Abstract
1. A resting sartorius muscle of the frog or toad possesses a special kind of elasticity which is shown to be due to a component lying between the two sets of filaments. The elastic effect is seen only for very small length changes, up to about 0·2% of the muscle length, and the `elastic limit' is then reached. If the length change then continues at a constant velocity the tension developed is maintained at a fixed level, producing a sort of frictional resistance. The component responsible is called the `short-range elastic component', or SREC.
2. It is also shown that a small part of the permanent tension of a resting muscle is probably due to `active' interaction between the filaments. This is called the `filamentary resting tension', or FRT. For a sarcomere length of 2·0 μ the FRT amounts to about 150 mg in a muscle weighing 100 mg.
3. The stiffness of the SREC and the magnitude of the FRT are shown to be related to one another. They are both increased, and may rise to high values, by making the external solution hypertonic.
4. The working hypothesis is as follows. In a resting muscle the cross-bridges on the myosin filaments are not entirely inactive, but a very small proportion of them are cross-linked with the actin filaments. The links are very stable and have a long `life'. The elastic behaviour is due to the flexural rigidity, or spring-like properties of these bridges. The elasticity is `short-range' because the bridges can be bent, or stretched, only a small way from the steady-state position before the contacts `slip'. The `filamentary resting tension' (which is present in the absence of any external length change) is attributed to an `active process', which operates by imparting `organized' potential energy to the participating cross-bridges, by potentiating their attachment, against their elastic resistance, to sites on the actin filaments which are displaced towards the Z line.
5. It is shown that the ability of the muscle to maintain a `frictional' resistance to a continuing, slow, length change is suppressed during a maintained tetanic contraction. The process of suppression starts during the period of the latency relaxation.
6. It is suggested that the latency relaxation may be due to a reduction of the filamentary resting tension.
