Abstract
1. The effects of tetraethylammonium (TEA) and zinc ions on the resting conductance of frog muscle were examined using a method which permitted control of the membrane potential near the end of a muscle fibre and measurement of an approximation of the membrane current.
2. TEA reduced the amplitude of the inactivating inward current obtained on hyperpolarizing, both when this was measured as initial current [Im(0)] less the estimated chloride current and as [Im(0) — Im(∞)]. 115 mM-TEA reduced the amplitude of [Im(0) — Im(∞)] by about 85%.
3. TEA had little effect on the time constant with which the inward potassium currents inactivated. This finding appeared to be in conflict with the view that the inactivation is due to depletion of potassium from the T-system, though the results in standard Ringer were in good agreement with such an hypothesis.
4. In the standard chloride Ringer, the resting membrane resistance was 3530 Ω cm2. 115 mM-TEA increased this 1·6 times to 5580 Ω cm2.
5. The effect of TEA on voltage—current relations obtained in high K2SO4 solutions was also examined. With large pulses, the voltage—current relations in TEA and control solutions tended to become linear. This linear part could be extrapolated and subtracted from the voltage—current relation.
6. TEA, at a concentration of 150 mM, reversibly reduced by 88% the amplitude of the currents obtained during both depolarizing and hyperpolarizing pulses; this figure was obtained after subtraction of the linear part of the relation, which was itself unaffected by TEA and which represented a resistance of 5600 Ω cm2.
7. The reductions in the inactivating potassium current and in the current flowing through the inwardly rectifying potassium channel in high K2SO4 solutions fit, fairly closely, a concentration—effect relation for TEA with a dissociation constant of 20 × 10-3 M.
