Abstract
1. The contribution by glial cells to surface recordings has been examined in the optic nerve of the amphibian Necturus maculosus. The method of current injection was employed selectively to alter the membrane potential of glial cells without affecting that of the axons. The resulting changes in potential were recorded simultaneously from the surface of the nerve using the sucrose gap method and intracellularly from a glial cell near the gap.
2. The sucrose gap method recorded 40% of the changes in glial membrane potential. This percentage was not affected when the current electrode was inserted into different glial cells while maintaining the recording conditions constant.
3. Following axonal degeneration, produced by removing the eye 2-3 months earlier, the percentage contribution by glia increased to 84%.
4. By measuring sucrose gap responses to changes in Ko it was possible to estimate that the sucrose gap method recorded 31-60% of changes in axonal membrane potential. It was also determined that the axons, unlike glial cells, are relatively insensitive to reductions in Ko. Surface responses to decreases in external potassium thus reflect the magnitude of the glial contribution.
5. It is concluded that changes in glial membrane potential contribute about as much to surface recordings from the optic nerve of Necturus as do equivalent changes in axonal membrane potential. The contributions by the glial cells and axons are related to the relative volumes of tissue they respectively occupy. The significance of these findings to the analysis of surface recordings from the mammalian brain is discussed. Since mammalian glial cells, like those in Amphibia and the leech, become depolarized during neuronal activity and on the basis of electron microscopic evidence appear to be electrically coupled, it is likely that they contribute to the electroencephalogram.
Full text
PDF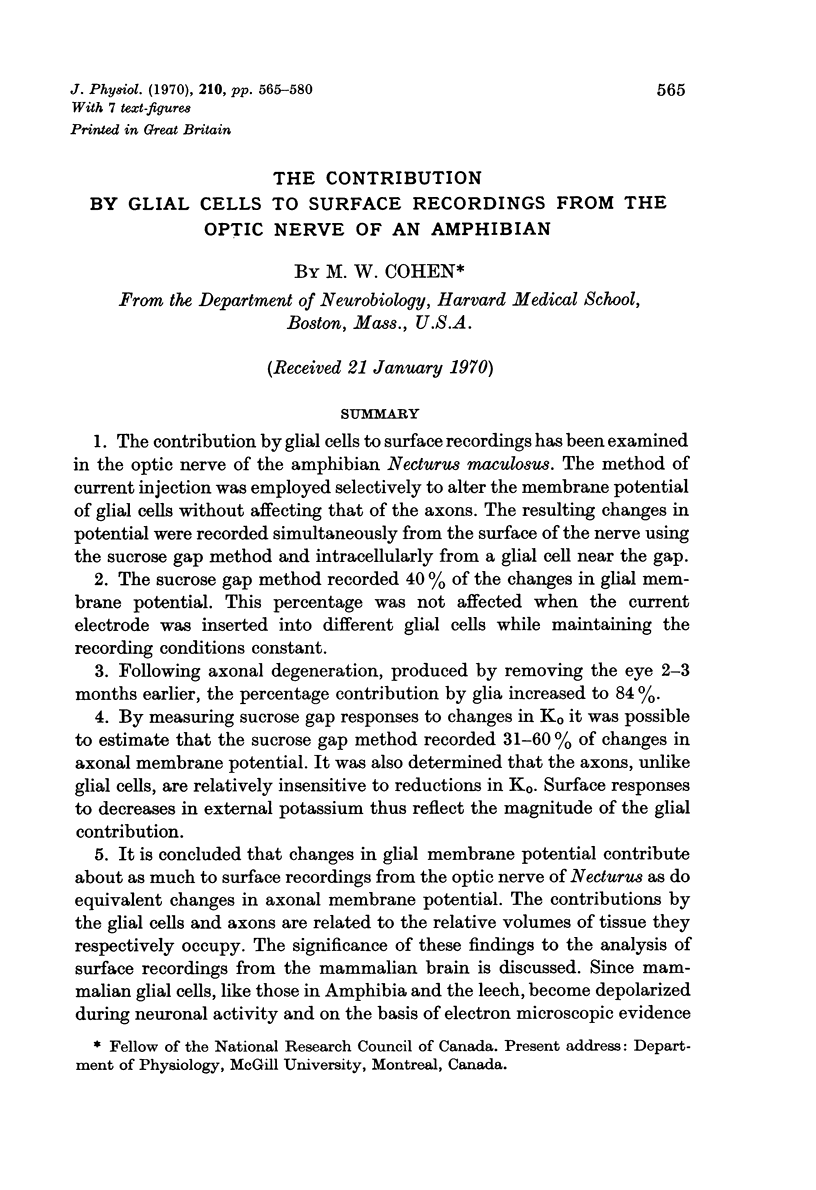
