Abstract
There is an increasing amount of experimental data on transport across biological membranes which cannot be readily accommodated by classical mobile carrier models. We propose models for membrane transport based upon current concepts in molecular enzymology, in which the membrane component involved in transport is an oligomeric protein which undergoes substrate-induced conformational changes. A number of paradoxical observations on glucose transport in the human erythrocyte are explained if the protein involved is a tetramer possessing two classes of binding sites with different affinities for glucose. We develop in detail a particular model of this type, the internal transfer model, in which transport occurs by transfer of substrate from one subunit to another of the protein. The fit of the predictions of the internal transfer model with most of the experimental data is very good. Those data which cannot be fitted by the model cannot be accounted for by any presently available model. We extend our model qualitatively to include the sodium-activated cotransport systems for sugars and amino acids.
Full text
PDF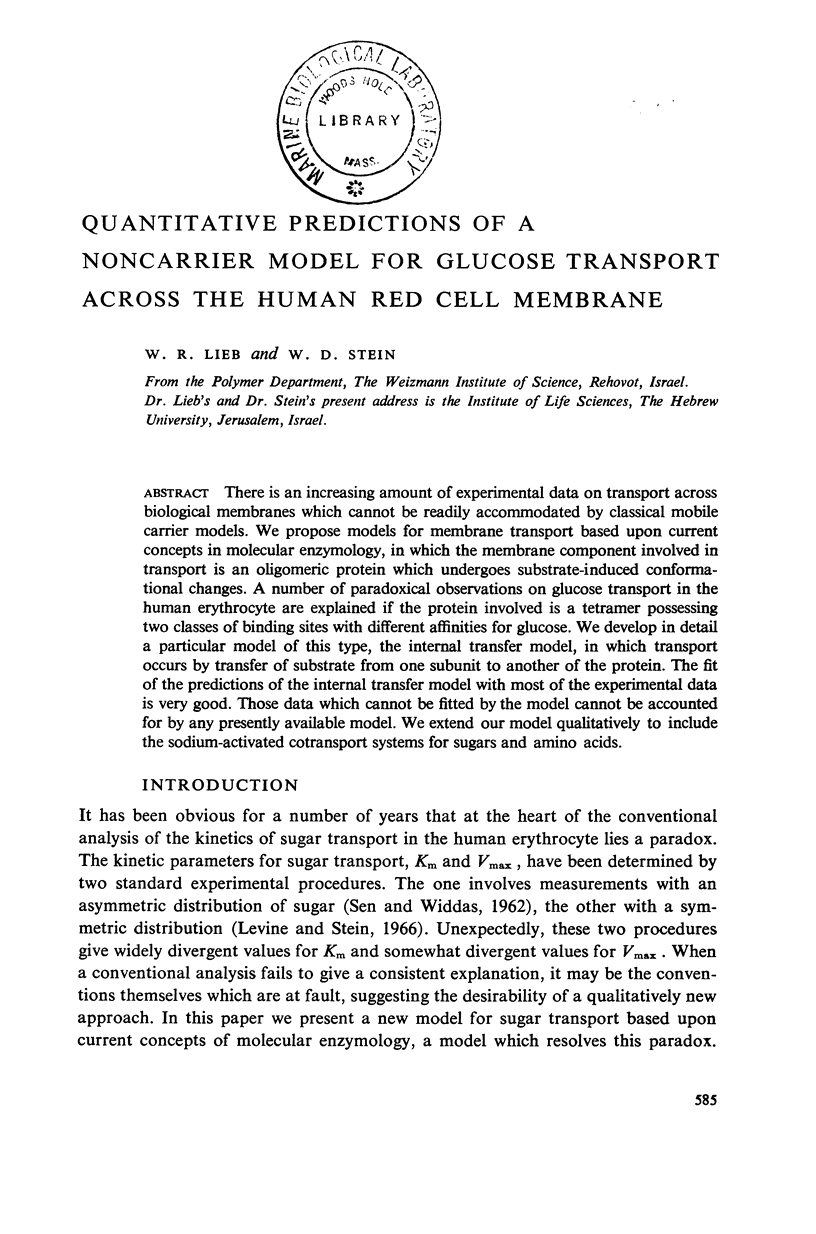
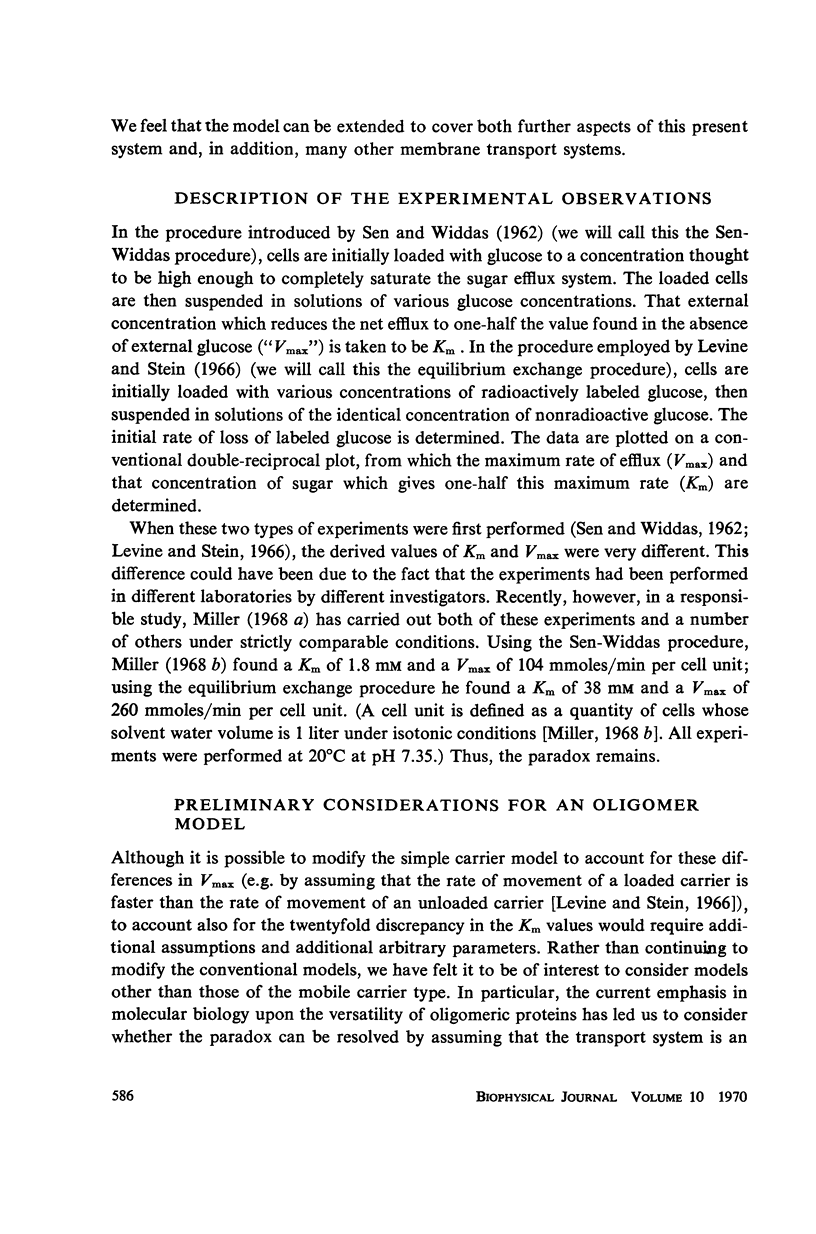
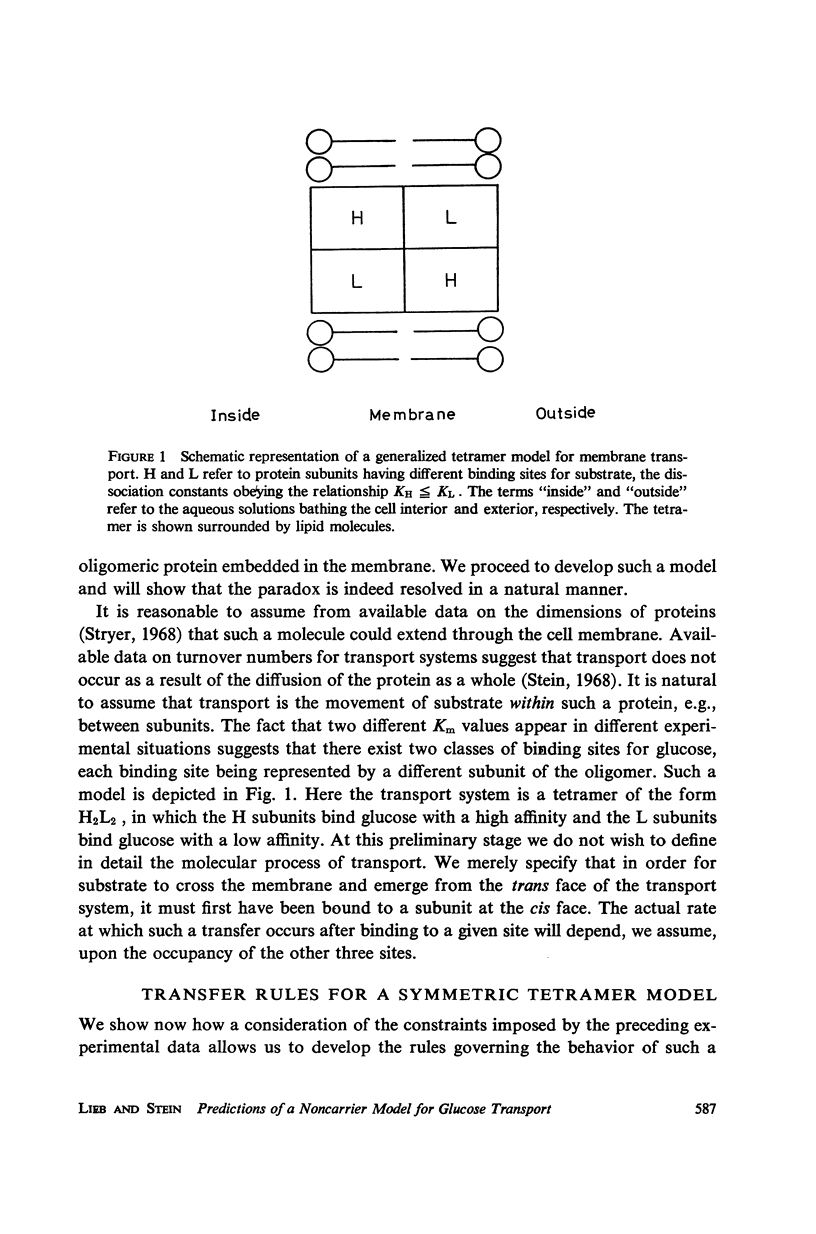
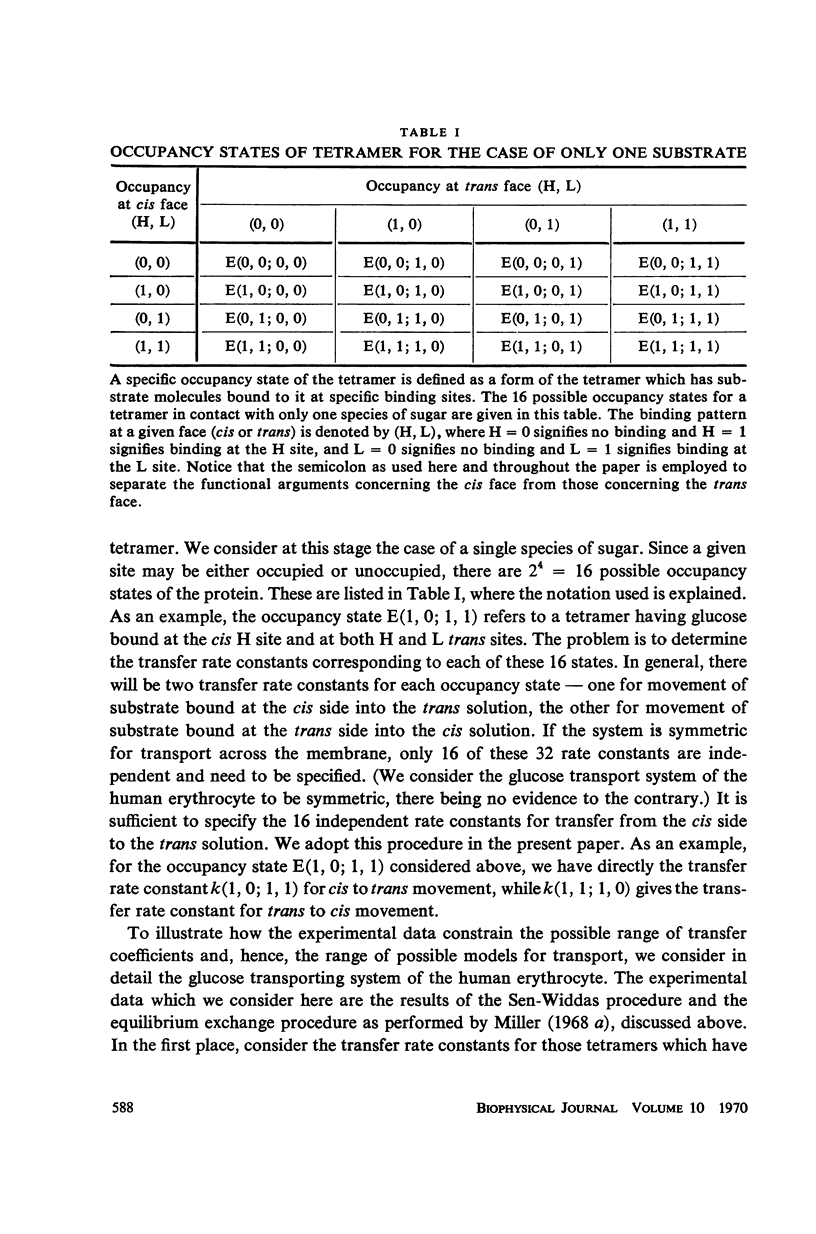
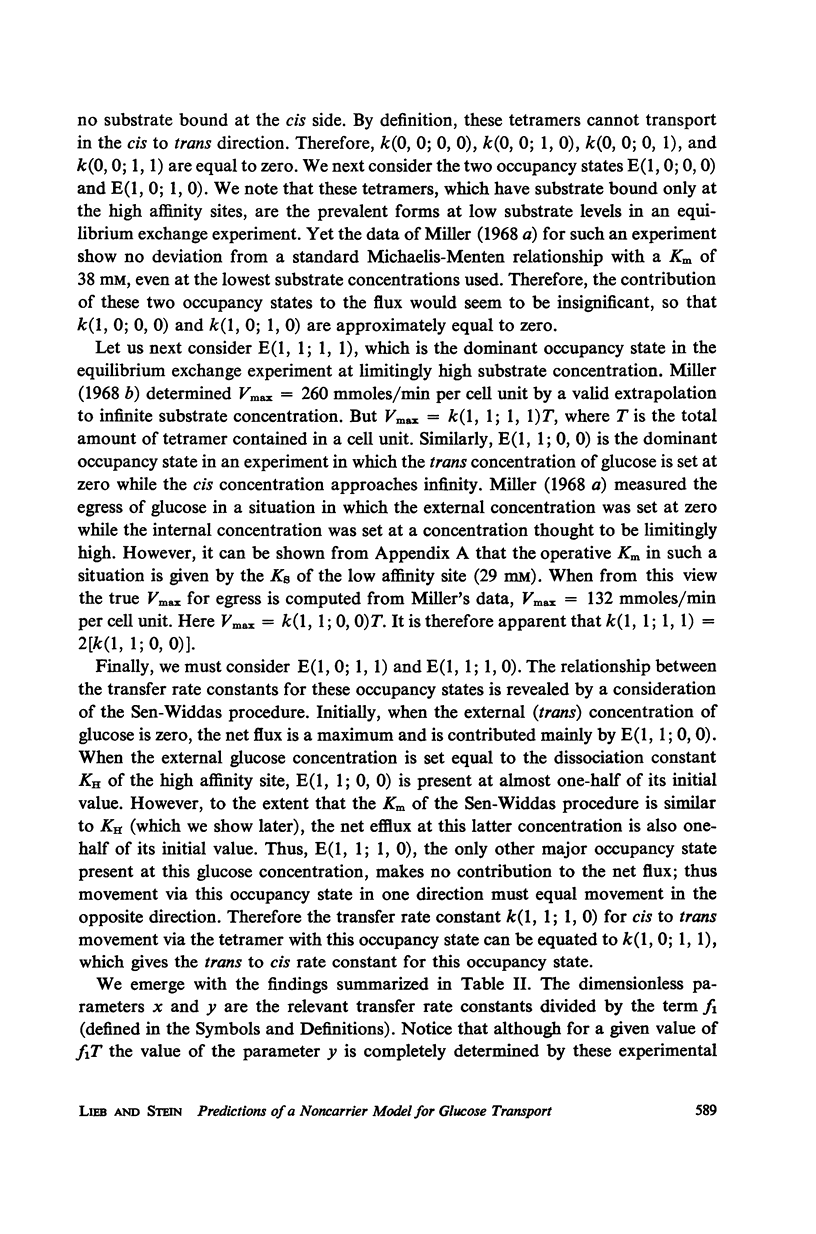
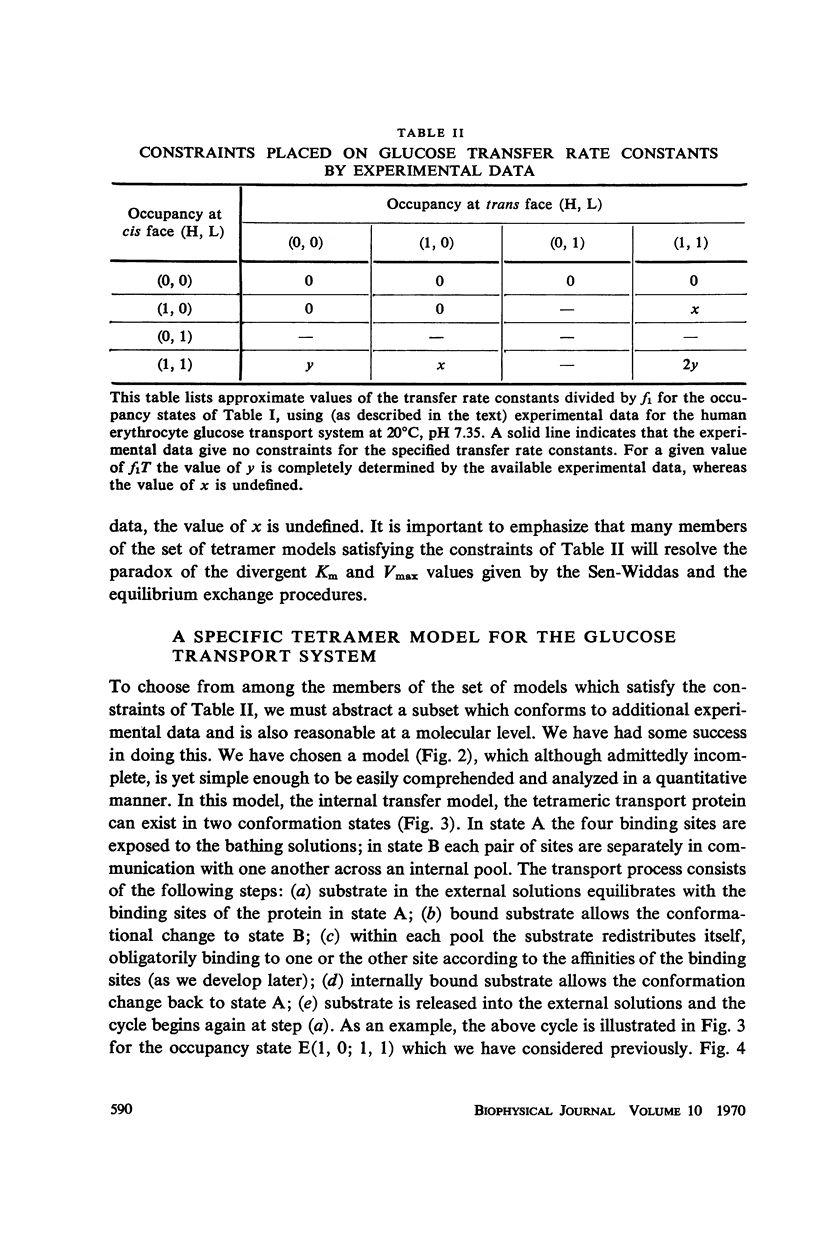
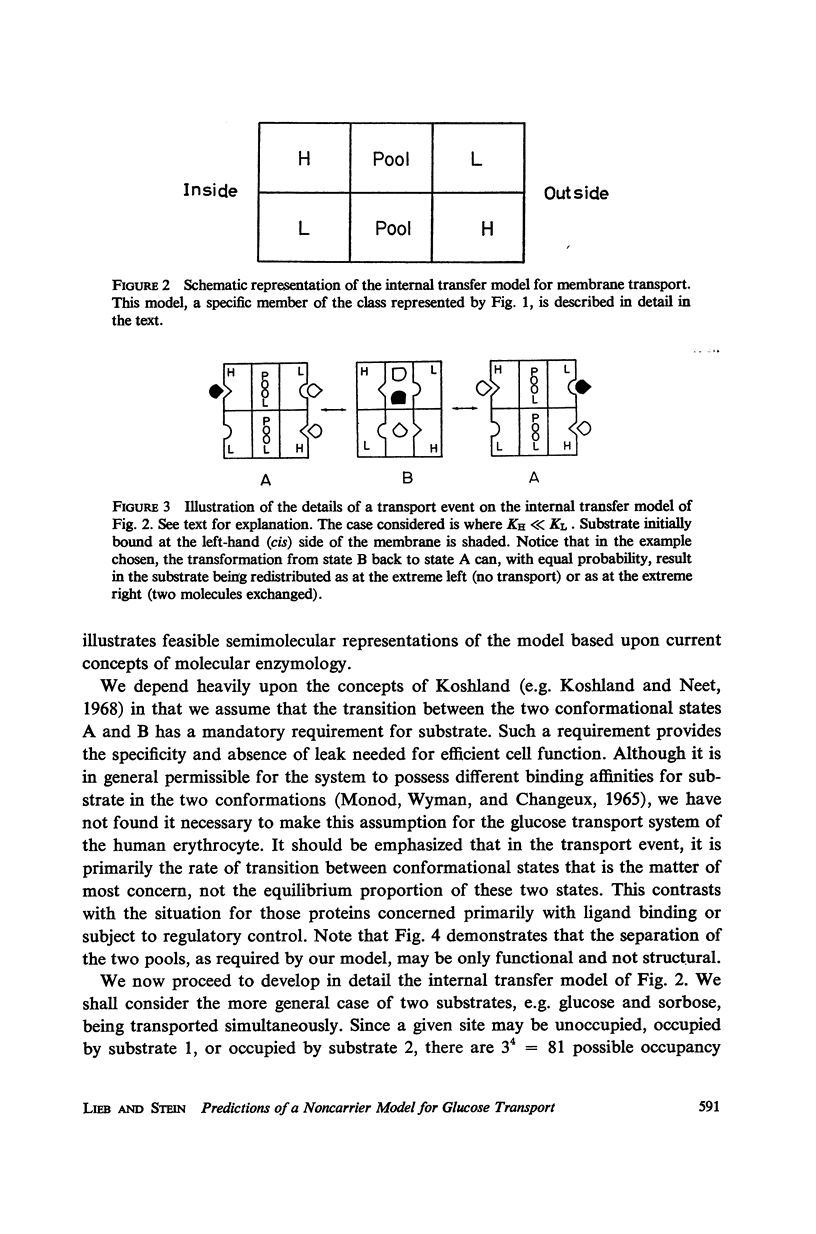
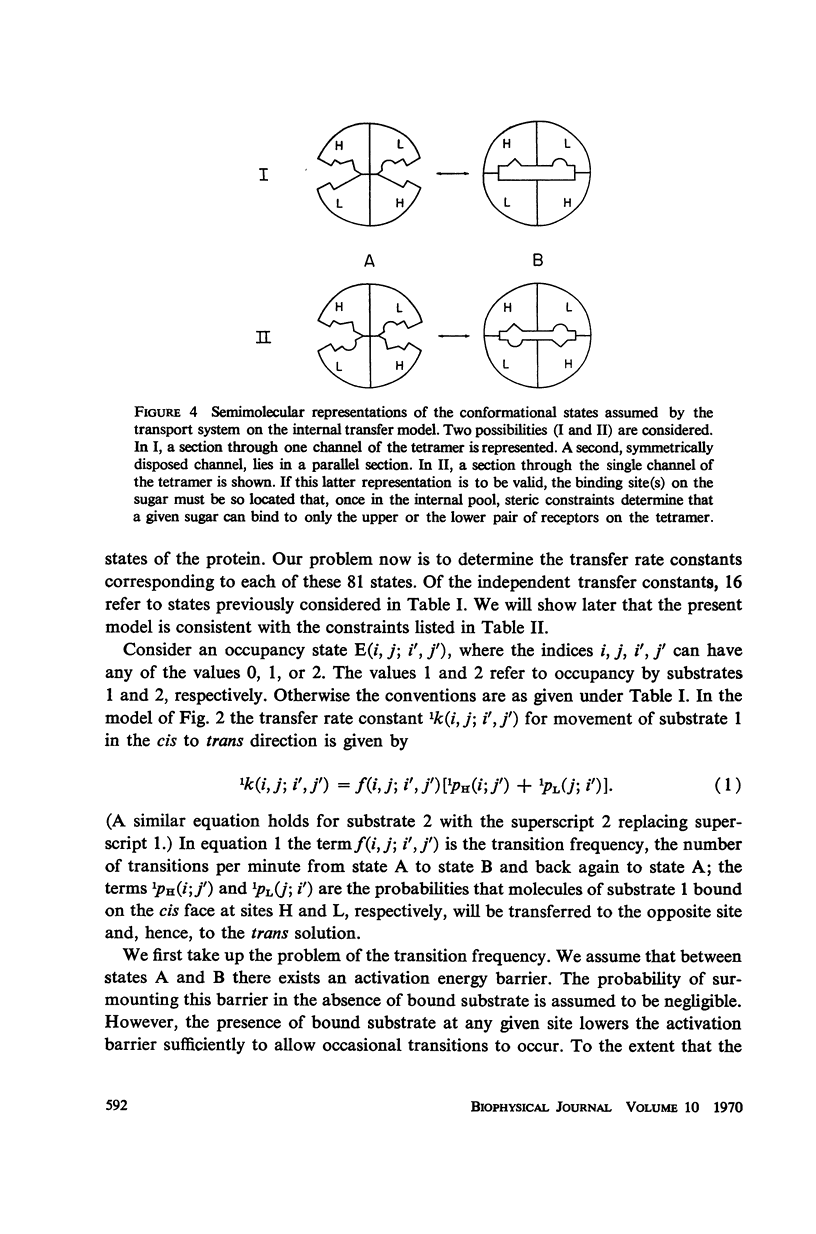
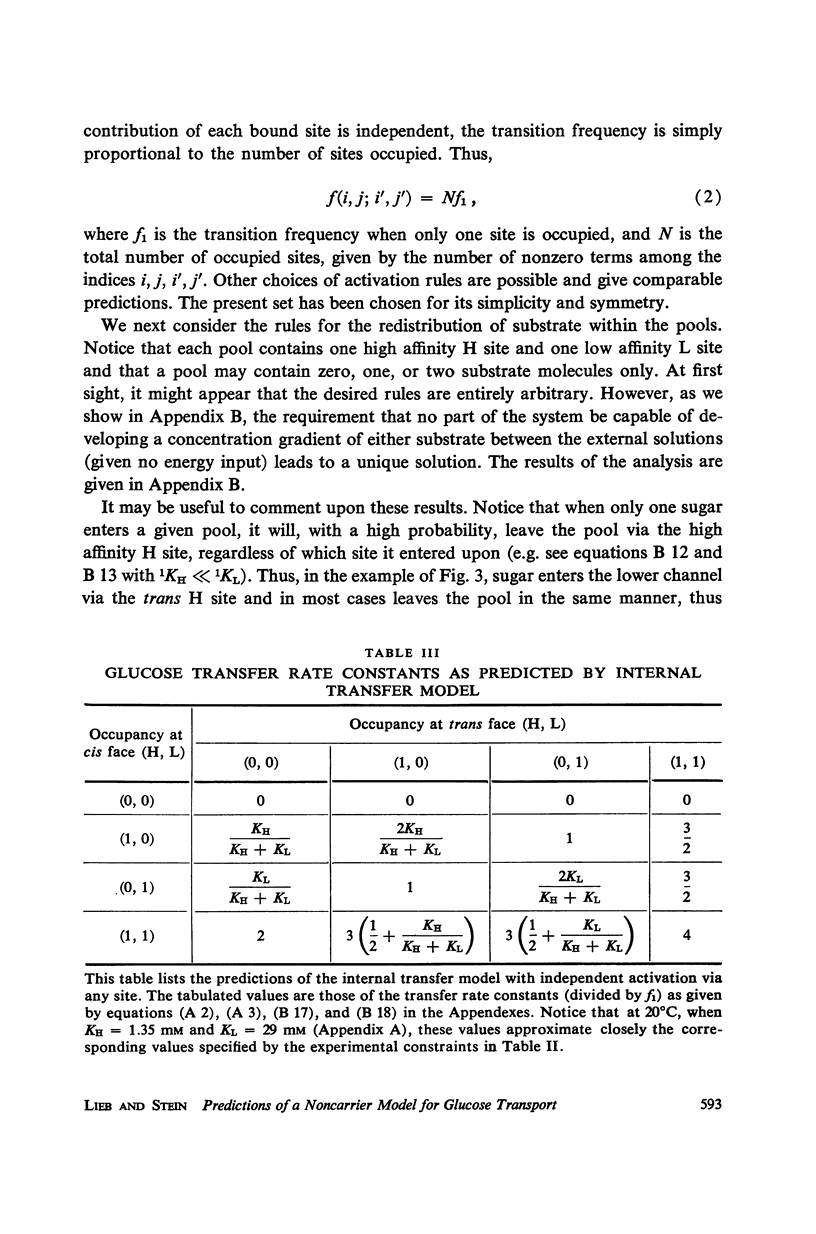
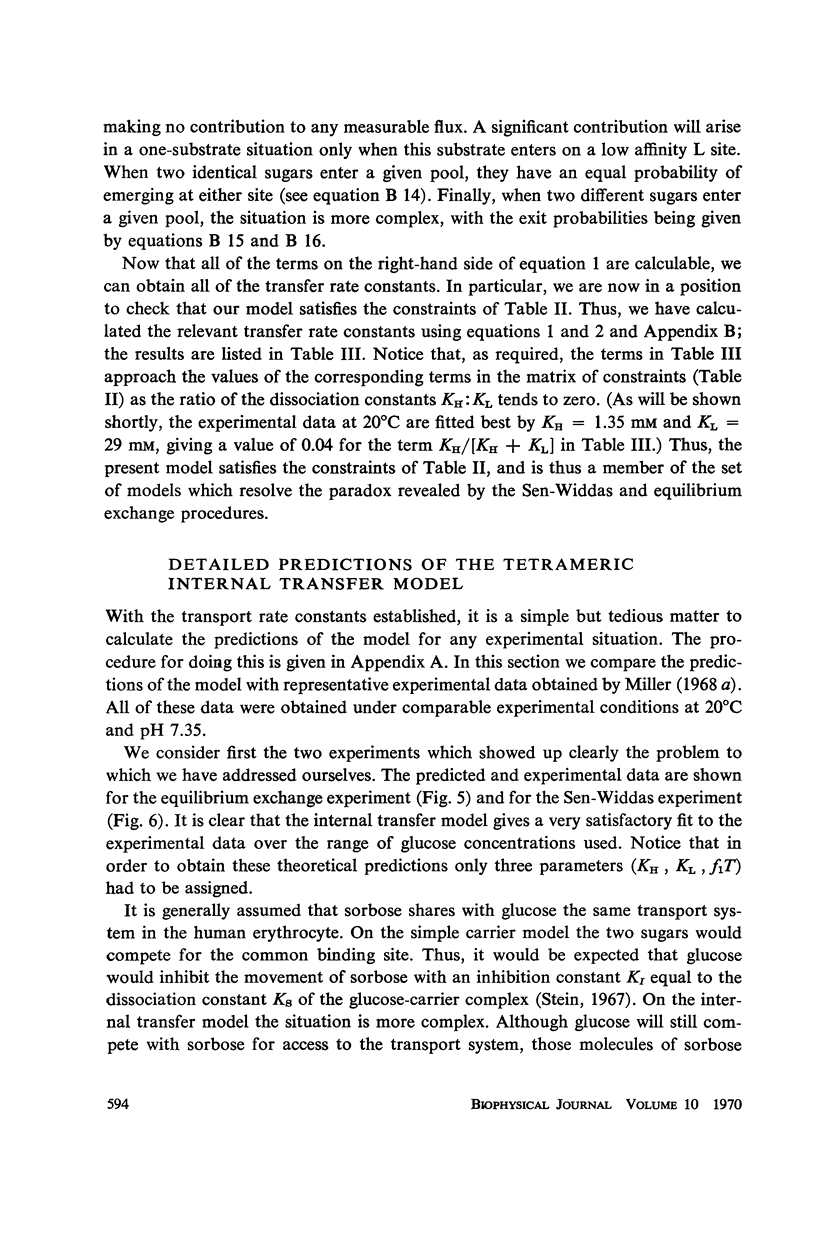
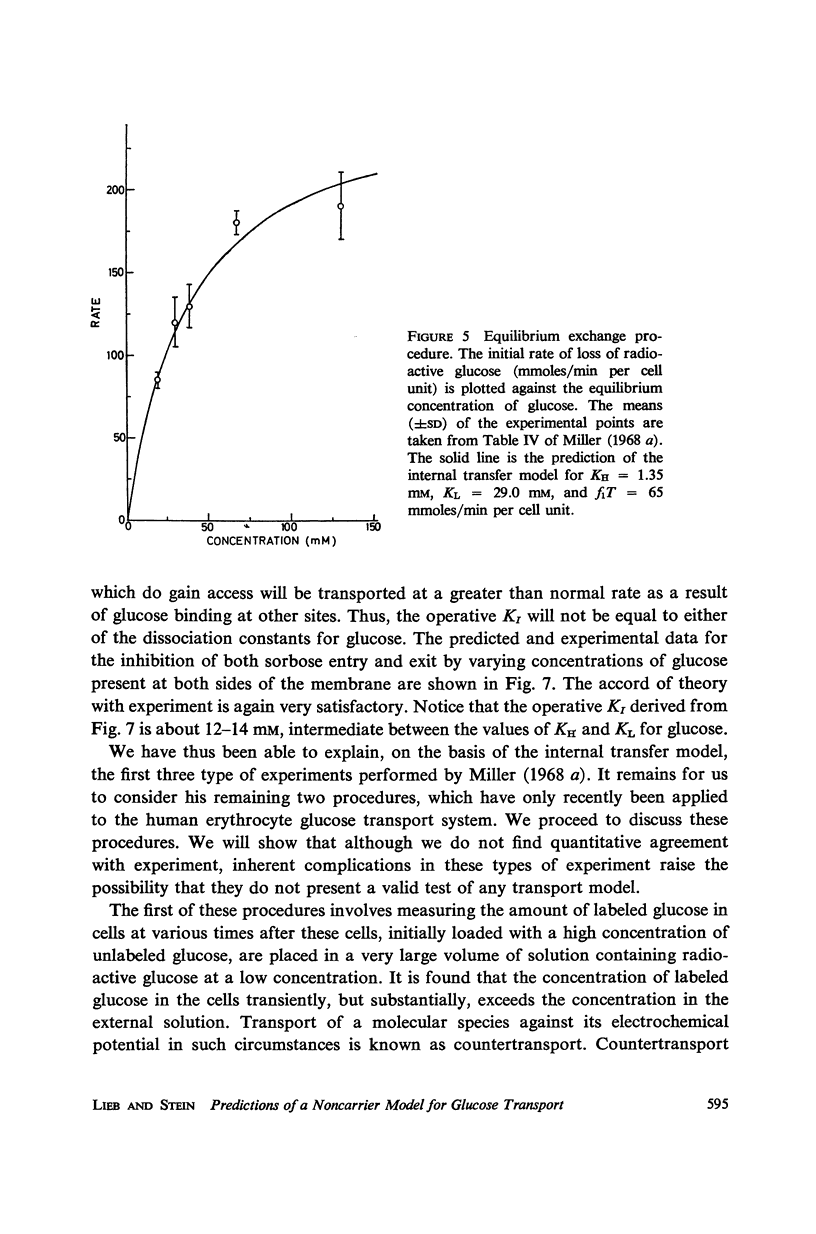