7. The experiments with the latency relaxation were facilitated by the use of strongly hypertonic solutions. The positive twitch tension is then greatly reduced, or may be practically abolished, while the latency relaxation remains at about its normal size and is extended in duration.
Full text
PDF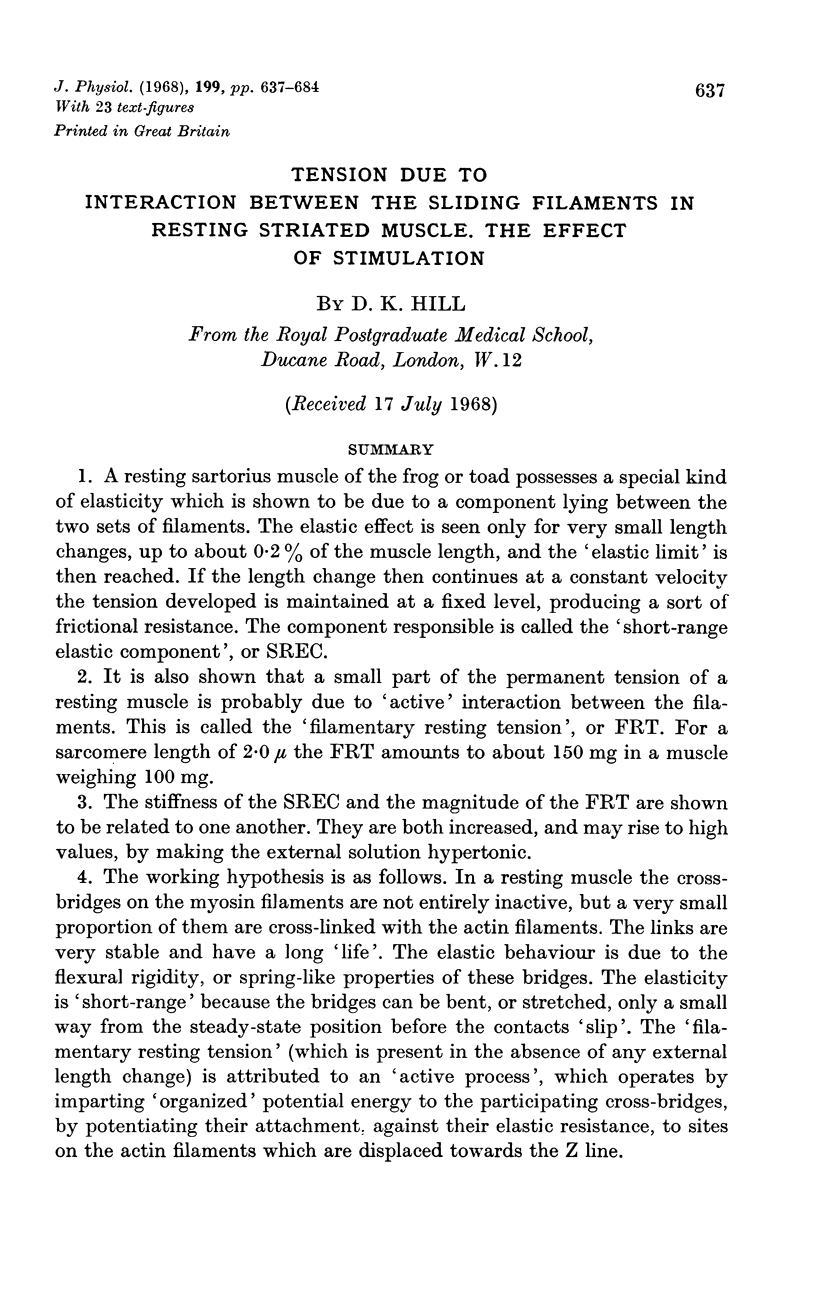
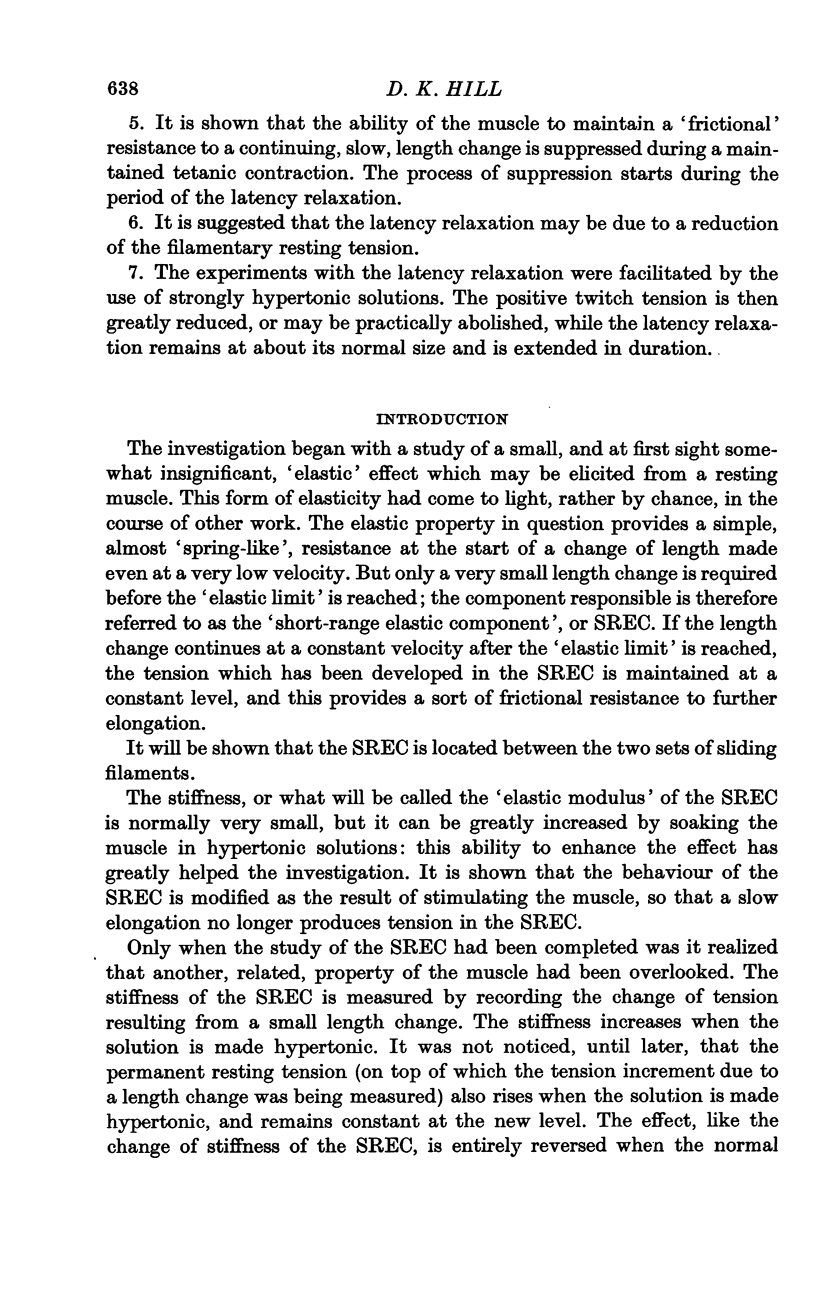
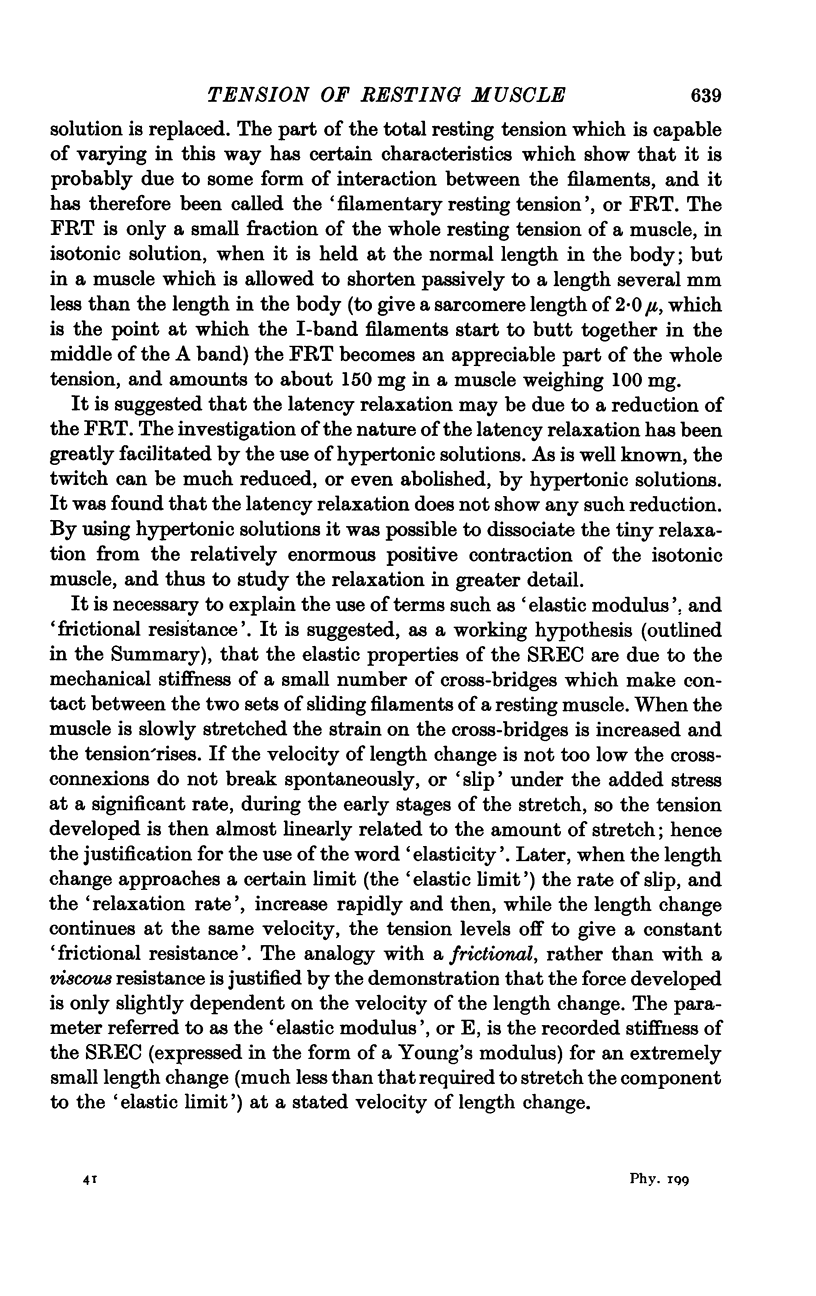
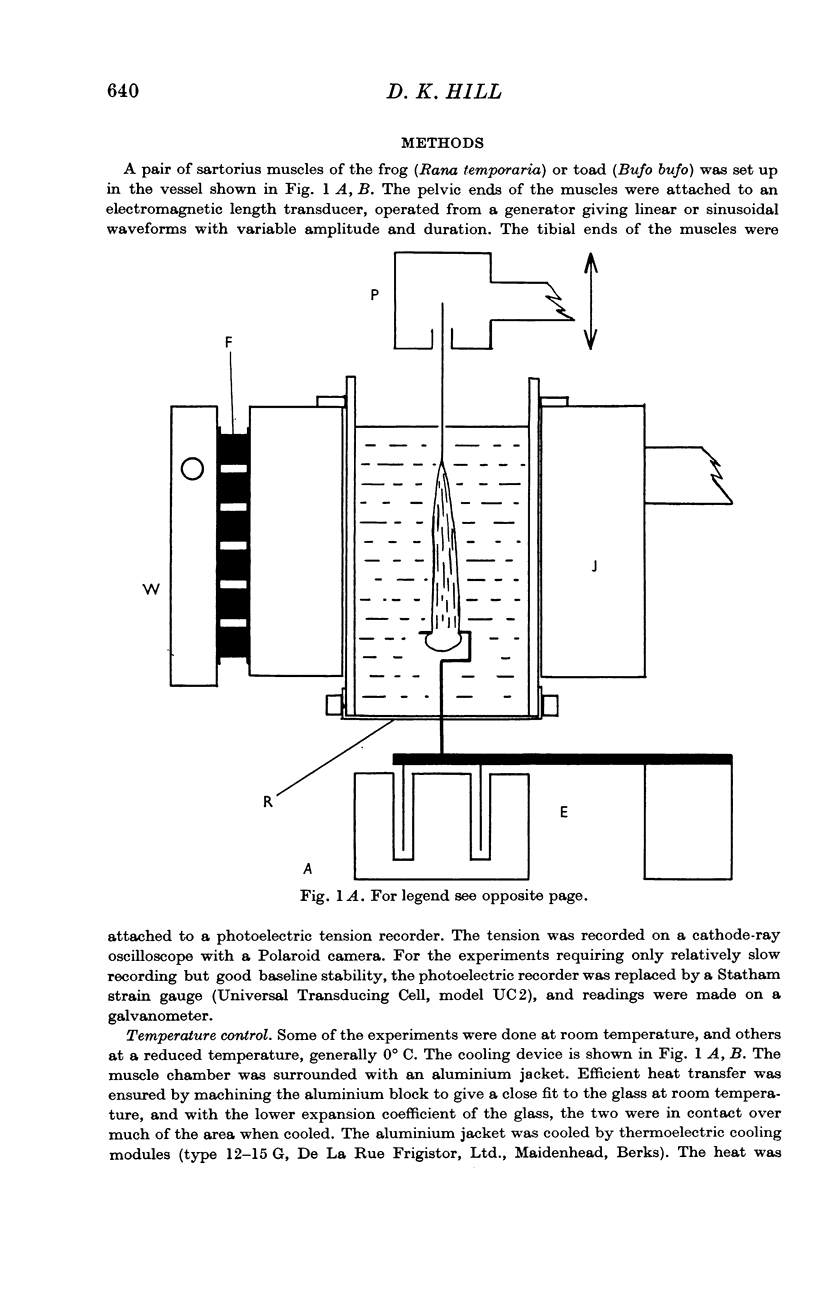
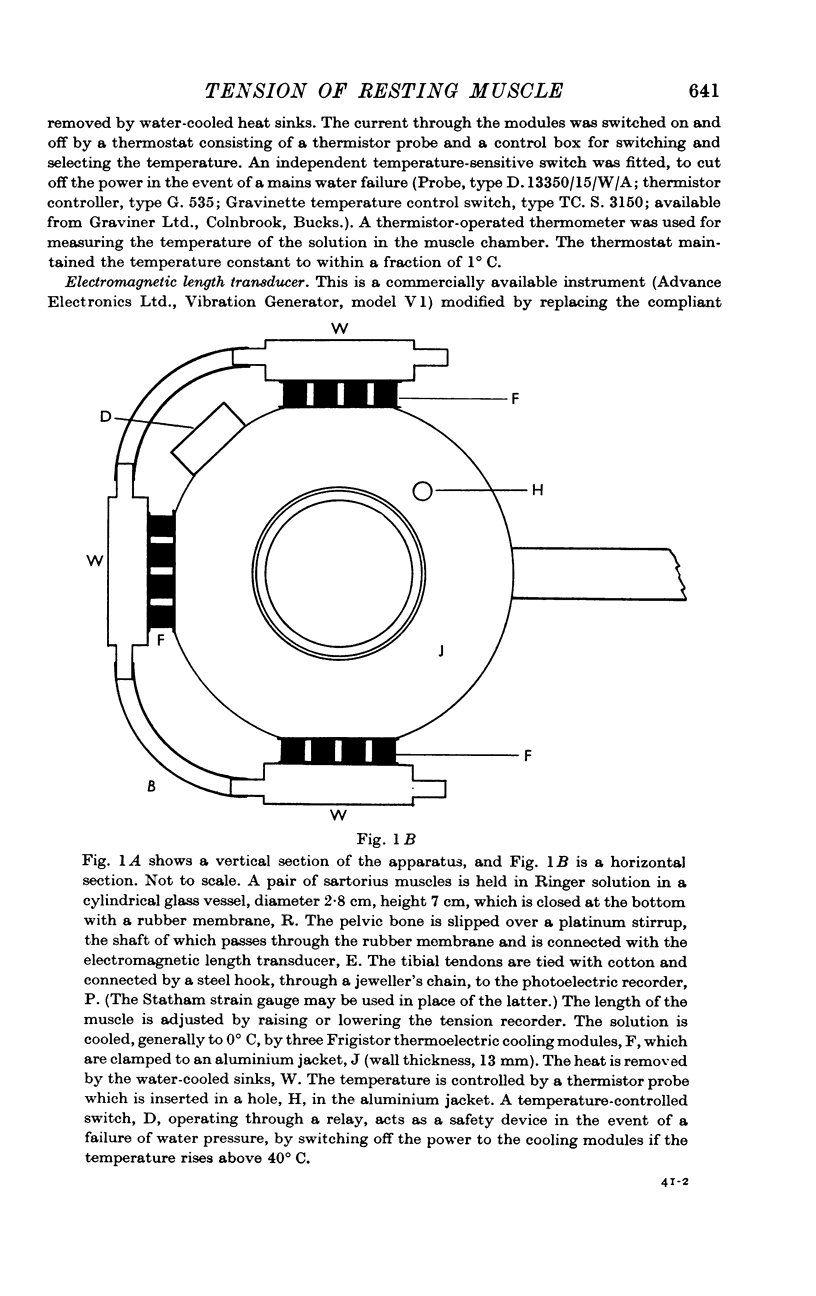
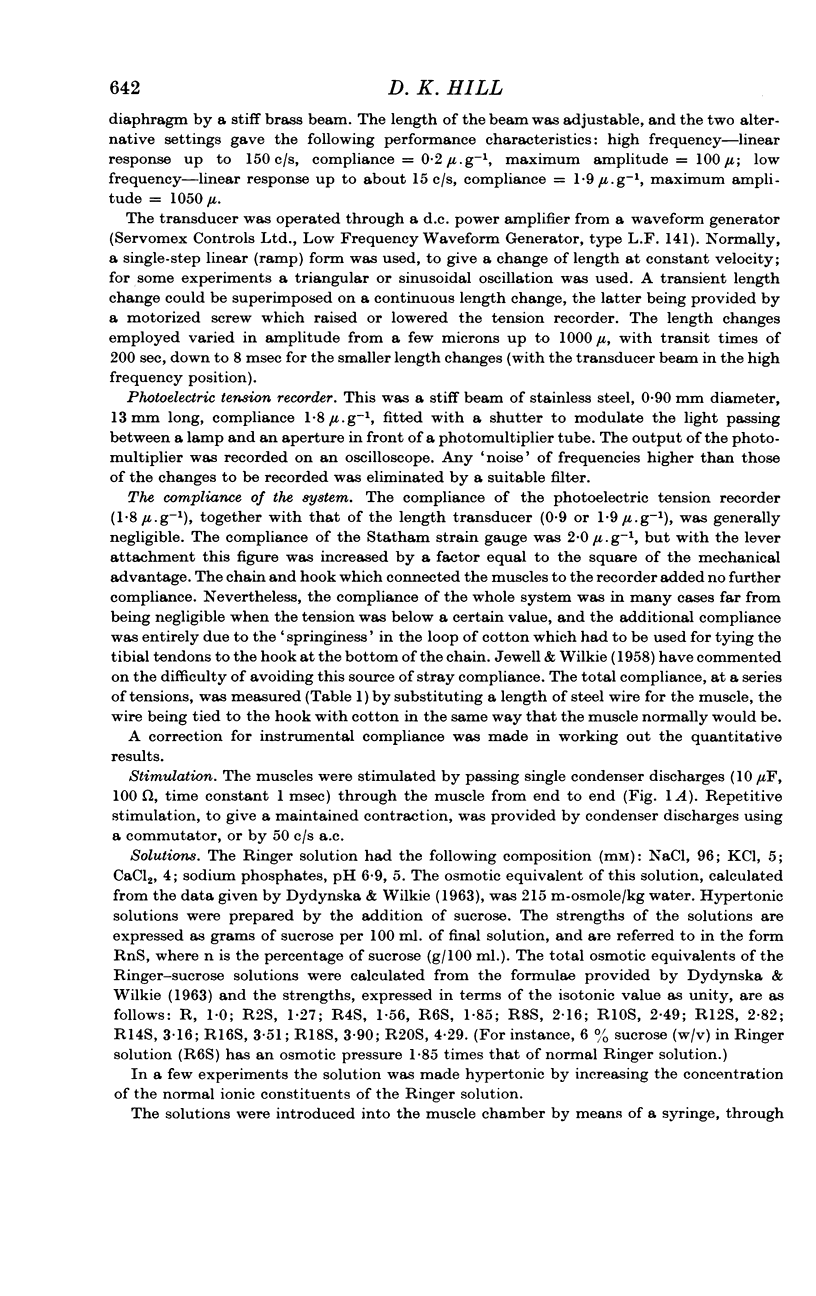
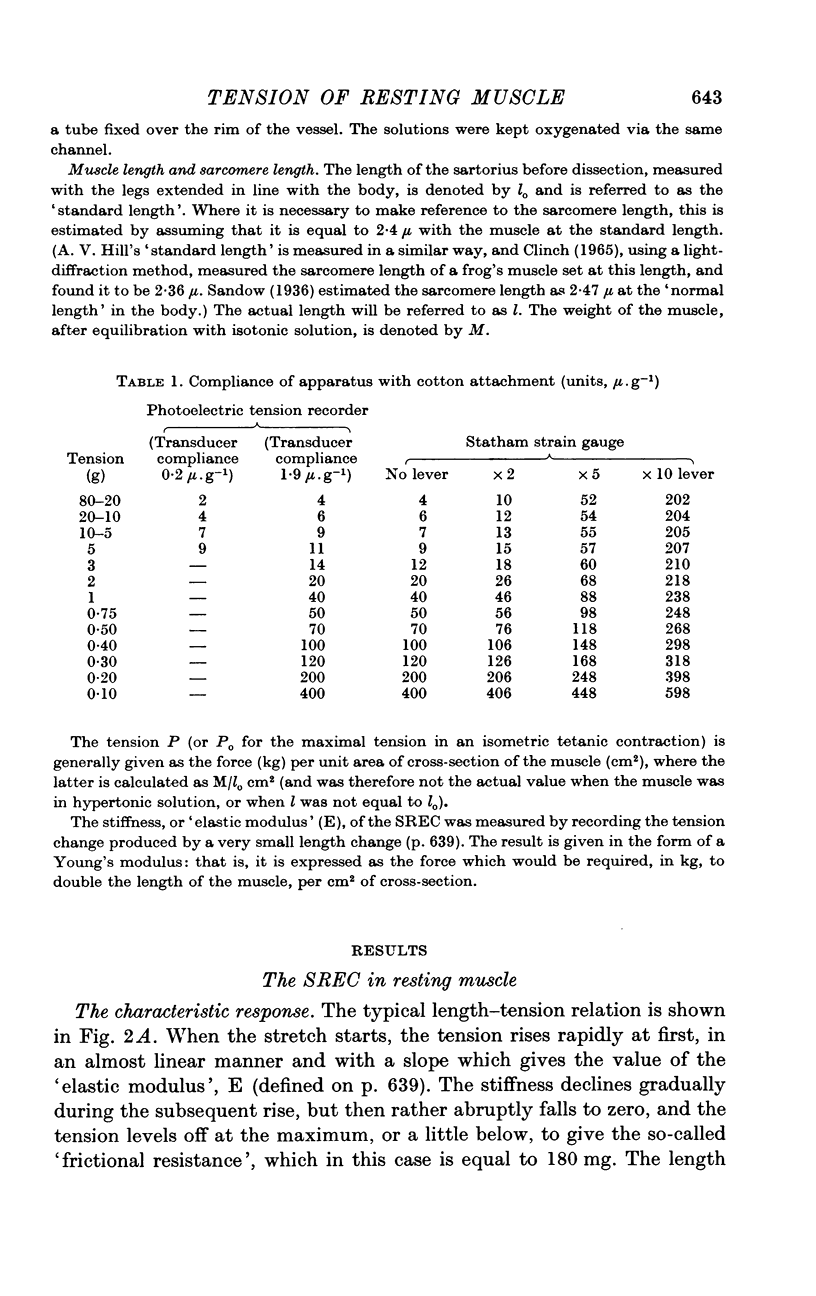
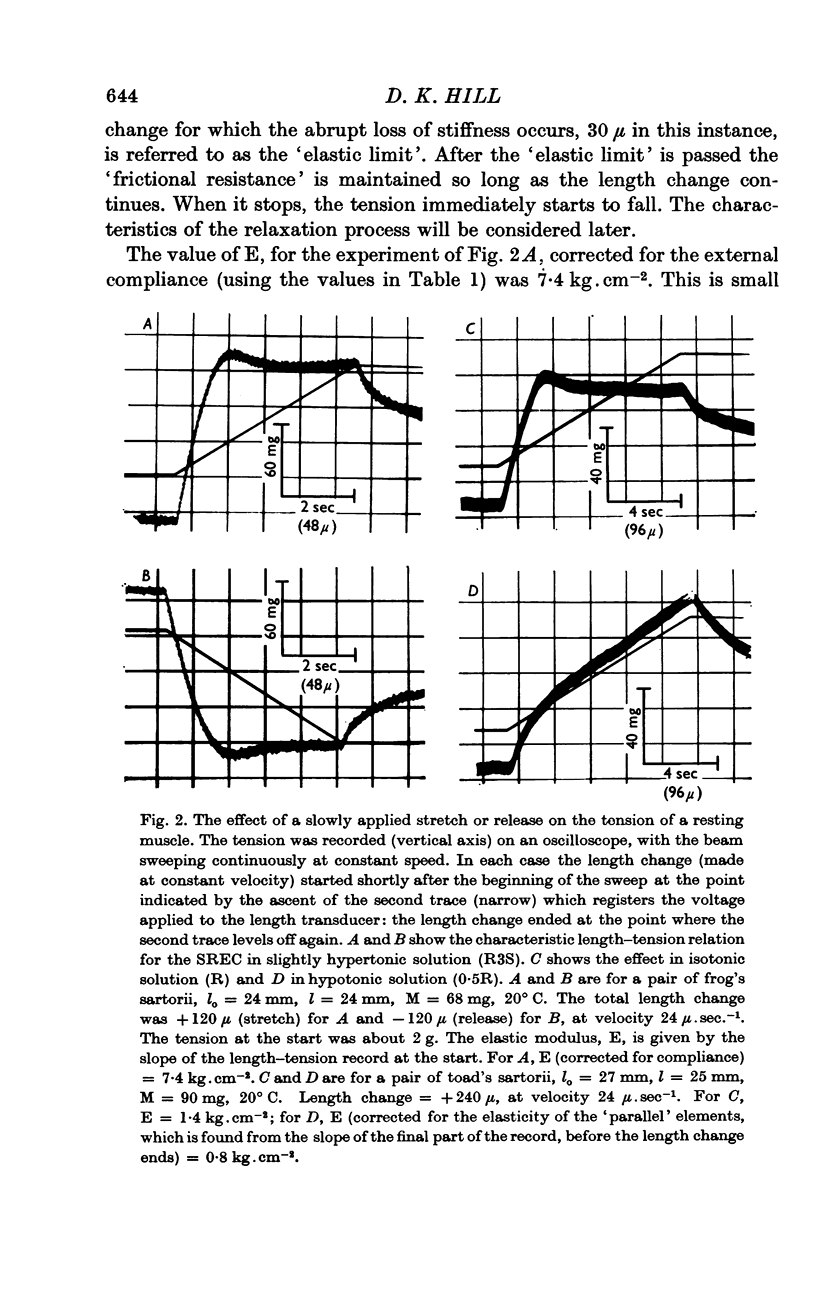
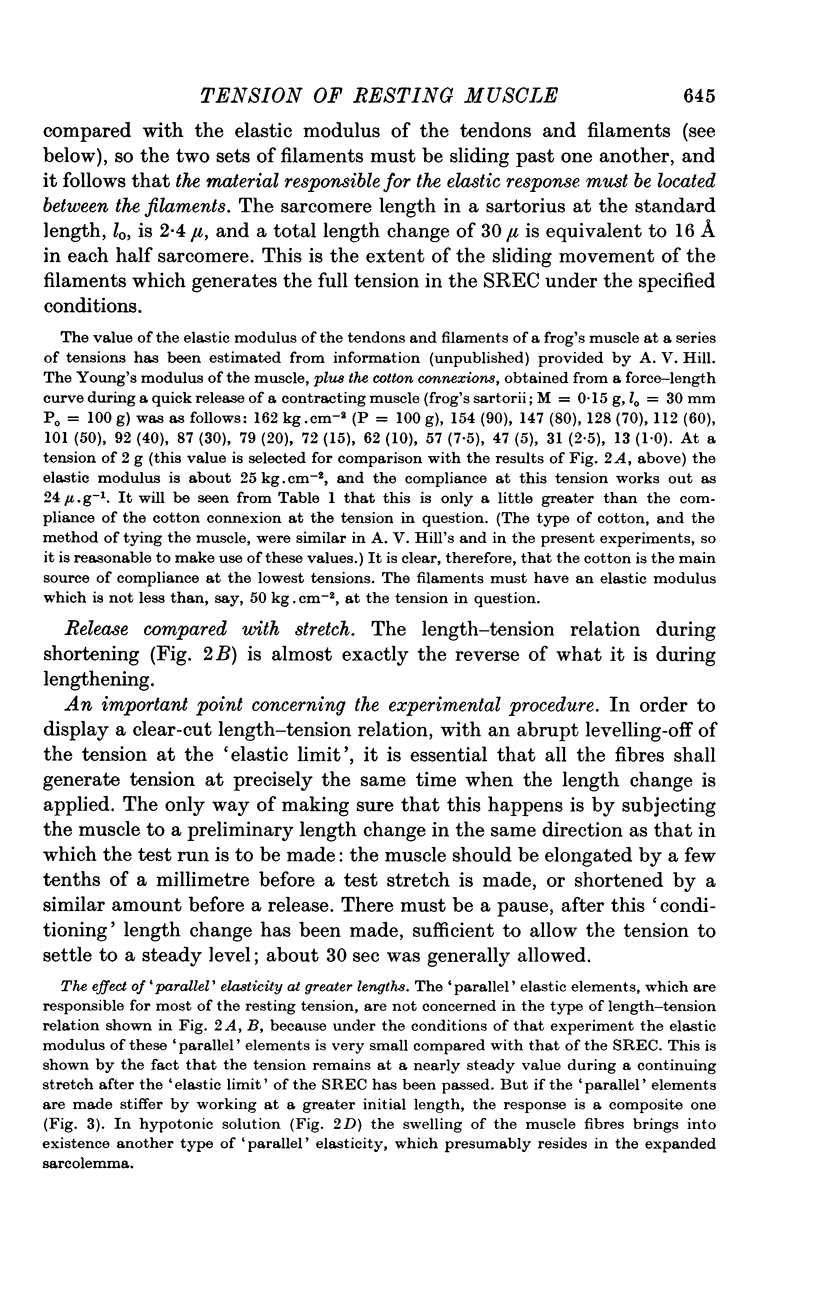
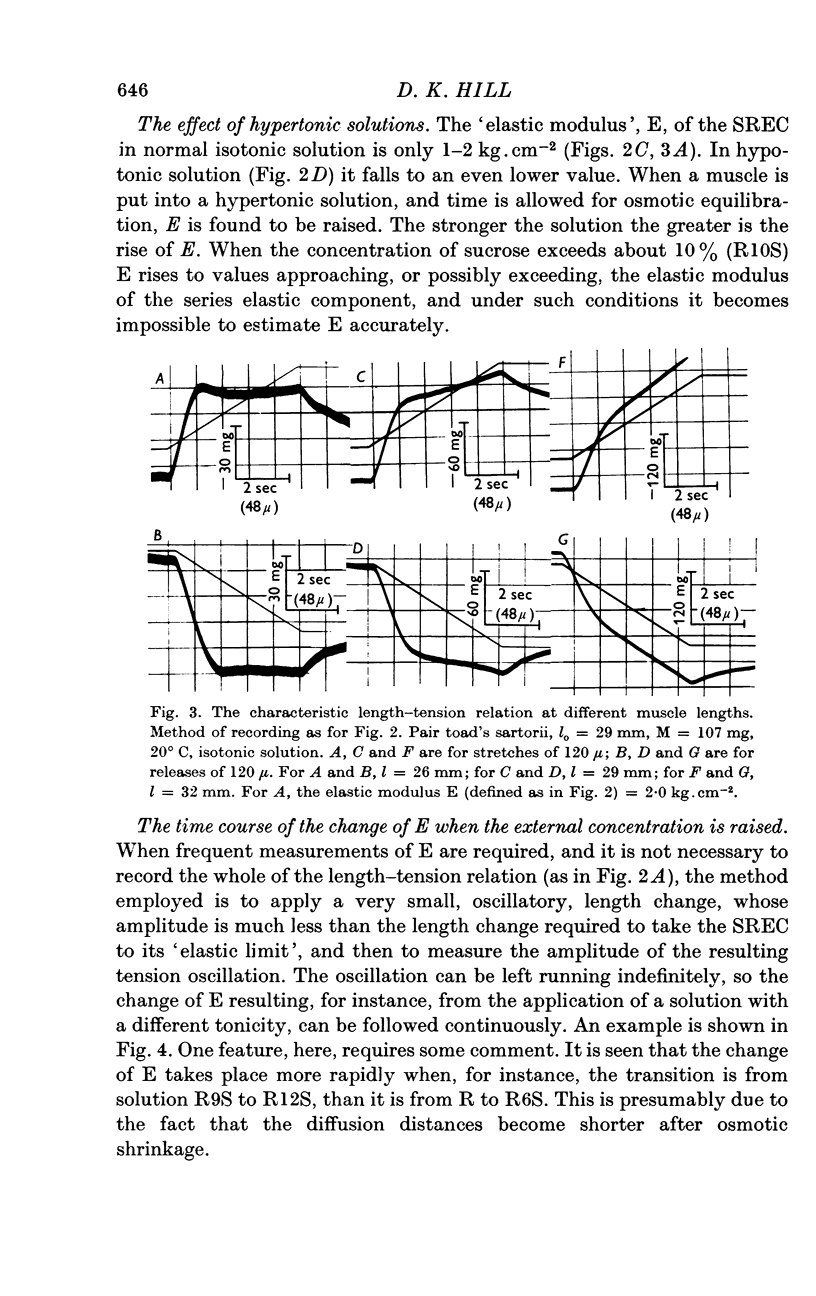
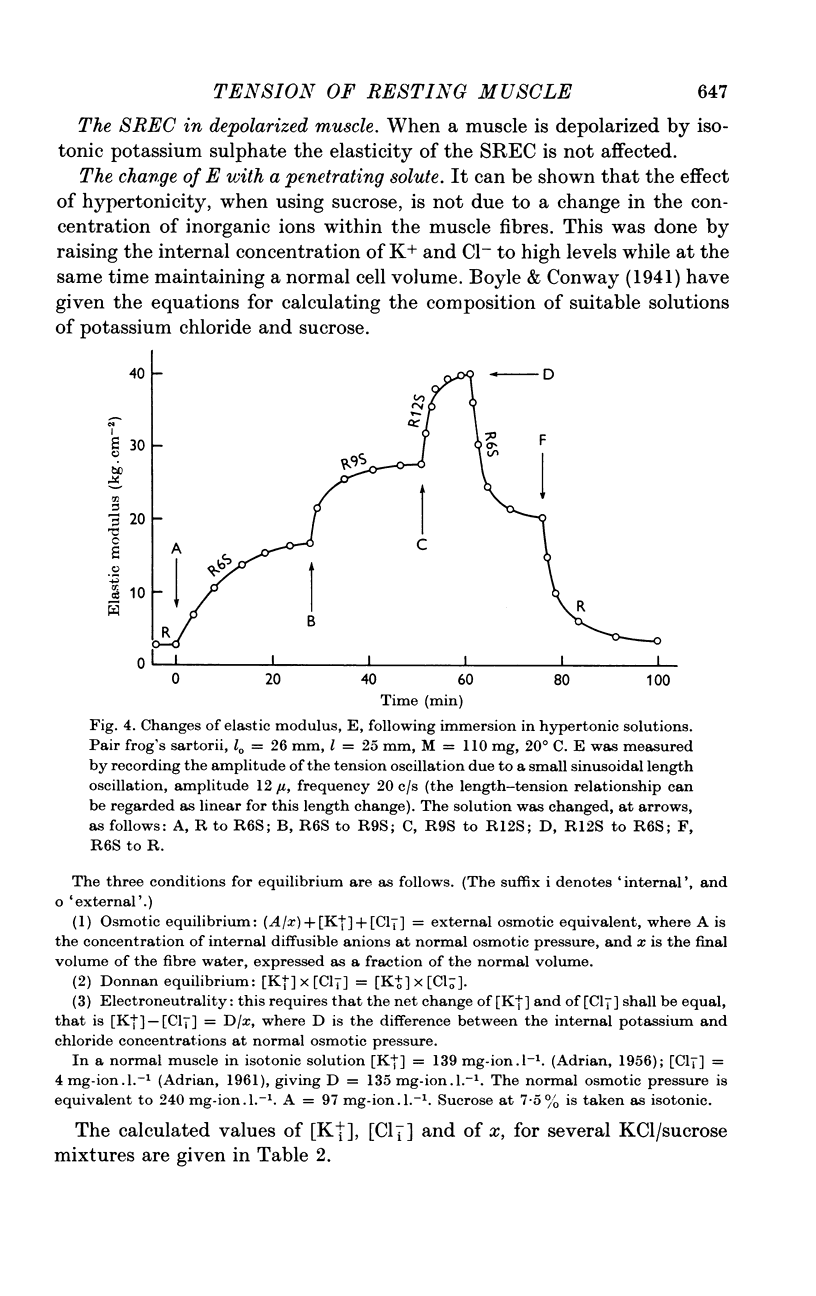
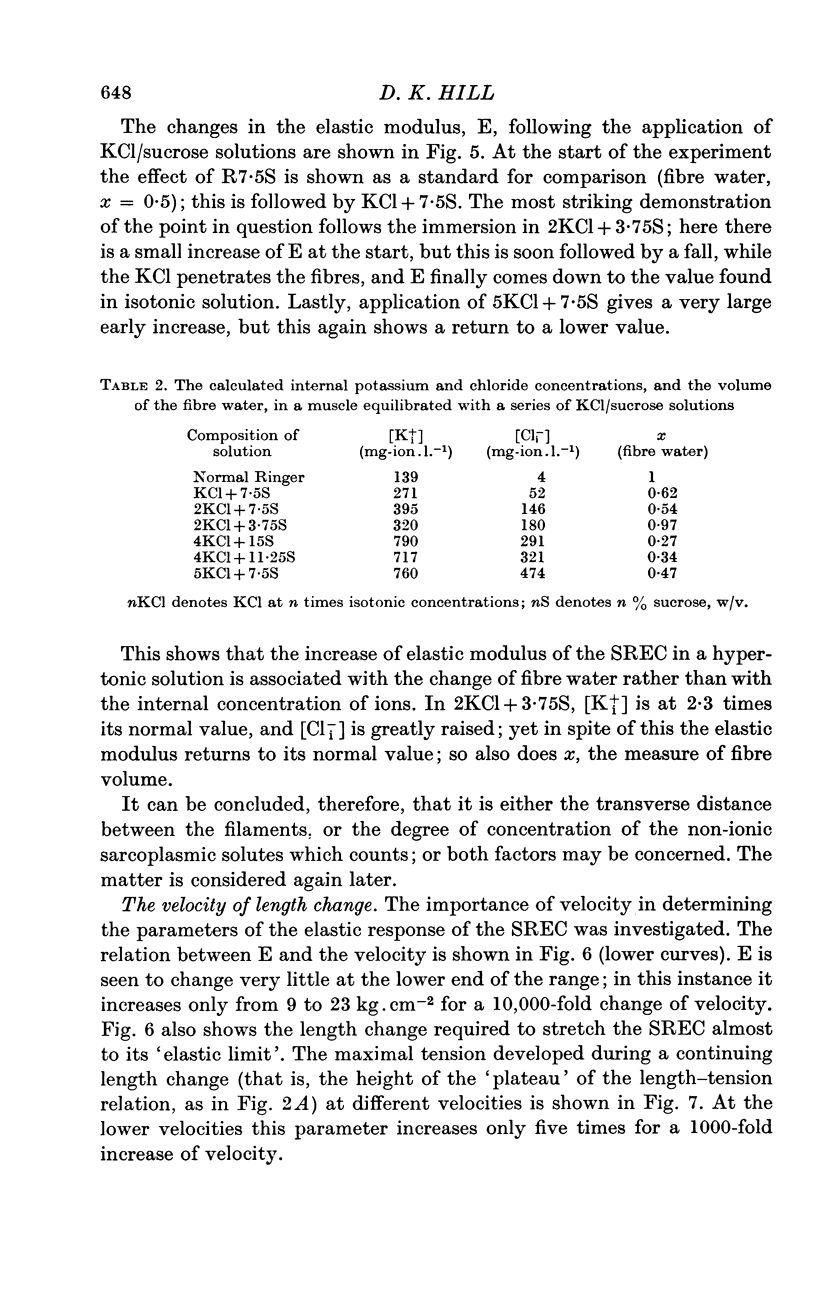
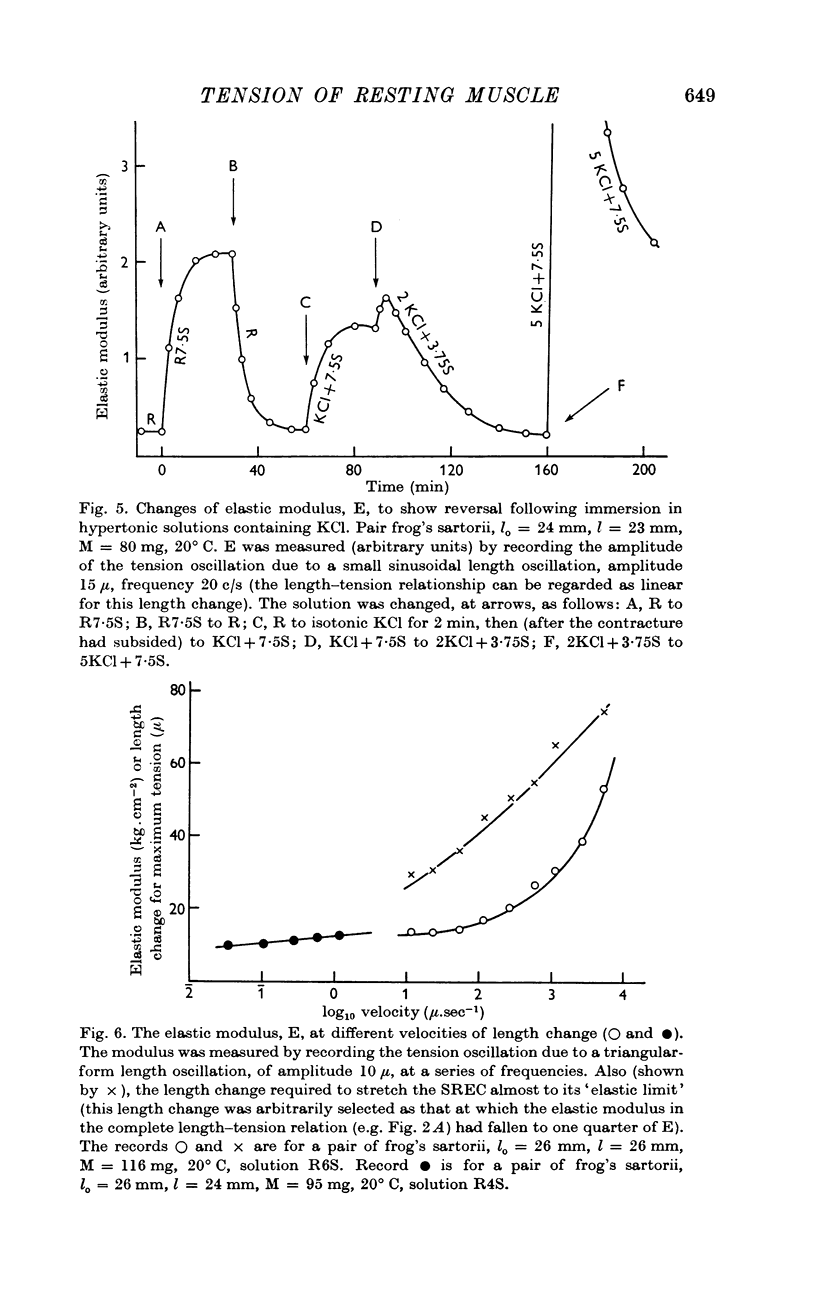
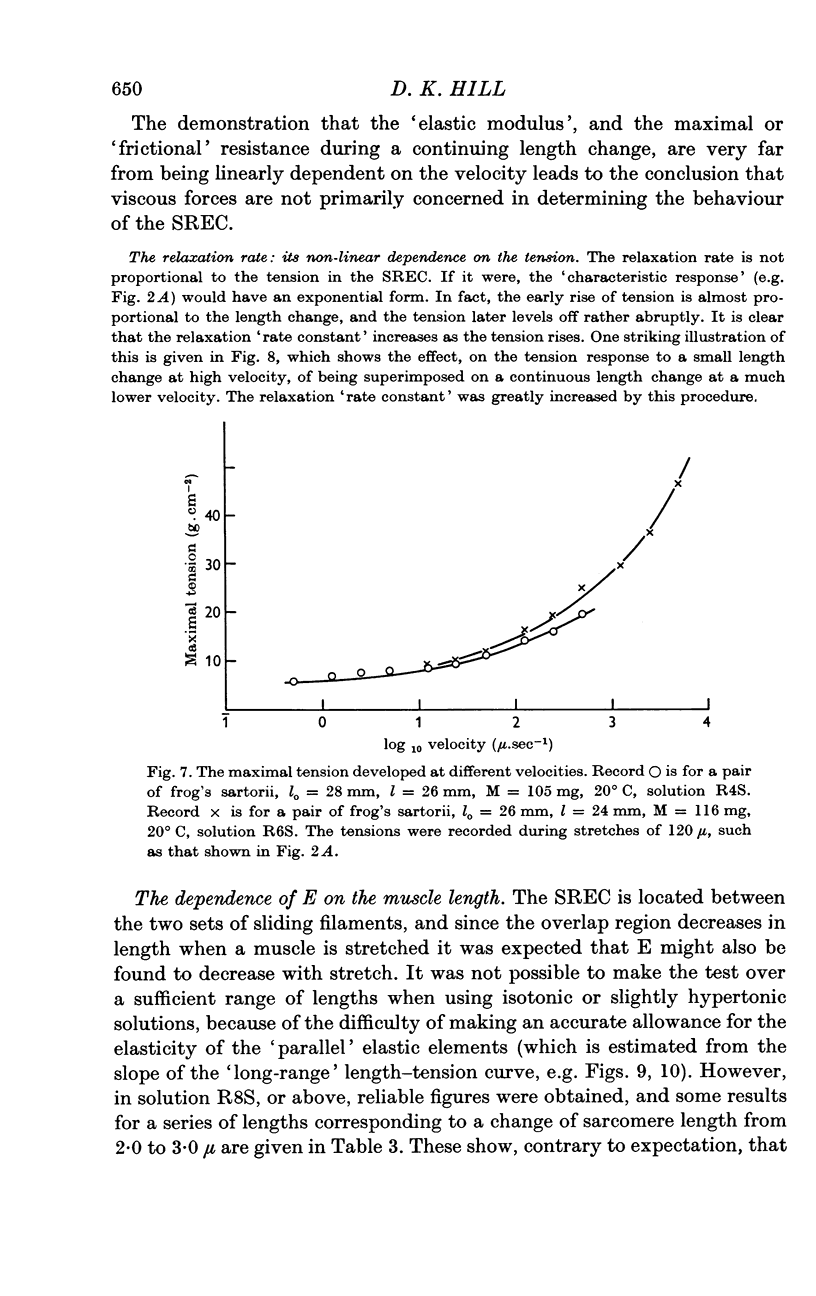
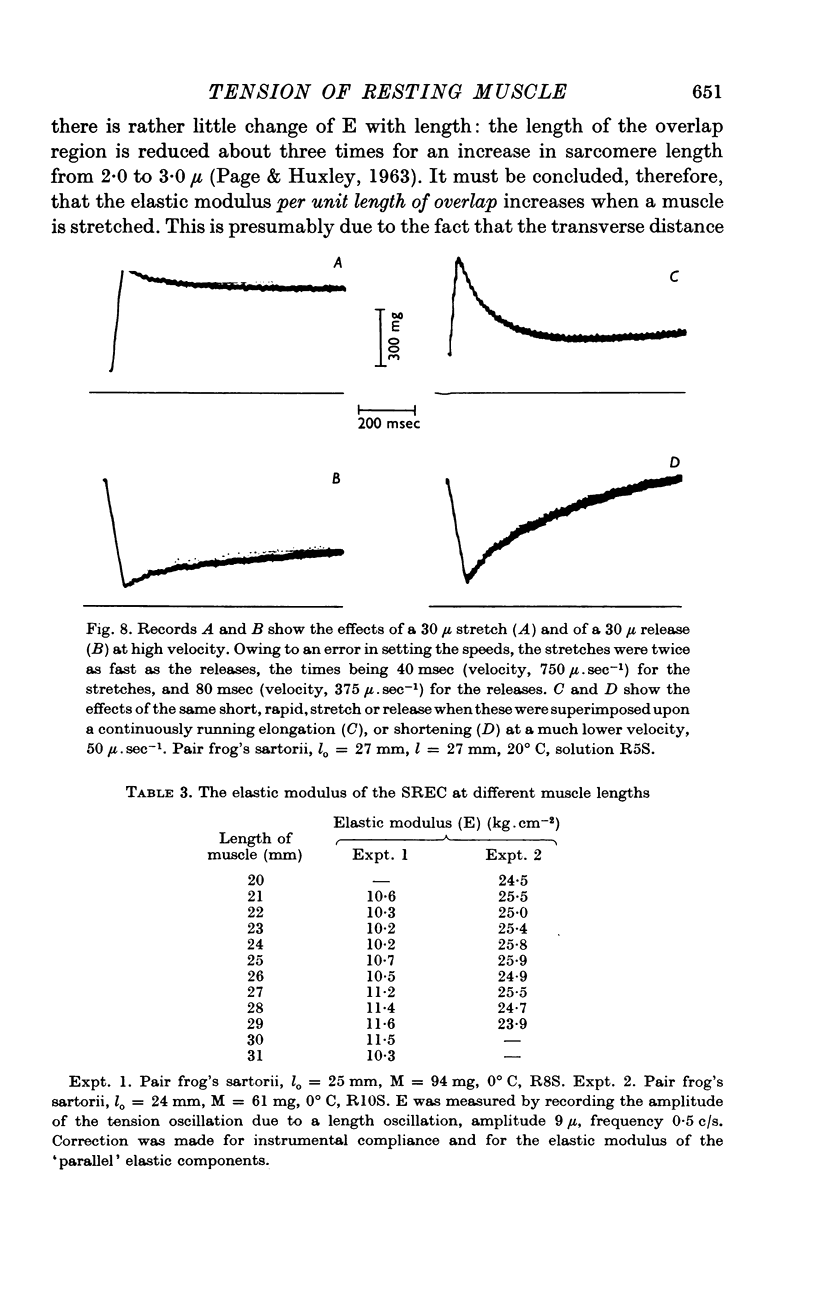
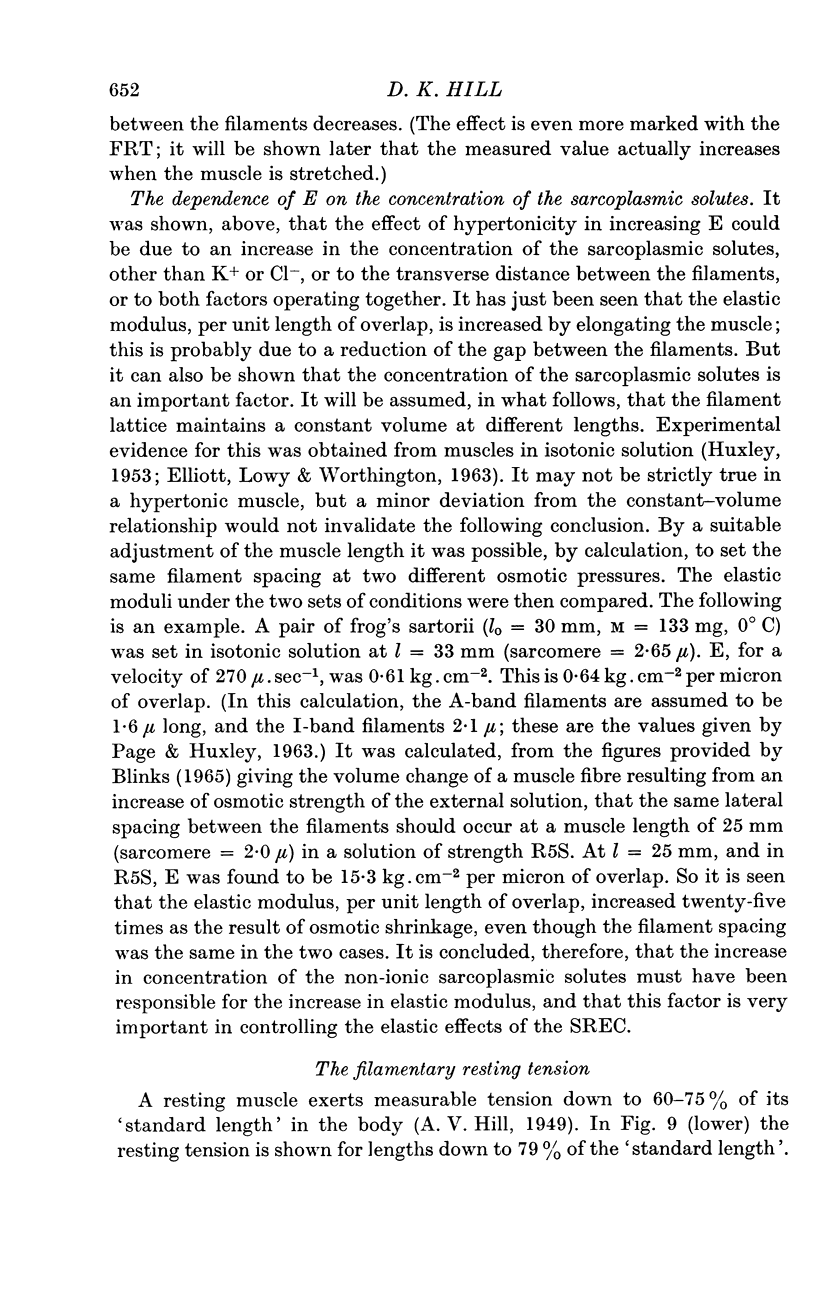
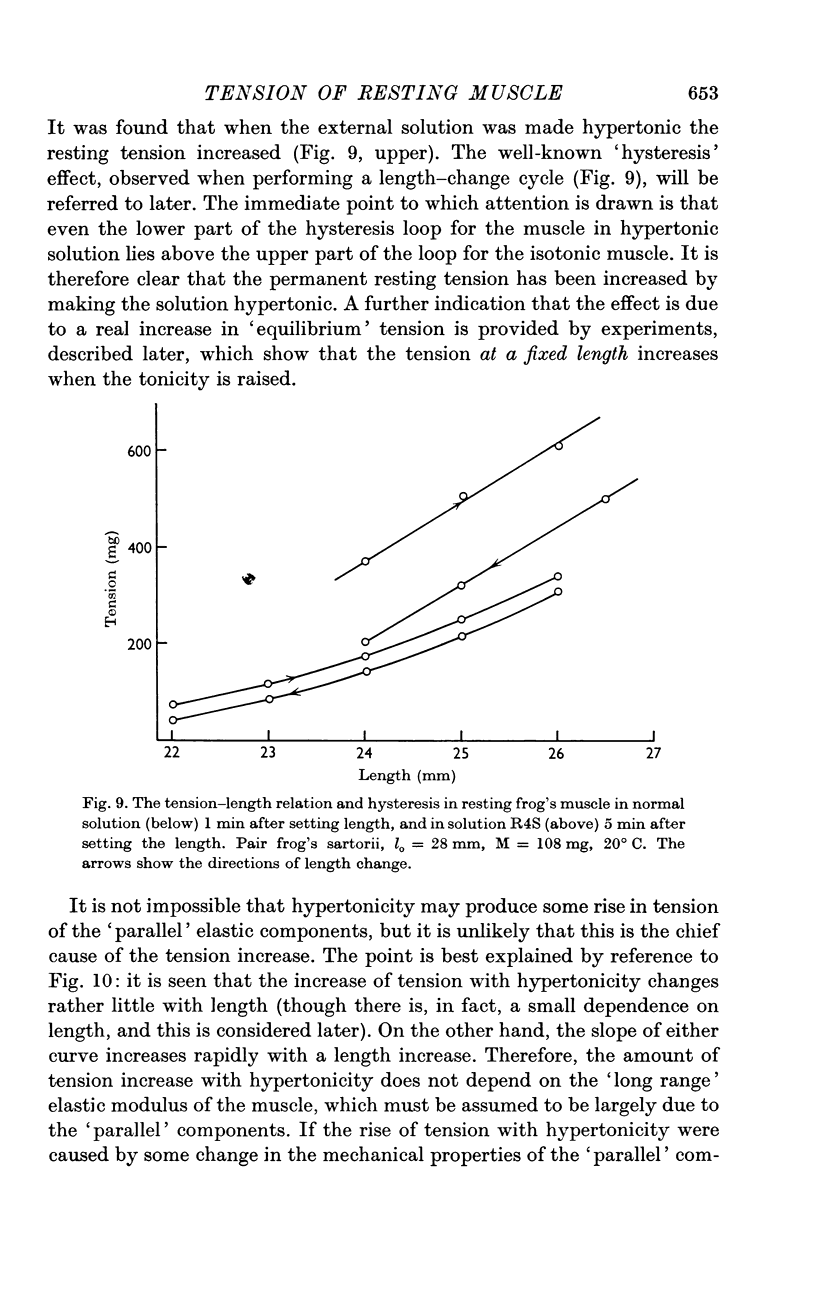
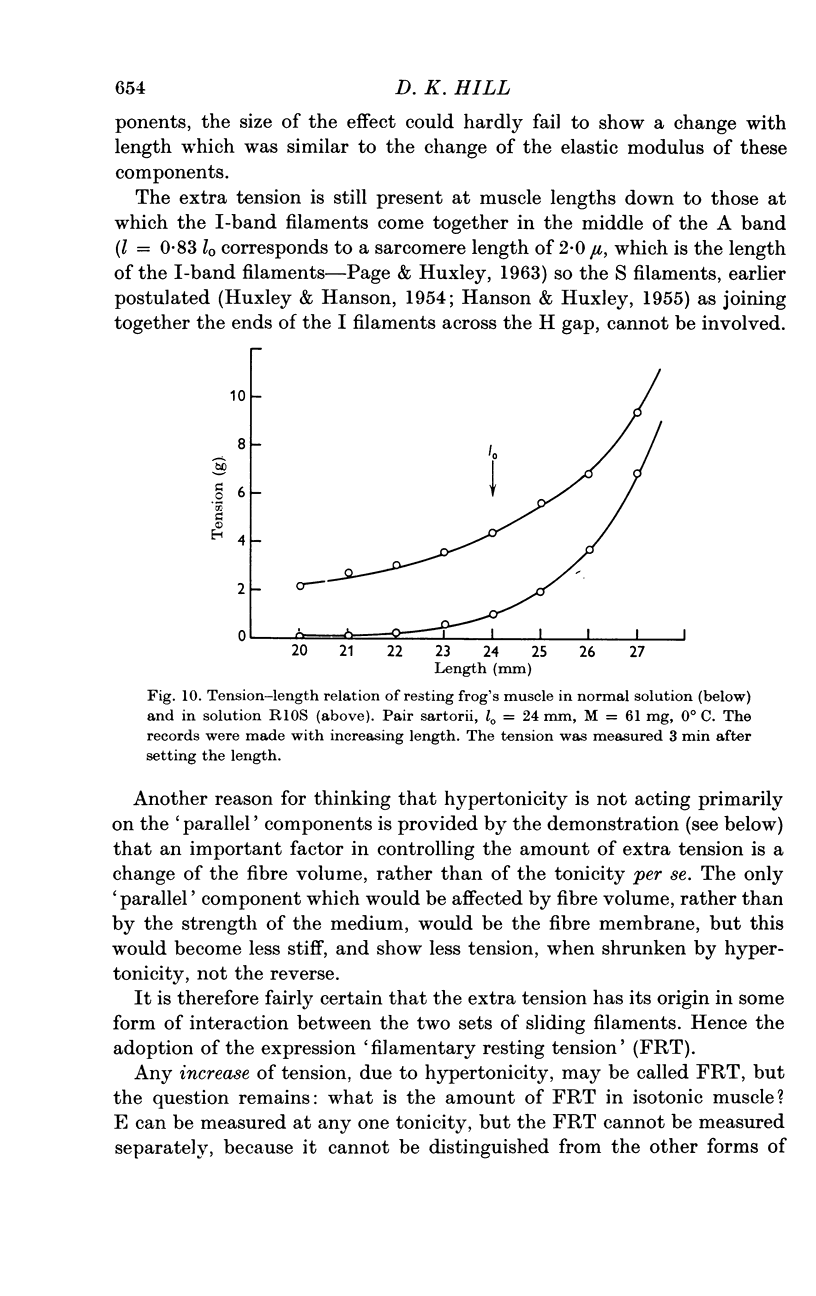
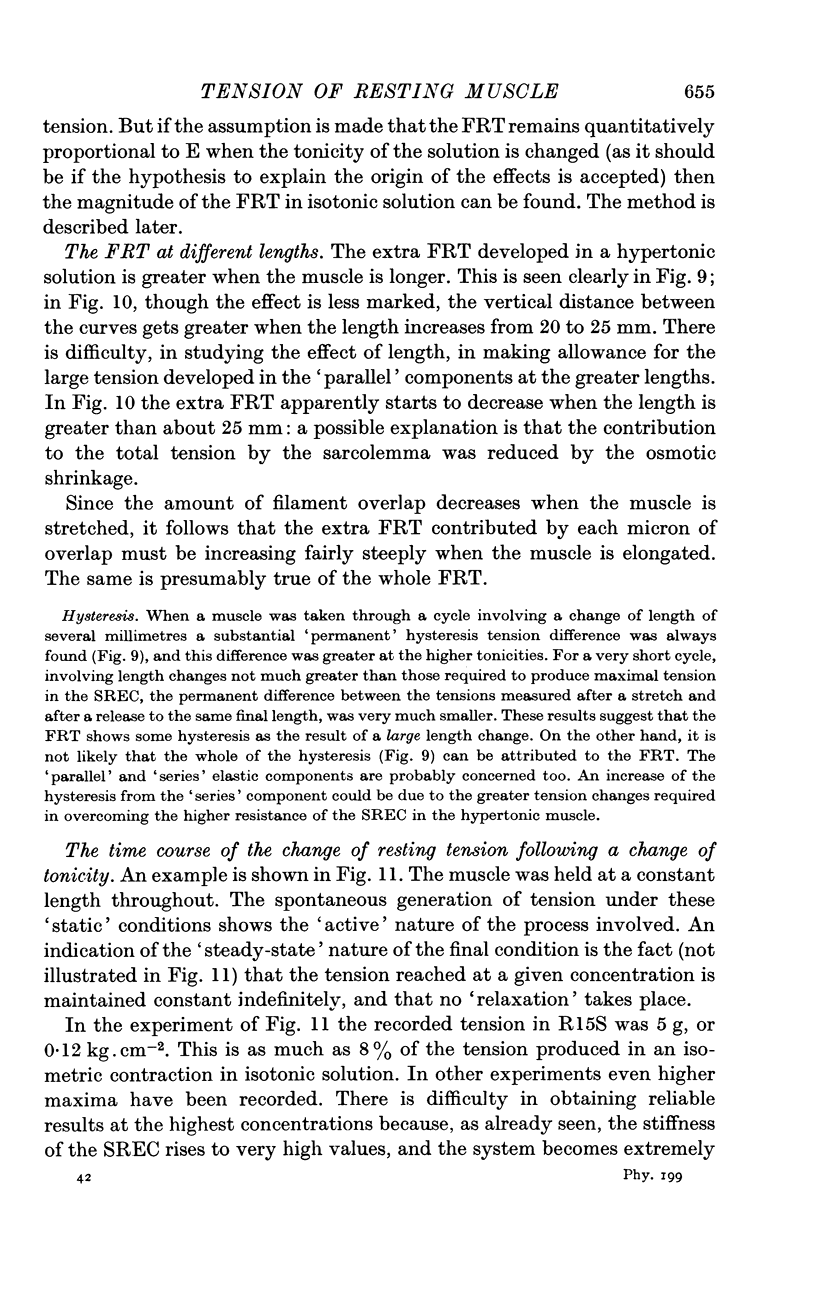
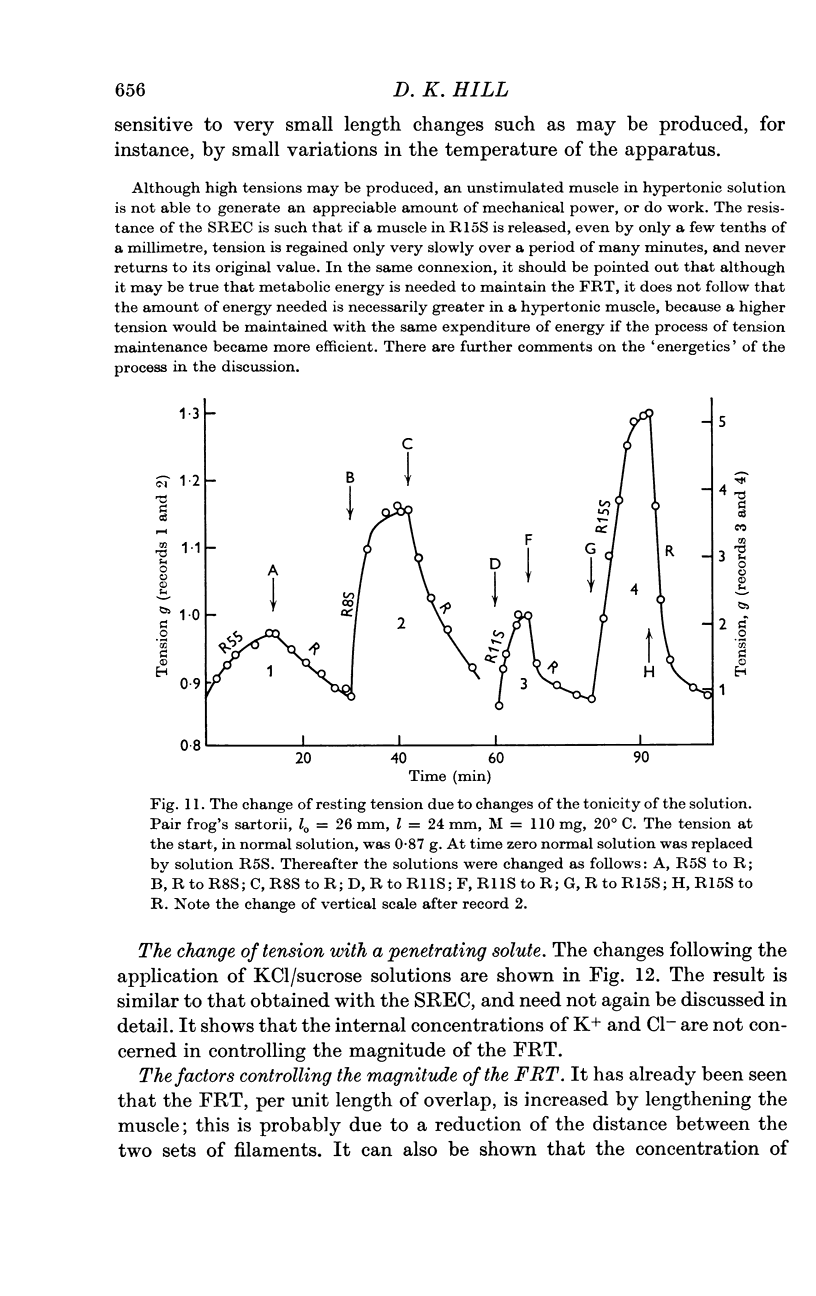
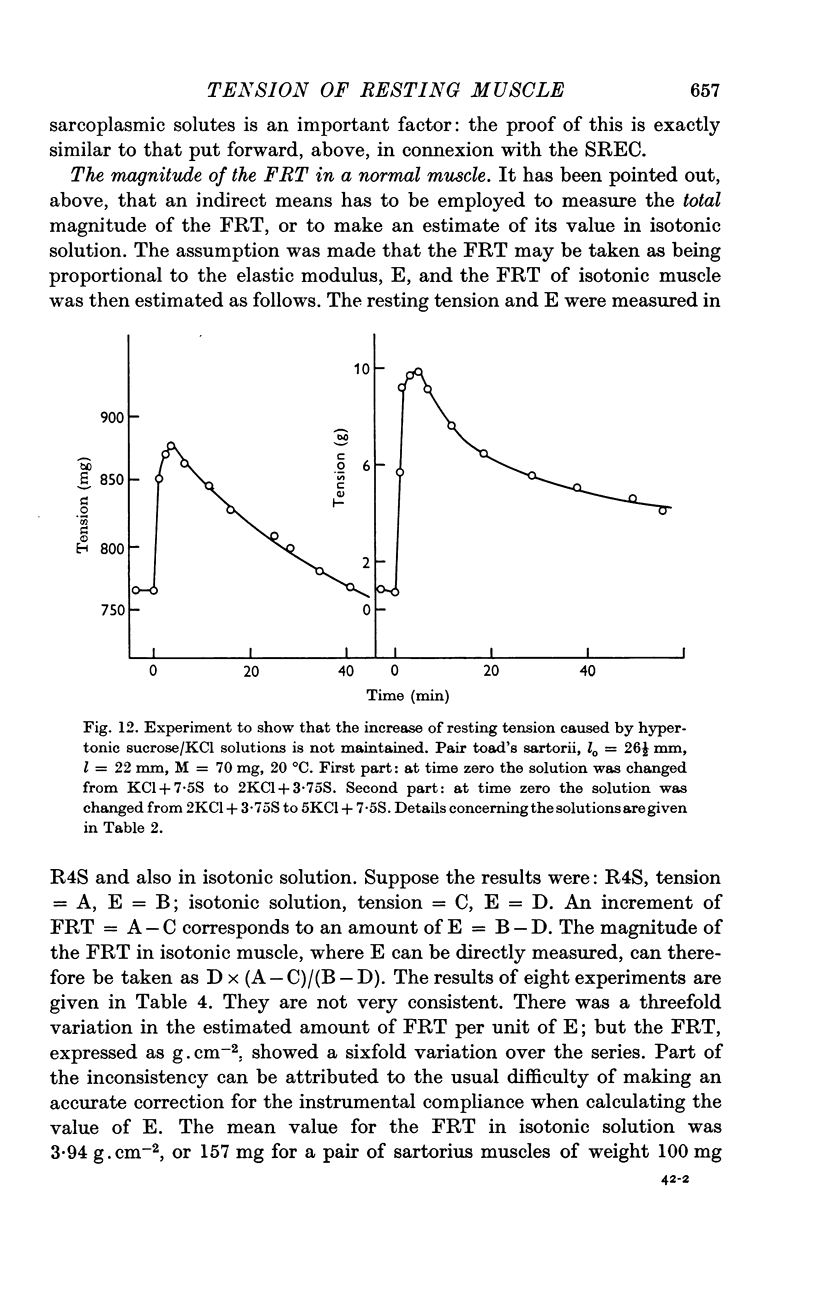
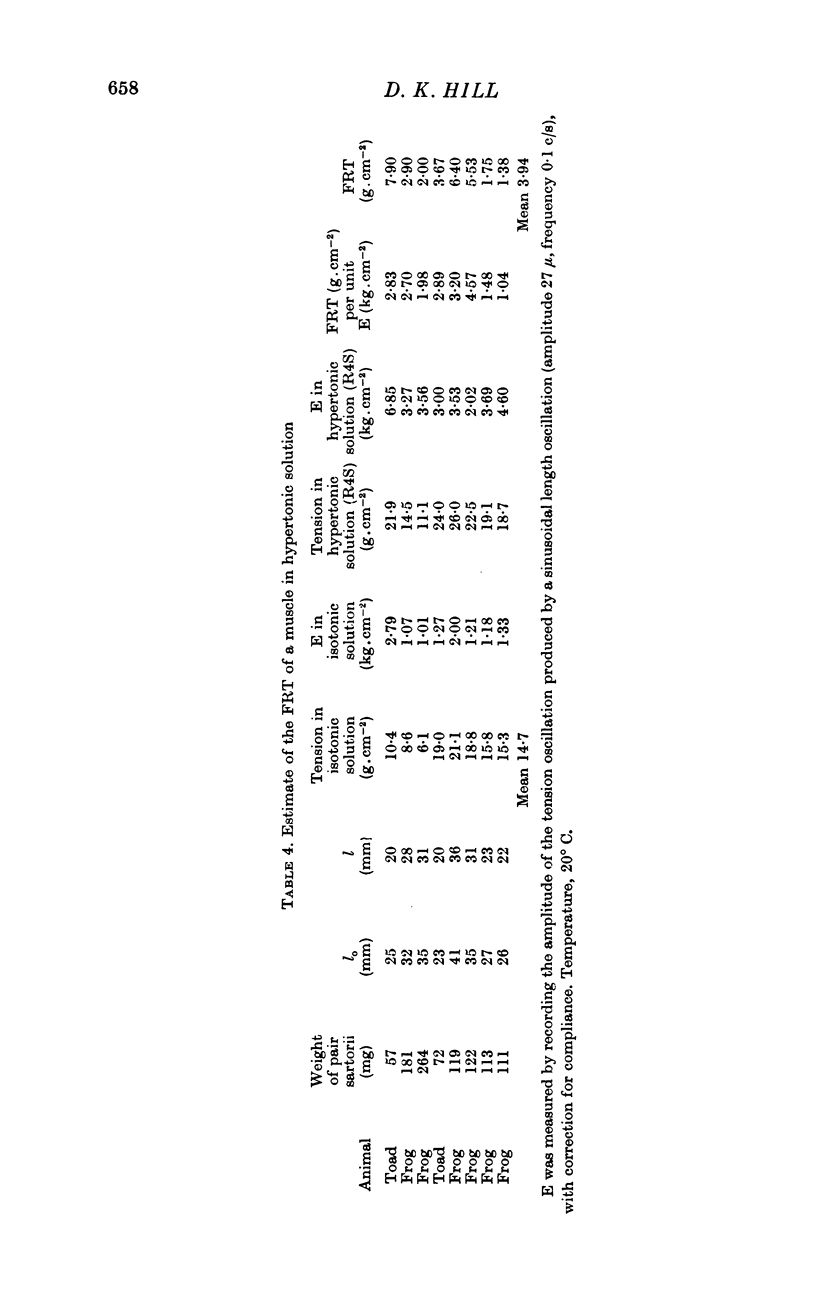
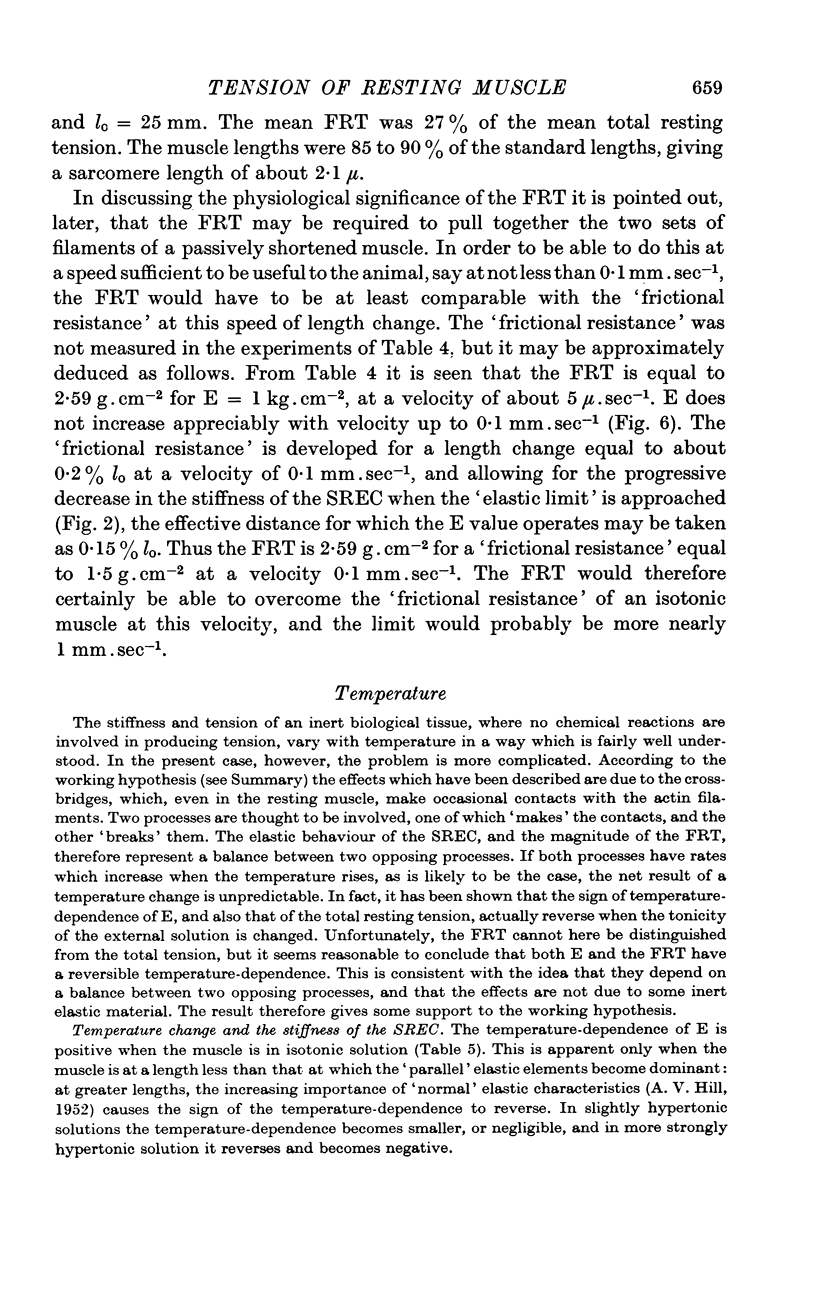
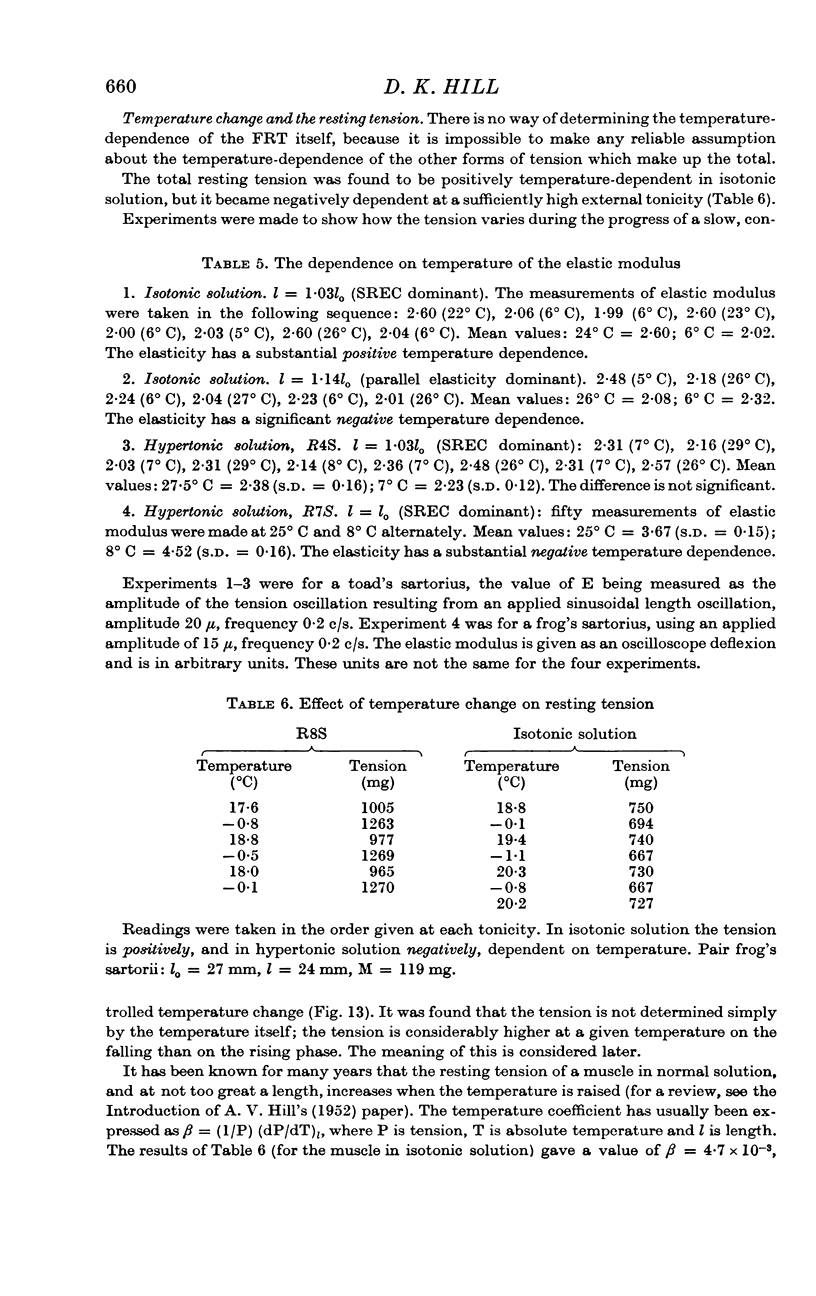
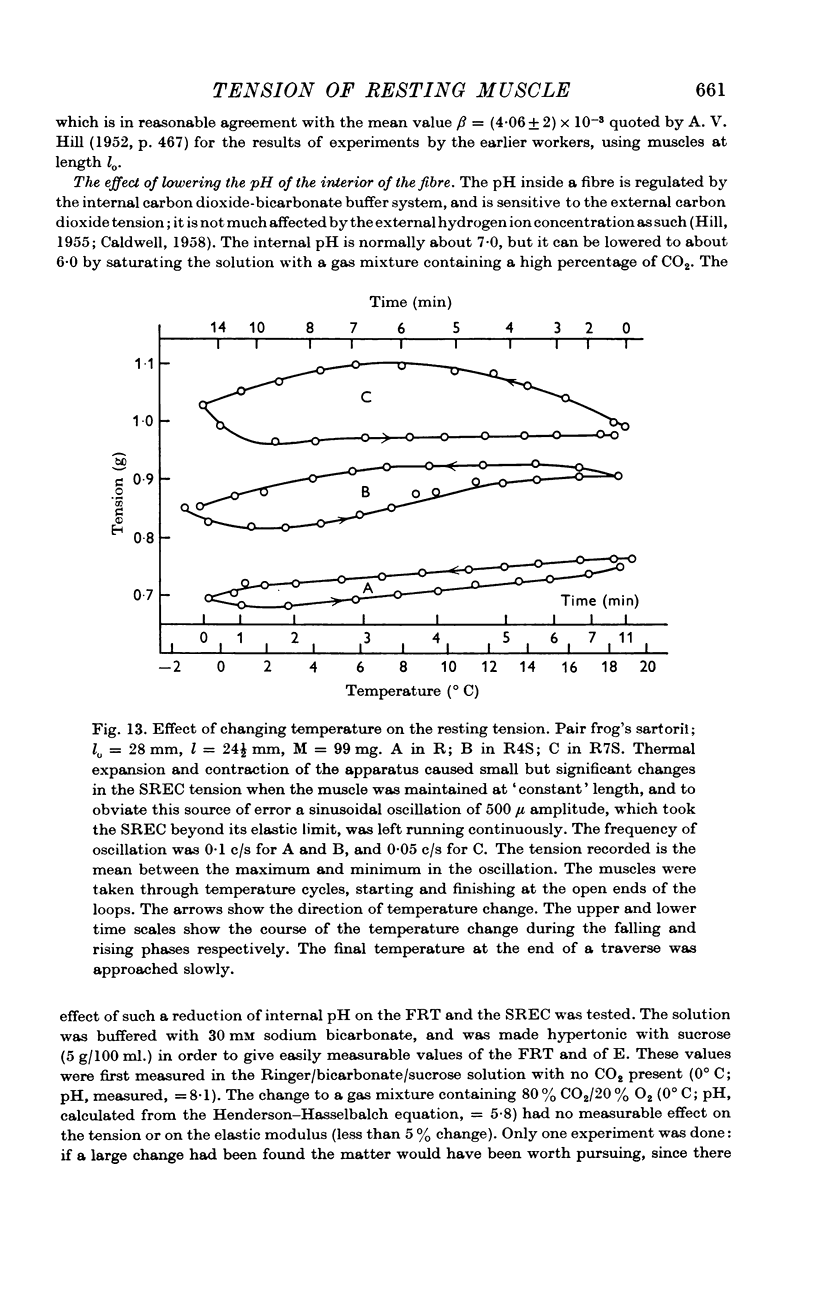
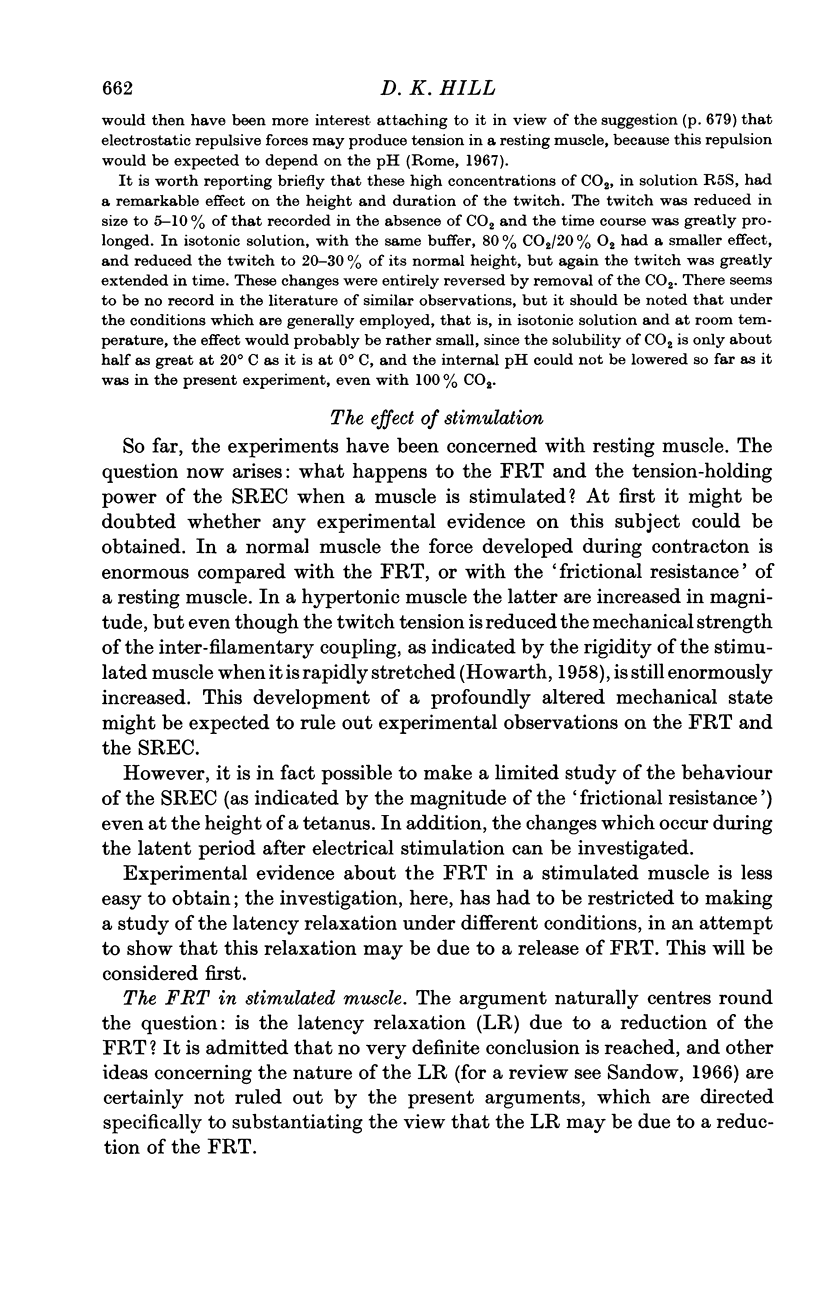