8. Also investigated were the slow time-dependent changes in membrane potential occurring when [K]o is altered from 2·5 to 10 mM at constant chloride (120 mM). The findings were consistent with the view that TEA reduced the potassium conductance without much effect on the chloride conductance. In particular, when muscles equilibrated for 1 hr in 10 mM-K Ringer were returned to 2·5 mM-K, the initial rate of repolarization was reduced, even though the driving force on K was slightly larger than in the control experiment.
9. Zinc ions appeared to be without effect either on the amplitude of the inactivating potassium current obtained when square hyperpolarizing pulses were applied to the fibre membrane, or on its time constant.
10. In standard Tris-buffered Ringer, the membrane resistance was 3350 Ω cm2. Zinc Ringer (2·5 mM) increased this rather more than twice to 6830 Ω cm2.
11. It is concluded that the two agents, TEA+ and Zn2+, which both reduce delayed potassium currents in muscle, act in different ways on the resting conductance of frog muscle.
Full text
PDF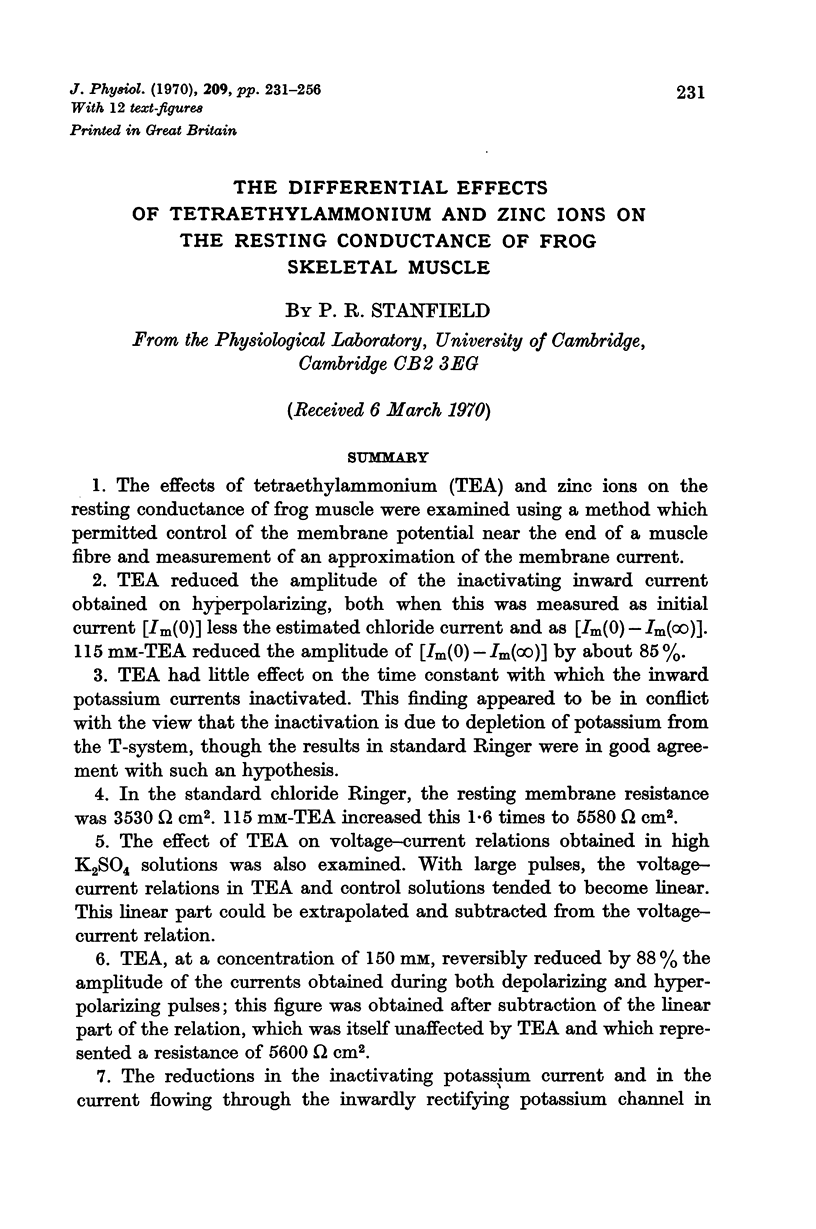
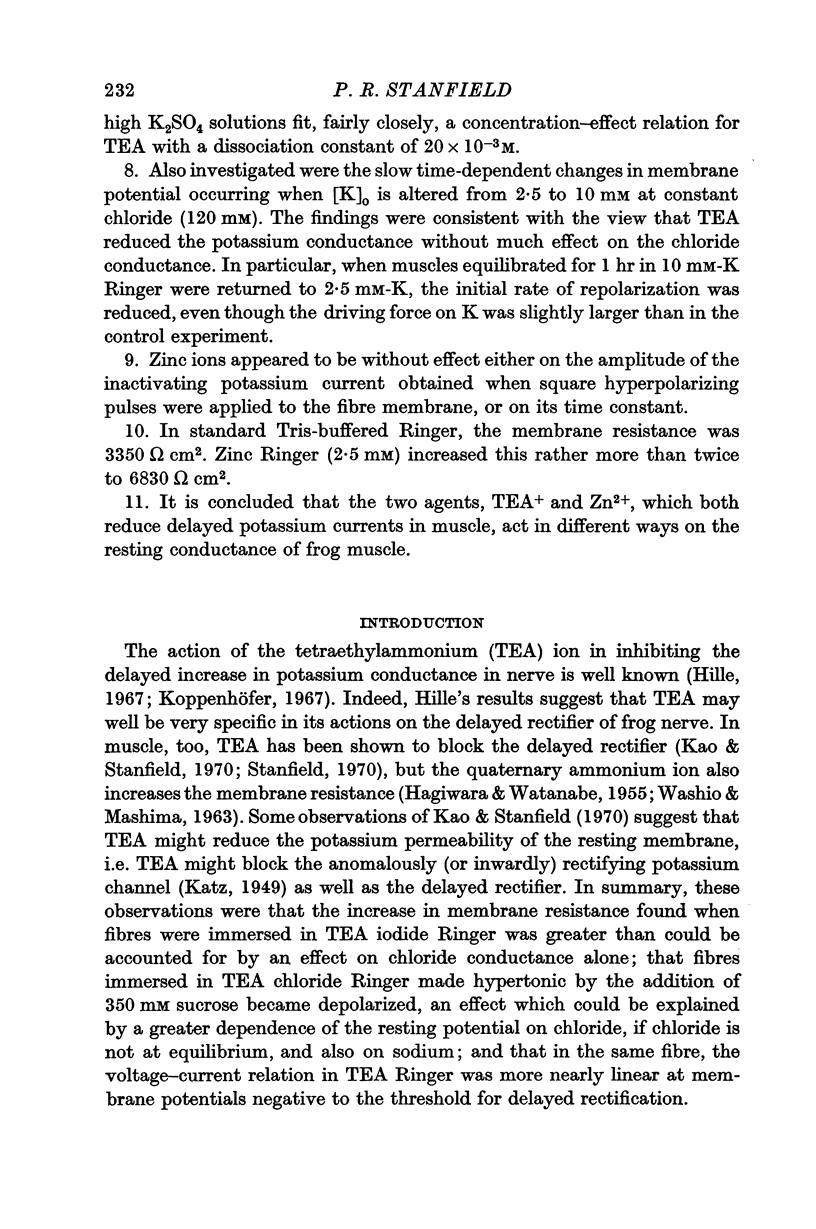
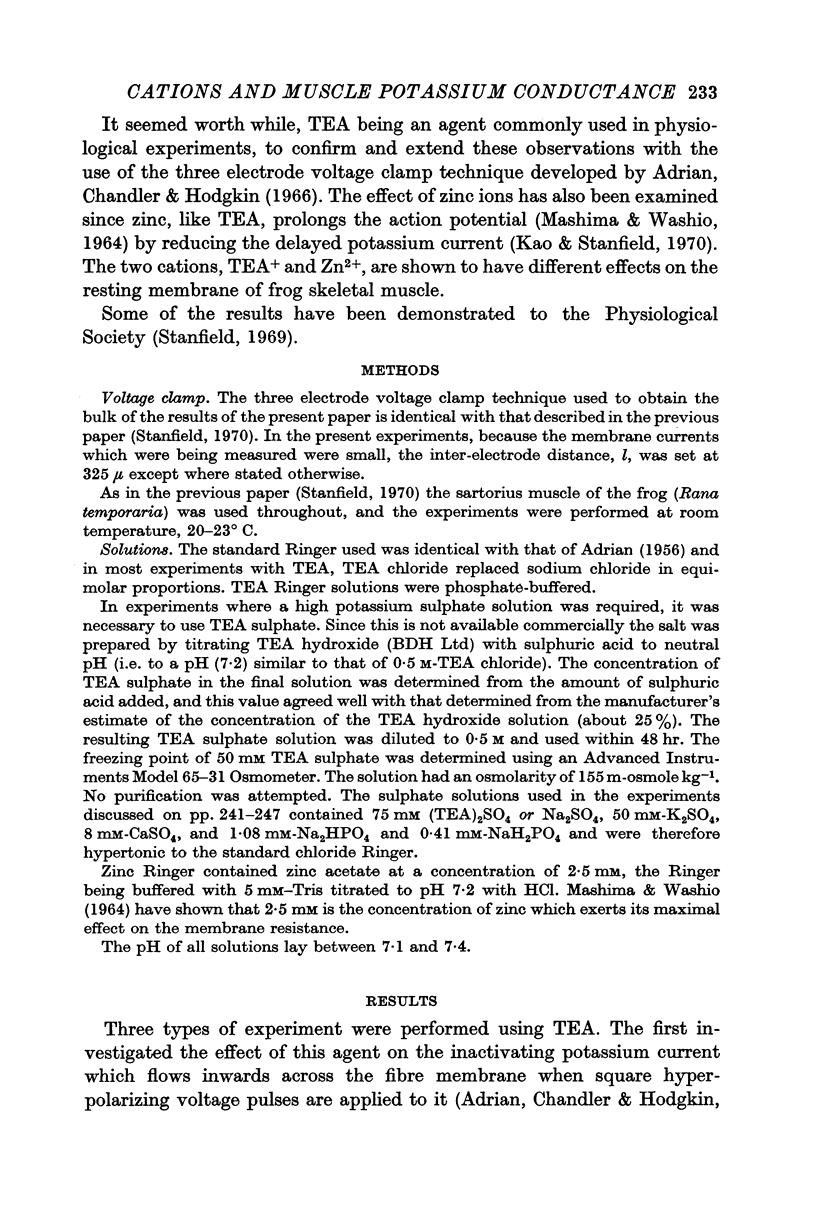
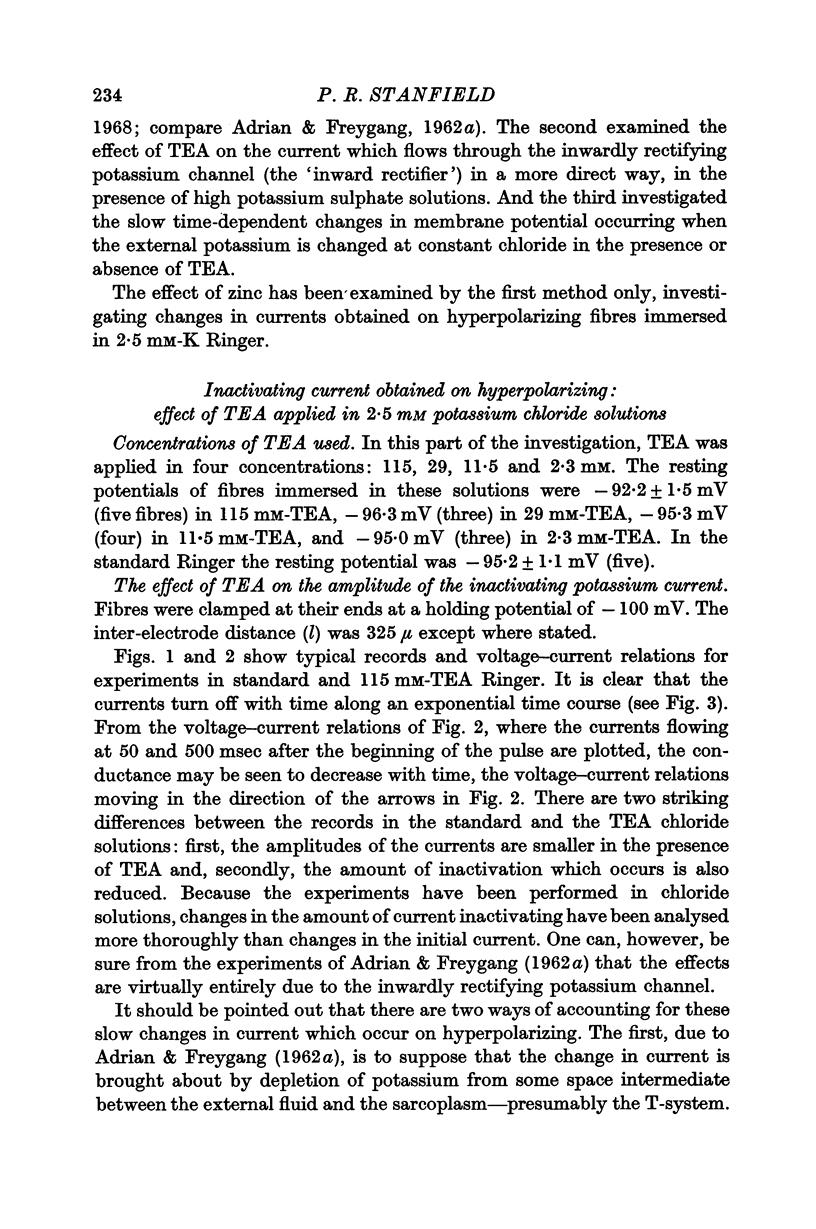
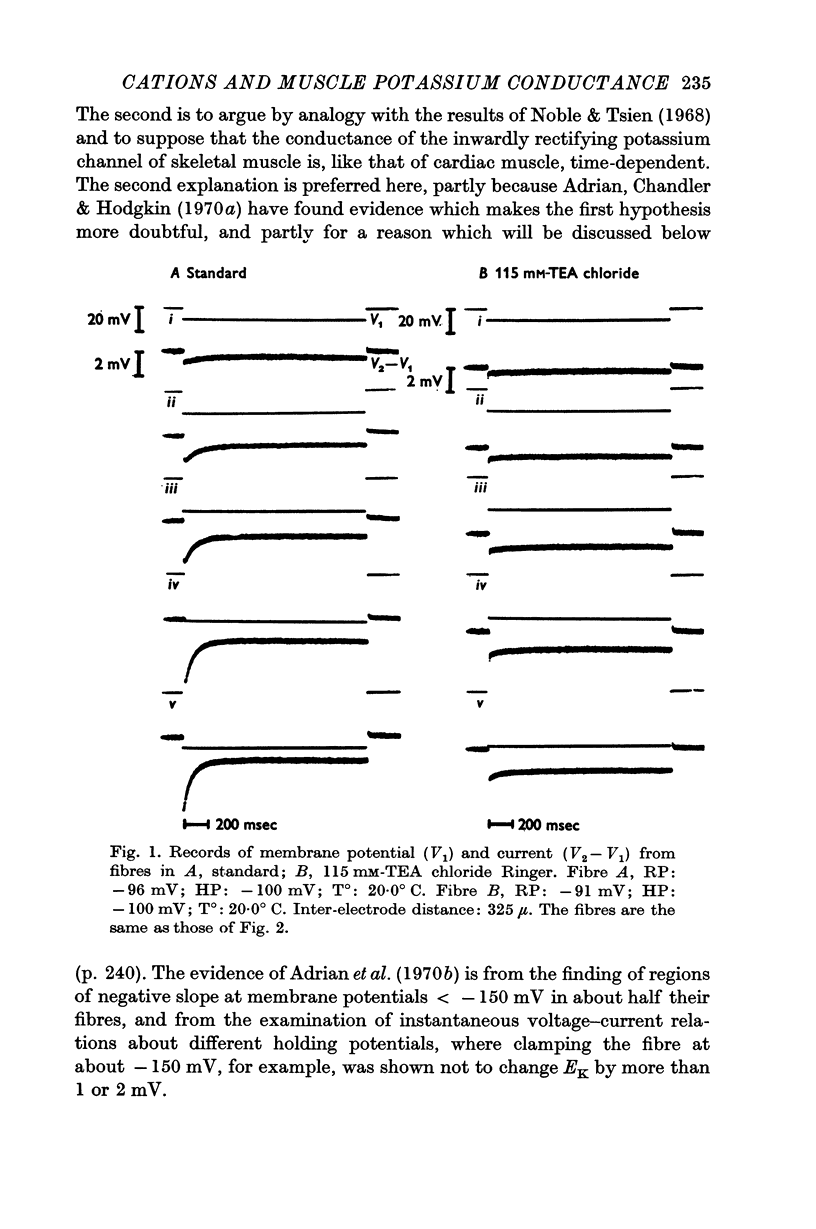
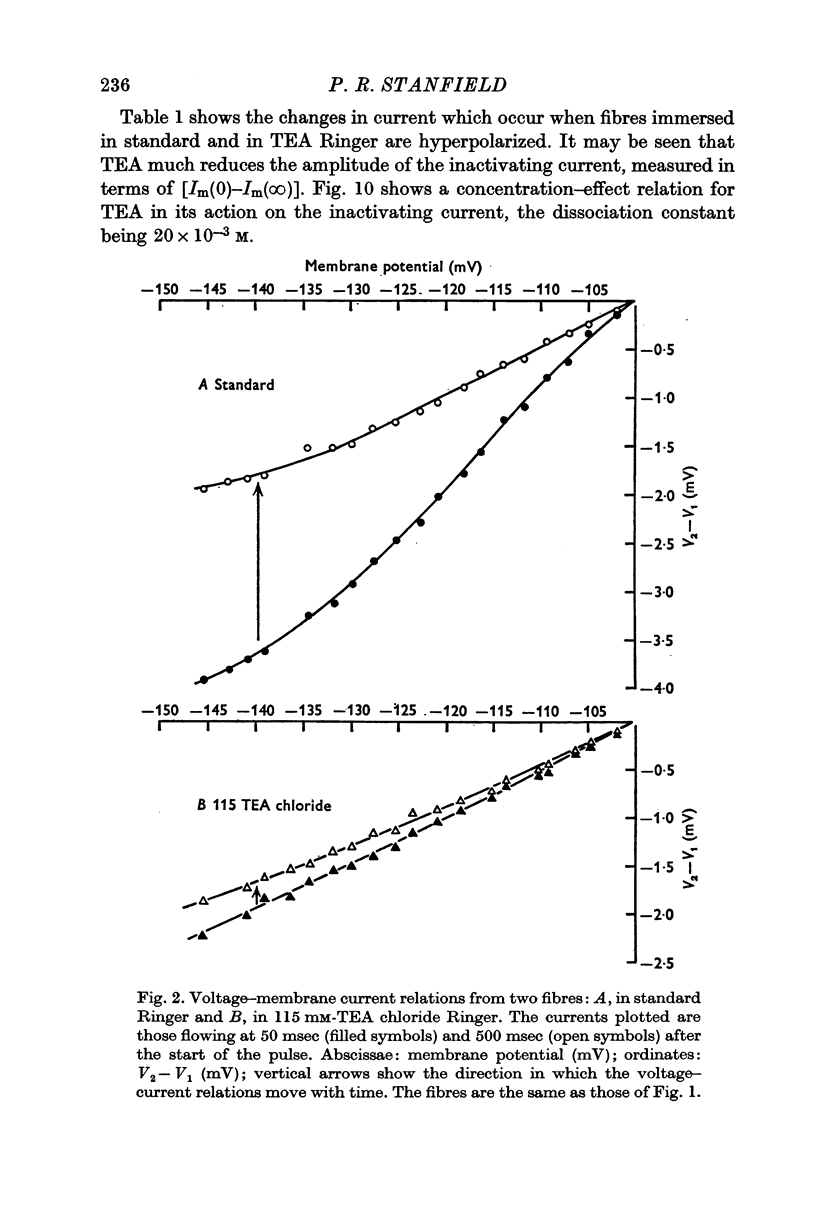
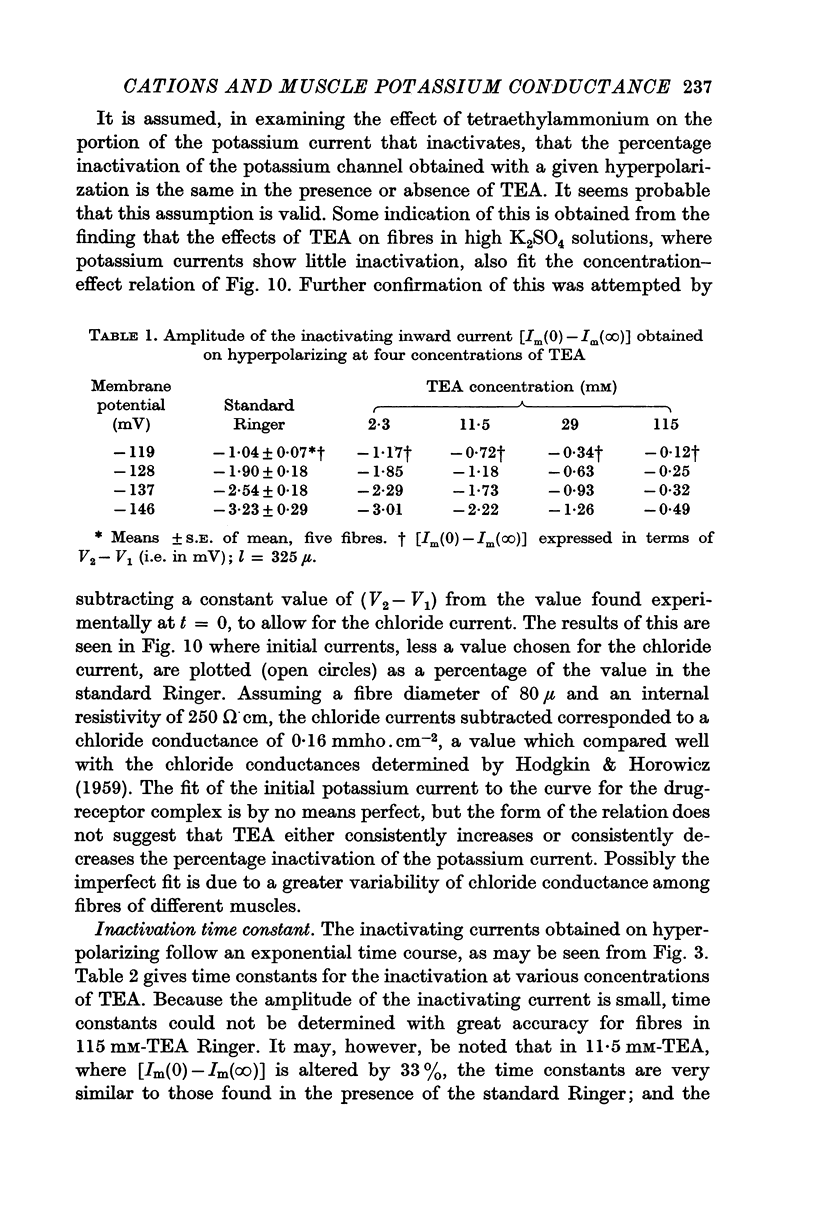
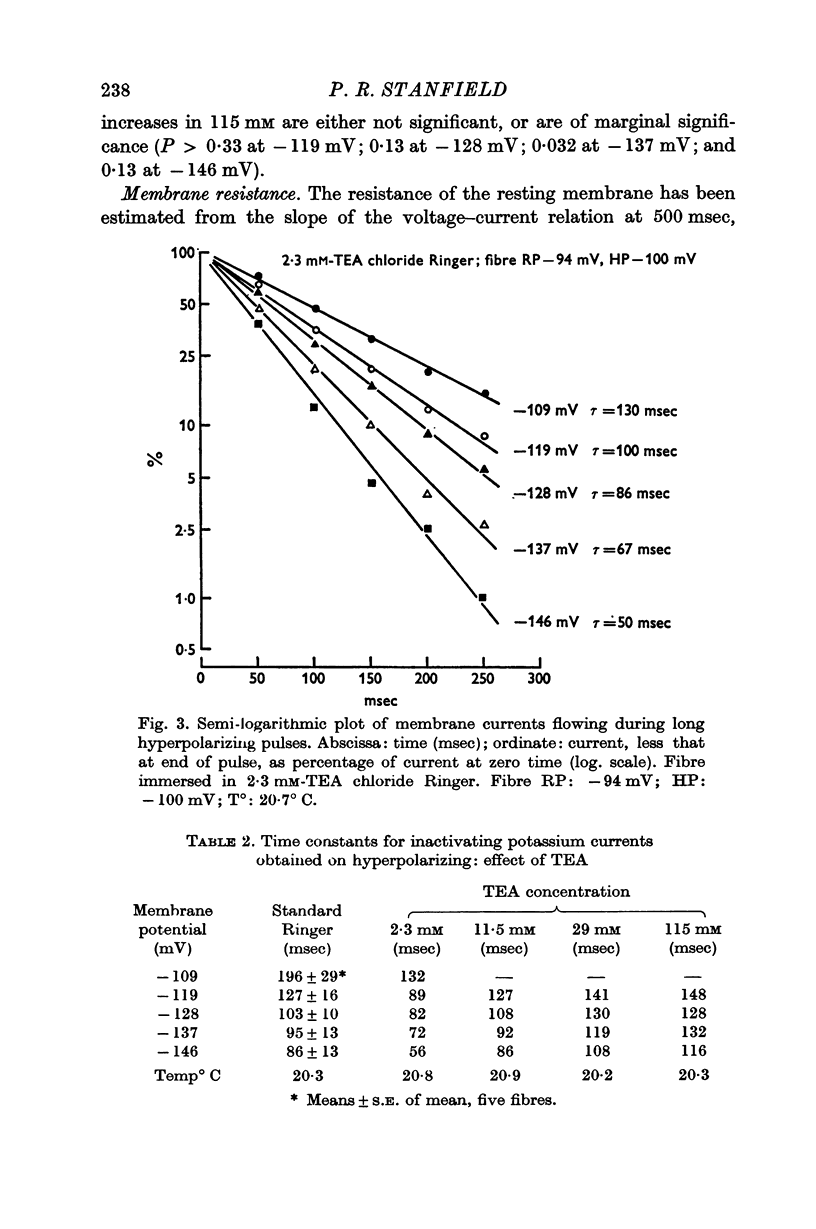
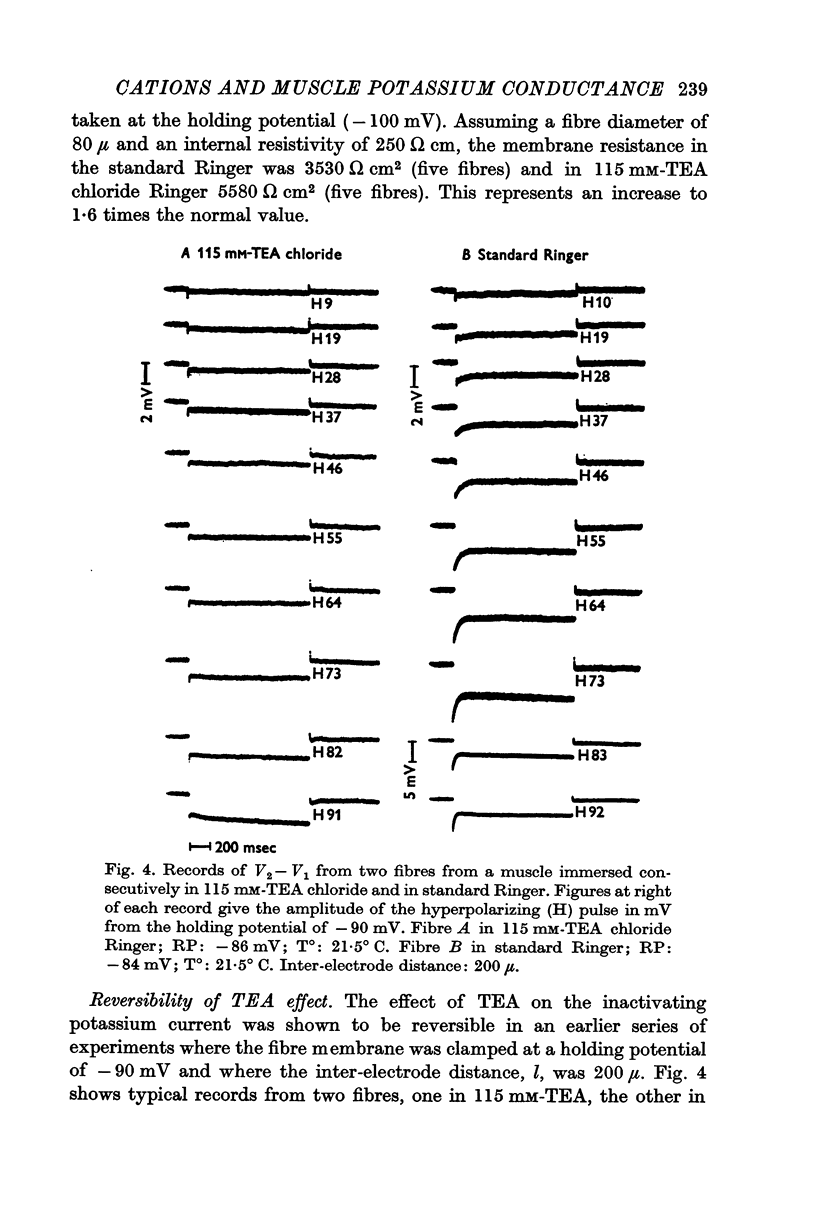
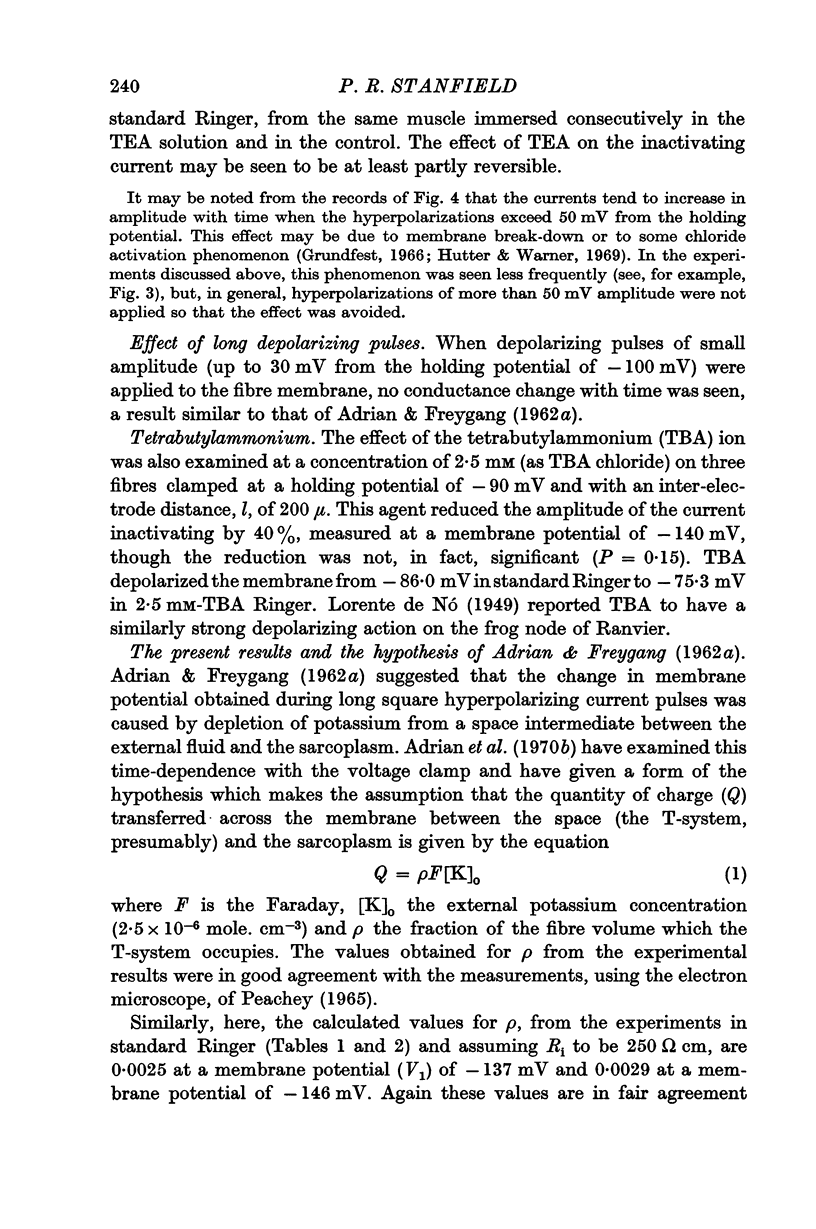
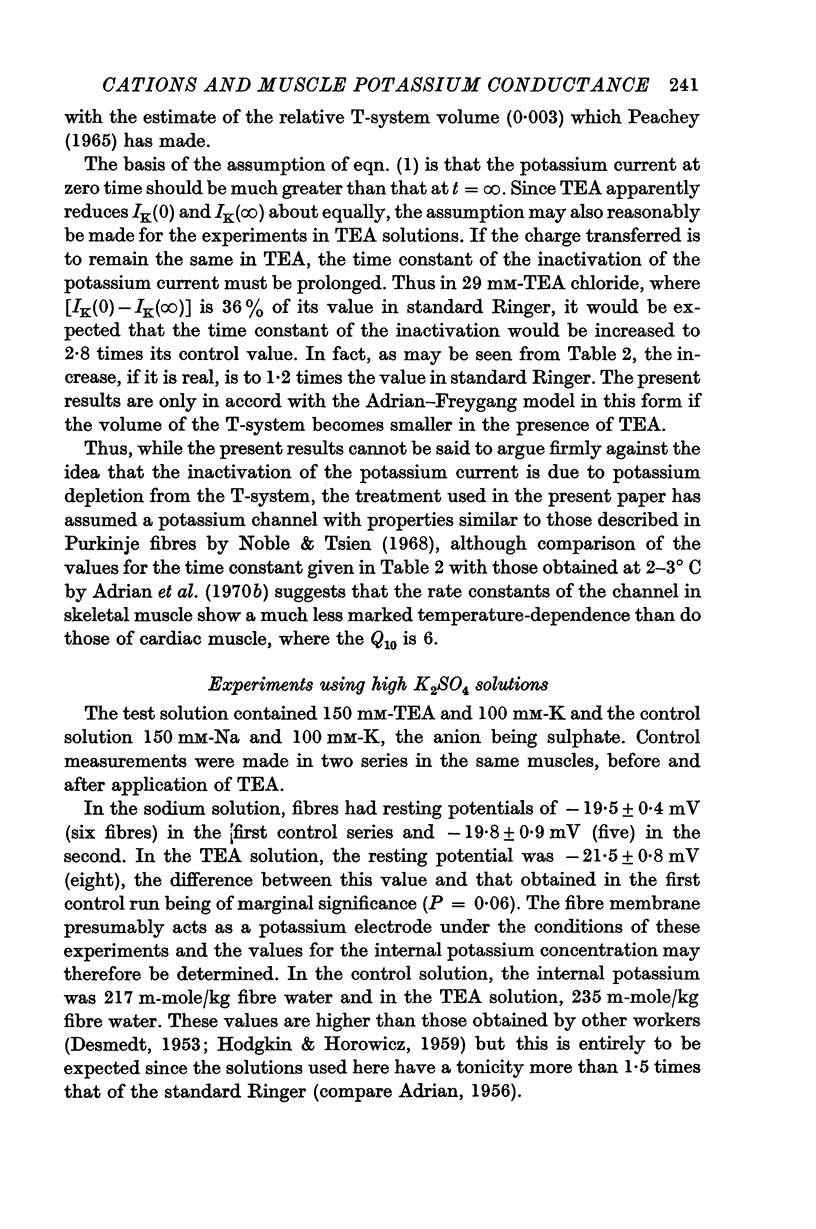
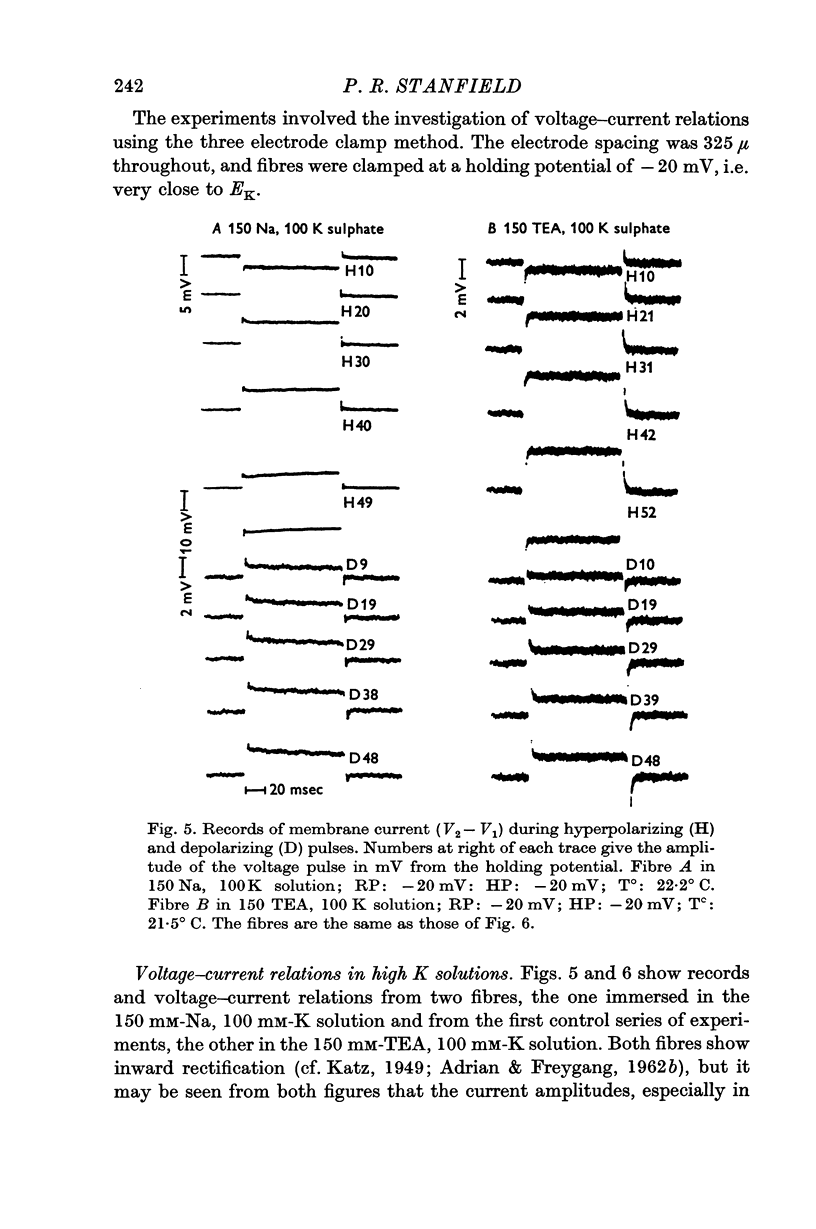
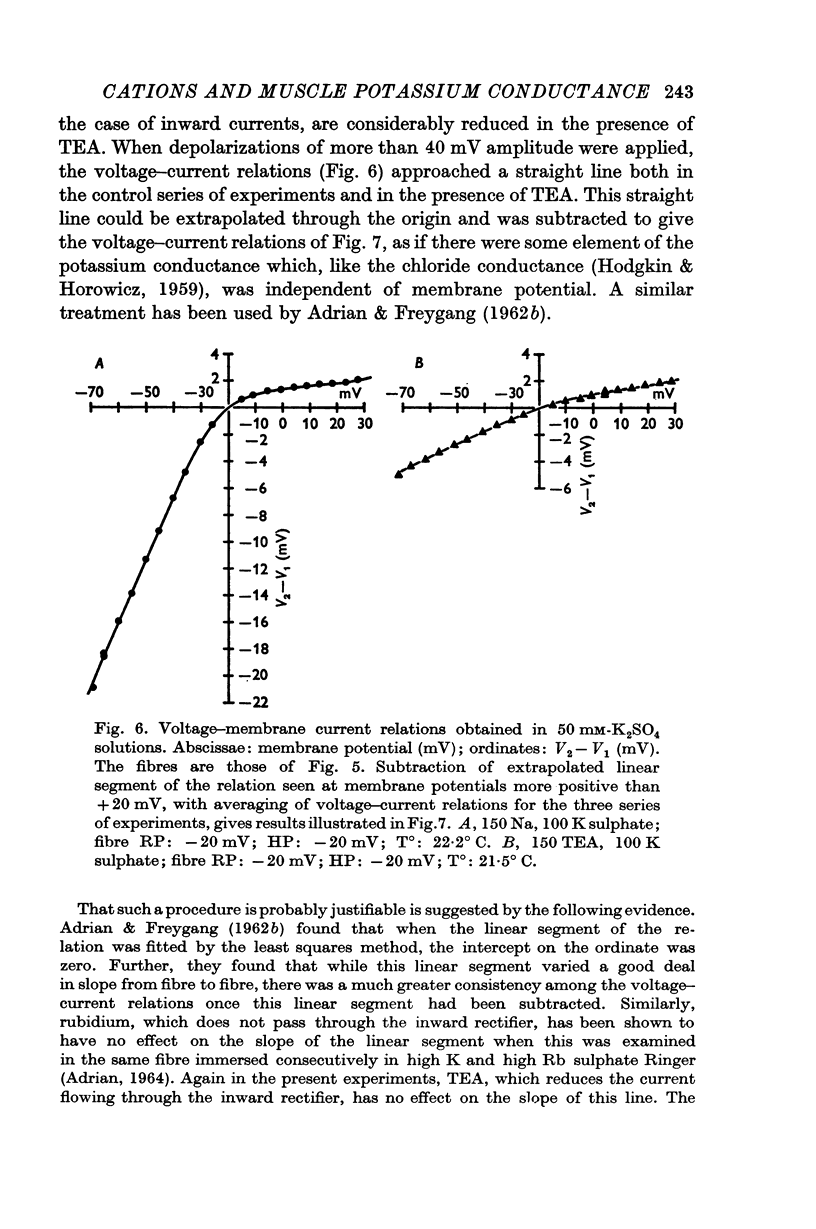
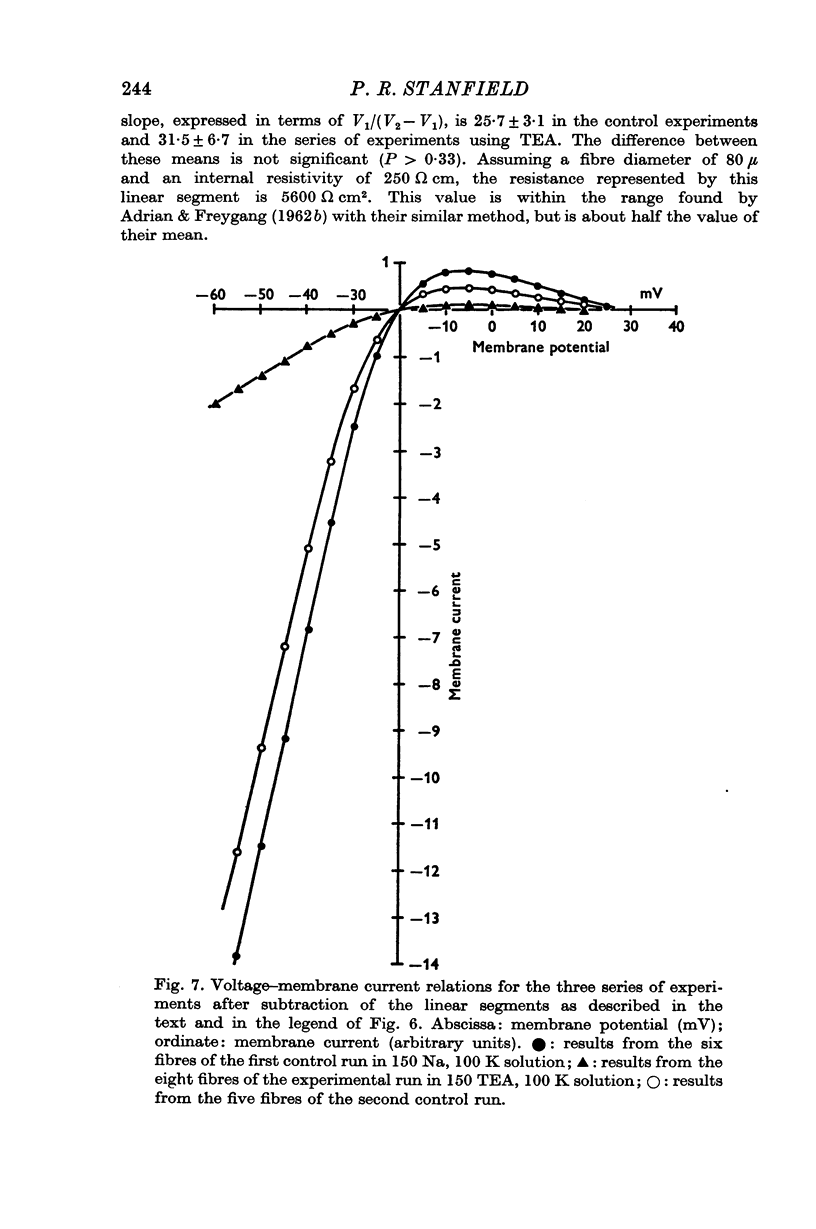
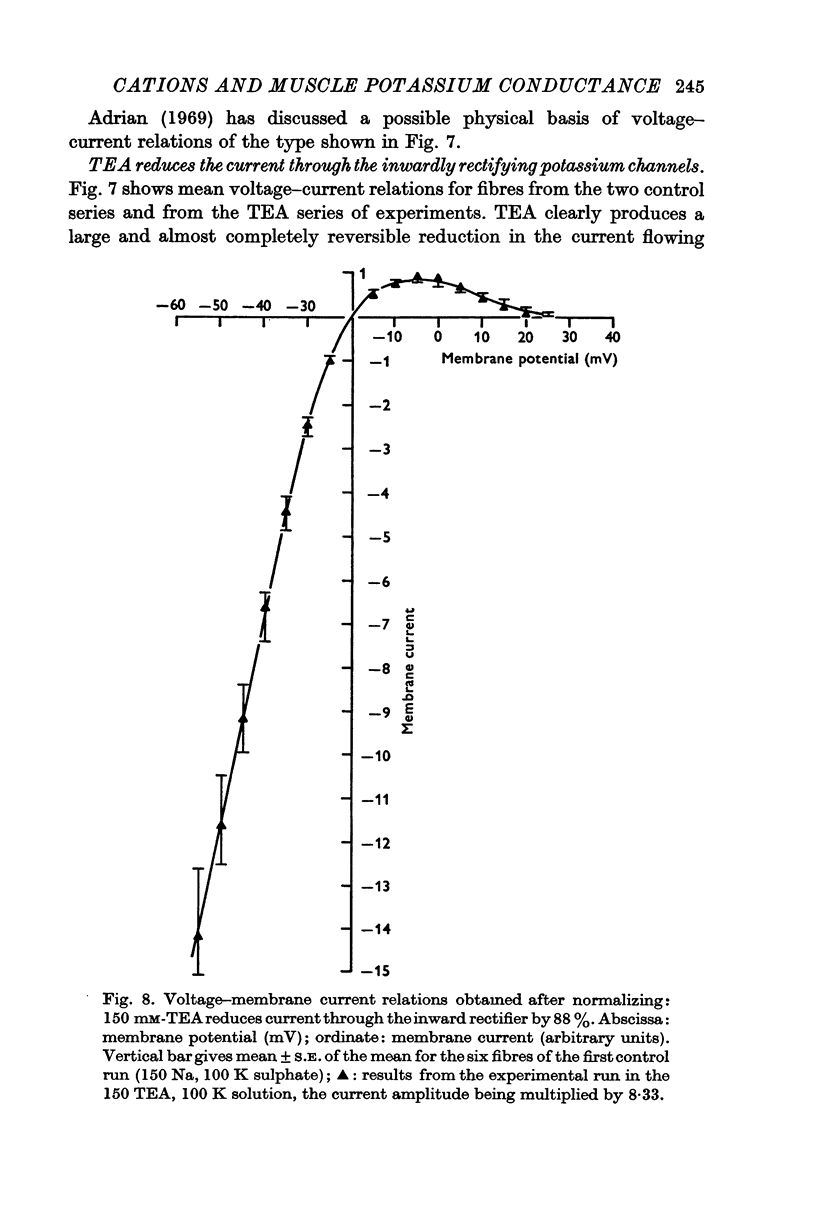
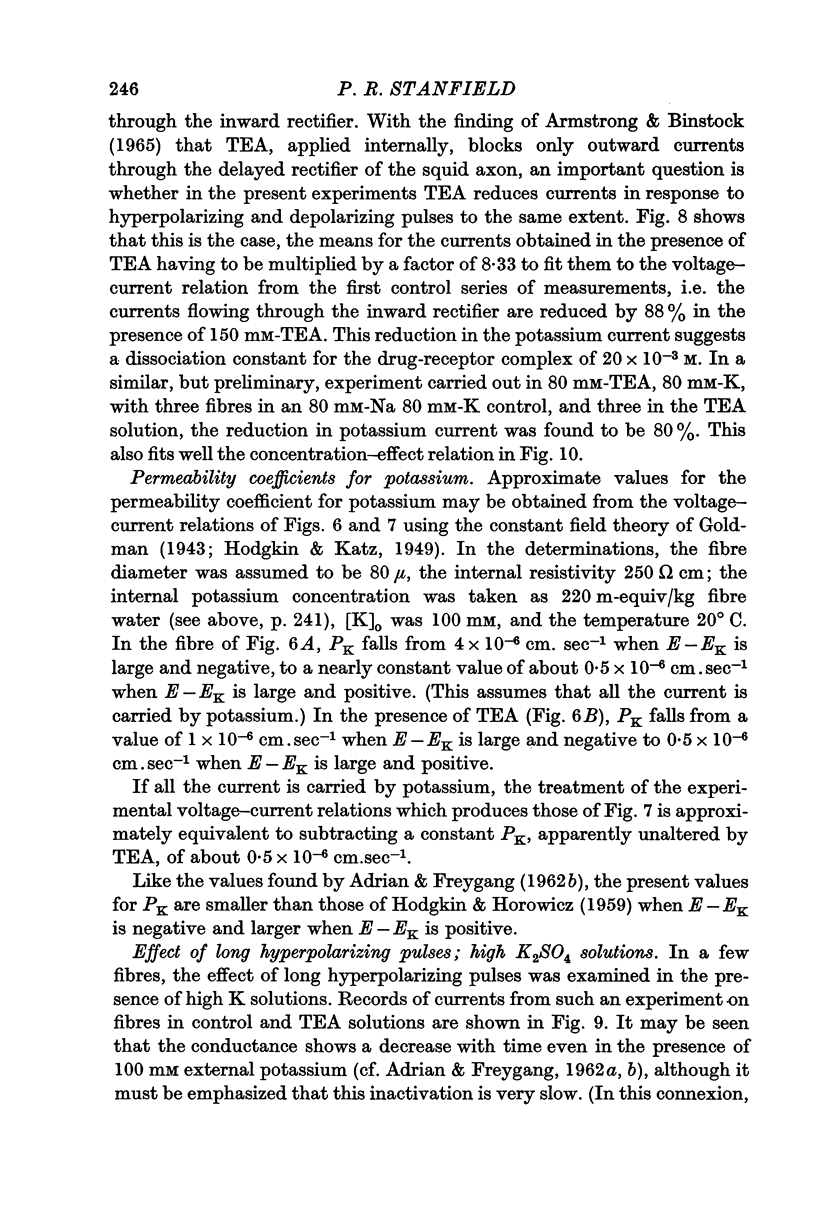
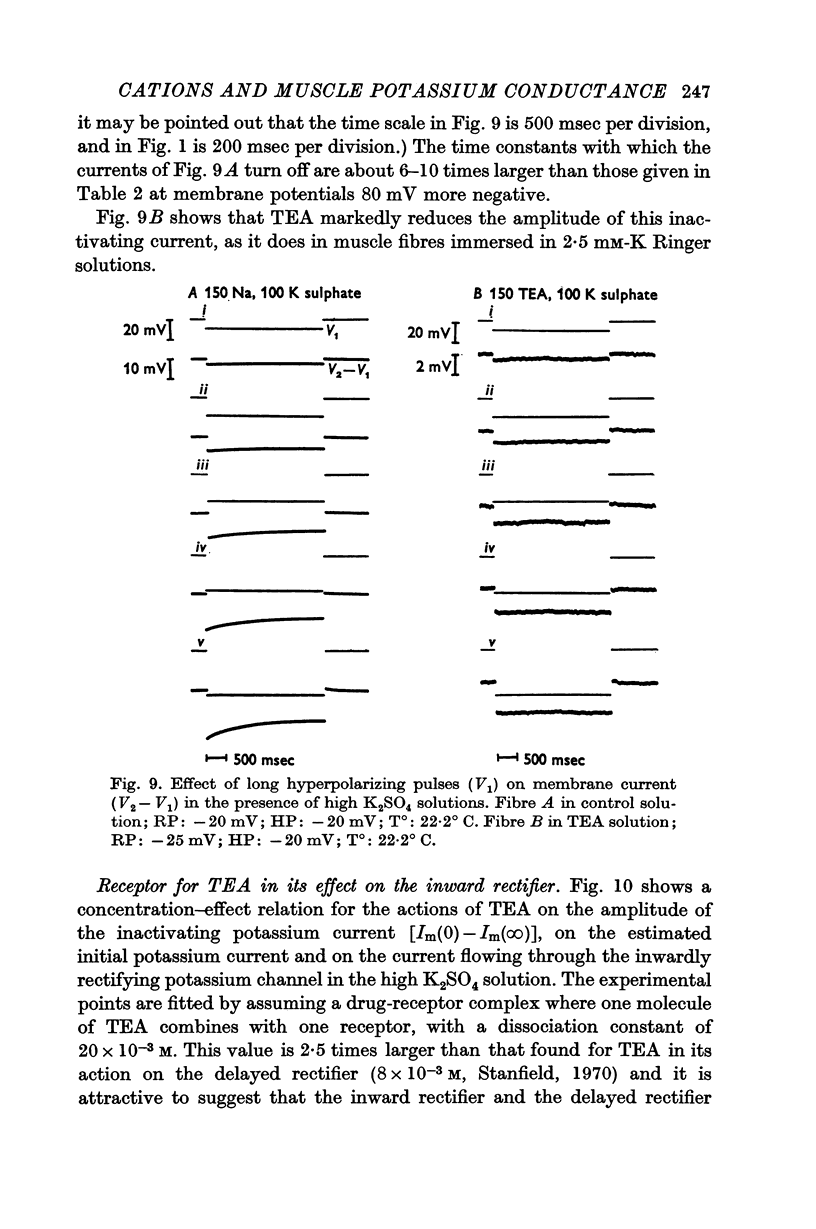
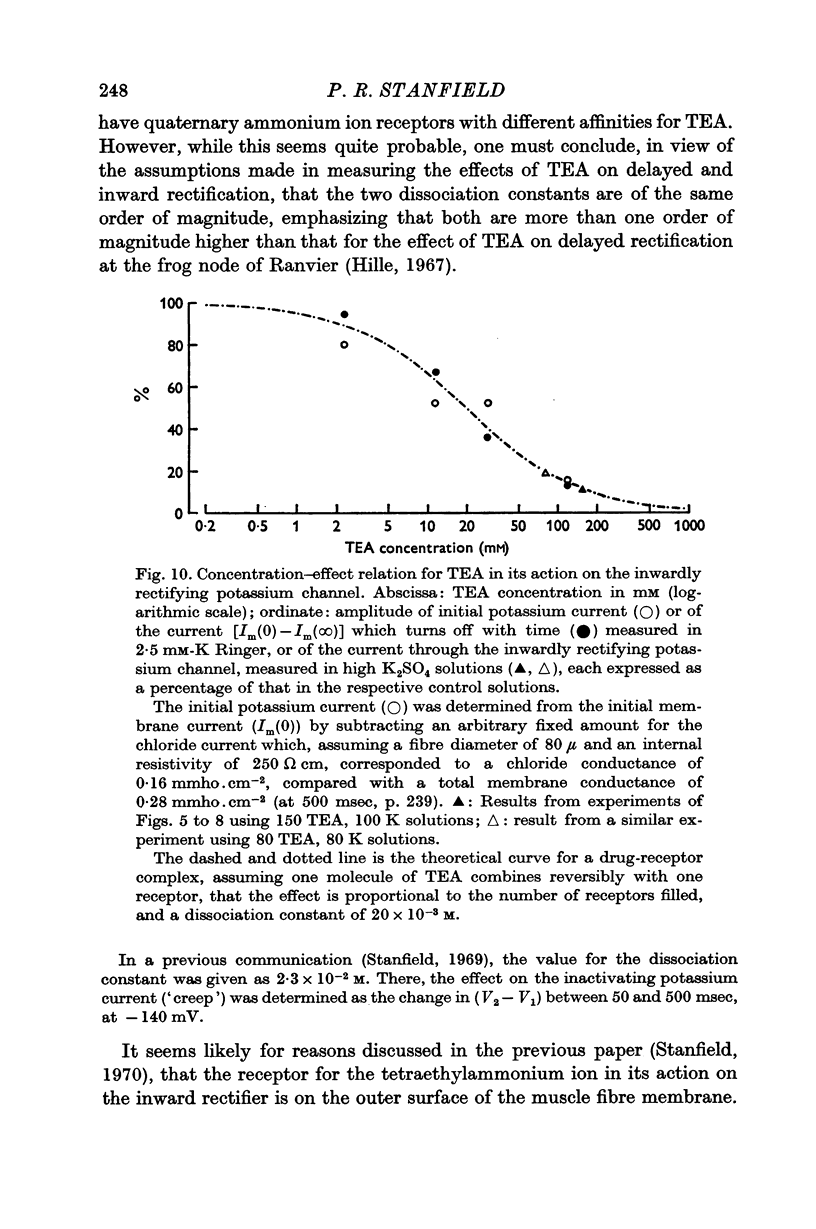
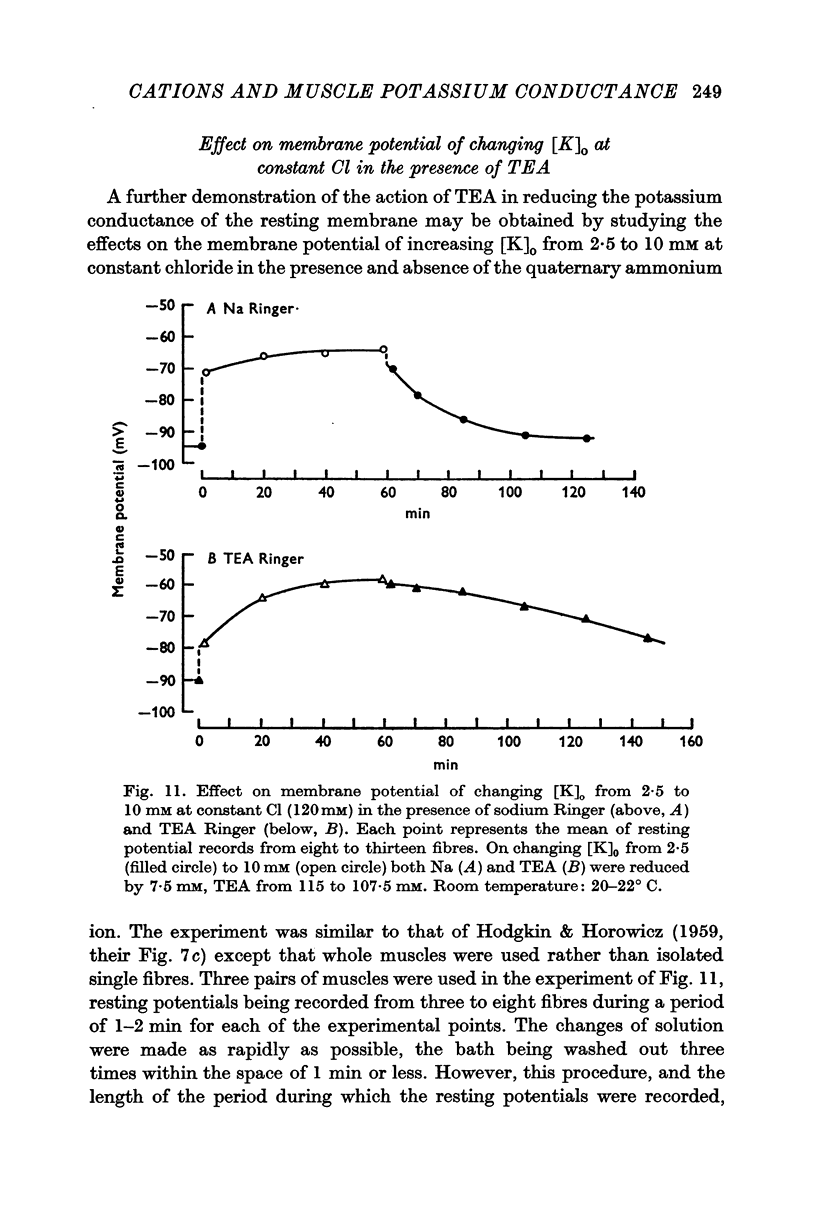
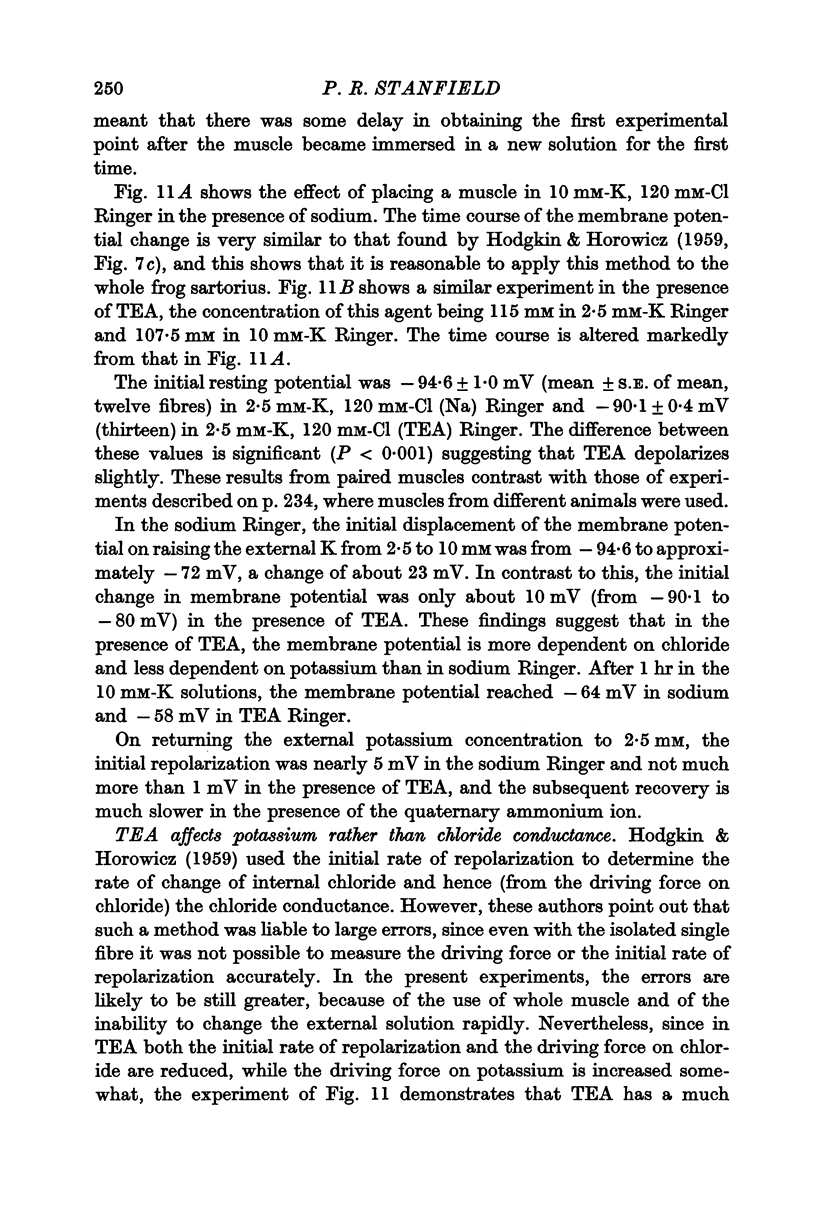
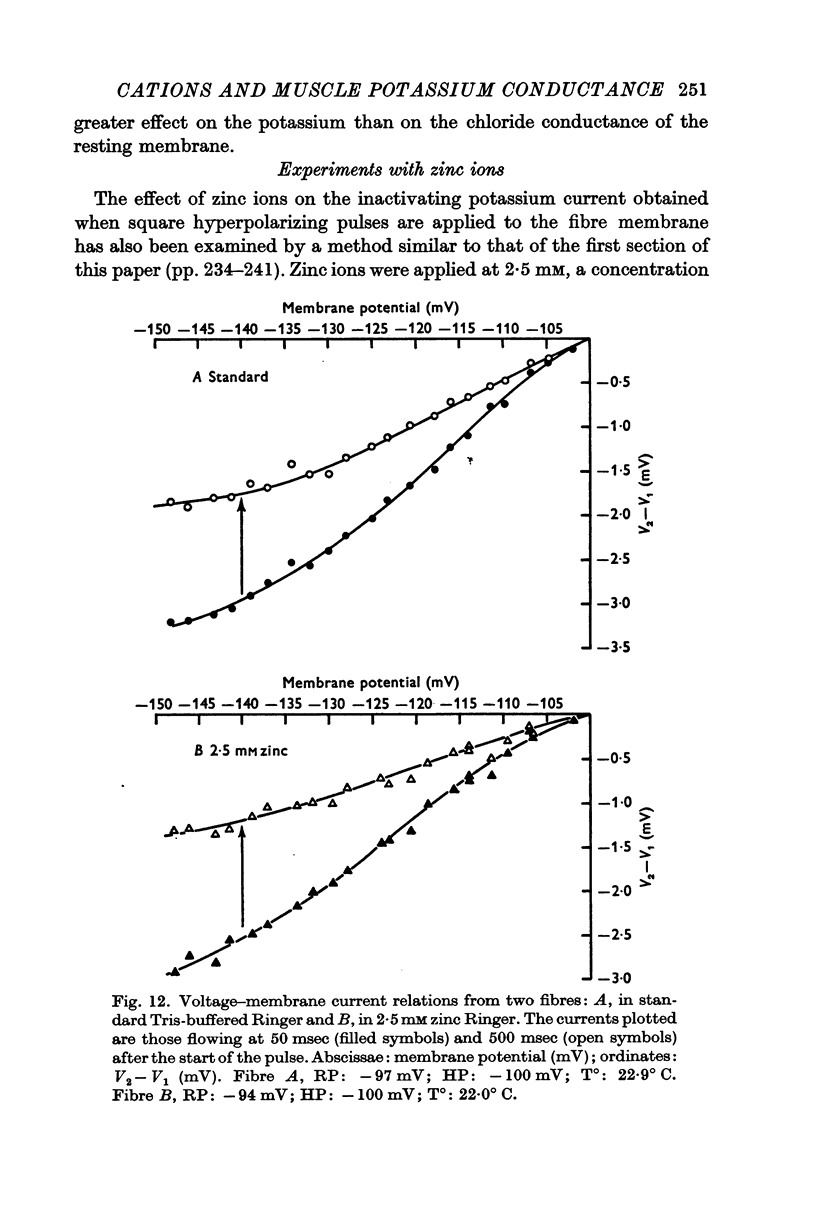