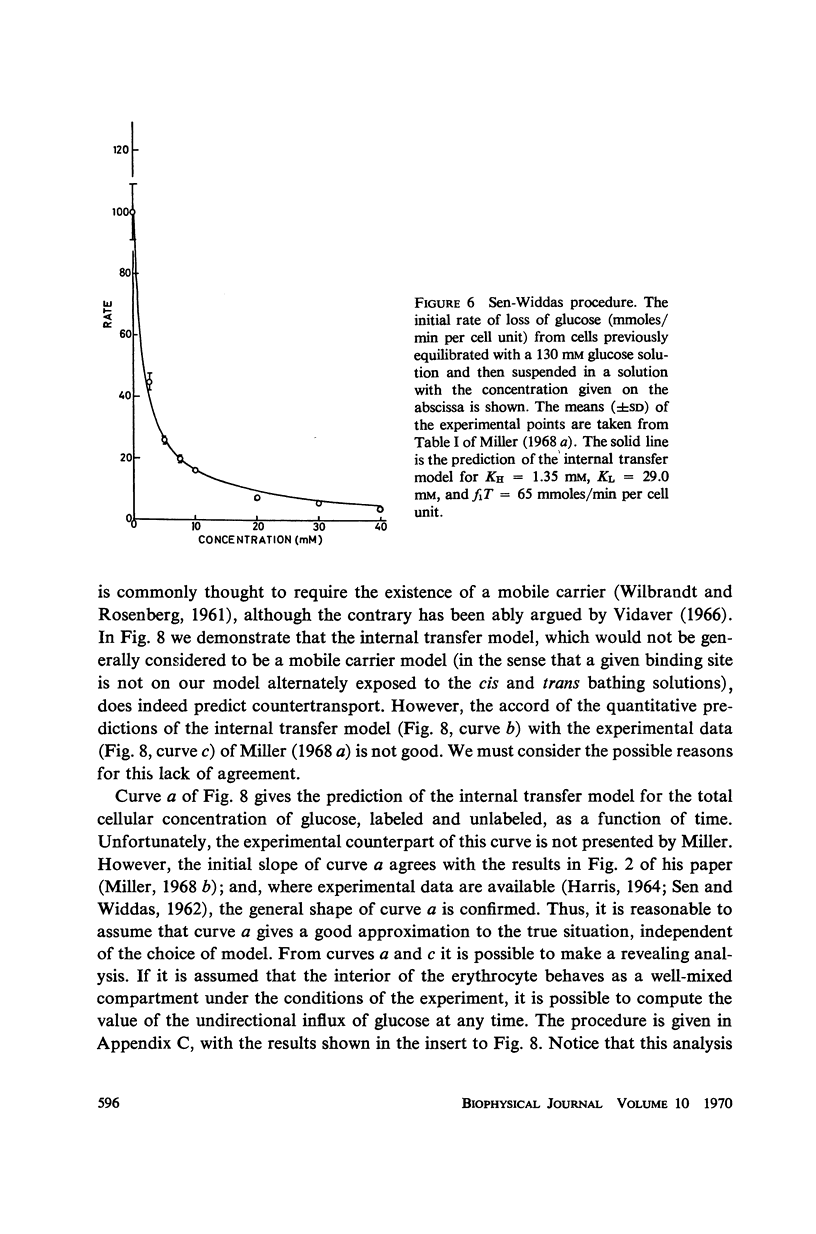
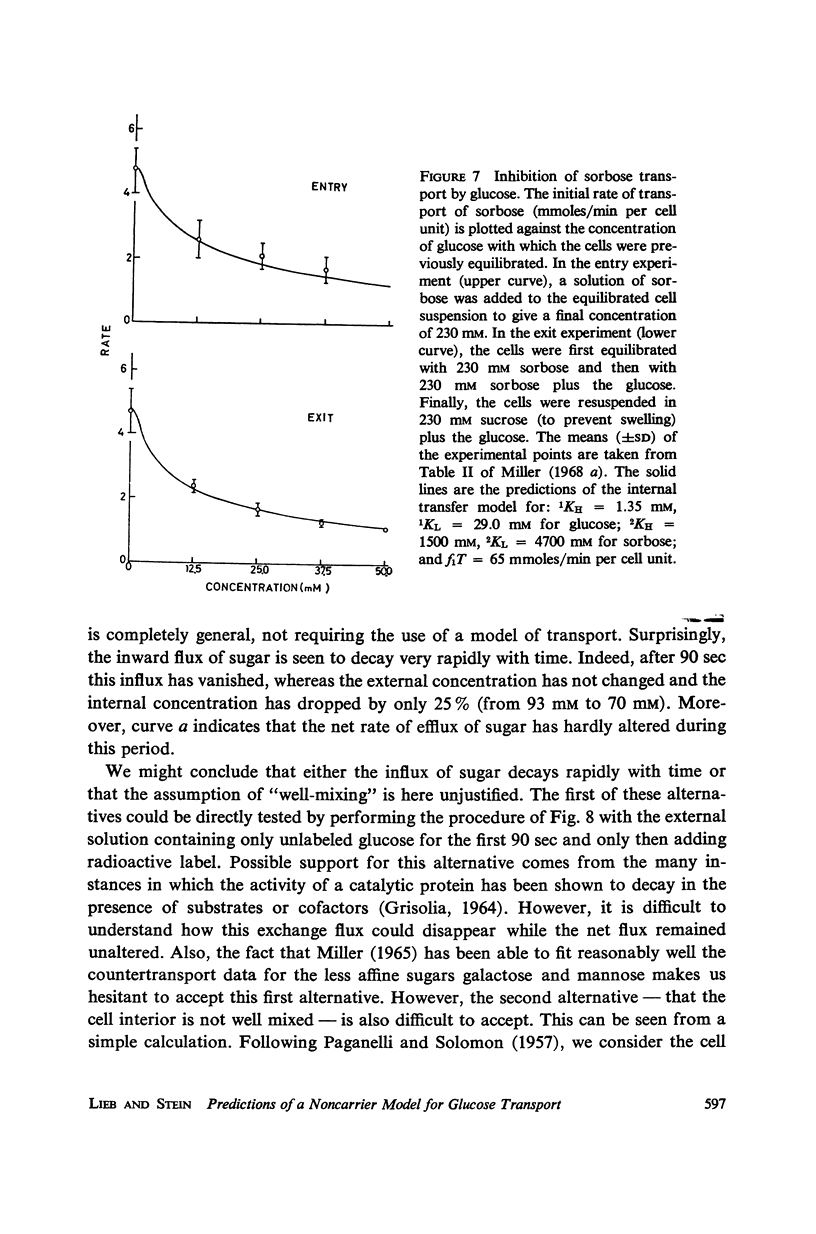
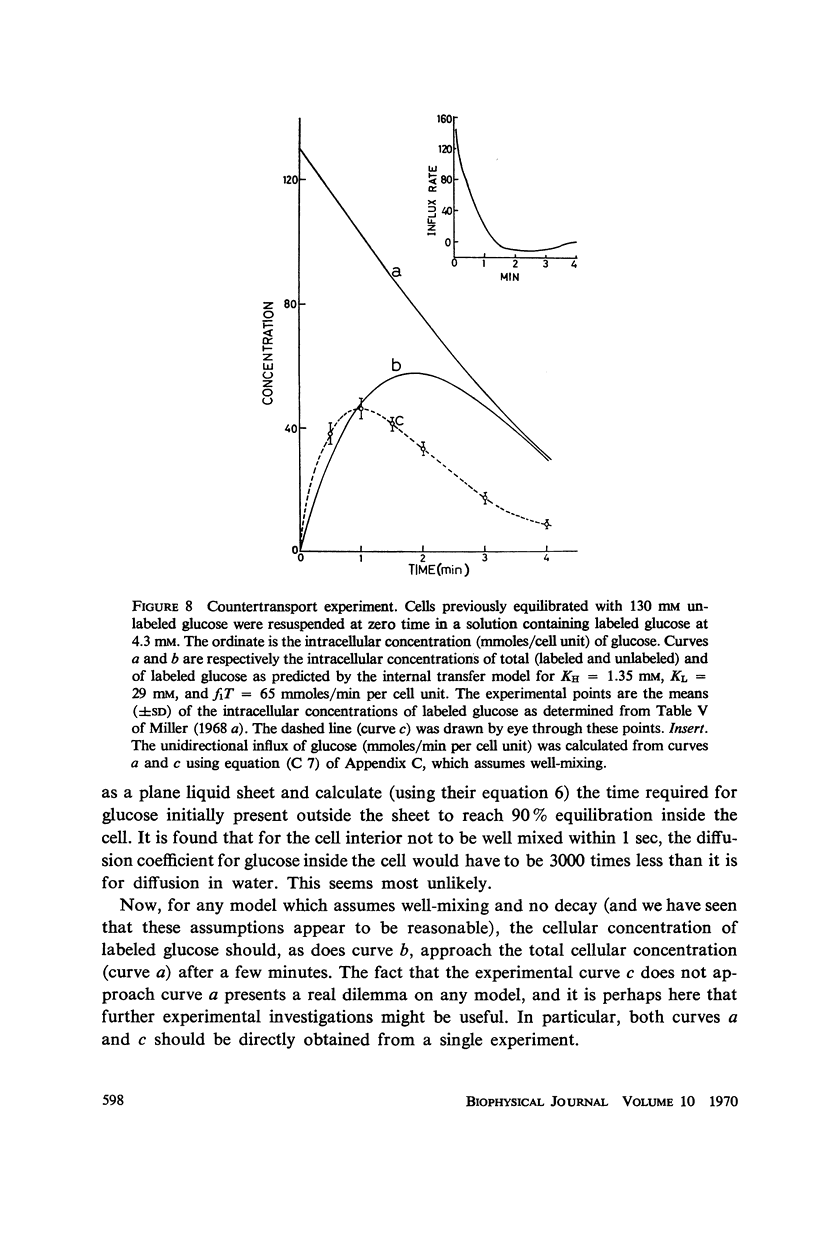
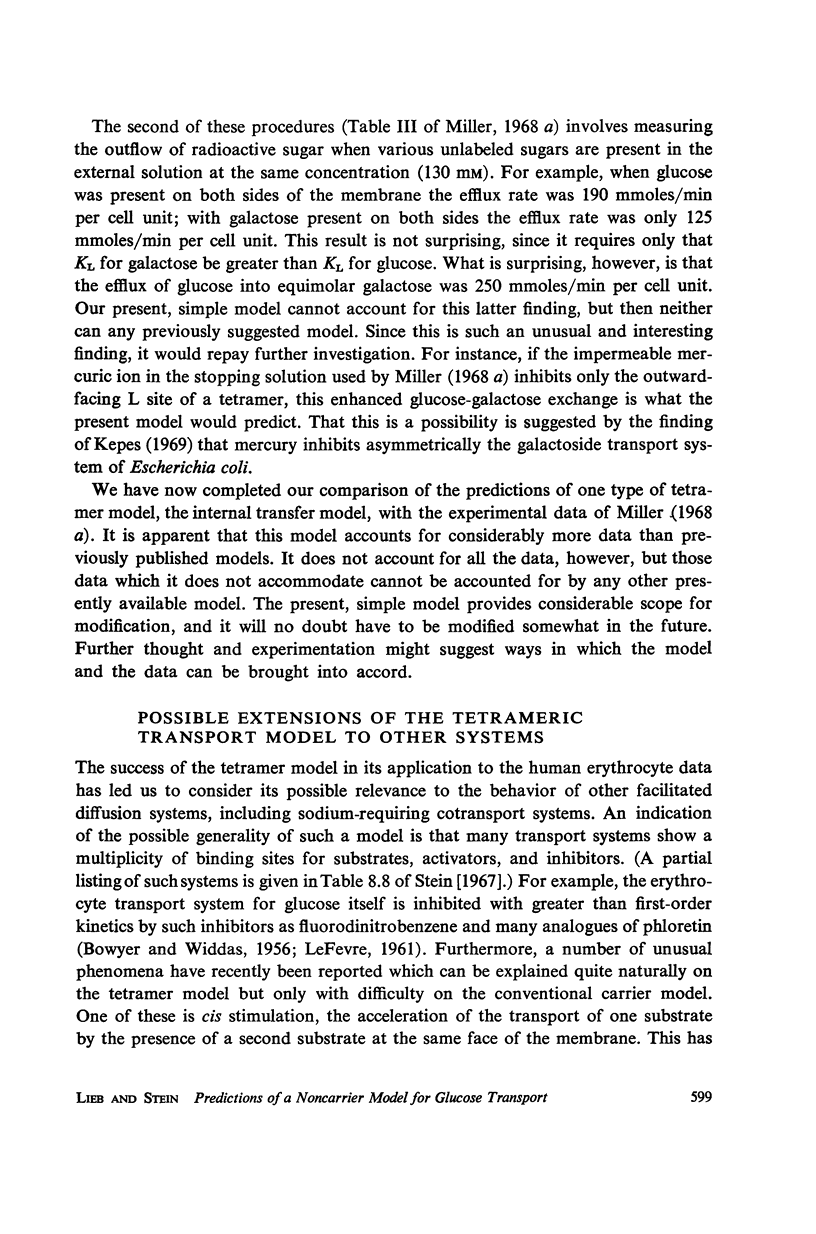
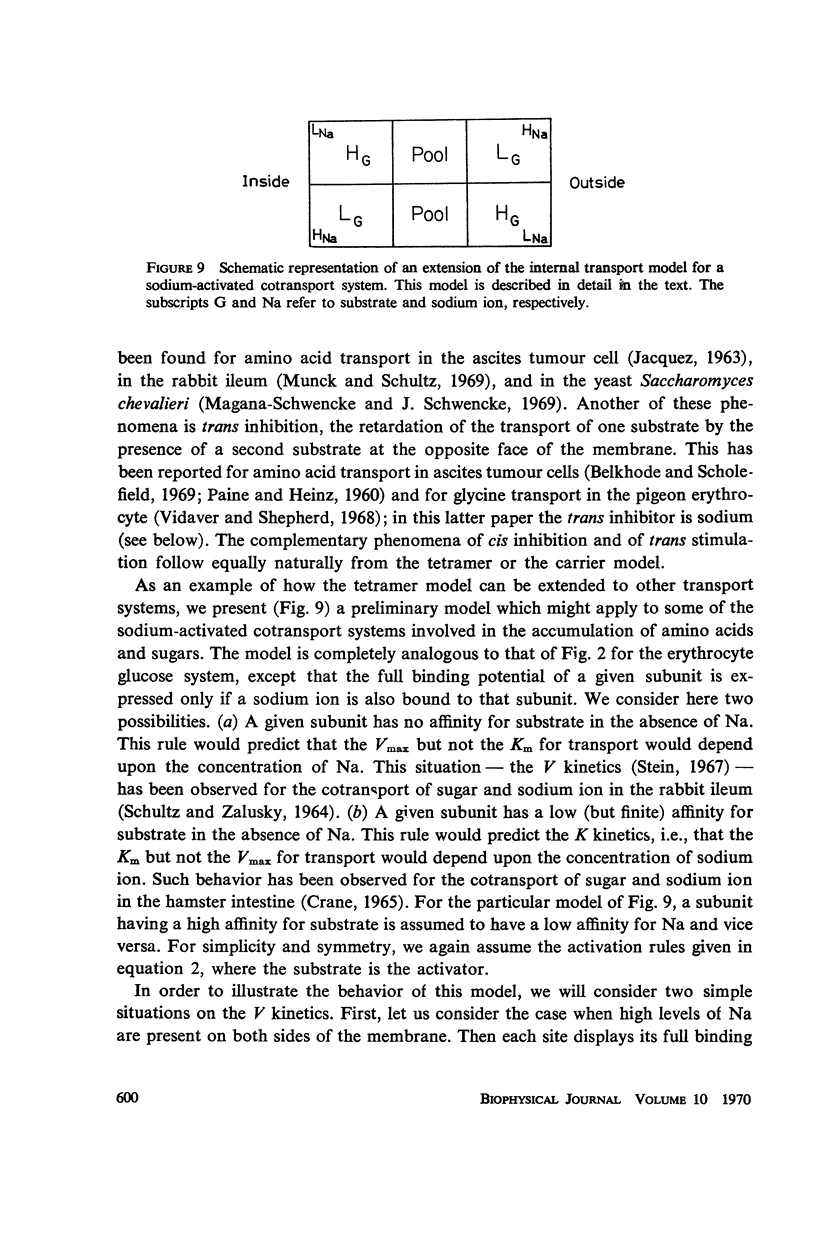
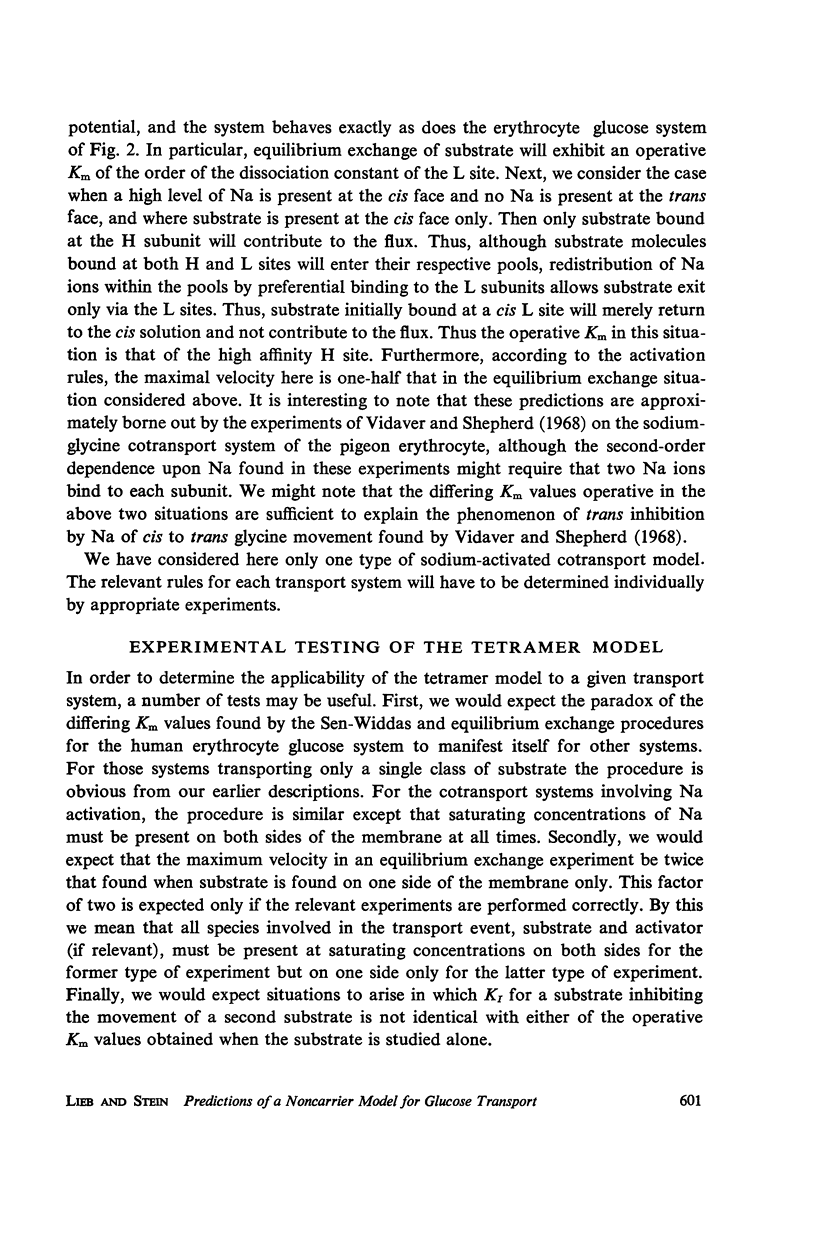
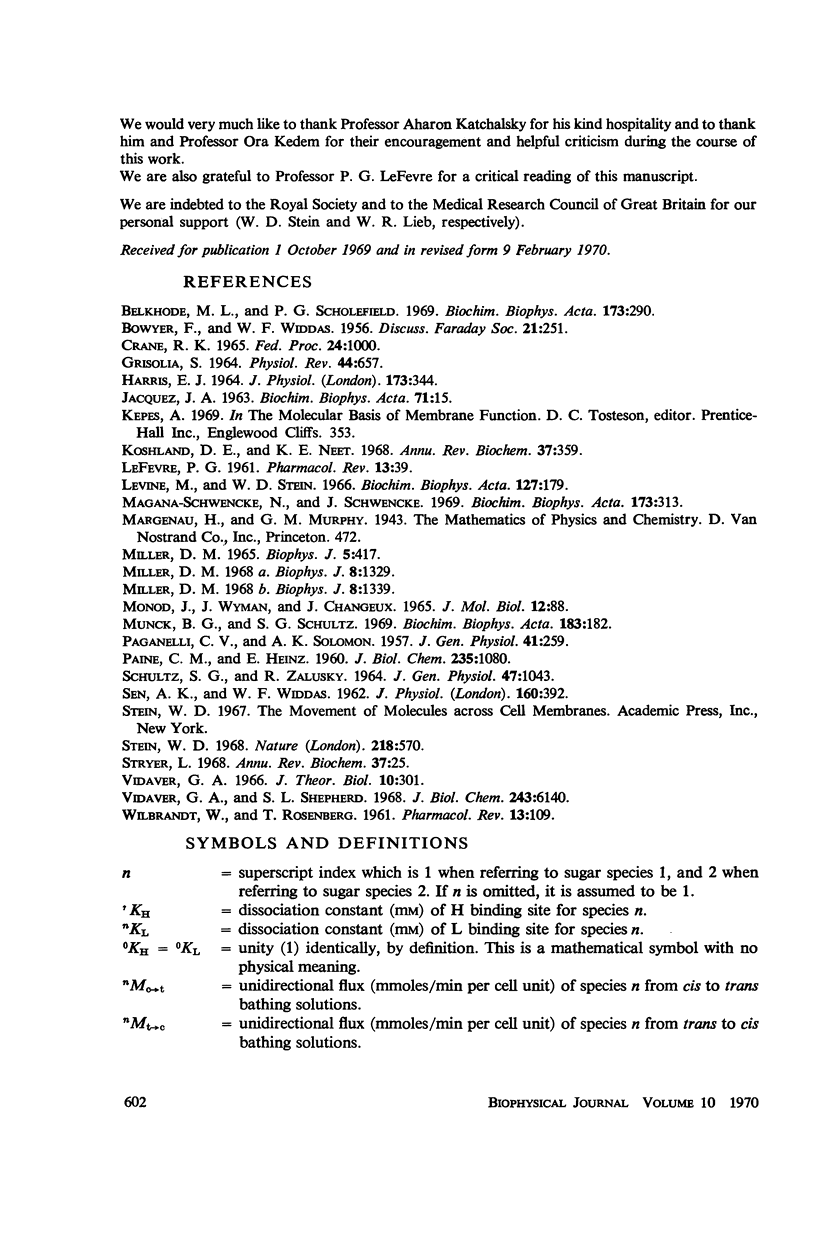
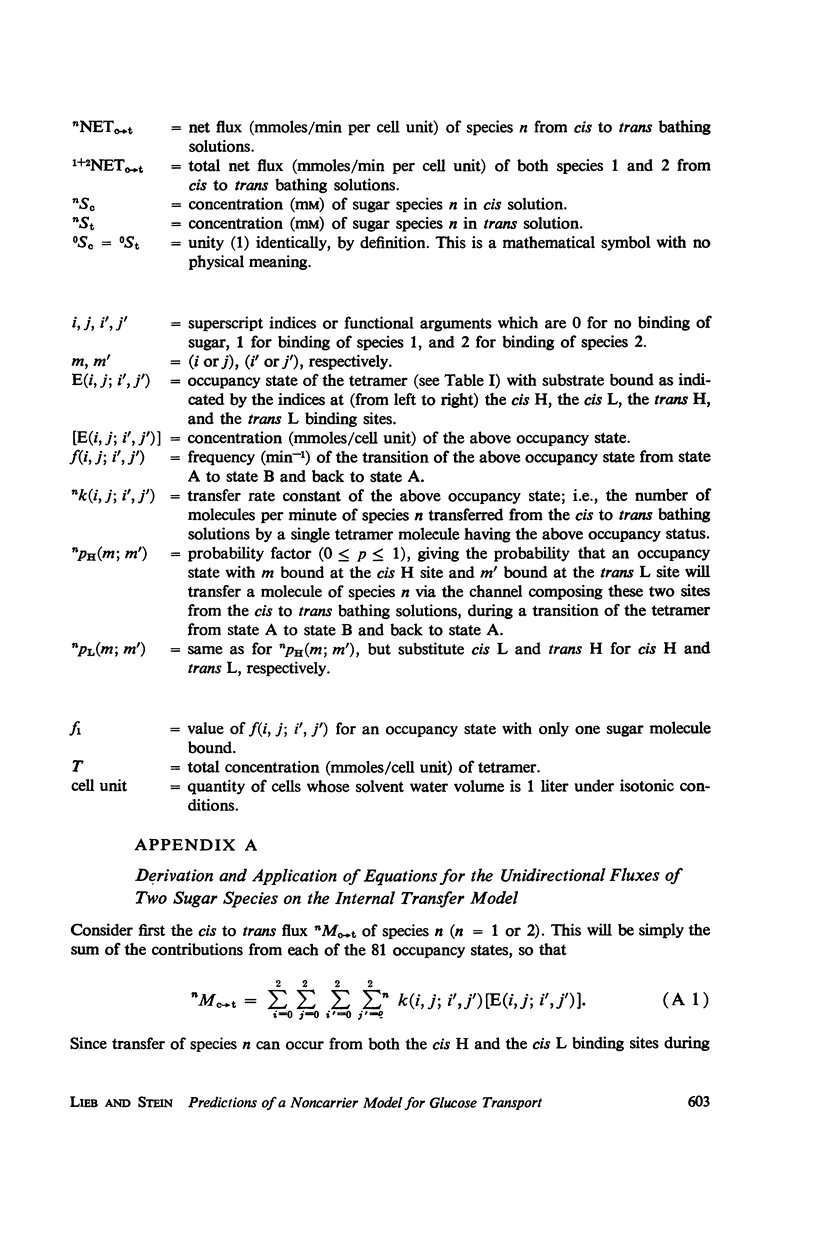
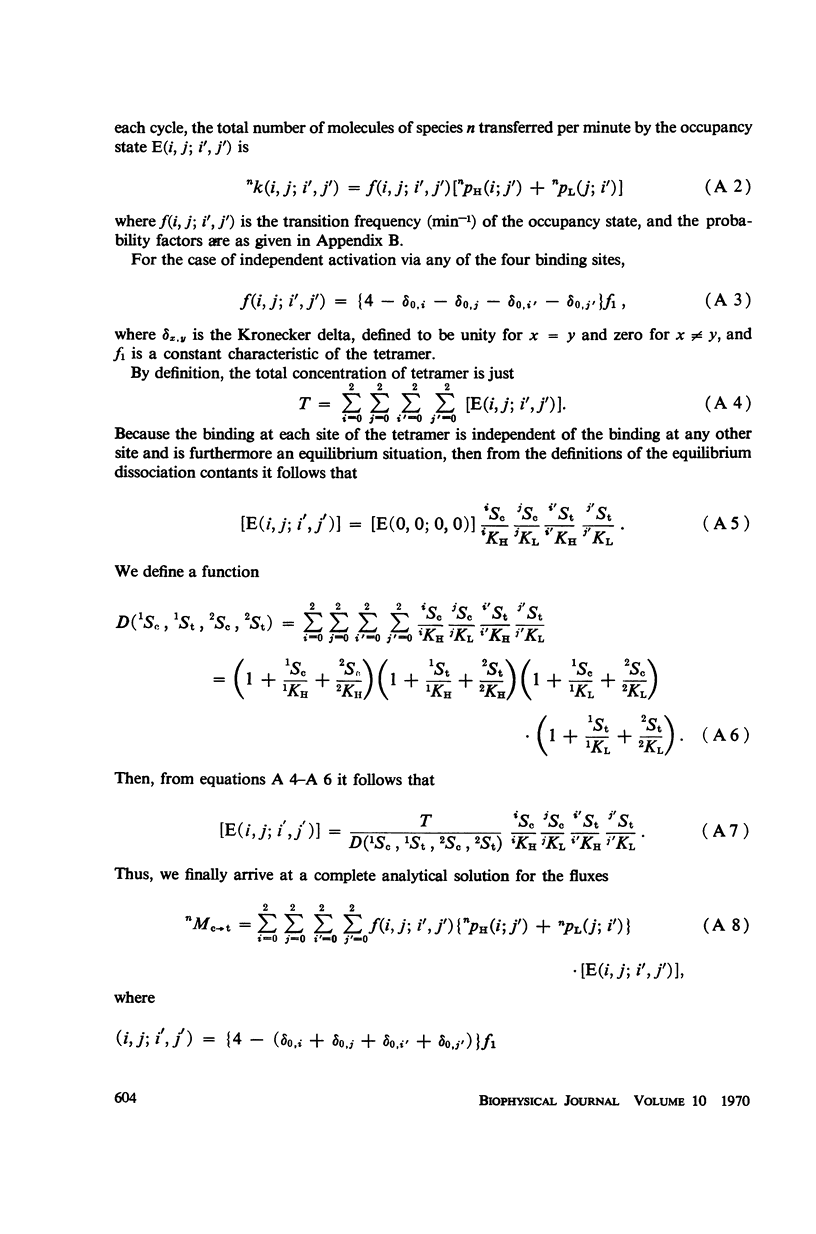
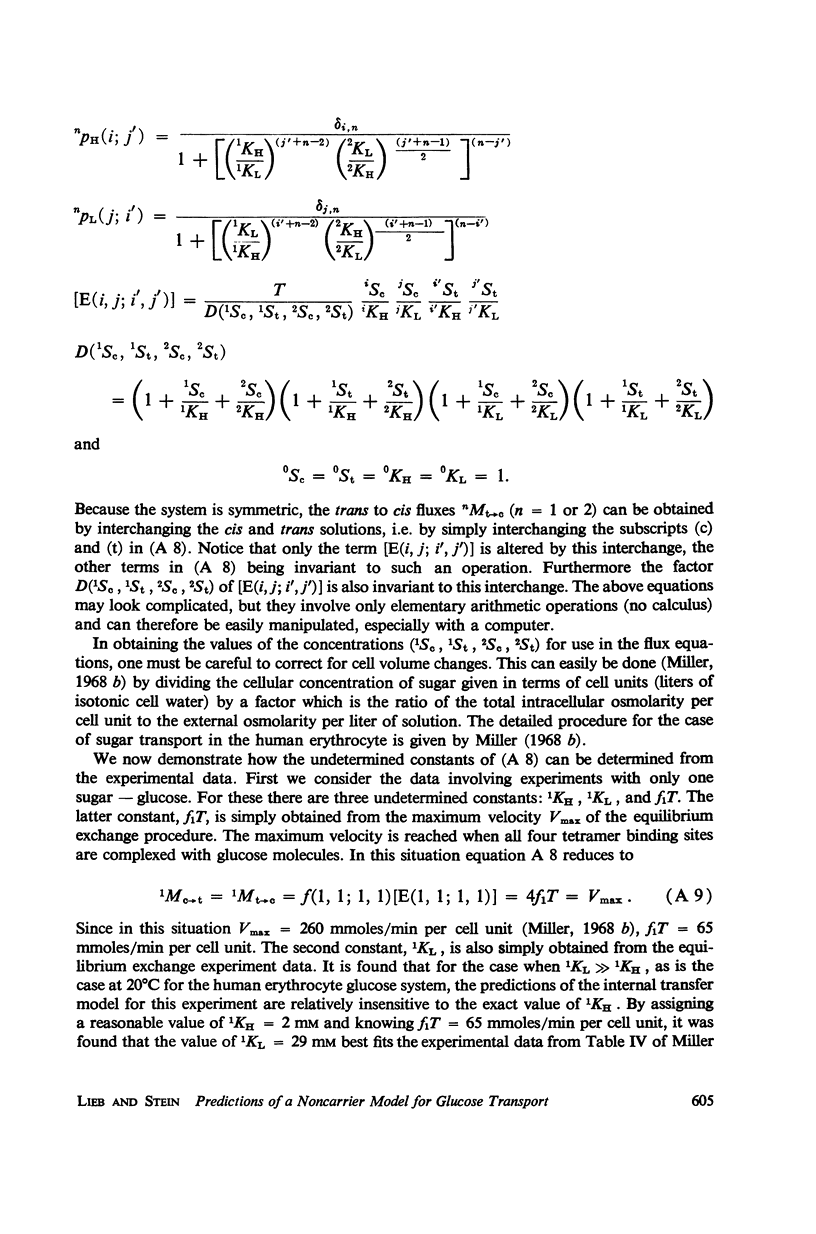
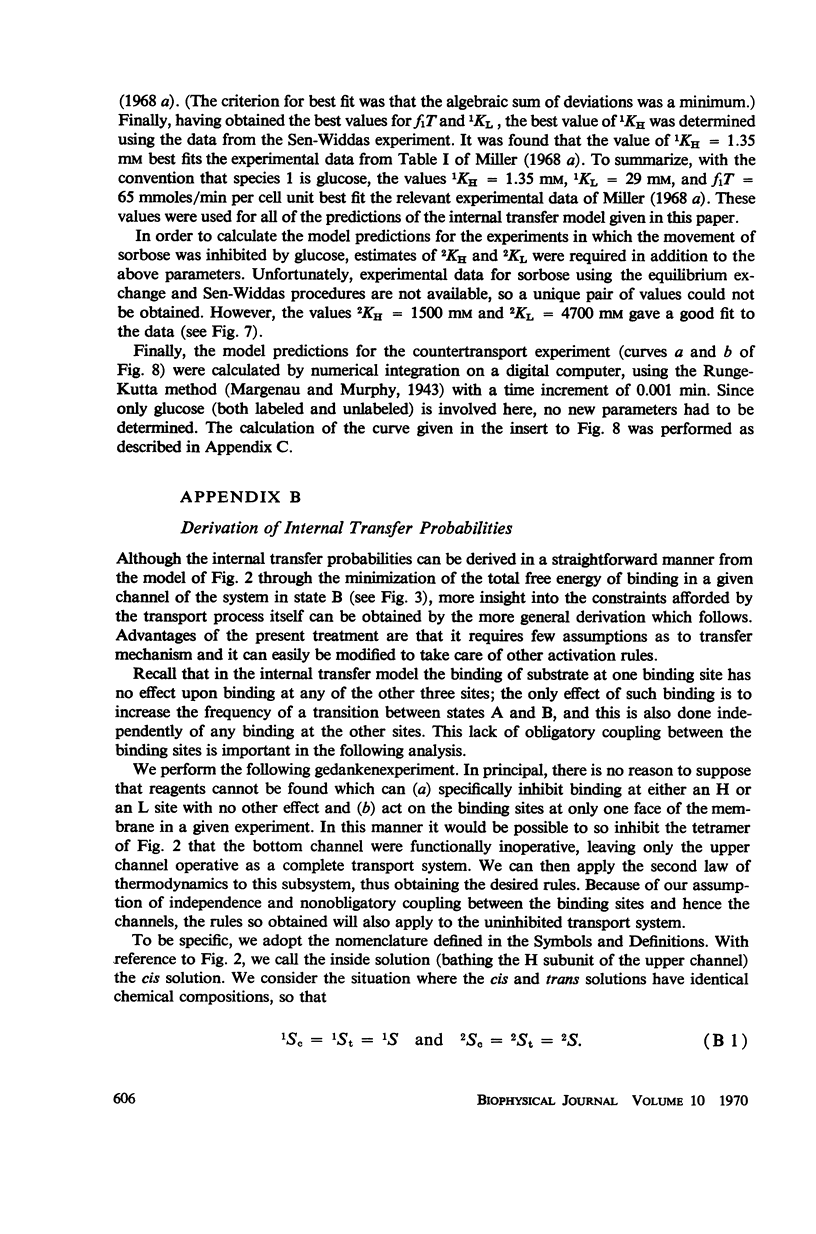
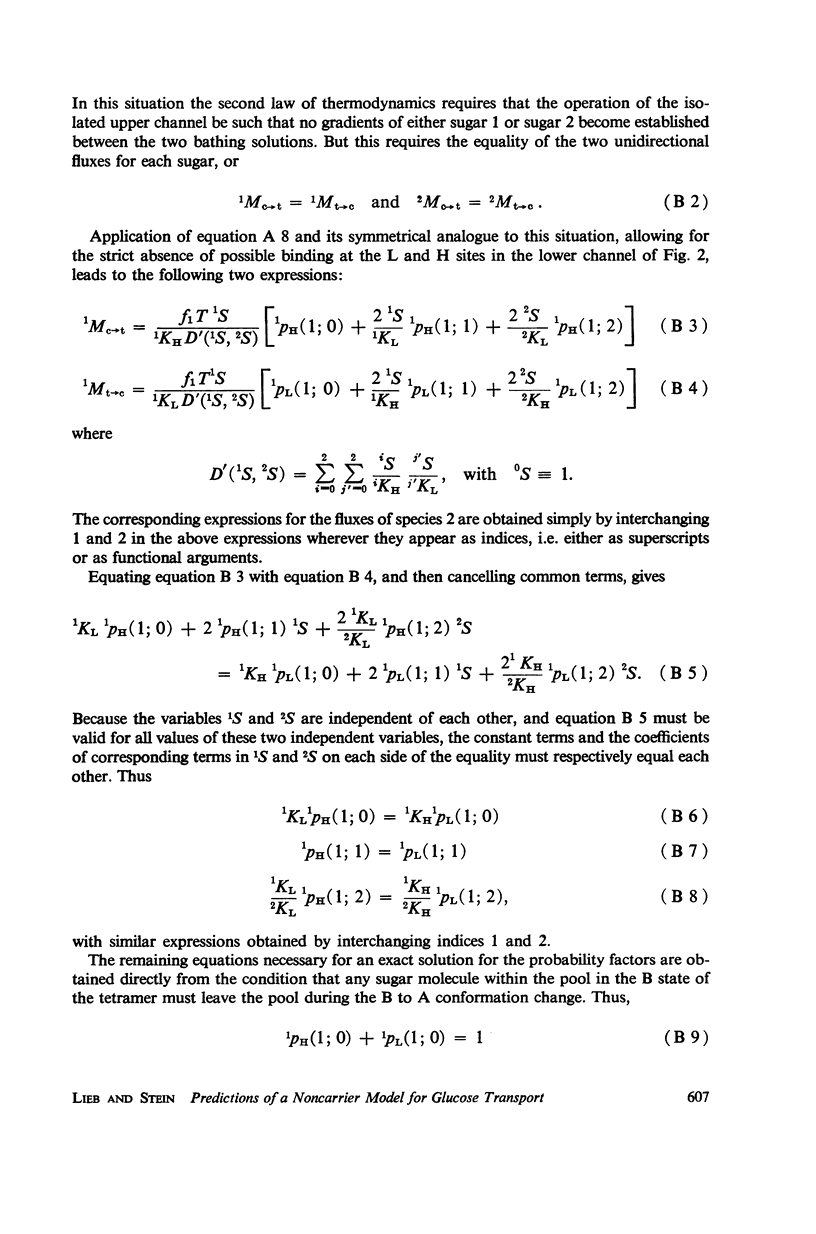
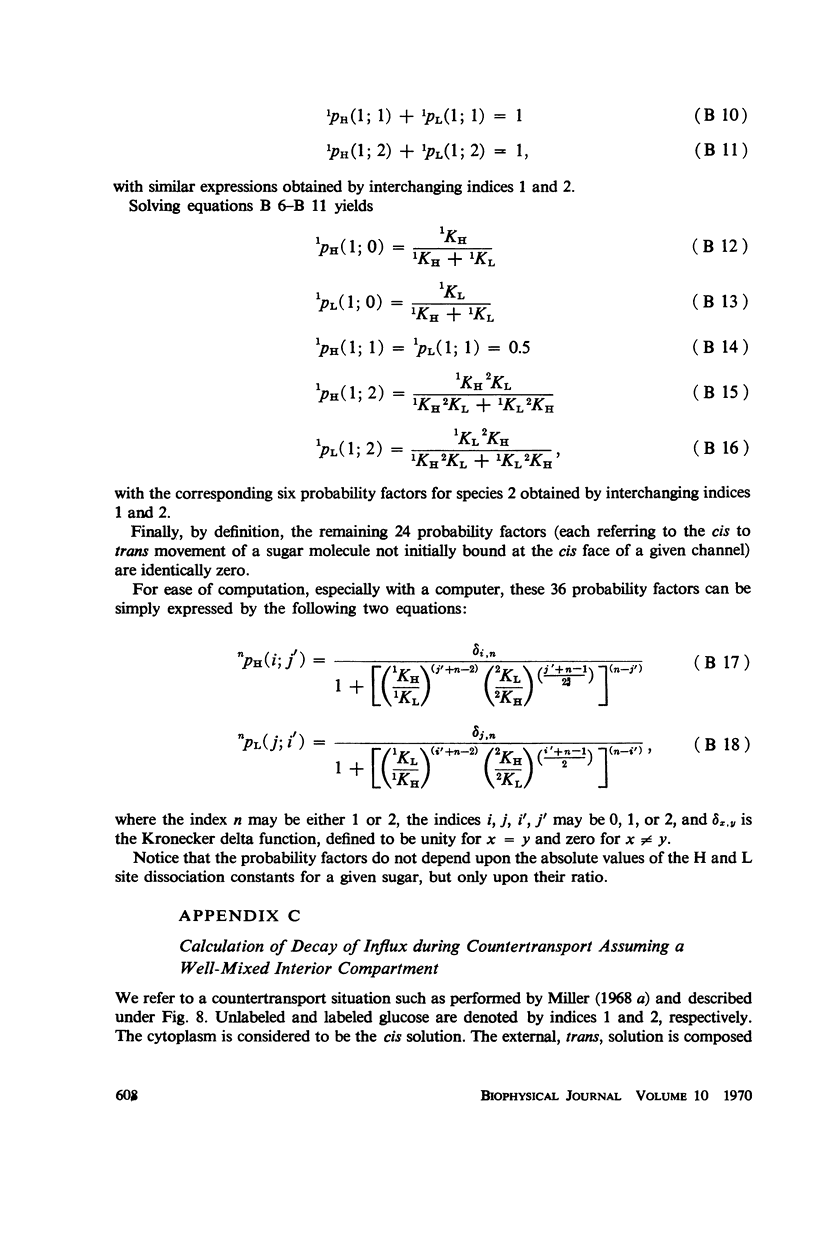
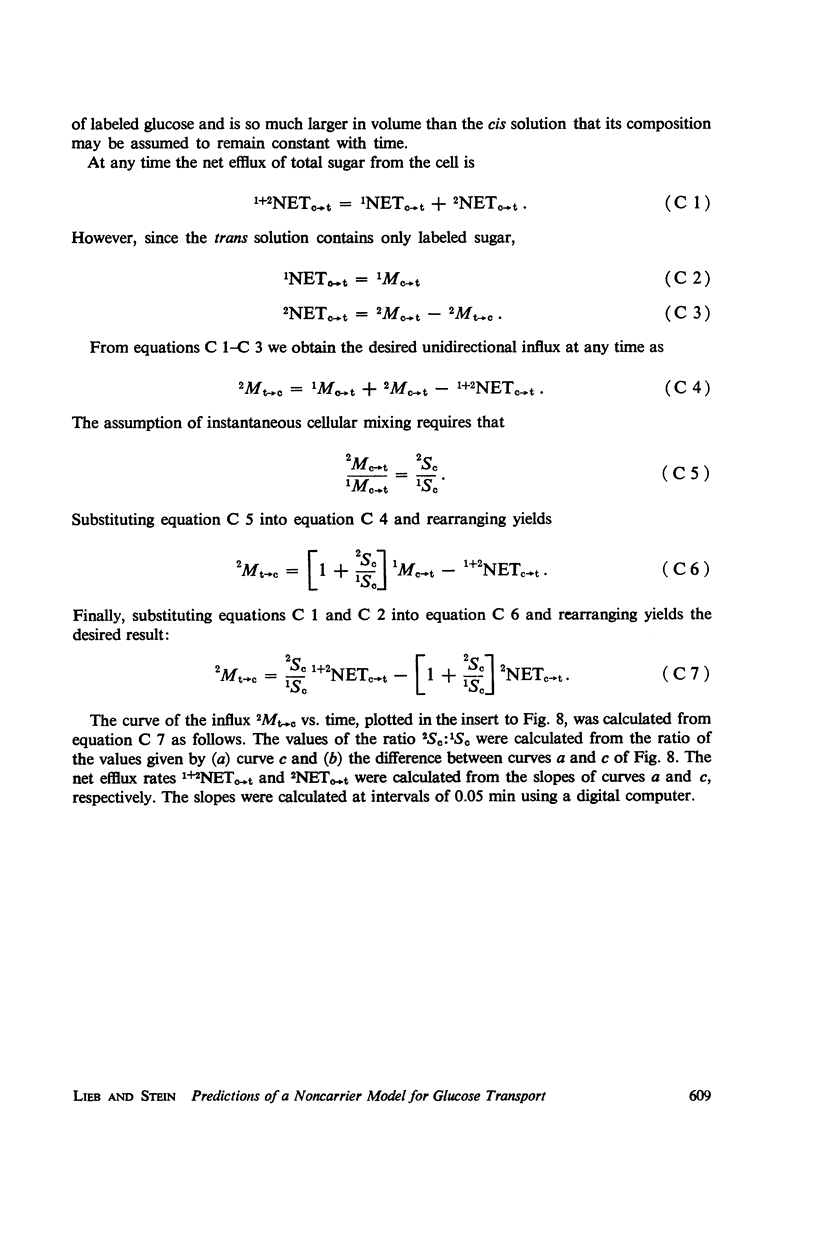
Selected References
These references are in PubMed. This may not be the complete list of references from this article.