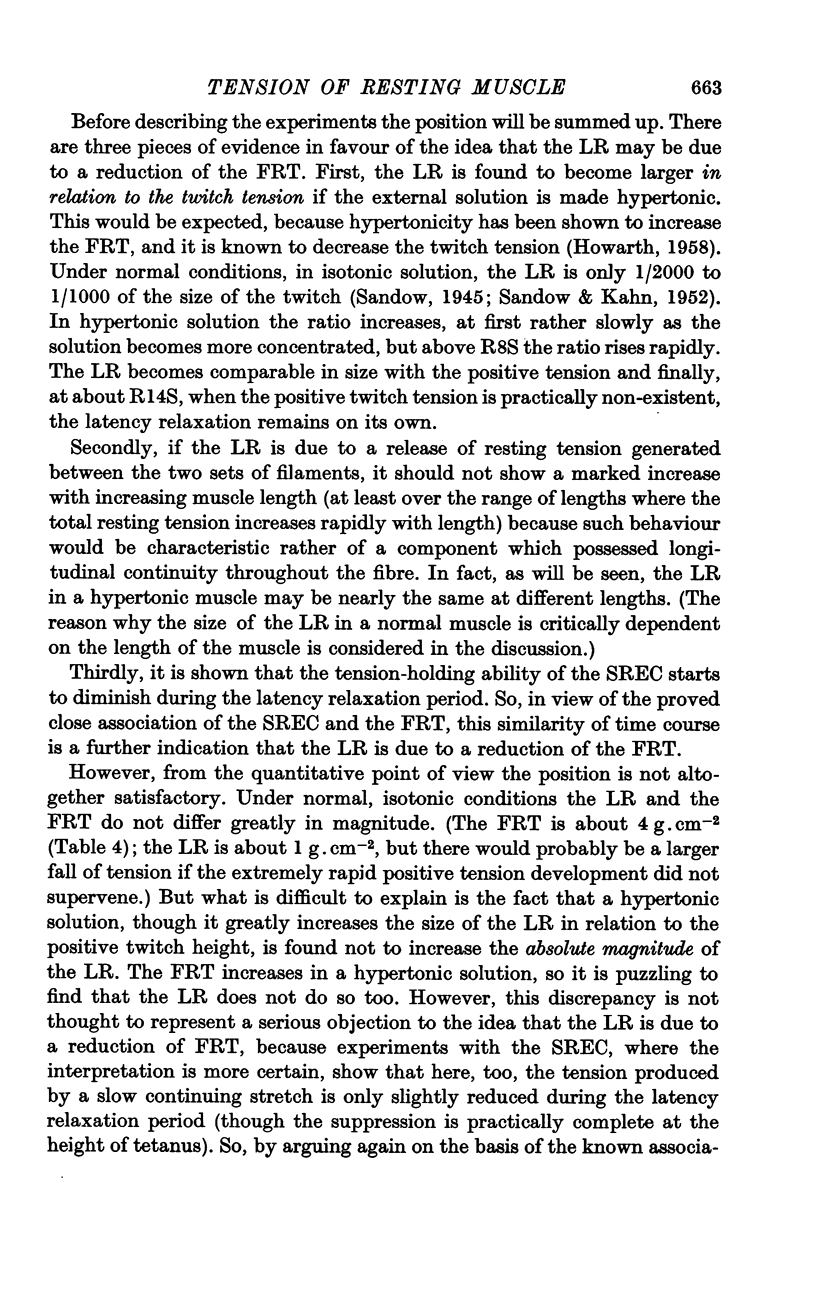
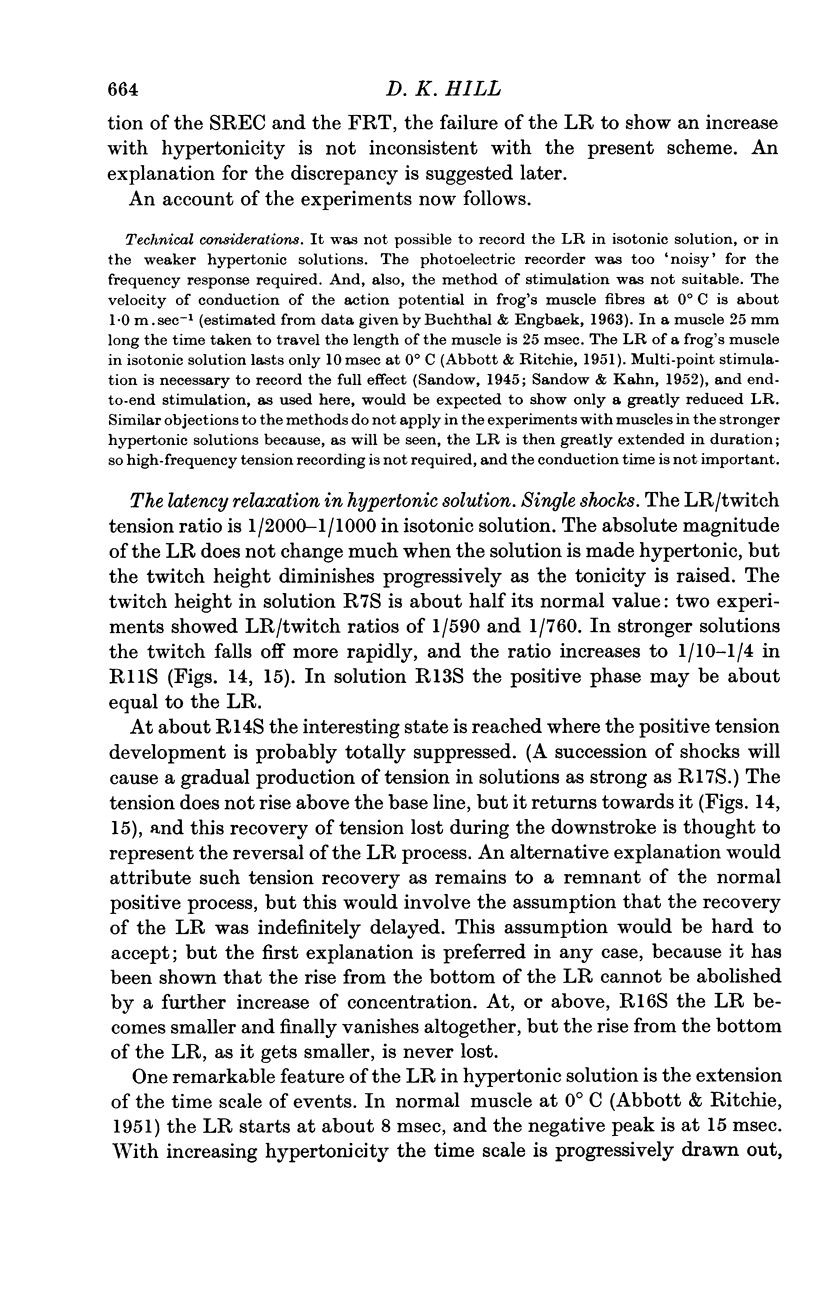
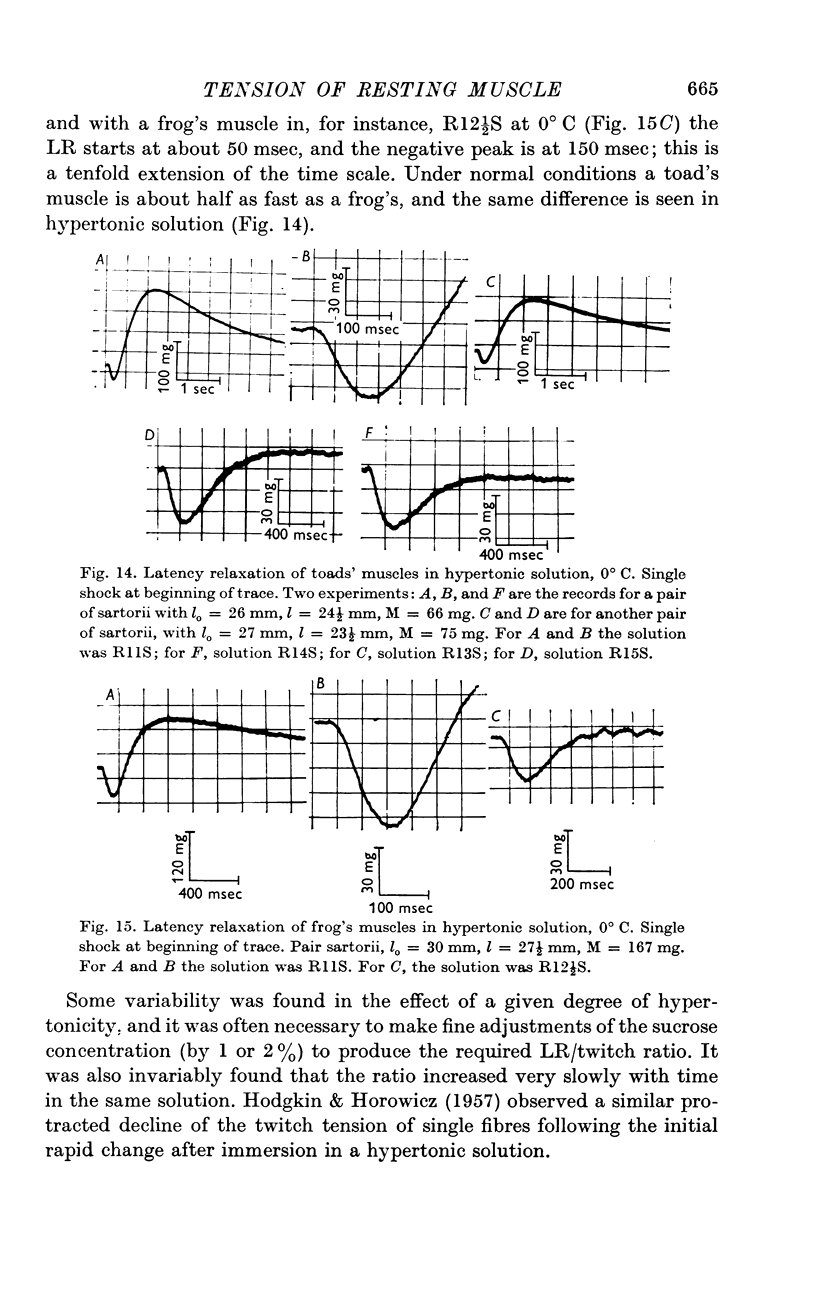
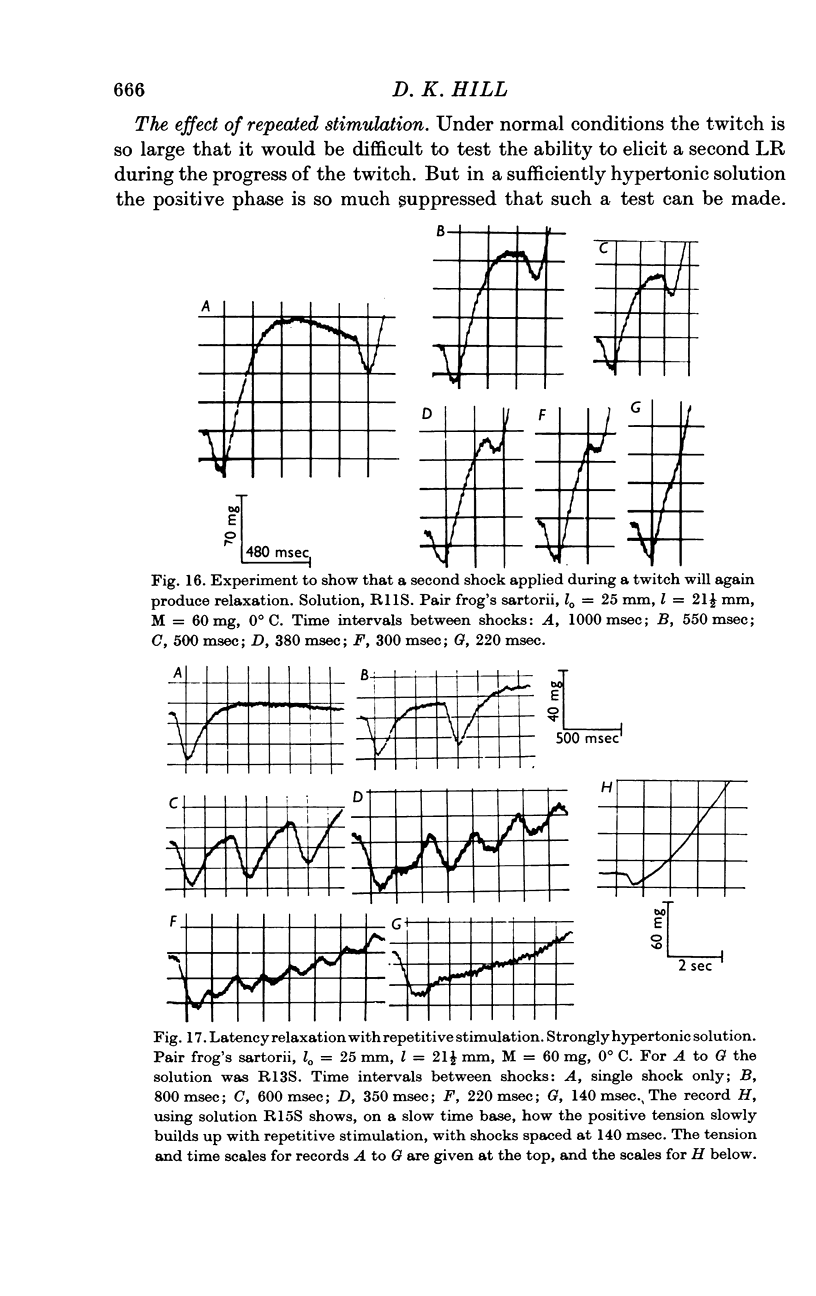
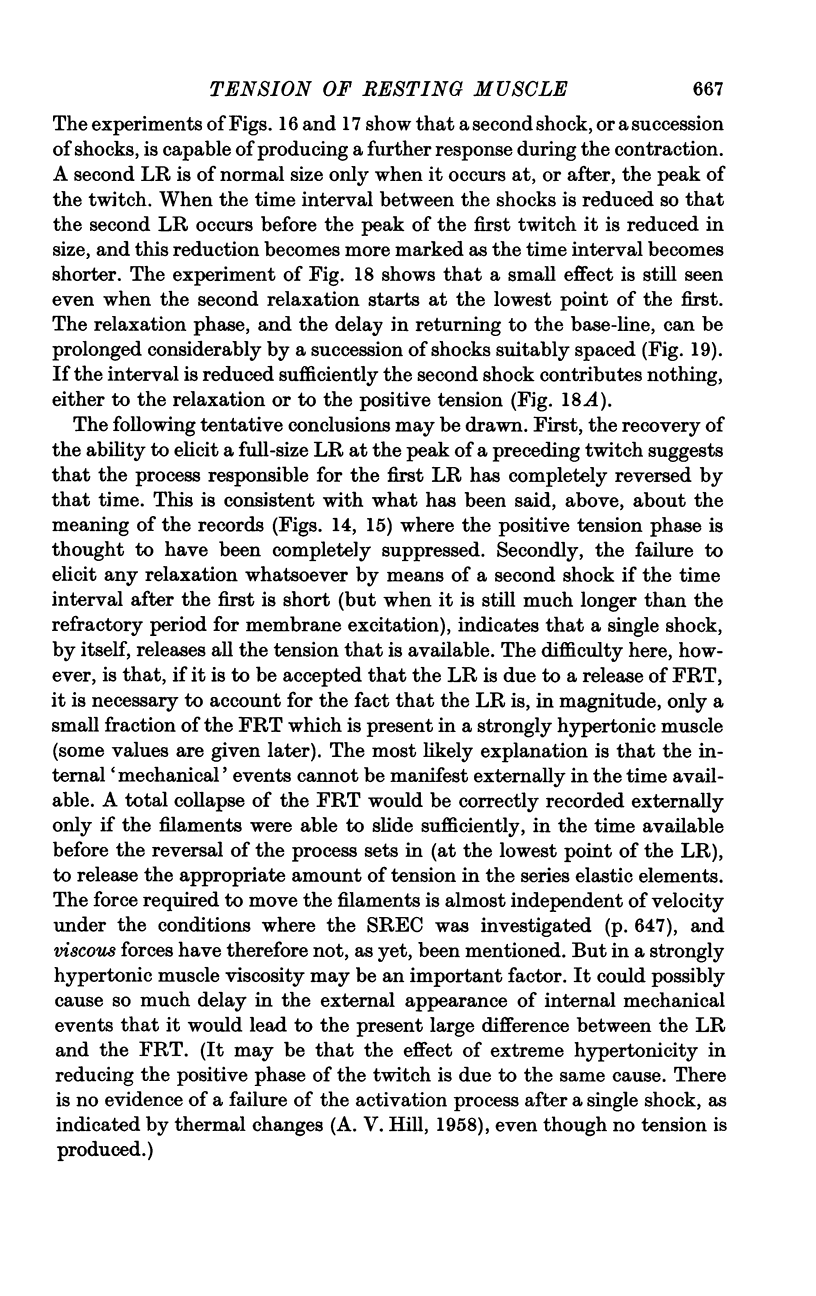
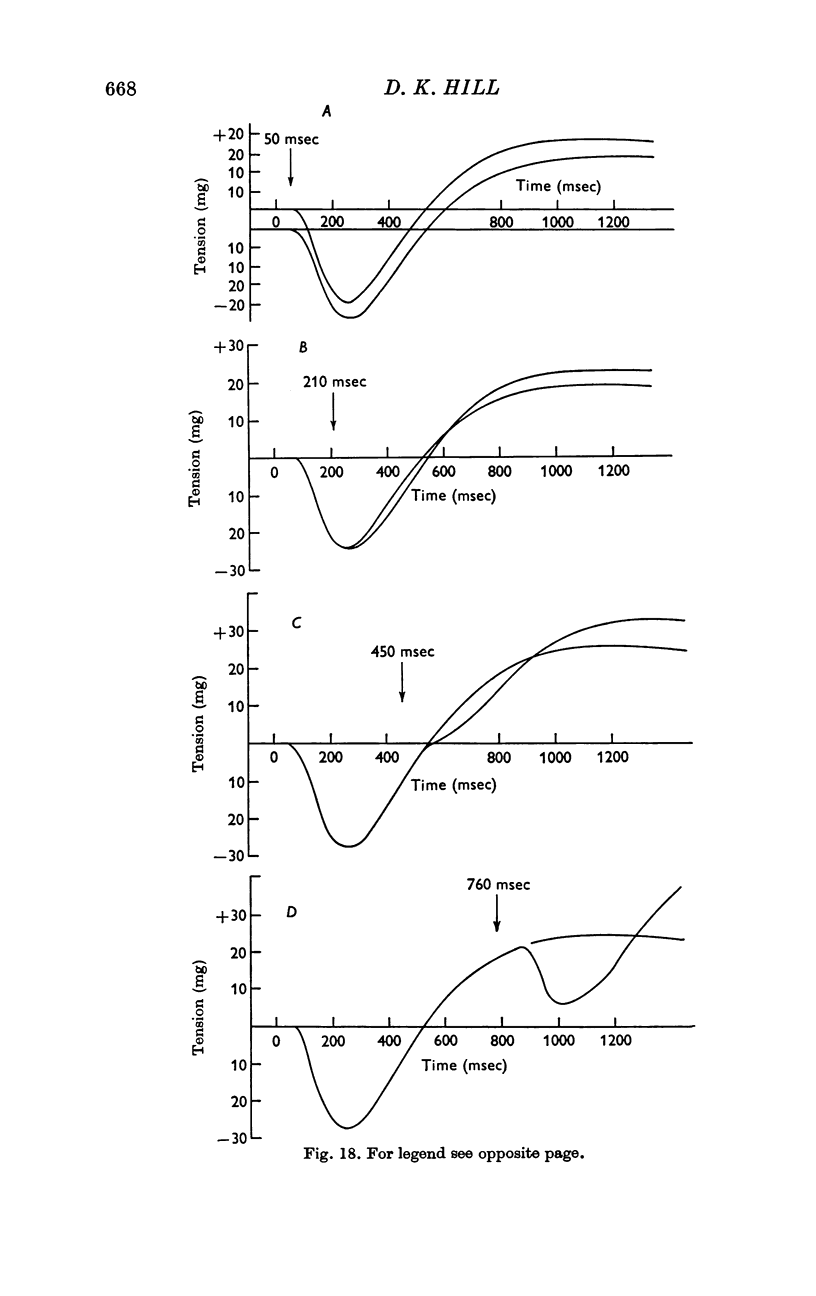
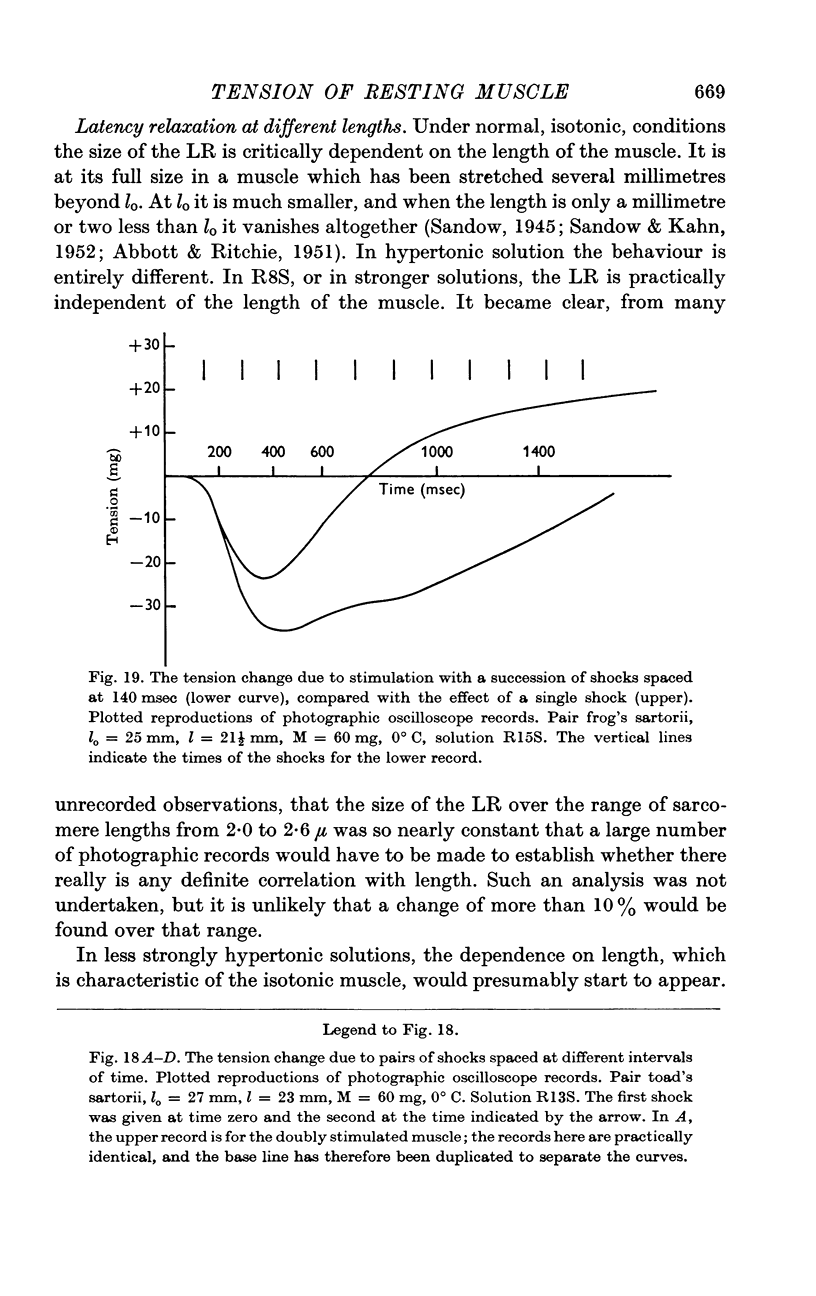
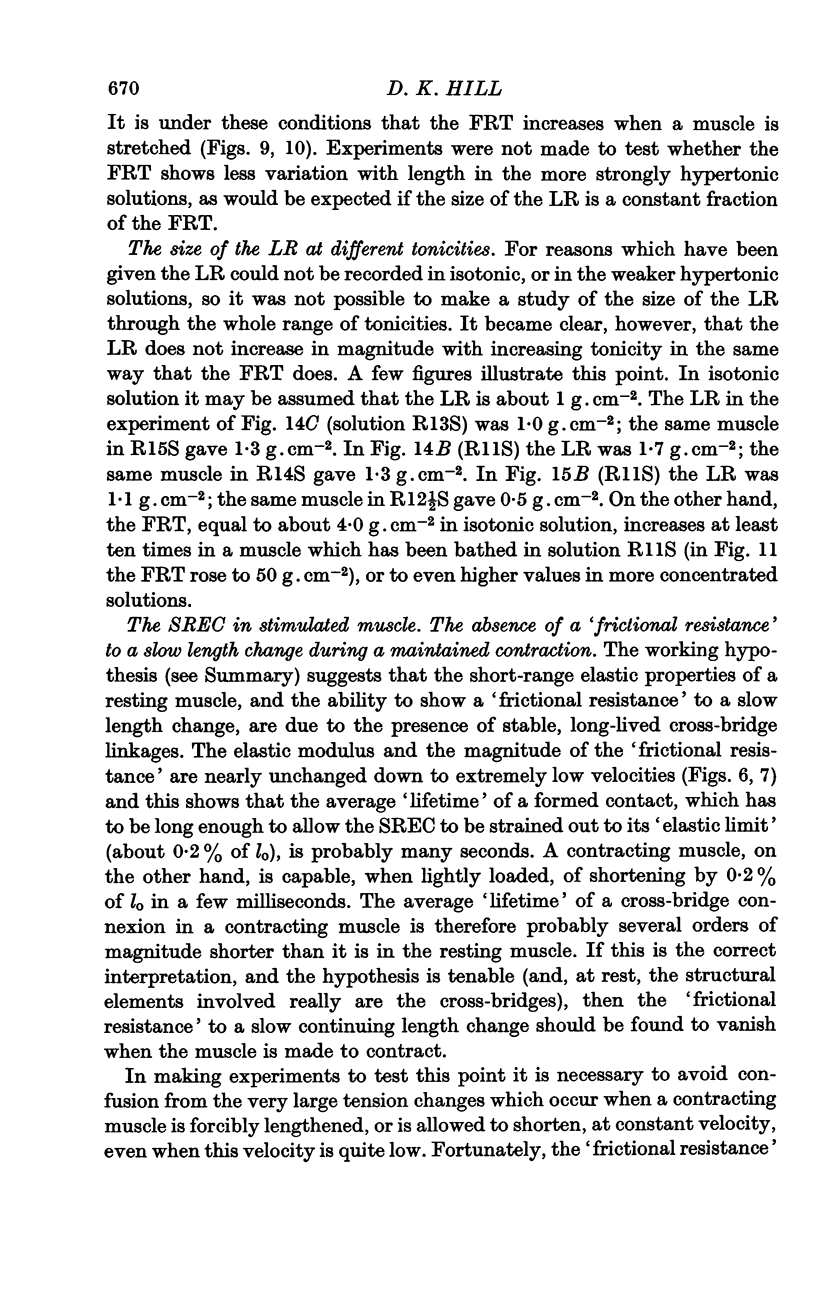
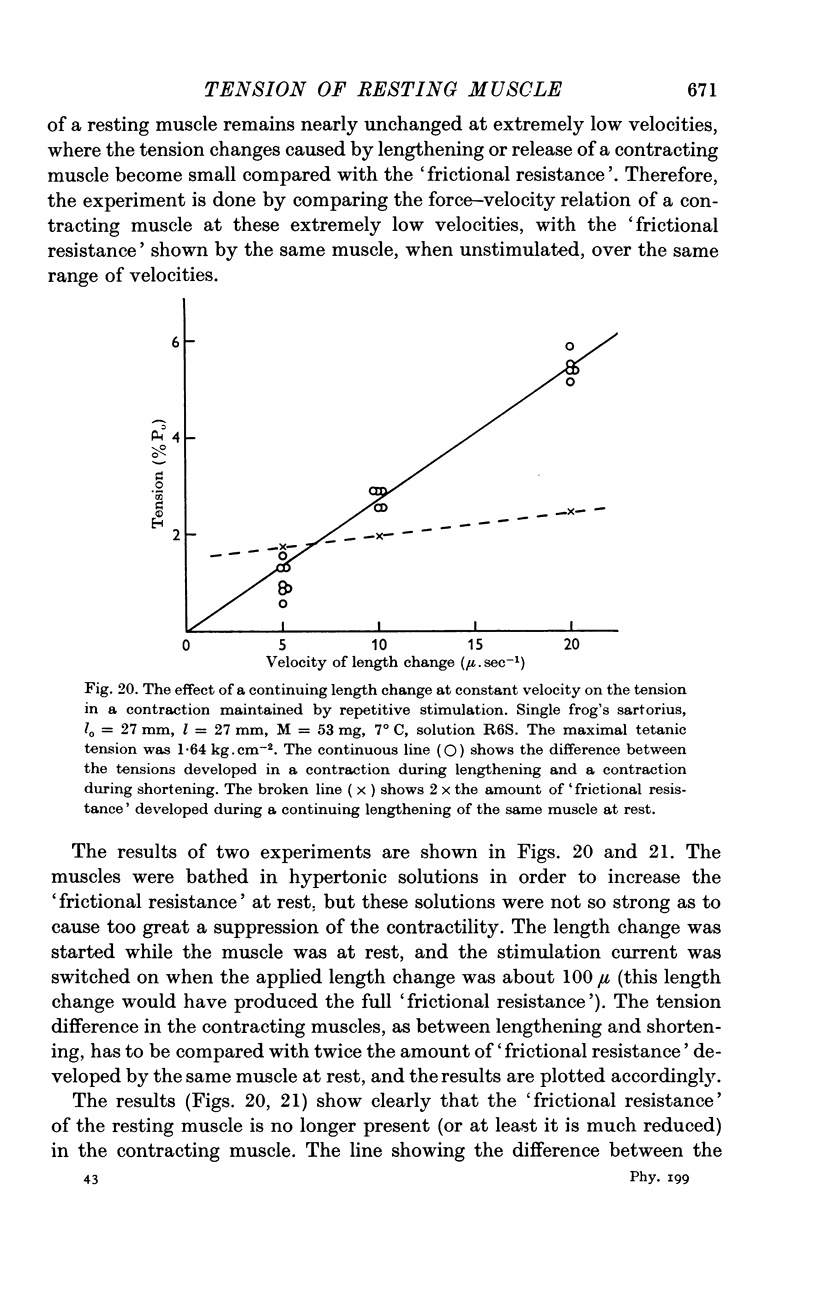
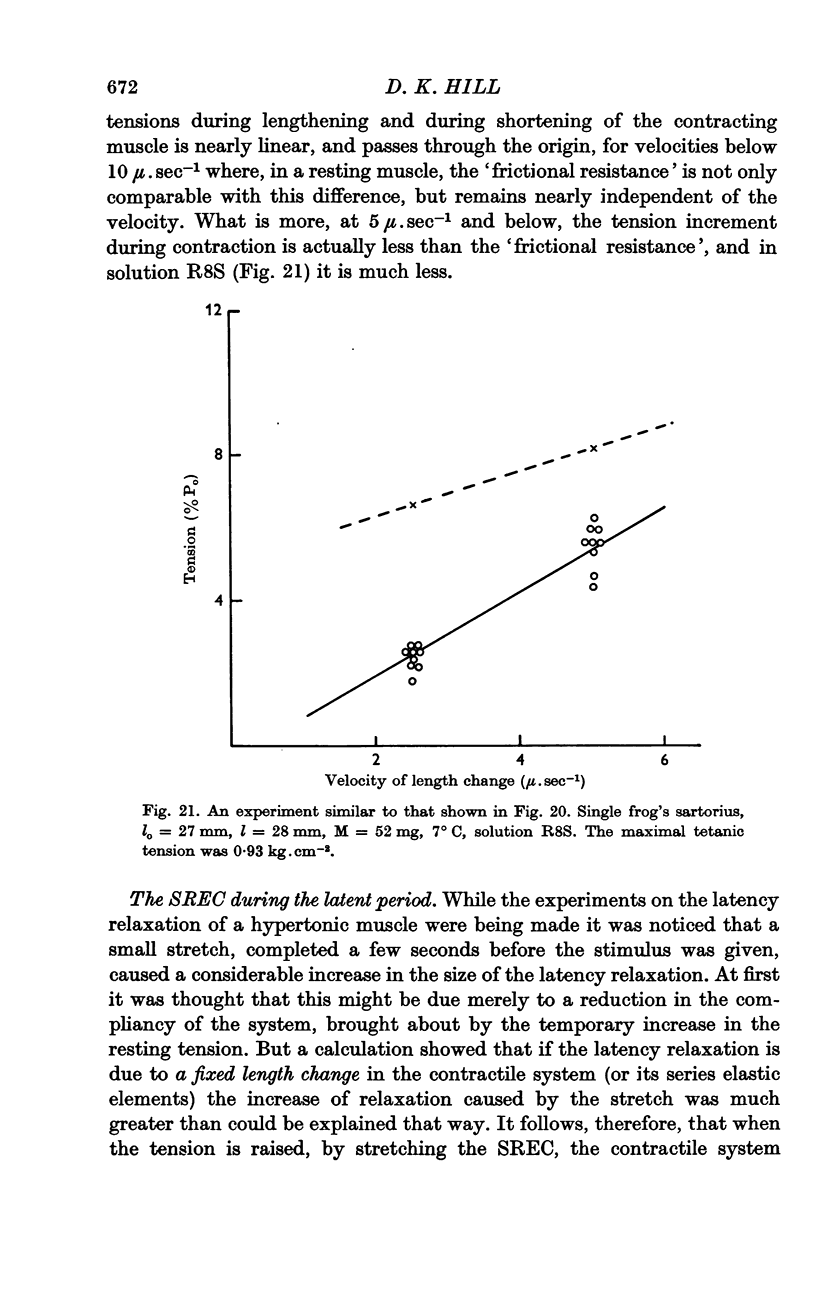
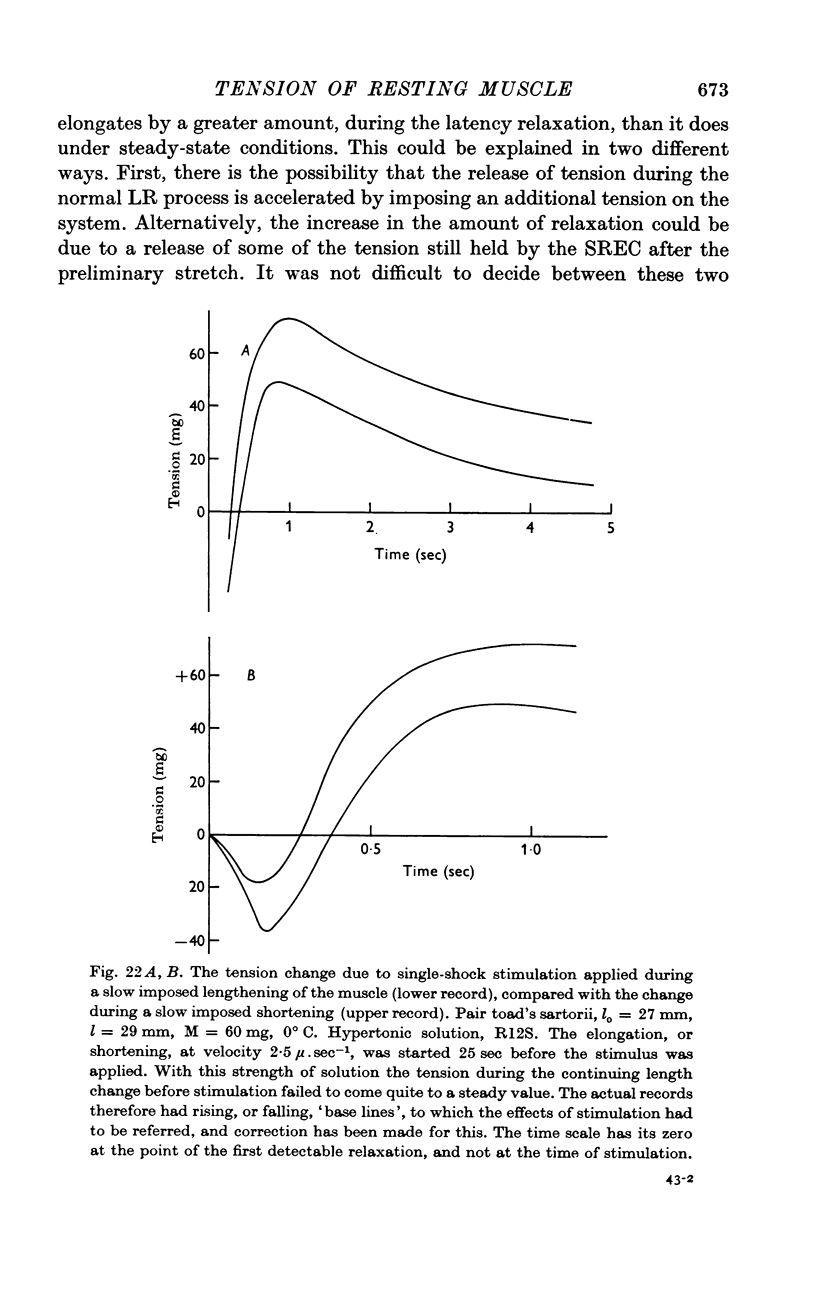
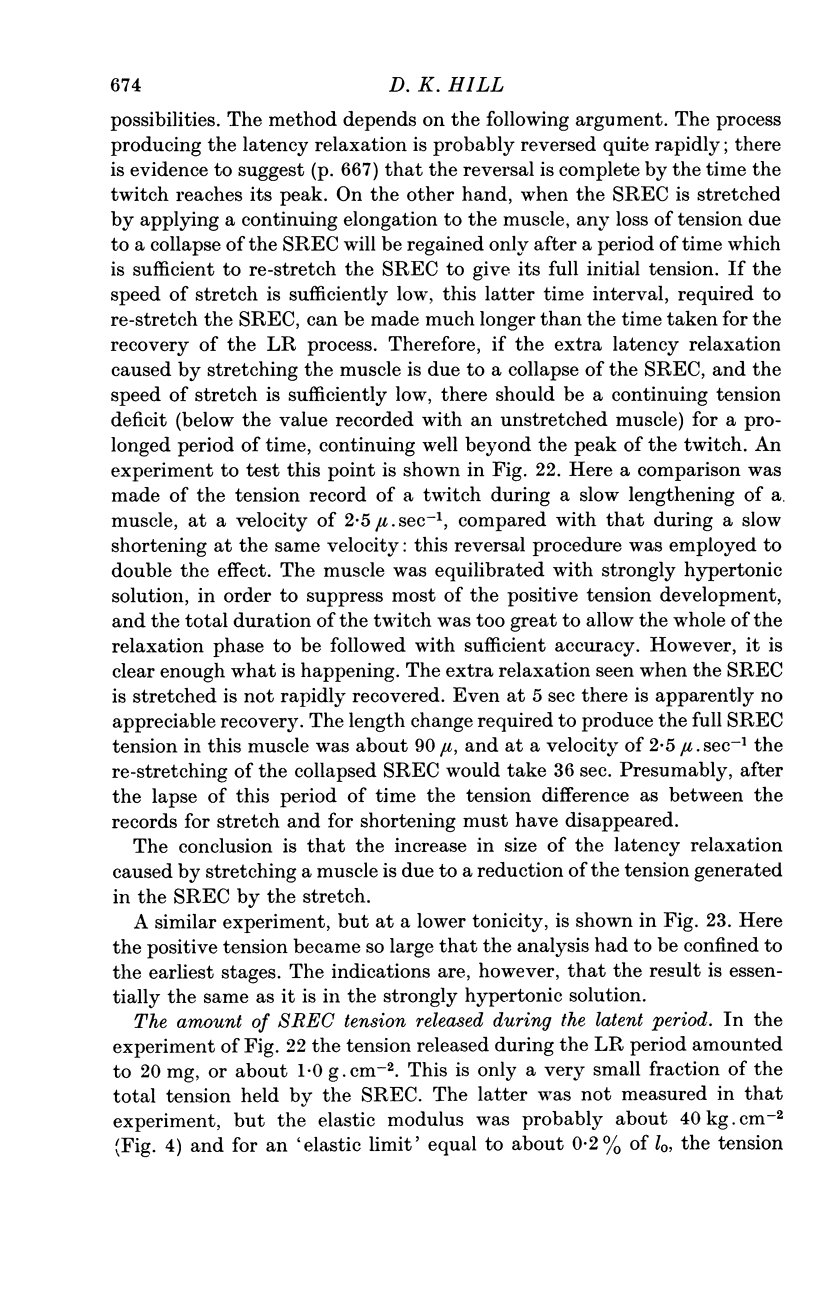
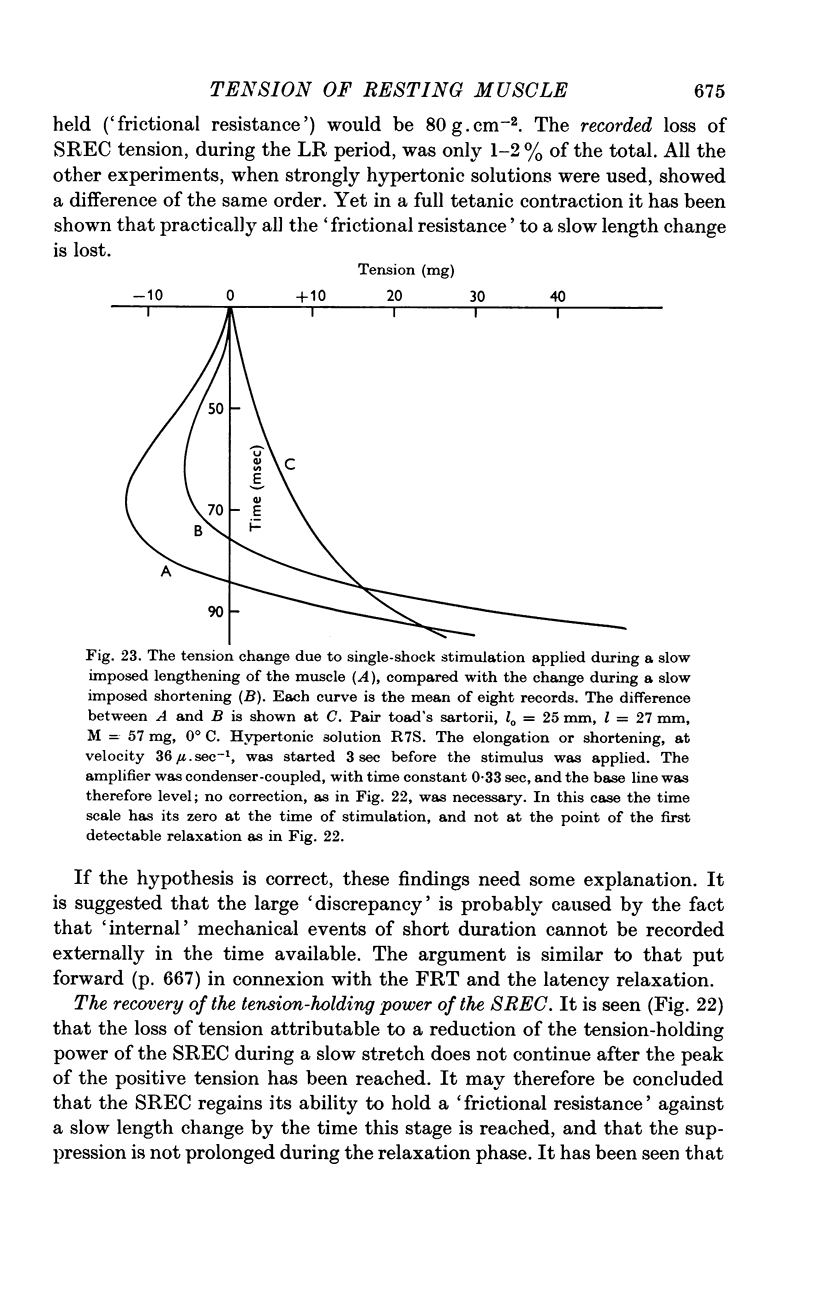
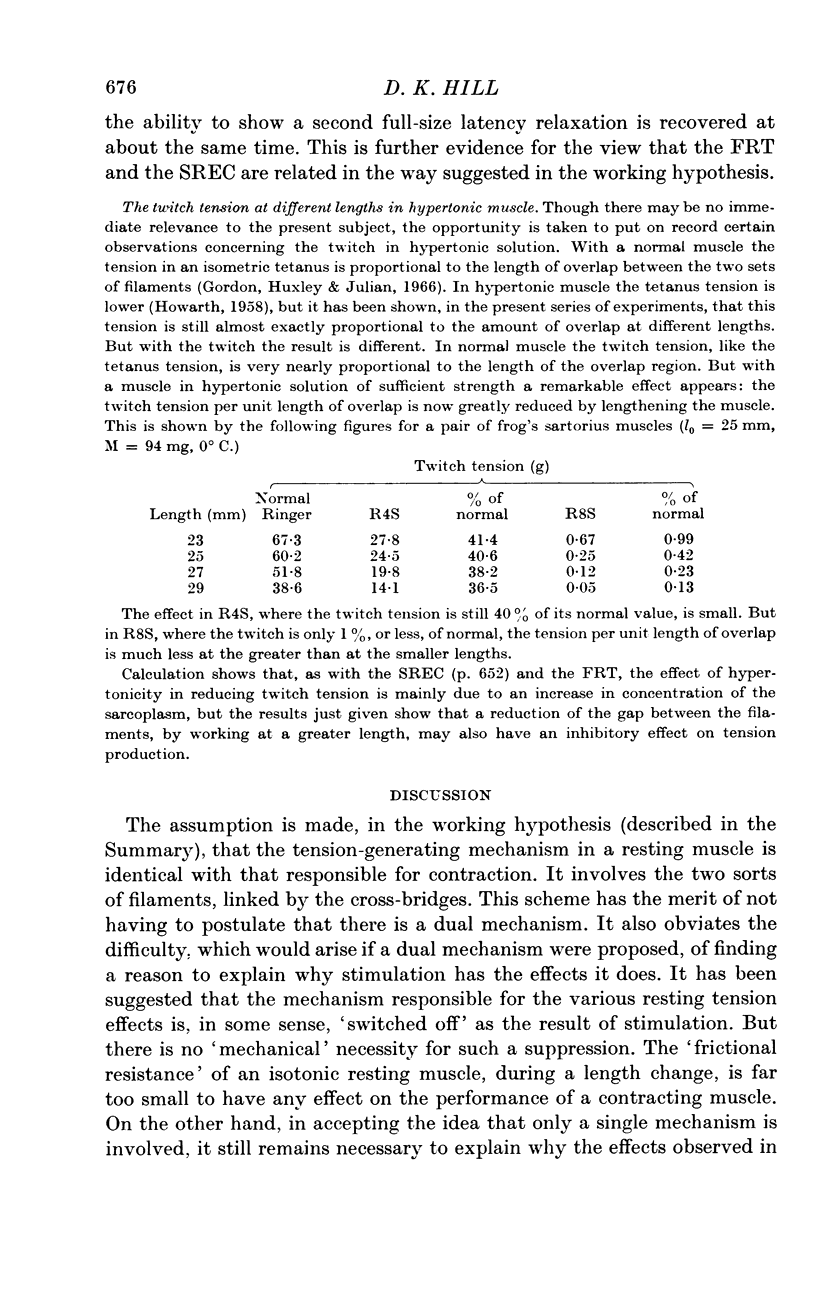
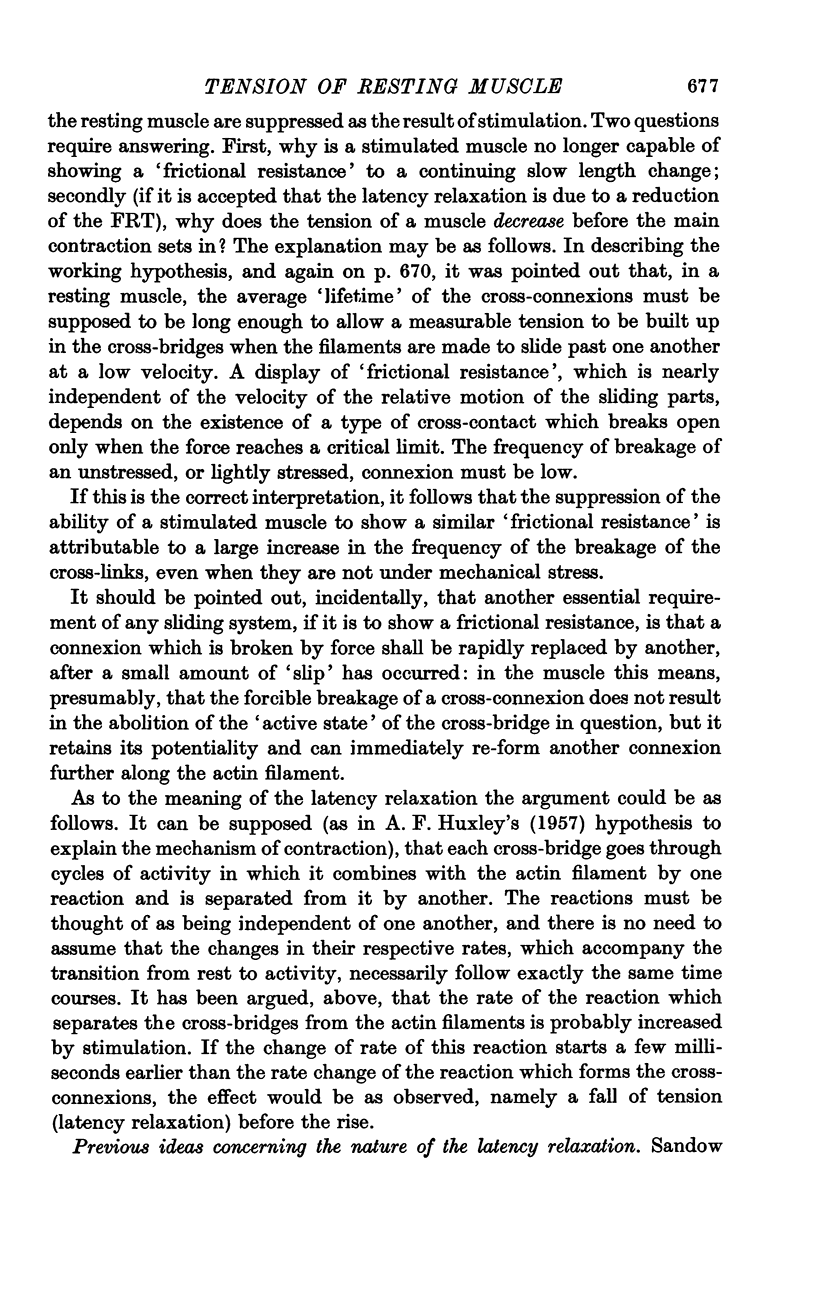
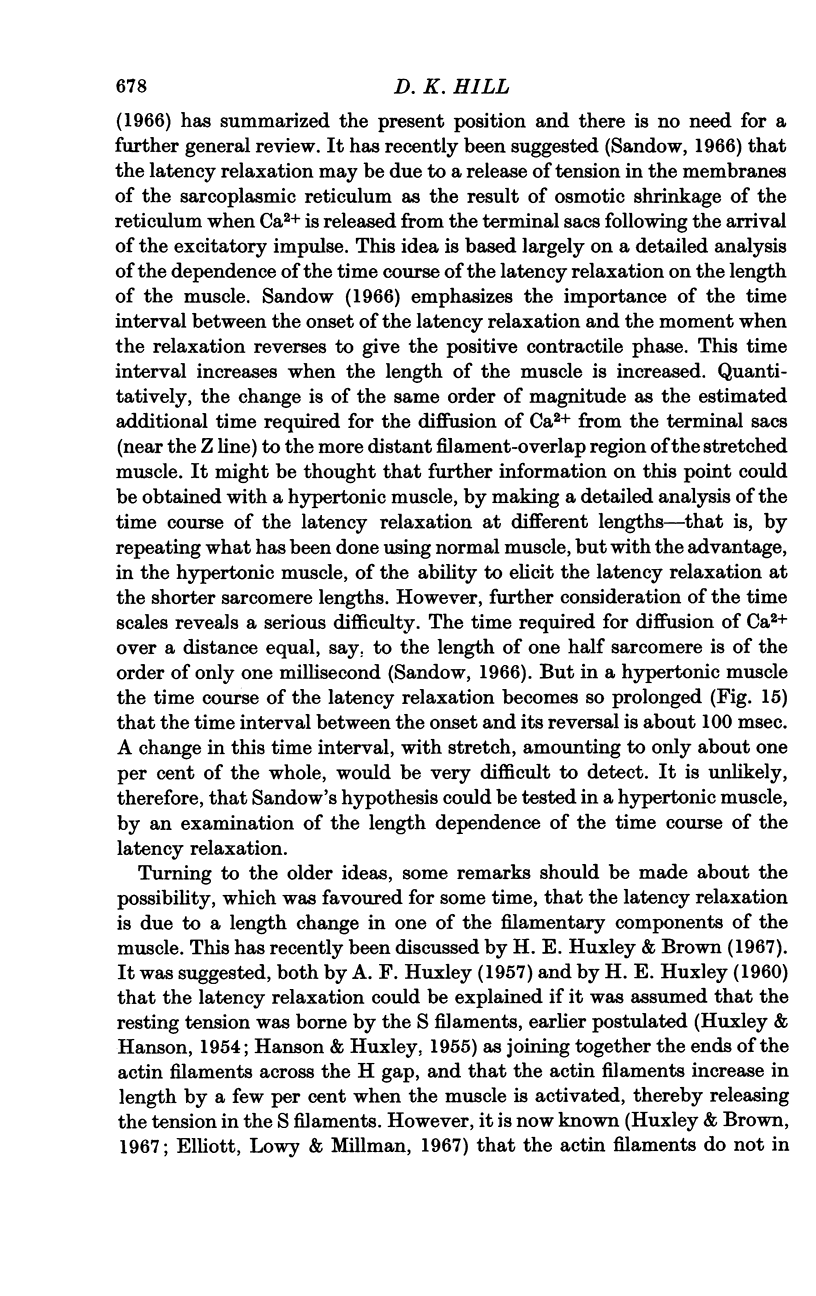
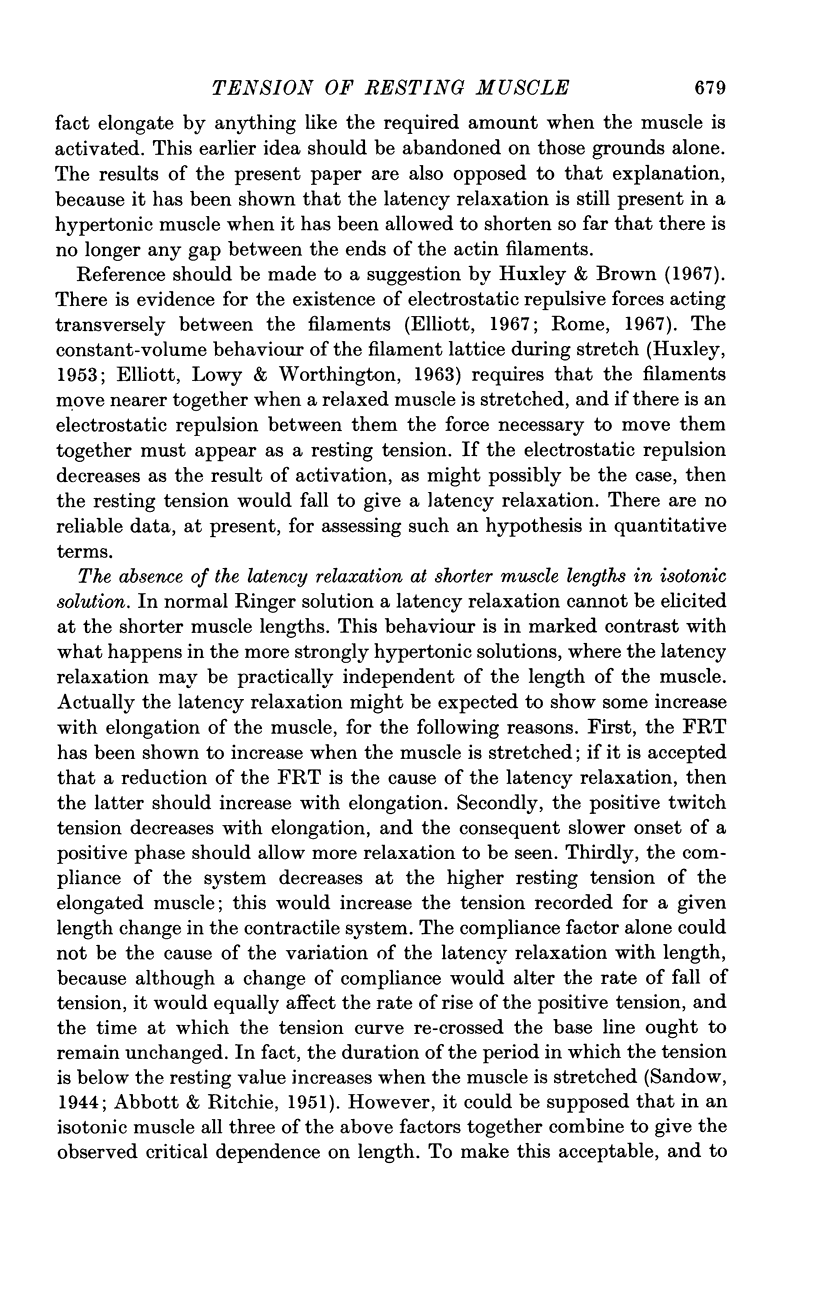
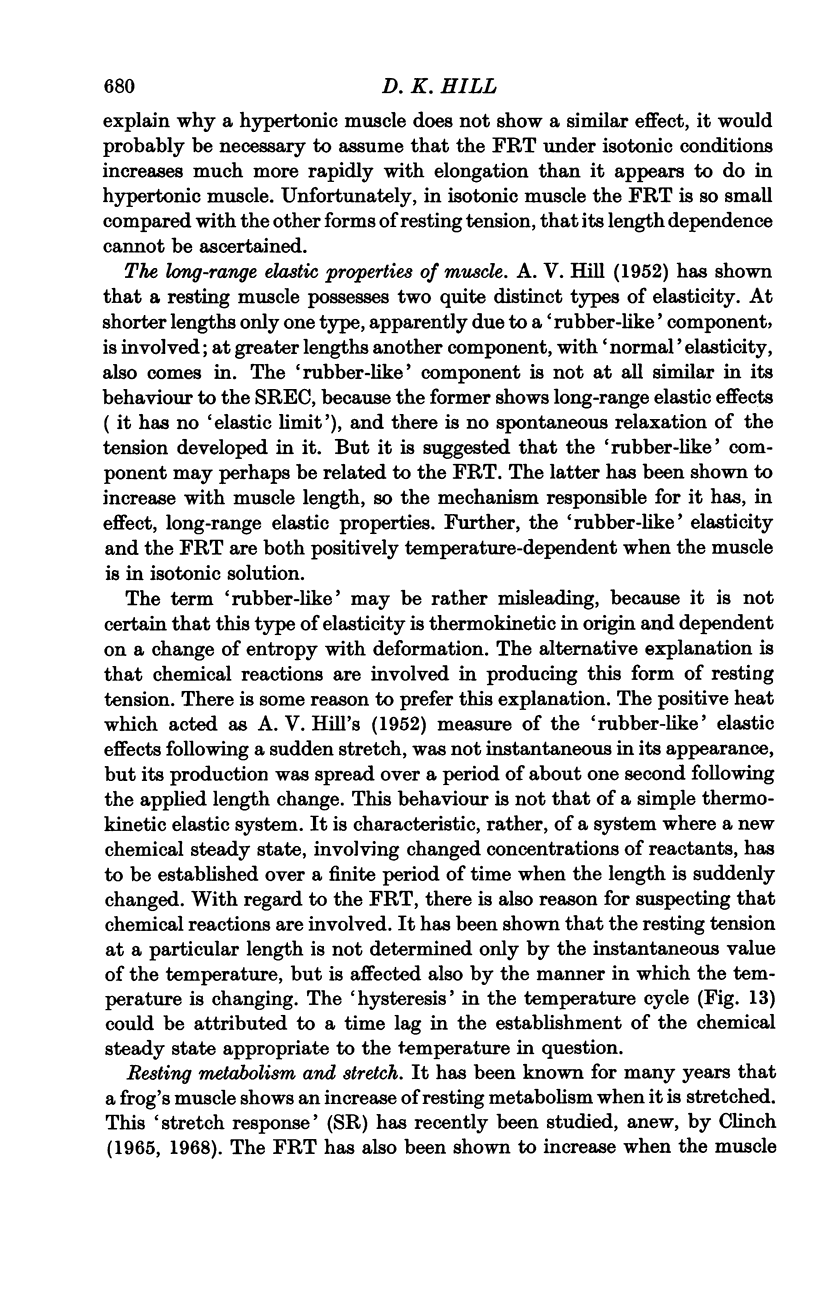
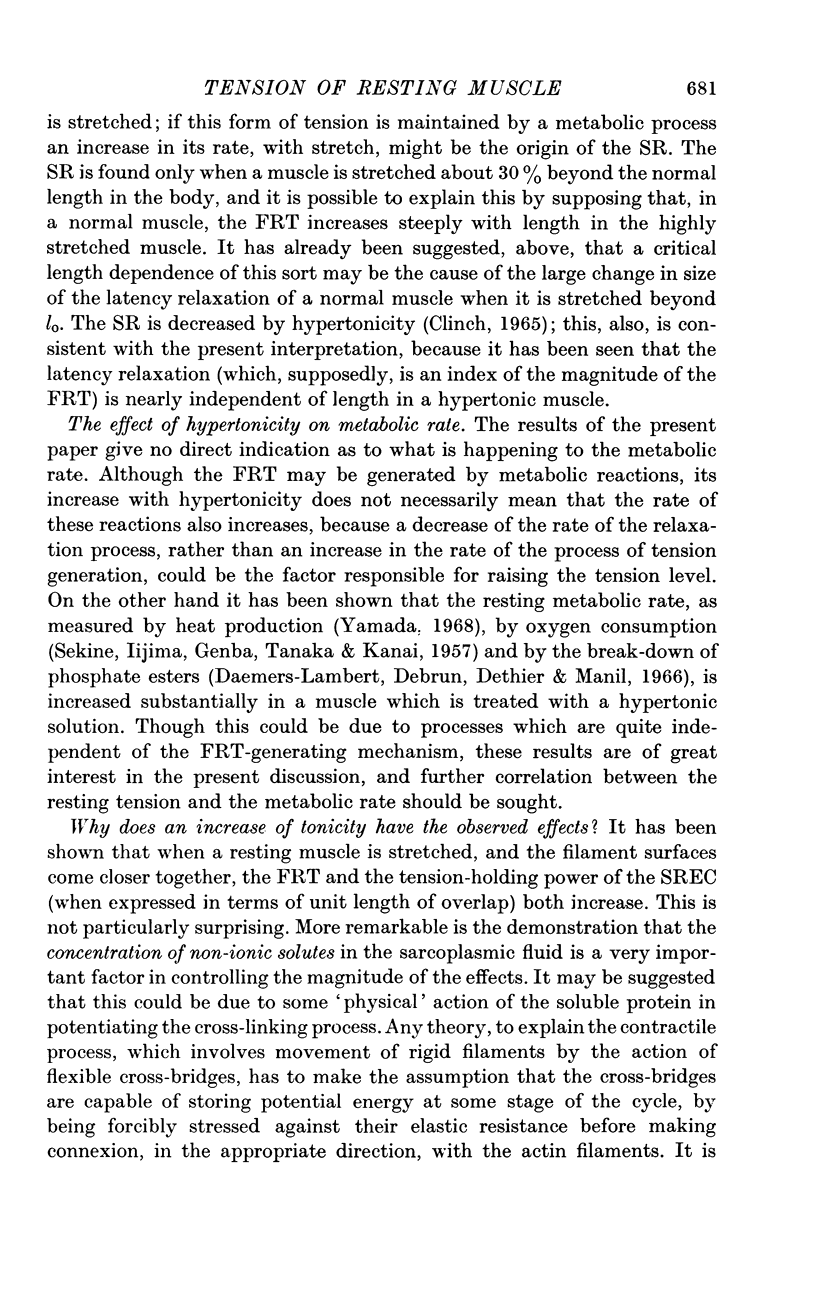
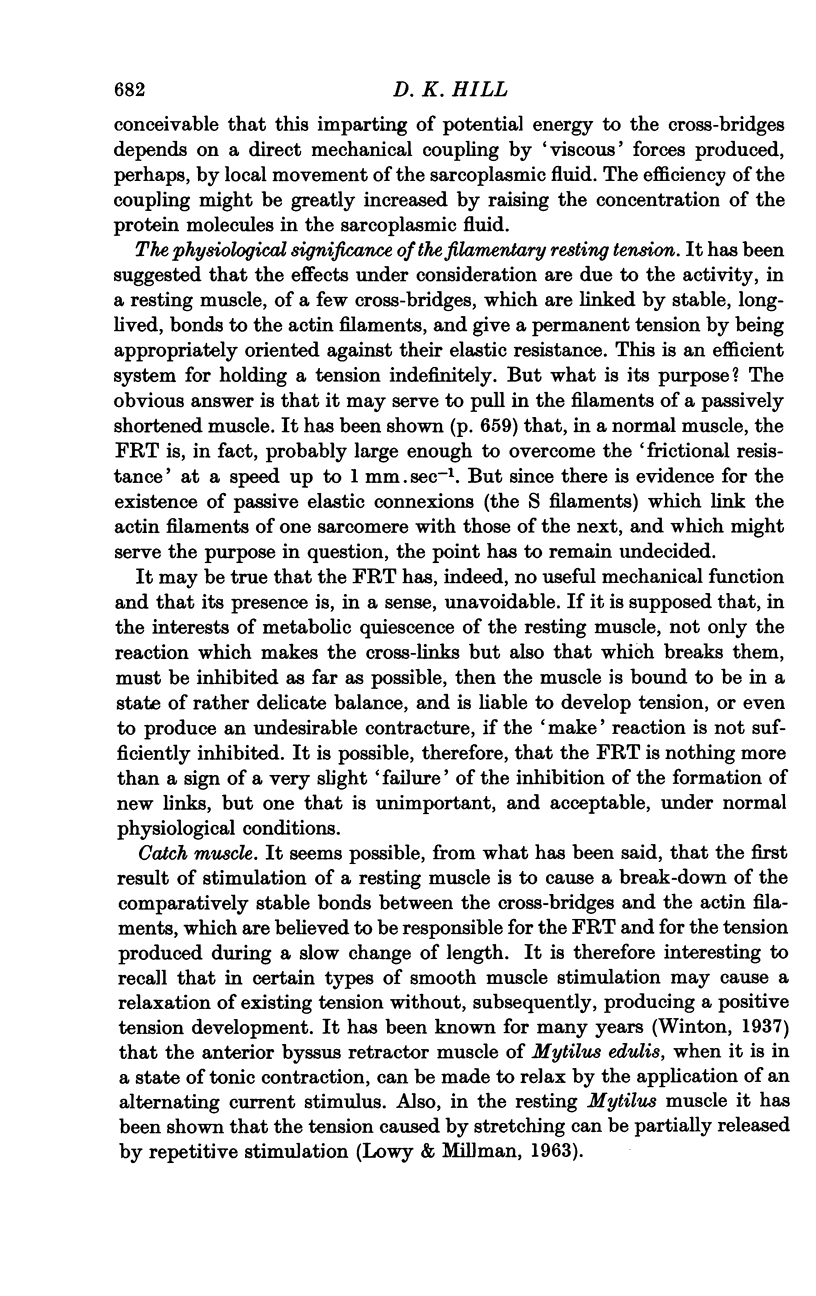
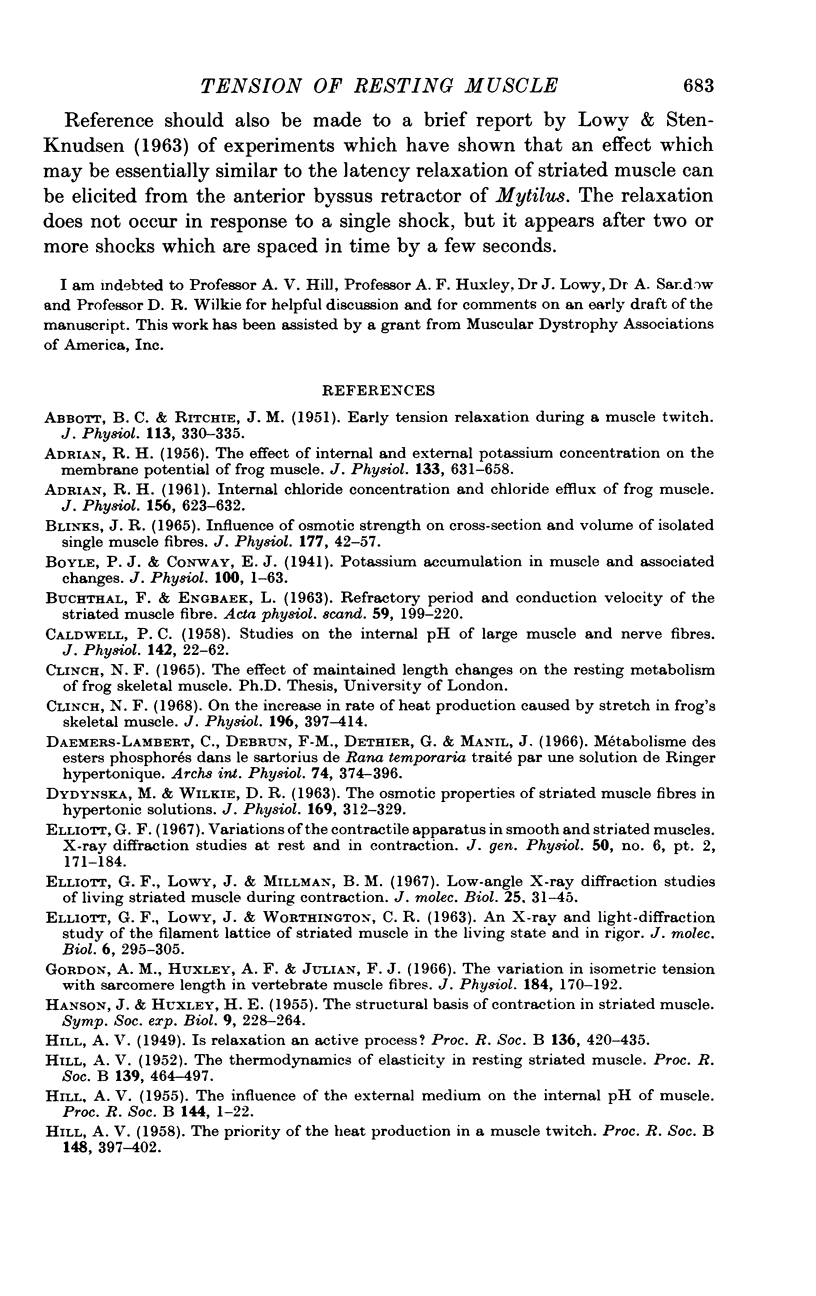
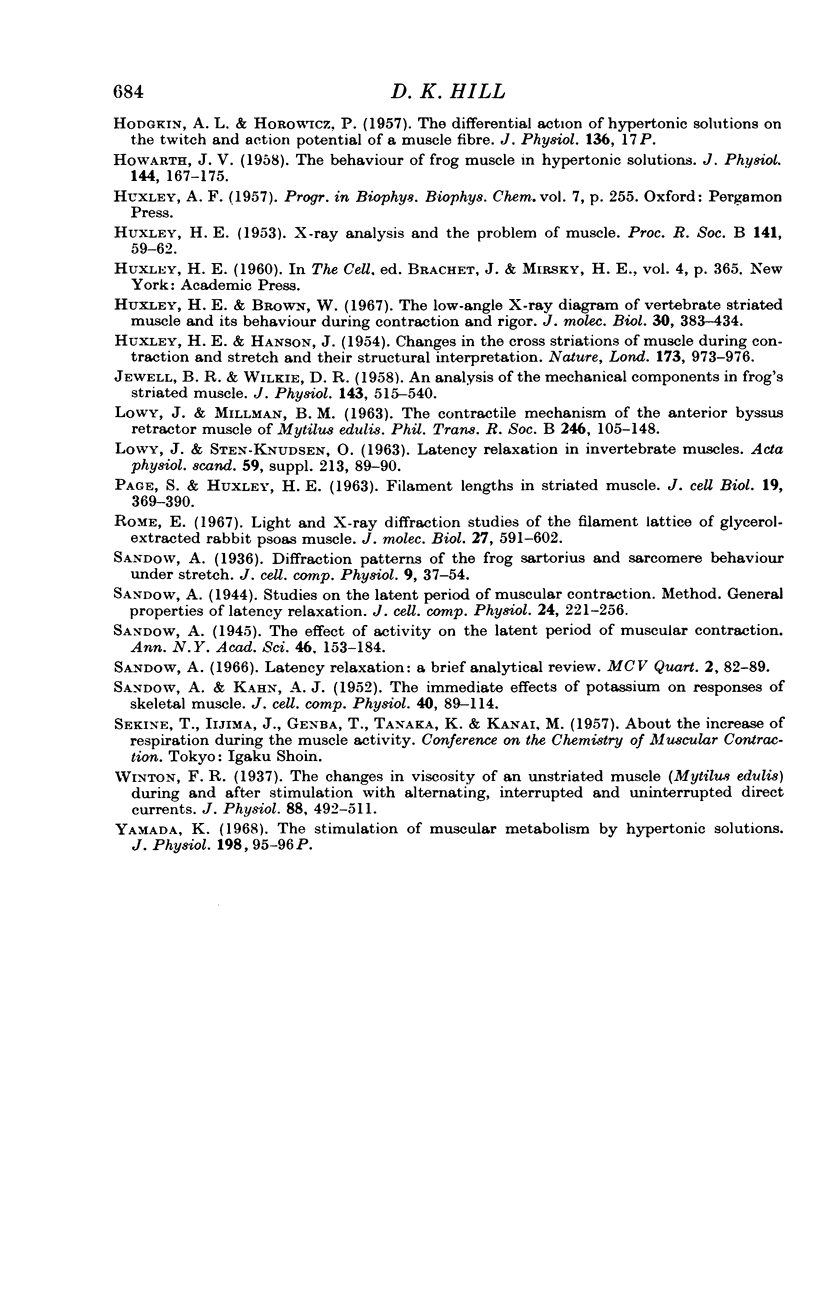
Selected References
These references are in PubMed. This may not be the complete list of references from this article.
- ADRIAN R. H. Internal chloride concentration and chloride efflux of frog muscle. J Physiol. 1961 May;156:623–632. doi: 10.1113/jphysiol.1961.sp006698. [DOI] [PMC free article] [PubMed] [Google Scholar]