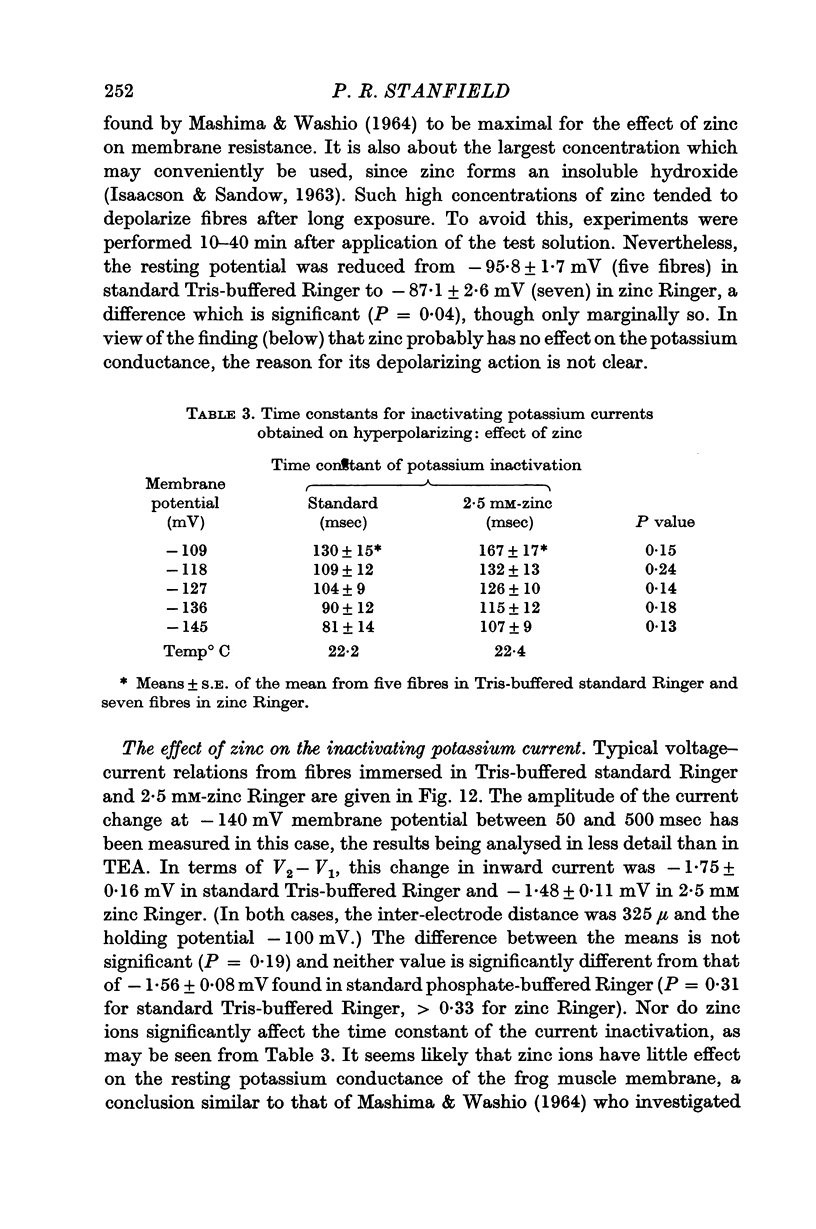
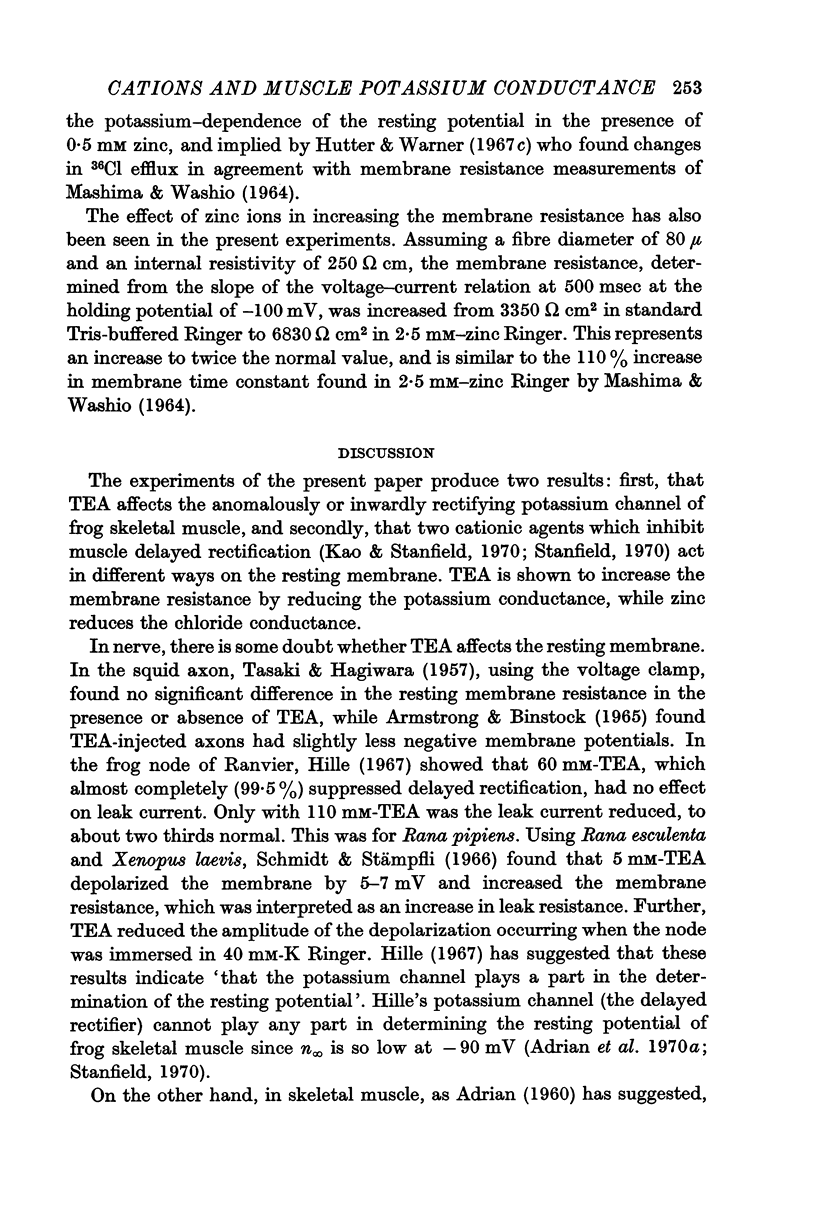
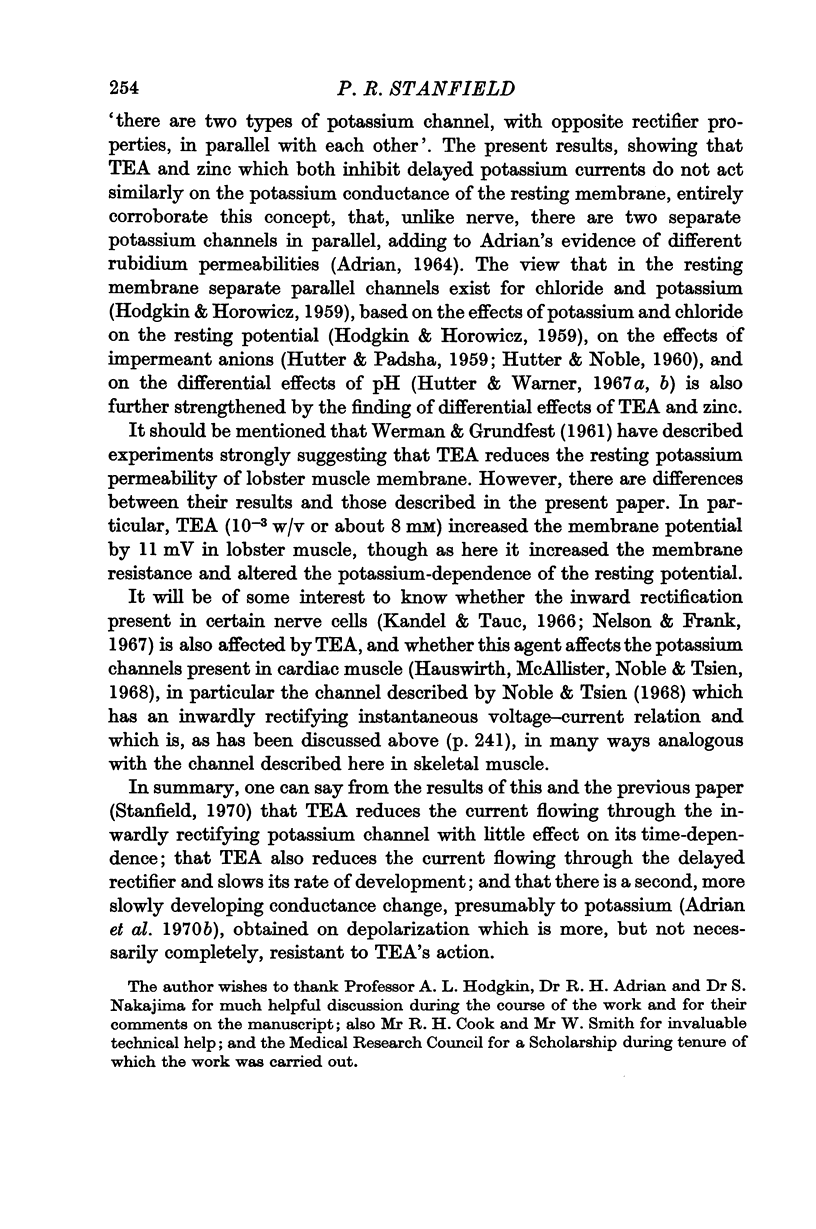
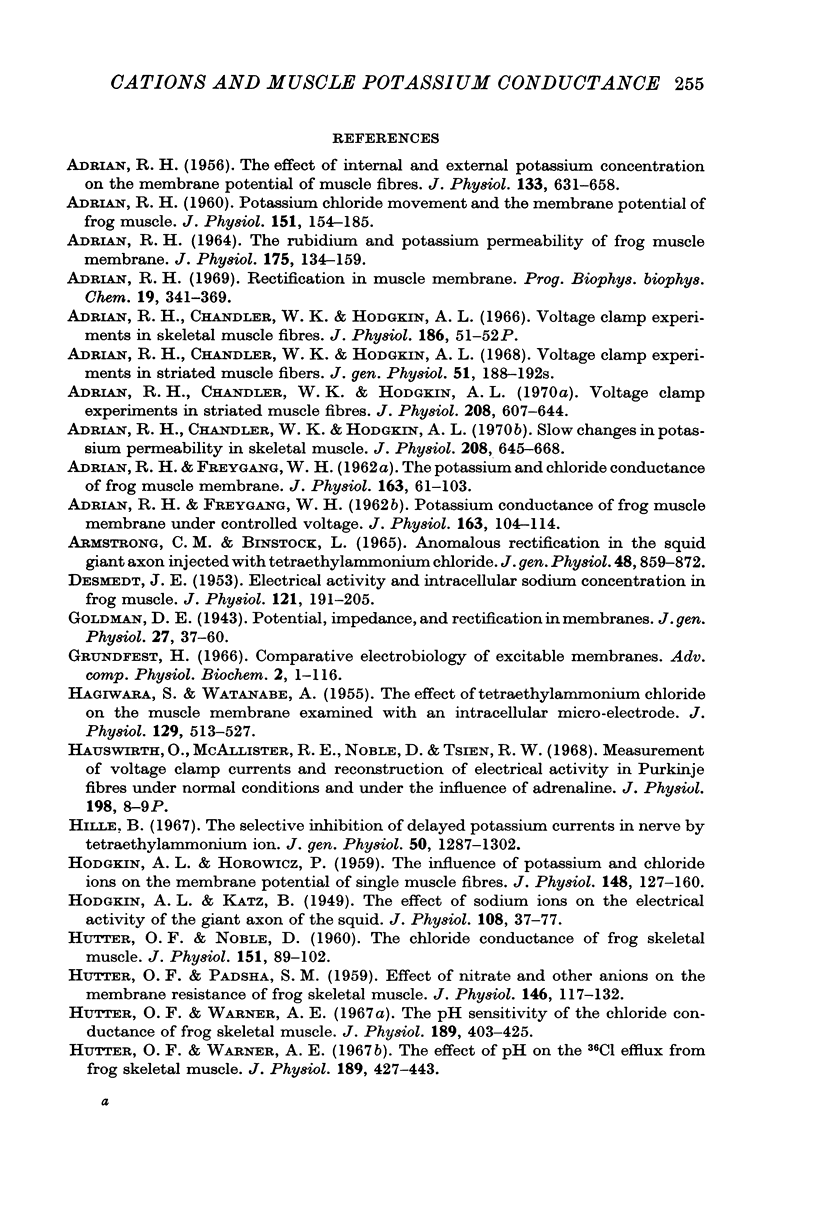
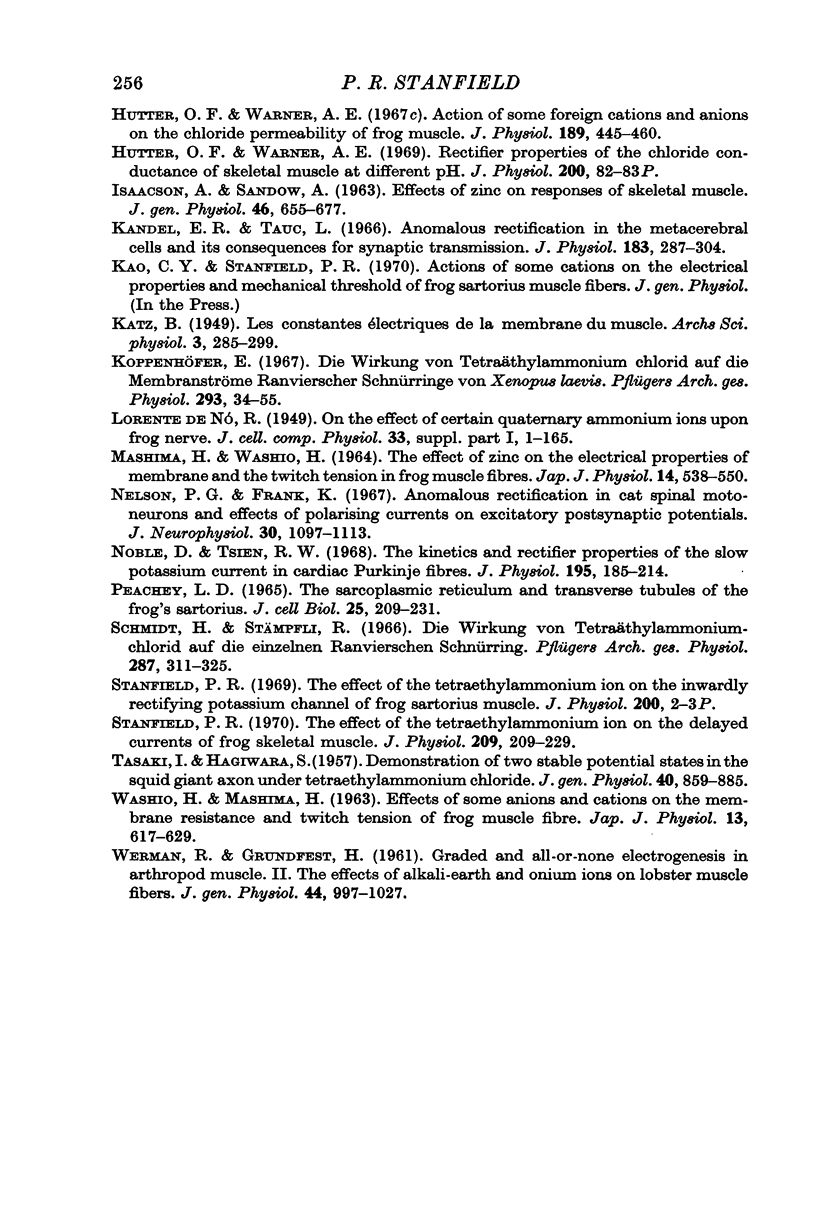
Selected References
These references are in PubMed. This may not be the complete list of references from this article.
- ADRIAN R. H., FREYGANG W. H. Potassium conductance of frog muscle membrane under controlled voltage. J Physiol. 1962 Aug;163:104–114. doi: 10.1113/jphysiol.1962.sp006960. [DOI] [PMC free article] [PubMed] [Google Scholar]
- ADRIAN R. H. Potassium chloride movement and the membrane potential of frog muscle. J Physiol. 1960 Apr;151:154–185. [PMC free article] [PubMed] [Google Scholar]
- ADRIAN R. H. THE RUBIDIUM AND POTASSIUM PERMEABILITY OF FROG MUSCLE MEMBRANE. J Physiol. 1964 Dec;175:134–159. doi: 10.1113/jphysiol.1964.sp007508. [DOI] [PMC free article] [PubMed] [Google Scholar]