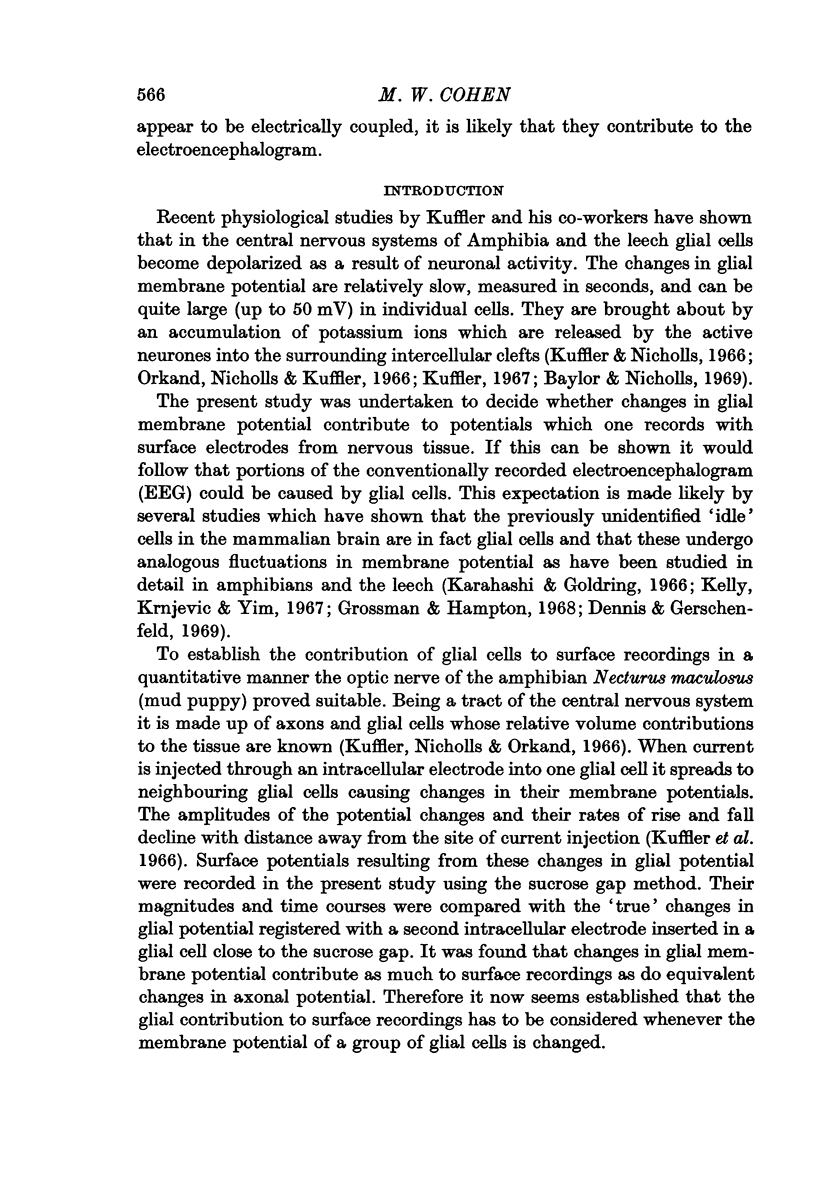
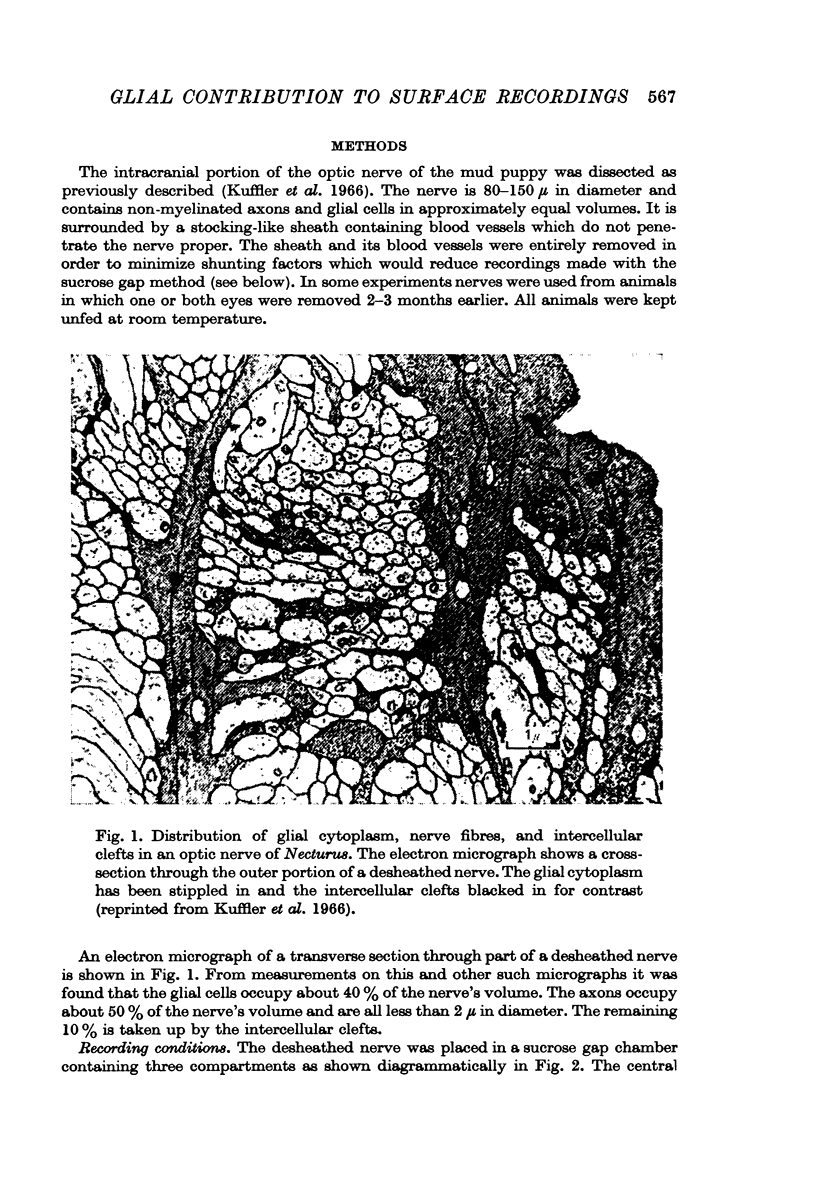
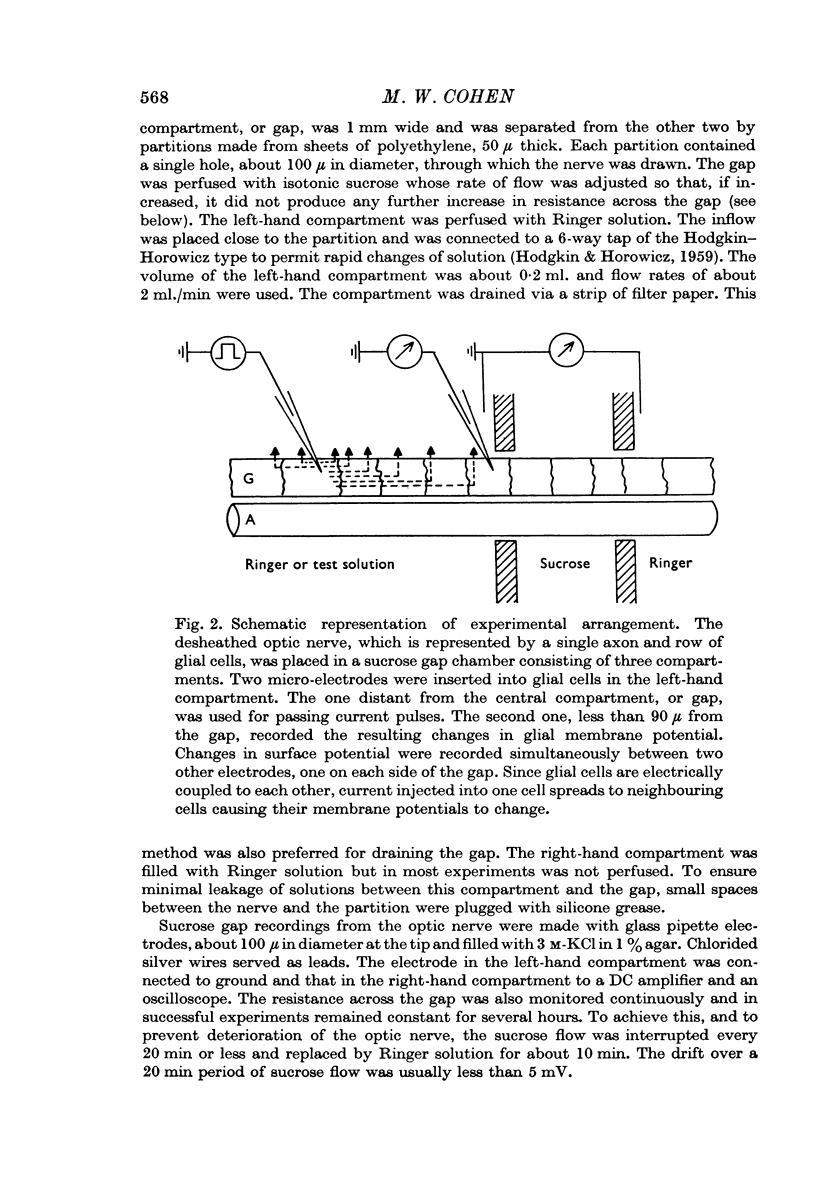
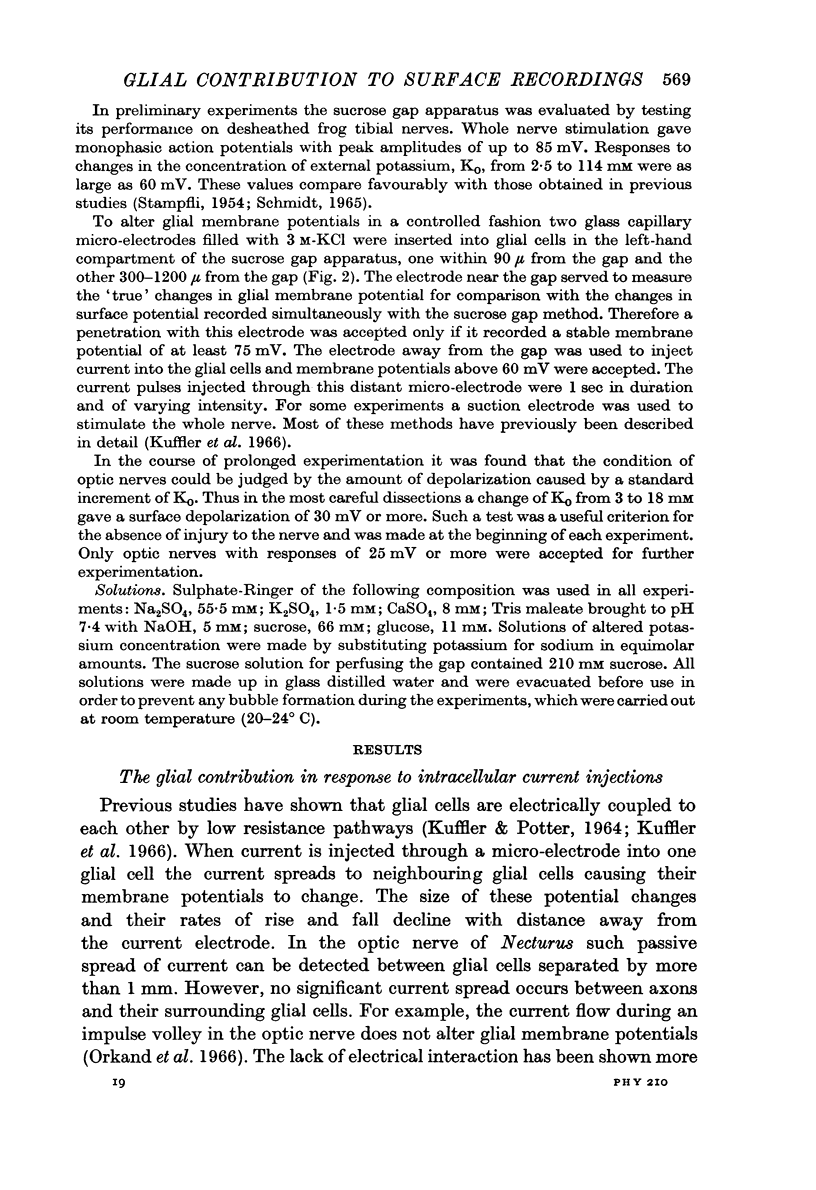
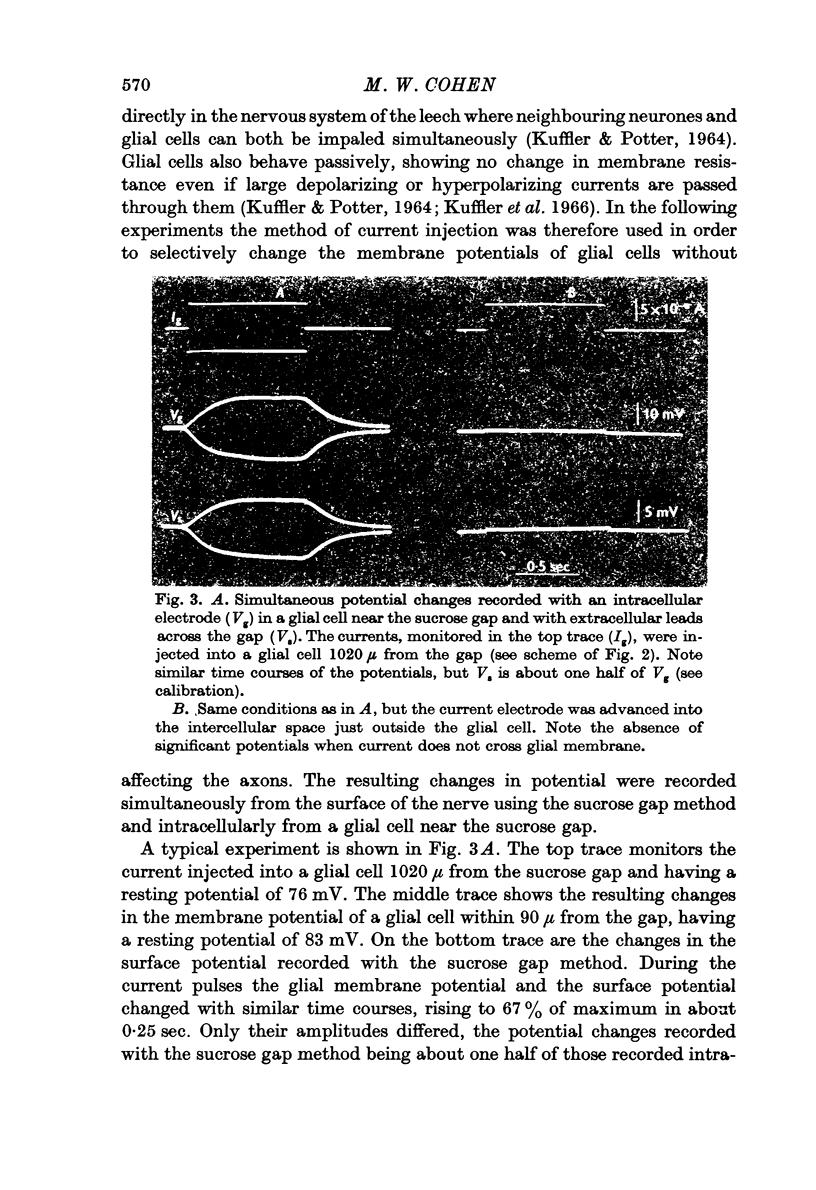
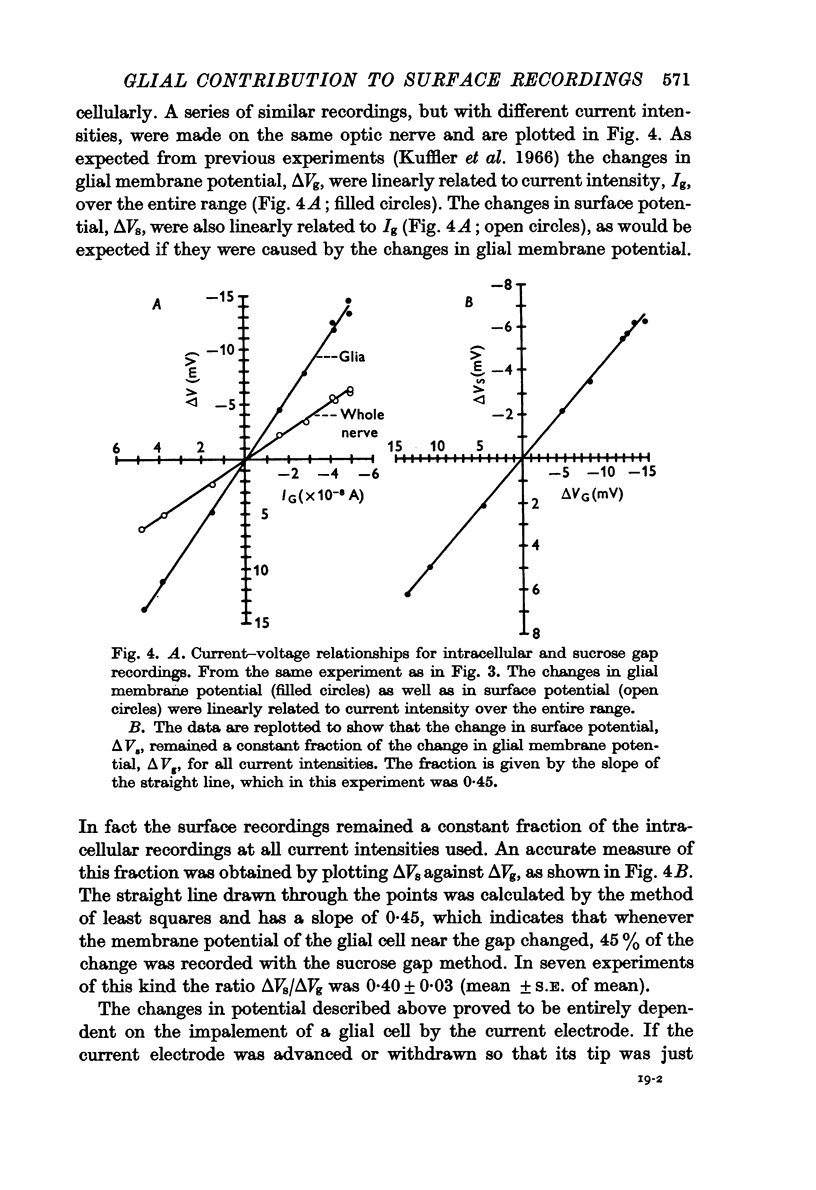
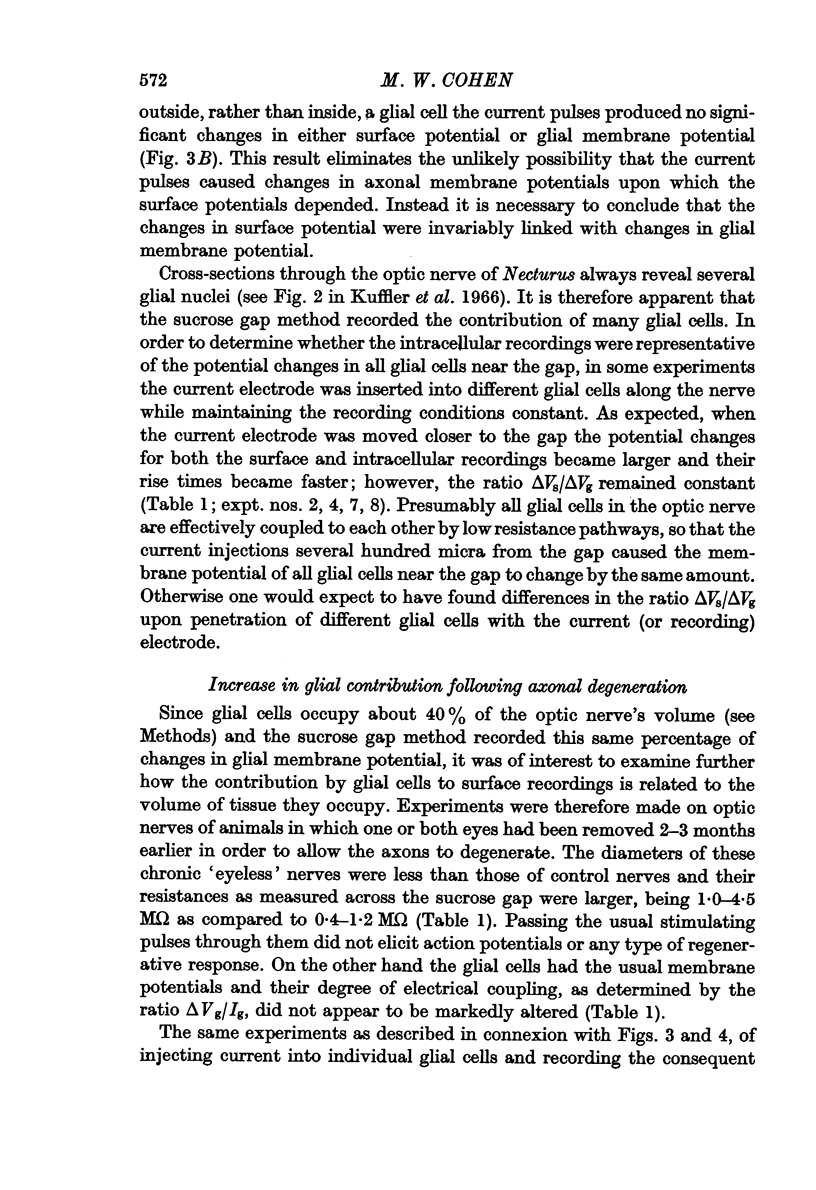
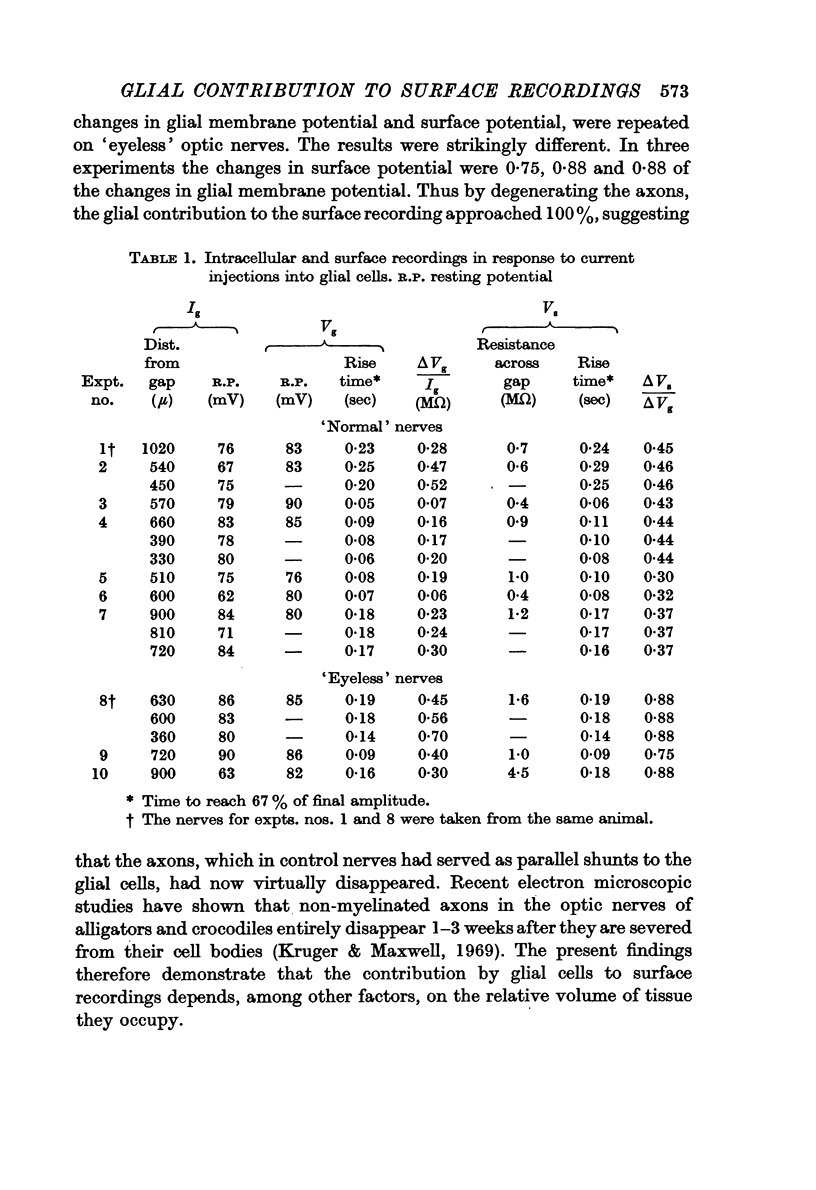
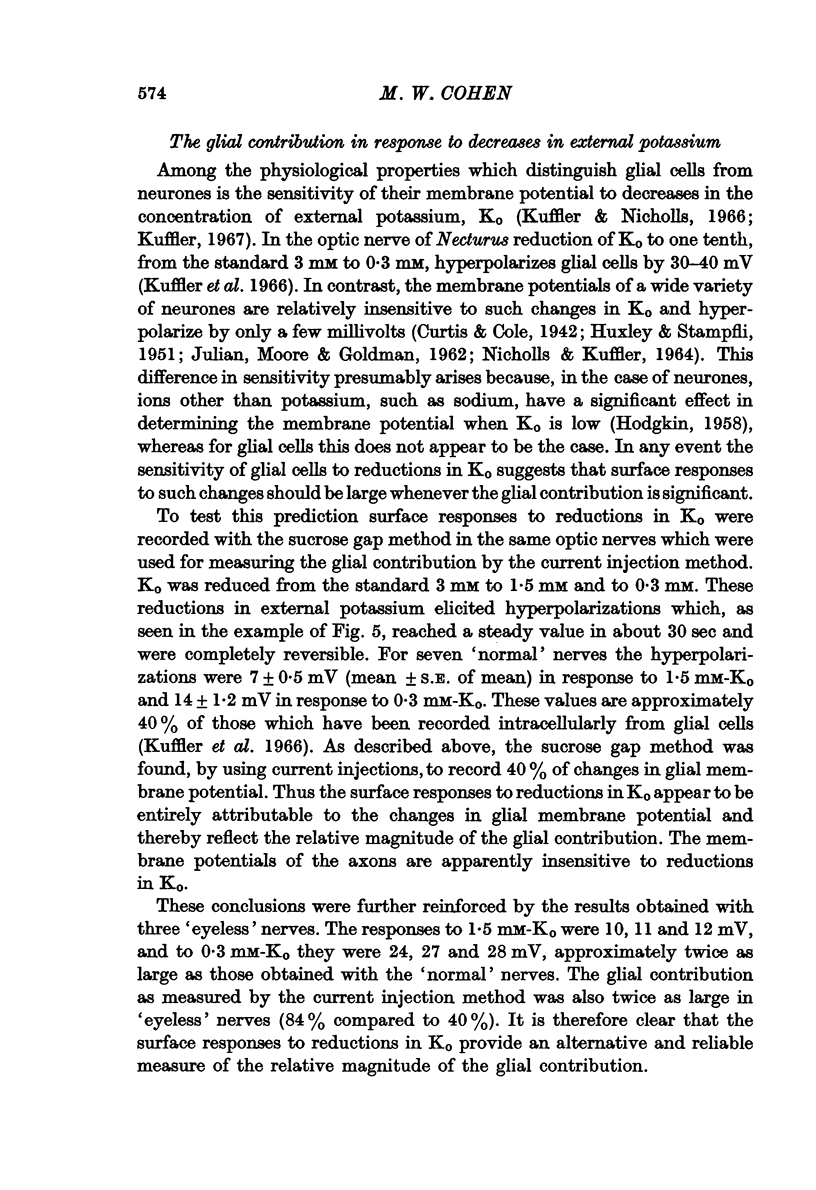