- Belkhode M. L., Scholefield P. G. Interactions between amino acids during transport and exchanage diffusion in Novikoff and Ehrlich ascites tumor cells. Biochim Biophys Acta. 1969 Mar 11;173(2):290–301. doi: 10.1016/0005-2736(69)90112-6. [DOI] [PubMed] [Google Scholar]
- Crane R. K. Na+ -dependent transport in the intestine and other animal tissues. Fed Proc. 1965 Sep-Oct;24(5):1000–1006. [PubMed] [Google Scholar]
- GRISOLIA S. THE CATALYTIC ENVIRONMENT AND ITS BIOLOGICAL IMPLICATIONS. Physiol Rev. 1964 Oct;44:657–712. doi: 10.1152/physrev.1964.44.4.657. [DOI] [PubMed] [Google Scholar]
- HARRIS E. J. AN ANALYTICAL STUDY OF THE KINETICS OF GLUCOSE MOVEMENT IN HUMAN ERYTHROCYTES. J Physiol. 1964 Oct;173:344–353. doi: 10.1113/jphysiol.1964.sp007460. [DOI] [PMC free article] [PubMed] [Google Scholar]
- JACQUEZ J. A. Carrier-amino acid stoichiometry in amino acid transport in Ehrlich ascites cells. Biochim Biophys Acta. 1963 Apr;71:15–33. doi: 10.1016/0006-3002(63)90981-8. [DOI] [PubMed] [Google Scholar]
- Koshland D. E., Jr, Neet K. E. The catalytic and regulatory properties of enzymes. Annu Rev Biochem. 1968;37:359–410. doi: 10.1146/annurev.bi.37.070168.002043. [DOI] [PubMed] [Google Scholar]
- Levine M., Stein W. D. The kinetic parameters of the monosaccharide transfer system of the human erythrocyte. Biochim Biophys Acta. 1966 Sep 26;127(1):179–193. doi: 10.1016/0304-4165(66)90488-0. [DOI] [PubMed] [Google Scholar]
- MONOD J., WYMAN J., CHANGEUX J. P. ON THE NATURE OF ALLOSTERIC TRANSITIONS: A PLAUSIBLE MODEL. J Mol Biol. 1965 May;12:88–118. doi: 10.1016/s0022-2836(65)80285-6. [DOI] [PubMed] [Google Scholar]