- ADRIAN R. H. The effect of internal and external potassium concentration on the membrane potential of frog muscle. J Physiol. 1956 Sep 27;133(3):631–658. doi: 10.1113/jphysiol.1956.sp005615. [DOI] [PMC free article] [PubMed] [Google Scholar]
- ARMSTRONG C. M., BINSTOCK L. ANOMALOUS RECTIFICATION IN THE SQUID GIANT AXON INJECTED WITH TETRAETHYLAMMONIUM CHLORIDE. J Gen Physiol. 1965 May;48:859–872. doi: 10.1085/jgp.48.5.859. [DOI] [PMC free article] [PubMed] [Google Scholar]
- Adrian R. H., Chandler W. K., Hodgkin A. L. Slow changes in potassium permeability in skeletal muscle. J Physiol. 1970 Jul;208(3):645–668. doi: 10.1113/jphysiol.1970.sp009140. [DOI] [PMC free article] [PubMed] [Google Scholar]
- Adrian R. H., Chandler W. K., Hodgkin A. L. Voltage clamp experiments in skeletal muscle fibres. J Physiol. 1966 Oct;186(2):51P–52P. [PubMed] [Google Scholar]
- Adrian R. H., Chandler W. K., Hodgkin A. L. Voltage clamp experiments in striated muscle fibers. J Gen Physiol. 1968 May 1;51(5):188–192. [PMC free article] [PubMed] [Google Scholar]