- ADRIAN R. H. The effect of internal and external potassium concentration on the membrane potential of frog muscle. J Physiol. 1956 Sep 27;133(3):631–658. doi: 10.1113/jphysiol.1956.sp005615. [DOI] [PMC free article] [PubMed] [Google Scholar]
- Abbott B. C., Ritchie J. M. Early tension relaxation during a muscle twitch. J Physiol. 1951 Apr 26;113(2-3):330–335. doi: 10.1113/jphysiol.1951.sp004576. [DOI] [PMC free article] [PubMed] [Google Scholar]
- BLINKS J. R. INFLUENCE OF OSMOTIC STRENGTH ON CROSS-SECTION AND VOLUME OF ISOLATED SINGLE MUSCLE FIBRES. J Physiol. 1965 Mar;177:42–57. doi: 10.1113/jphysiol.1965.sp007574. [DOI] [PMC free article] [PubMed] [Google Scholar]
- BUCHTHAL F., ENGBAEK L. REFRACTORY PERIOD AND CONDUCTION VELOCITY OF THE STRIATED MUSCLE FIBRE. Acta Physiol Scand. 1963 Nov;59:199–220. doi: 10.1111/j.1748-1716.1963.tb02737.x. [DOI] [PubMed] [Google Scholar]
- Boyle P. J., Conway E. J. Potassium accumulation in muscle and associated changes. J Physiol. 1941 Aug 11;100(1):1–63. doi: 10.1113/jphysiol.1941.sp003922. [DOI] [PMC free article] [PubMed] [Google Scholar]