- Magaña-Schwencke N., Schwencke J. A proline transport system in Saccharomyces chevalieri. Biochim Biophys Acta. 1969 Mar 11;173(2):313–323. doi: 10.1016/0005-2736(69)90114-x. [DOI] [PubMed] [Google Scholar]
- Munck B. G., Schultz S. G. Interactions between leucine and lysine transport in rabbit ileum. Biochim Biophys Acta. 1969 Jun 3;183(1):182–193. doi: 10.1016/0005-2736(69)90142-4. [DOI] [PubMed] [Google Scholar]
- PAGANELLI C. V., SOLOMON A. K. The rate of exchange of tritiated water across the human red cell membrane. J Gen Physiol. 1957 Nov 20;41(2):259–277. doi: 10.1085/jgp.41.2.259. [DOI] [PMC free article] [PubMed] [Google Scholar]
- PAINE C. M., HEINZ E. The structural specificity of the glycine transport system of Ehrlich carcinoma cells. J Biol Chem. 1960 Apr;235:1080–1085. [PubMed] [Google Scholar]
- SCHULTZ S. G., ZALUSKY R. ION TRANSPORT IN ISOLATED RABBIT ILEUM. II. THE INTERACTION BETWEEN ACTIVE SODIUM AND ACTIVE SUGAR TRANSPORT. J Gen Physiol. 1964 Jul;47:1043–1059. doi: 10.1085/jgp.47.6.1043. [DOI] [PMC free article] [PubMed] [Google Scholar]
- SEN A. K., WIDDAS W. F. Determination of the temperature and pH dependence of glucose transfer across the human erythrocyte membrane measured by glucose exit. J Physiol. 1962 Mar;160:392–403. doi: 10.1113/jphysiol.1962.sp006854. [DOI] [PMC free article] [PubMed] [Google Scholar]
- Stein W. D. Turnover numbers of membrane carriers and the action of polypeptide antibiotics. Nature. 1968 May 11;218(5141):570–571. doi: 10.1038/218570a0. [DOI] [PubMed] [Google Scholar]
- Stryer L. Implications of X-ray crystallographic studies of protein structure. Annu Rev Biochem. 1968;37:25–50. doi: 10.1146/annurev.bi.37.070168.000325. [DOI] [PubMed] [Google Scholar]
- Vidaver G. A. Inhibition of parallel flux and augmentation of counter flux shown by transport models not involving a mobile carrier. J Theor Biol. 1966 Feb;10(2):301–306. doi: 10.1016/0022-5193(66)90128-7. [DOI] [PubMed] [Google Scholar]
- Vidaver G. A., Shepherd S. L. Transport of glycine by hemolyzed and restored pigeon red blood cells. Symmetry properties, trans effects of sodium ion and glycine, and their description by a single rate equation. J Biol Chem. 1968 Dec 10;243(23):6140–6150. [PubMed] [Google Scholar]
- WILBRANDT W., ROSENBERG T. The concept of carrier transport and its corollaries in pharmacology. Pharmacol Rev. 1961 Jun;13:109–183. [PubMed] [Google Scholar]