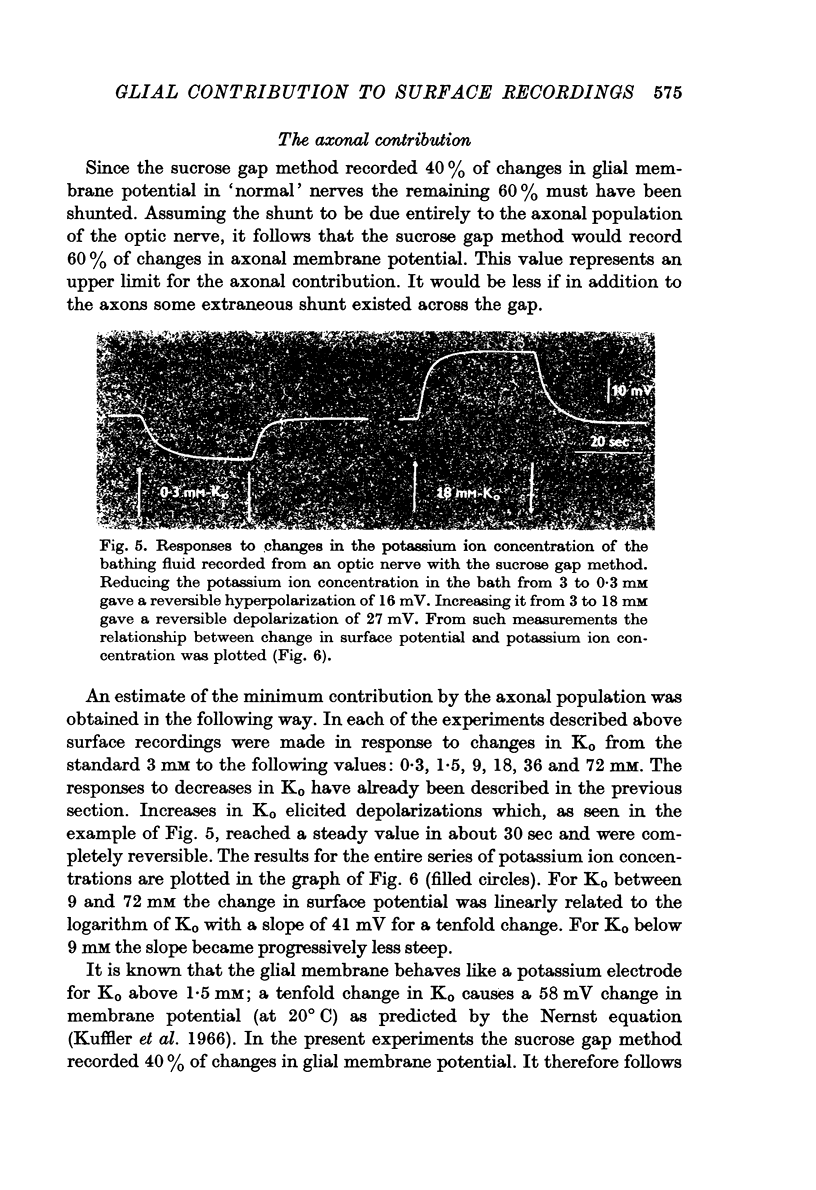
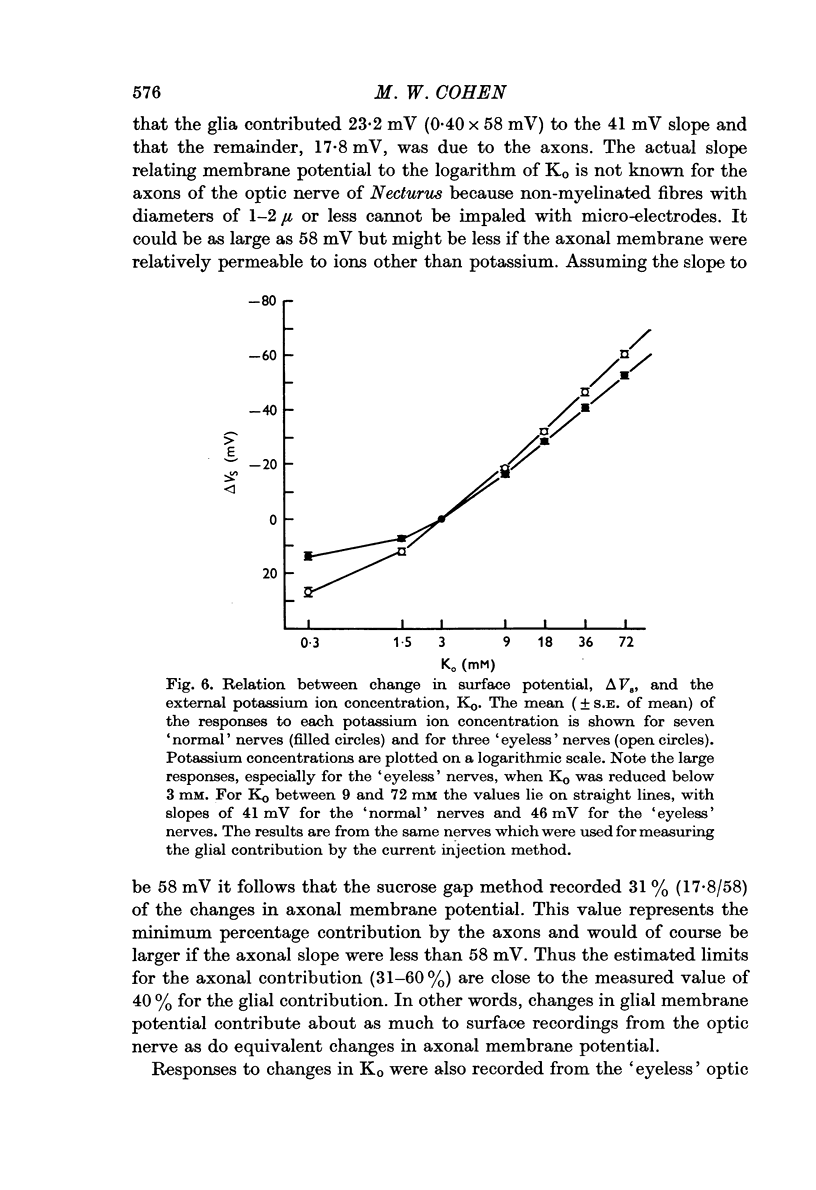
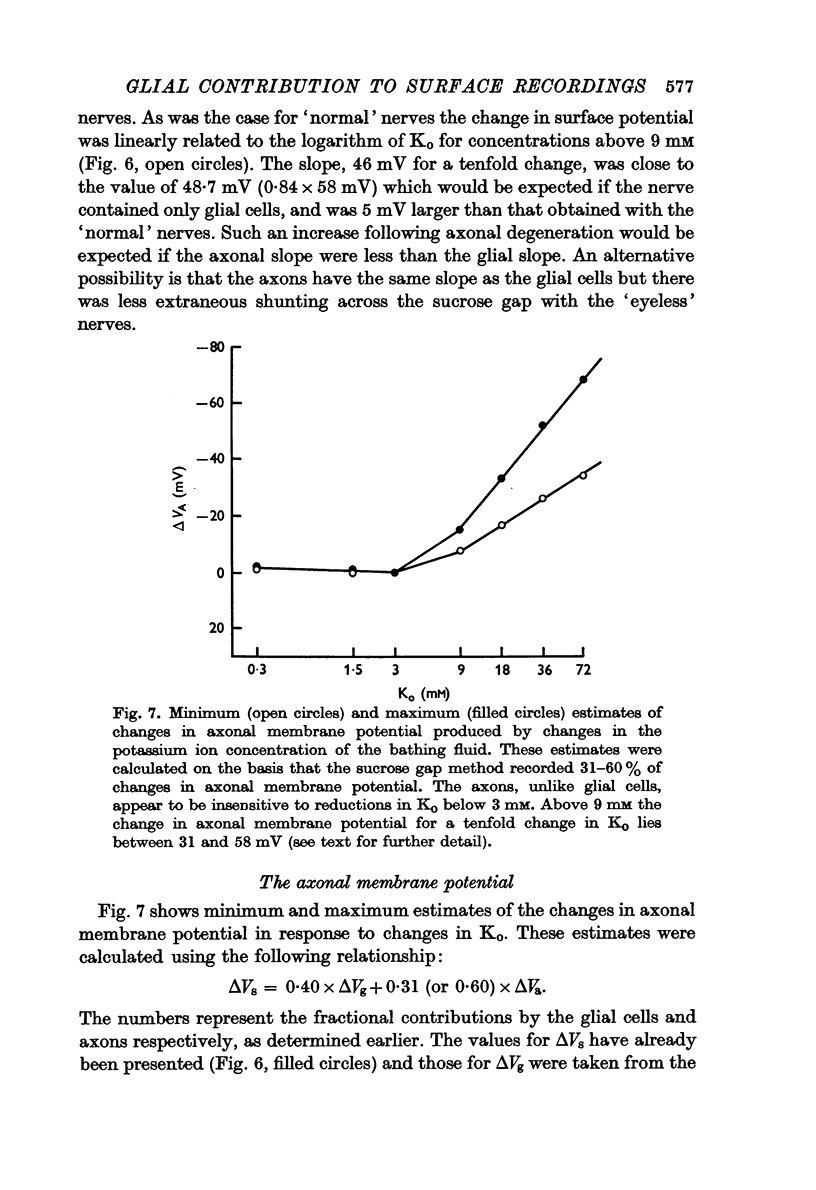
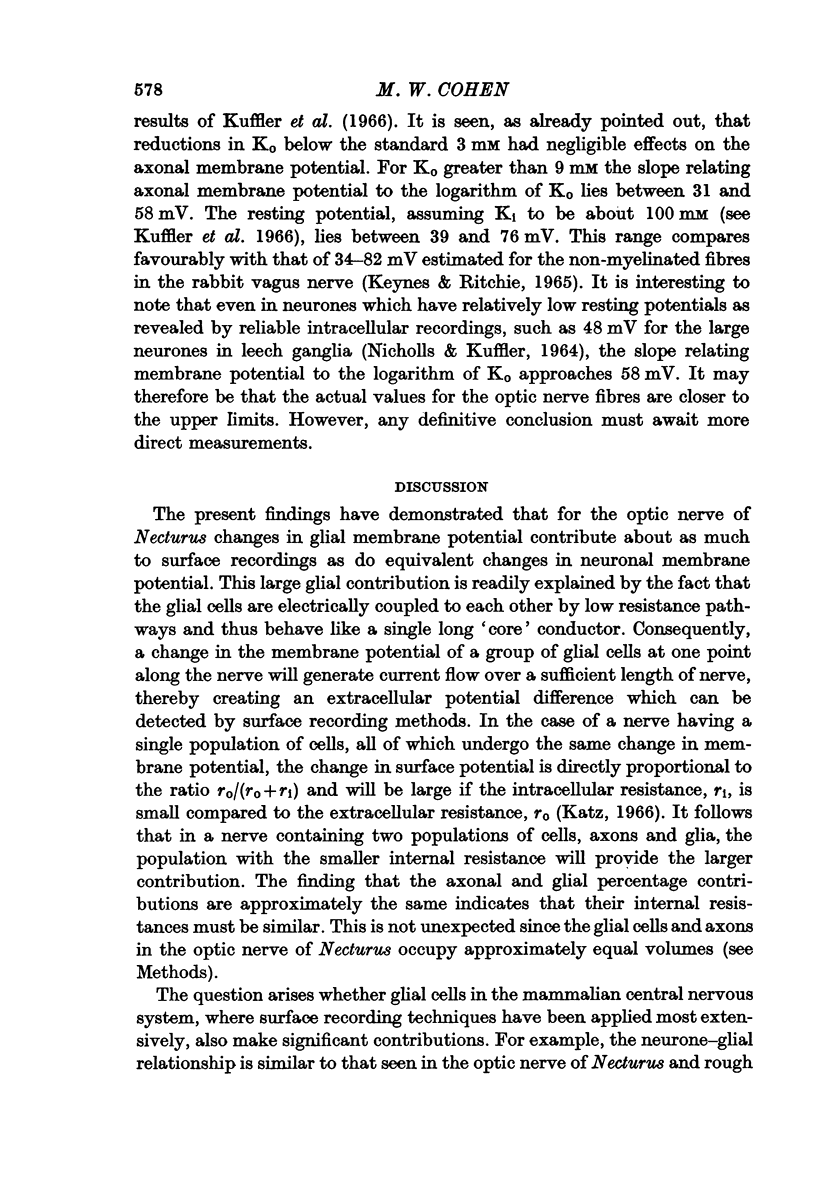
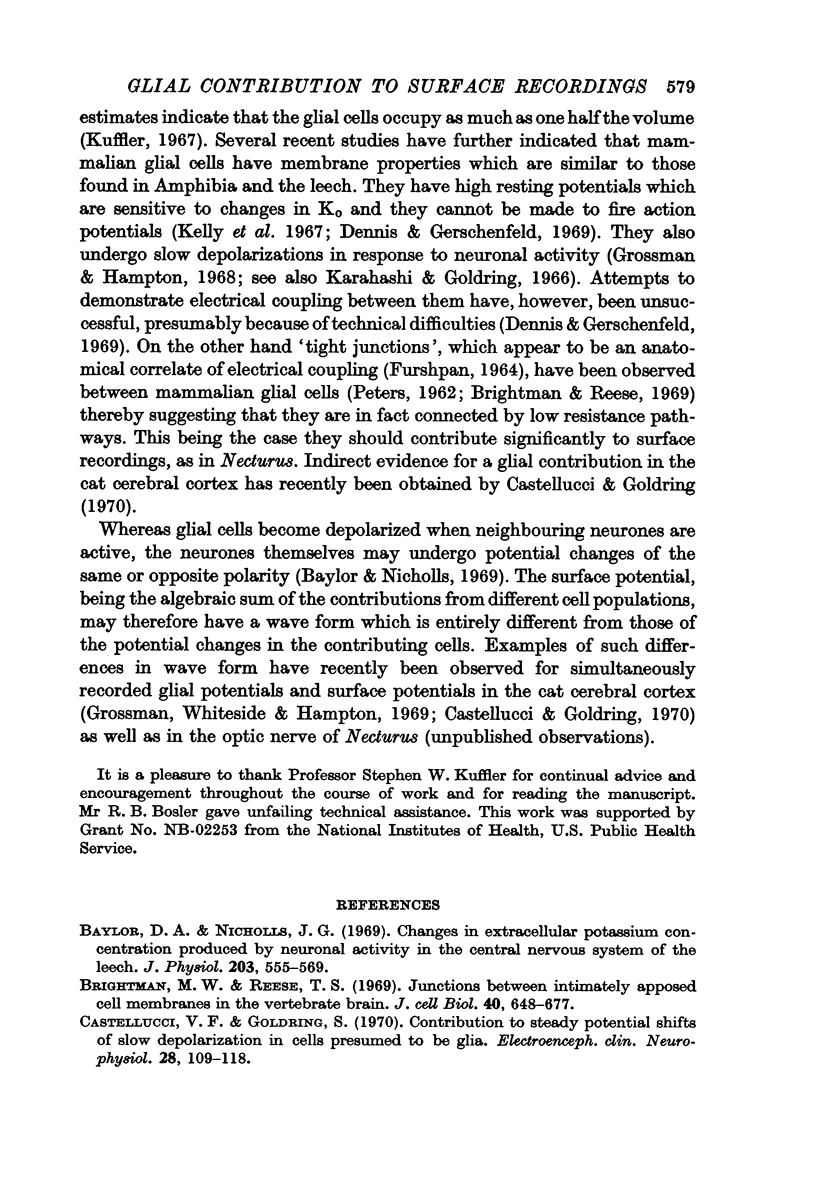
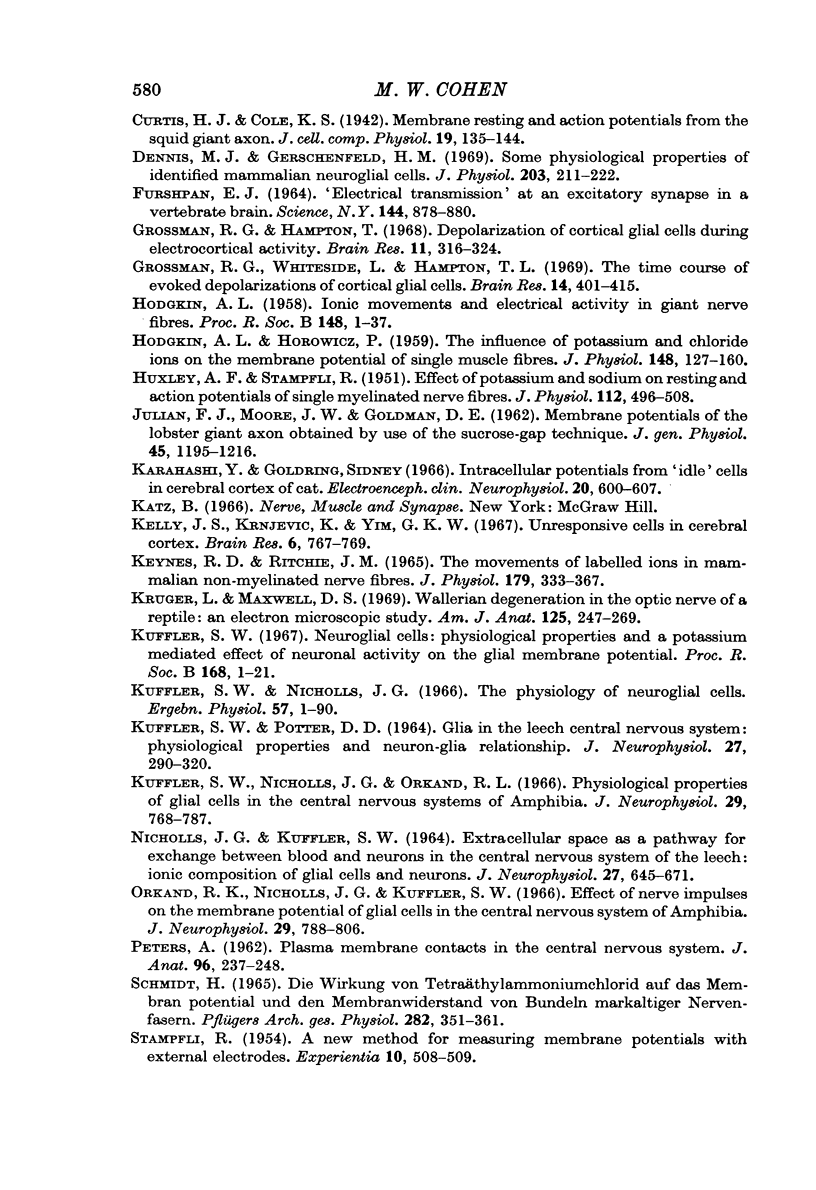
Images in this article
Selected References
These references are in PubMed. This may not be the complete list of references from this article.