- CALDWELL P. C. Studies on the internal pH of large muscle and nerve fibres. J Physiol. 1958 Jun 18;142(1):22–62. doi: 10.1113/jphysiol.1958.sp005998. [DOI] [PMC free article] [PubMed] [Google Scholar]
- Clinch N. F. On the increase in rate of heat production caused by stretch in frog's skeletal muscle. J Physiol. 1968 May;196(2):397–414. doi: 10.1113/jphysiol.1968.sp008514. [DOI] [PMC free article] [PubMed] [Google Scholar]
- DYDYNSKA M., WILKIE D. R. THE OSMOTIC PROPERTIES OF STRIATED MUSCLE FIBERS IN HYPERTONIC SOLUTIONS. J Physiol. 1963 Nov;169:312–329. doi: 10.1113/jphysiol.1963.sp007258. [DOI] [PMC free article] [PubMed] [Google Scholar]
- Daemers-Lambert C., Debrun F. M., Dethier G., Manil J. Métabolisme des esters phosphorés dans le sartorius de Rana temporaria traité par une solution de Ringer hypertonique. Arch Int Physiol Biochim. 1966 Jun;74(3):374–396. doi: 10.3109/13813456609059918. [DOI] [PubMed] [Google Scholar]
- Elliott G. F., Lowy J., Millman B. M. Low-angle x-ray diffraction studies of living striated muscle during contraction. J Mol Biol. 1967 Apr 14;25(1):31–45. doi: 10.1016/0022-2836(67)90277-x. [DOI] [PubMed] [Google Scholar]
- Gordon A. M., Huxley A. F., Julian F. J. The variation in isometric tension with sarcomere length in vertebrate muscle fibres. J Physiol. 1966 May;184(1):170–192. doi: 10.1113/jphysiol.1966.sp007909. [DOI] [PMC free article] [PubMed] [Google Scholar]
