] [PubMed] [Google Scholar]
- MAETZ J., GARCIAROMEU F. THE MECHANISM OF SODIUM AND CHLORIDE UPTAKE BY THE GILLS OF A FRESH-WATER FISH, CARASSIUS AURATUS. II. EVIDENCE FOR NH4 ION/NA ION AND HCO3 ION/C1 ION EXCHANGES. J Gen Physiol. 1964 Jul;47:1209–1227. doi: 10.1085/jgp.47.6.1209. [DOI] [PMC free article] [PubMed] [Google Scholar]
- Maetz J., Bornancin M. Biochemical and biophysical aspects of salt excretion by chloride cells in teleosts. Fortschr Zool. 1975;23(2-3):322–362. [PubMed] [Google Scholar]
- Maetz J. Seawater teleosts: evidence for a sodium-potassium exchange in the branchial sodium-excreting pump. Science. 1969 Oct 31;166(3905):613–615. doi: 10.1126/science.166.3905.613. [DOI] [PubMed] [Google Scholar]
- Motais R., Romeu F. G., Maetz J. Exchange diffusion effect and euryhalinity in teleosts. J Gen Physiol. 1966 Nov;50(2):391–422. doi: 10.1085/jgp.50.2.391. [DOI] [PMC free article] [PubMed] [Google Scholar]
- PHILPOTT C. W., COPELAND D. E. FINE STRUCTURE OF CHLORIDE CELLS FROM THREE SPECIES OF FUNDULUS. J Cell Biol. 1963 Aug;18:389–404. doi: 10.1083/jcb.18.2.389. [DOI] [PMC free article] [PubMed] [Google Scholar]
- Pic P., Mayer-Gostan N., Maetz J. Branchial effects of epinephrine in the seawater-adapted mullet. I. Water permeability. Am J Physiol. 1974 Mar;226(3):698–702. doi: 10.1152/ajplegacy.1974.226.3.698. [DOI] [PubMed] [Google Scholar]
- Pic P., Mayer-Gostan N., Maetz J. Branchial effects of epinephrine in the seawater-adapted mullet. II. Na+ and Cl- extrusion. Am J Physiol. 1975 Feb;228(2):441–447. doi: 10.1152/ajplegacy.1975.228.2.441. [DOI] [PubMed] [Google Scholar]
- Pickford G. E., Srivastava A. K., Slicher A. M., Pang P. K. The stress response in the abundance of circulating leucocytes in the killifish, Fundulus heteroclitus. II. The role of catecholamines. J Exp Zool. 1971 May;177(1):97–108. doi: 10.1002/jez.1401770111. [DOI] [PubMed] [Google Scholar]
- REYNOLDS E. S. The use of lead citrate at high pH as an electron-opaque stain in electron microscopy. J Cell Biol. 1963 Apr;17:208–212. doi: 10.1083/jcb.17.1.208. [DOI] [PMC free article] [PubMed] [Google Scholar]
- RICHARDSON K. C., JARETT L., FINKE E. H. Embedding in epoxy resins for ultrathin sectioning in electron microscopy. Stain Technol. 1960 Nov;35:313–323. doi: 10.3109/10520296009114754. [DOI] [PubMed] [Google Scholar]
- Ritch R., Philpott C. W. Repeating particles associated with an electrolyte-transport membrane. Exp Cell Res. 1969 Apr;55(1):17–24. doi: 10.1016/0014-4827(69)90448-0. [DOI] [PubMed] [Google Scholar]
- Siegel N. J., Silva P., Epstein F. H., Maren T. H., Hayslett J. P. Functional correlates of the dogfish rectal gland during in vitro perfusion. Comp Biochem Physiol A Comp Physiol. 1975 Jul 1;51(3):593–597. doi: 10.1016/0300-9629(75)90346-1. [DOI] [PubMed] [Google Scholar]
- Silva P., Solomon R., Spokes K., Epstein F. Ouabain inhibition of gill Na-K-ATPase: relationship to active chloride transport. J Exp Zool. 1977 Mar;199(3):419–426. doi: 10.1002/jez.1401990316. [DOI] [PubMed] [Google Scholar]
- Solomon R. J., Silva P., Bend J. R., Epstein F. H. Thiocyanate inhibition of ATPase and its relationship to anion transport. Am J Physiol. 1975 Sep;229(3):801–806. doi: 10.1152/ajplegacy.1975.229.3.801. [DOI] [PubMed] [Google Scholar]
- Spurr A. R. A low-viscosity epoxy resin embedding medium for electron microscopy. J Ultrastruct Res. 1969 Jan;26(1):31–43. doi: 10.1016/s0022-5320(69)90033-1. [DOI] [PubMed] [Google Scholar]
- USSING H. H., ZERAHN K. Active transport of sodium as the source of electric current in the short-circuited isolated frog skin. Acta Physiol Scand. 1951 Aug 25;23(2-3):110–127. doi: 10.1111/j.1748-1716.1951.tb00800.x. [DOI] [PubMed] [Google Scholar]
- ZADUNAISKY J. A., CANDIA O. A., CHIARANDINI D. J. THE ORIGIN OF THE SHORT-CIRCUIT CURRENT IN THE ISOLATED SKIN OF THE SOUTH AMERICAN FROG LEPTODACTYLUS OCELLATUS. J Gen Physiol. 1963 Nov;47:393–402. doi: 10.1085/jgp.47.2.393. [DOI] [PMC free article] [PubMed] [Google Scholar]
- Zadunaisky J. A., Degnan K. J. Passage of sugars and urea across the isolated retina pigment epithelium of the frog. Exp Eye Res. 1976 Aug;23(2):191–196. doi: 10.1016/0014-4835(76)90202-5. [DOI] [PubMed] [Google Scholar]