- Baylor D. A., Nicholls J. G. Changes in extracellular potassium concentration produced by neuronal activity in the central nervous system of the leech. J Physiol. 1969 Aug;203(3):555–569. doi: 10.1113/jphysiol.1969.sp008879. [DOI] [PMC free article] [PubMed] [Google Scholar]
- Brightman M. W., Reese T. S. Junctions between intimately apposed cell membranes in the vertebrate brain. J Cell Biol. 1969 Mar;40(3):648–677. doi: 10.1083/jcb.40.3.648. [DOI] [PMC free article] [PubMed] [Google Scholar]
- Castellucci V. F., Goldring S. Contribution to steady potential shifts of slow depolarization in cells presumed to be glia. Electroencephalogr Clin Neurophysiol. 1970 Feb;28(2):109–118. doi: 10.1016/0013-4694(70)90178-1. [DOI] [PubMed] [Google Scholar]
- Dennis M. J., Gerschenfeld H. M. Some physiological properties of identified mammalian neuroglial cells. J Physiol. 1969 Jul;203(1):211–222. doi: 10.1113/jphysiol.1969.sp008860. [DOI] [PMC free article] [PubMed] [Google Scholar]
- FURSHPAN E. J. "ELECTRICAL TRANSMISSION" AT AN EXCITATORY SYNAPSE IN A VERTEBRATE BRAIN. Science. 1964 May 15;144(3620):878–880. doi: 10.1126/science.144.3620.878. [DOI] [PubMed] [Google Scholar]
- Grossman R. G., Hampton T. Depolarization of cortical glial cells during electrocortical activity. Brain Res. 1968 Nov;11(2):316–324. doi: 10.1016/0006-8993(68)90027-9. [DOI] [PubMed] [Google Scholar]
- Grossman R. G., Whiteside L., Hampton T. L. The time course of evoked depolarization of cortical glial cells. Brain Res. 1969 Jul;14(2):401–415. doi: 10.1016/0006-8993(69)90118-8. [DOI] [PubMed] [Google Scholar]
- HODGKIN A. L., HOROWICZ P. The influence of potassium and chloride ions on the membrane potential of single muscle fibres. J Physiol. 1959 Oct;148:127–160. doi: 10.1113/jphysiol.1959.sp006278. [DOI] [PMC free article] [PubMed] [Google Scholar]
- HODGKIN A. L. Ionic movements and electrical activity in giant nerve fibres. Proc R Soc Lond B Biol Sci. 1958 Jan 1;148(930):1–37. doi: 10.1098/rspb.1958.0001. [DOI] [PubMed] [Google Scholar]
- HUXLEY A. F., STAMPFLI R. Effect of potassium and sodium on resting and action potentials of single myelinated nerve fibers. J Physiol. 1951 Feb;112(3-4):496–508. doi: 10.1113/jphysiol.1951.sp004546. [DOI] [PMC free article] [PubMed] [Google Scholar]
- KUFFLER S. W., POTTER D. D. GLIA IN THE LEECH CENTRAL NERVOUS SYSTEM: PHYSIOLOGICAL PROPERTIES AND NEURON-GLIA RELATIONSHIP. J Neurophysiol. 1964 Mar;27:290–320. doi: 10.1152/jn.1964.27.2.290. [DOI] [PubMed] [Google Scholar]
- Karahashi Y., Goldring S. Intracellular potentials from "idle" cells in cerebral cortex of cat. Electroencephalogr Clin Neurophysiol. 1966 Jun;20(6):600–607. doi: 10.1016/0013-4694(66)90024-1. [DOI] [PubMed] [Google Scholar]
- Kelly J. S., Krnjević K., Yim G. K. Unresponsive cells in cerebral cortex. Brain Res. 1967 Dec;6(4):767–769. doi: 10.1016/0006-8993(67)90132-1. [DOI] [PubMed] [Google Scholar]
- Keynes R. D., Ritchie J. M. The movements of labelled ions in mammalian non-myelinated nerve fibres. J Physiol. 1965 Jul;179(2):333–367. doi: 10.1113/jphysiol.1965.sp007666. [DOI] [PMC free article] [PubMed] [Google Scholar]
- Kruger L., Maxwell D. S. Wallerian degeneration in the optic nerve of a reptile: an electron microscopic study. Am J Anat. 1969 Jul;125(3):247–269. doi: 10.1002/aja.1001250302. [DOI] [PubMed] [Google Scholar]
- Kuffler S. W., Nicholls J. G., Orkand R. K. Physiological properties of glial cells in the central nervous system of amphibia. J Neurophysiol. 1966 Jul;29(4):768–787. doi: 10.1152/jn.1966.29.4.768. [DOI] [PubMed] [Google Scholar]
- Kuffler S. W., Nicholls J. G. The physiology of neuroglial cells. Ergeb Physiol. 1966;57:1–90. [PubMed] [Google Scholar]
- NICHOLLS J. G., KUFFLER S. W. EXTRACELLULAR SPACE AS A PATHWAY FOR EXCHANGE BETWEEN BLOOD AND NEURONS IN THE CENTRAL NERVOUS SYSTEM OF THE LEECH: IONIC COMPOSITION OF GLIAL CELLS AND NEURONS. J Neurophysiol. 1964 Jul;27:645–671. doi: 10.1152/jn.1964.27.4.645. [DOI] [PubMed] [Google Scholar]
- Orkand R. K., Nicholls J. G., Kuffler S. W. Effect of nerve impulses on the membrane potential of glial cells in the central nervous system of amphibia. J Neurophysiol. 1966 Jul;29(4):788–806. doi: 10.1152/jn.1966.29.4.788. [DOI] [PubMed] [Google Scholar]
- PETERS A. Plasma membrane contacts in the central nervous system. J Anat. 1962 Apr;96:237–248. [PMC free article] [PubMed] [Google Scholar]
- SCHMIDT H. DIE WIRKUNG VON TETRAAETHYLAMMONIUMCHLORID AUF DAS MEMBRANPOTENTIAL UND DEN MEMBRANWIDERSTAND VON BUENDELN MARKHALTIGER NERVENFASERN. Pflugers Arch Gesamte Physiol Menschen Tiere. 1965 Jan 11;282:351–361. [PubMed] [Google Scholar]
- STAMPFLI R. A new method for measuring membrane potentials with external electrodes. Experientia. 1954 Dec 15;10(12):508–509. doi: 10.1007/BF02166189. [DOI] [PubMed] [Google Scholar]