- HILL A. V. The priority of the heat production in a muscle twitch. Proc R Soc Lond B Biol Sci. 1958 Mar 18;148(932):397–402. doi: 10.1098/rspb.1958.0033. [DOI] [PubMed] [Google Scholar]
- HILL A. V. The thermodynamics of elasticity in resting striated muscle. Proc R Soc Lond B Biol Sci. 1952 Jul 10;139(897):464–passim. doi: 10.1098/rspb.1952.0024. [DOI] [PubMed] [Google Scholar]
- HOWARTH J. V. The behaviour of frog muscle in hypertonic solutions. J Physiol. 1958 Nov 10;144(1):167–175. doi: 10.1113/jphysiol.1958.sp006093. [DOI] [PMC free article] [PubMed] [Google Scholar]
- HUXLEY H. E. X-ray analysis and the problem of muscle. Proc R Soc Lond B Biol Sci. 1953 Mar 11;141(902):59–62. doi: 10.1098/rspb.1953.0017. [DOI] [PubMed] [Google Scholar]
- HUXLEY H., HANSON J. Changes in the cross-striations of muscle during contraction and stretch and their structural interpretation. Nature. 1954 May 22;173(4412):973–976. doi: 10.1038/173973a0. [DOI] [PubMed] [Google Scholar]
- Huxley H. E., Brown W. The low-angle x-ray diagram of vertebrate striated muscle and its behaviour during contraction and rigor. J Mol Biol. 1967 Dec 14;30(2):383–434. doi: 10.1016/s0022-2836(67)80046-9. [DOI] [PubMed] [Google Scholar]
- JEWELL B. R., WILKIE D. R. An analysis of the mechanical components in frog's striated muscle. J Physiol. 1958 Oct 31;143(3):515–540. doi: 10.1113/jphysiol.1958.sp006075. [DOI] [PMC free article] [PubMed] [Google Scholar]
- PAGE S. G., HUXLEY H. E. FILAMENT LENGTHS IN STRIATED MUSCLE. J Cell Biol. 1963 Nov;19:369–390. doi: 10.1083/jcb.19.2.369. [DOI] [PMC free article] [PubMed] [Google Scholar]
- Rome E. Light and X-ray diffraction studies of the filament lattice of glycerol-extracted rabbit psoas muscle. J Mol Biol. 1967 Aug 14;27(3):591–602. doi: 10.1016/0022-2836(67)90061-7. [DOI] [PubMed] [Google Scholar]
- SANDOW A., KAHN A. J. The immediate effects of potassium on responses of skeletal muscle. J Cell Physiol. 1952 Aug;40(1):89–114. doi: 10.1002/jcp.1030400107. [DOI] [PubMed] [Google Scholar]
- Winton F. R. The changes in viscosity of an unstriated muscle (Mytilus edulis) during and after stimulation with alternating, interrupted and uninterrupted direct currents. J Physiol. 1937 Jan 18;88(4):492–511. doi: 10.1113/jphysiol.1937.sp003455. [DOI] [PMC free article] [PubMed] [Google Scholar]