- Adrian R. H., Chandler W. K., Hodgkin A. L. Voltage clamp experiments in striated muscle fibres. J Physiol. 1970 Jul;208(3):607–644. doi: 10.1113/jphysiol.1970.sp009139. [DOI] [PMC free article] [PubMed] [Google Scholar]
- Adrian R. H., Freygang W. H. The potassium and chloride conductance of frog muscle membrane. J Physiol. 1962 Aug;163(1):61–103. doi: 10.1113/jphysiol.1962.sp006959. [DOI] [PMC free article] [PubMed] [Google Scholar]
- Adrian R. H. Rectification in muscle membrane. Prog Biophys Mol Biol. 1969;19(2):339–369. [PubMed] [Google Scholar]
- DESMEDT J. E. Electrical activity and intracellular sodium concentration in frog muscle. J Physiol. 1953 Jul;121(1):191–205. doi: 10.1113/jphysiol.1953.sp004940. [DOI] [PMC free article] [PubMed] [Google Scholar]
- Goldman D. E. POTENTIAL, IMPEDANCE, AND RECTIFICATION IN MEMBRANES. J Gen Physiol. 1943 Sep 20;27(1):37–60. doi: 10.1085/jgp.27.1.37. [DOI] [PMC free article] [PubMed] [Google Scholar]
- Grundfest H. Comparative electrobiology of excitable membranes. Adv Comp Physiol Biochem. 1966;2:1–116. doi: 10.1016/b978-0-12-395511-1.50006-8. [DOI] [PubMed] [Google Scholar]
- HAGIWARA S., WATANABE A. The effect of tetraethylammonium chloride on the muscle membrane examined with an intracellular microelectrode. J Physiol. 1955 Sep 28;129(3):513–527. doi: 10.1113/jphysiol.1955.sp005374. [DOI] [PMC free article] [PubMed] [Google Scholar]
- HODGKIN A. L., HOROWICZ P. The influence of potassium and chloride ions on the membrane potential of single muscle fibres. J Physiol. 1959 Oct;148:127–160. doi: 10.1113/jphysiol.1959.sp006278. [DOI] [PMC free article] [PubMed] [Google Scholar]
- HODGKIN A. L., KATZ B. The effect of sodium ions on the electrical activity of giant axon of the squid. J Physiol. 1949 Mar 1;108(1):37–77. doi: 10.1113/jphysiol.1949.sp004310. [DOI] [PMC free article] [PubMed] [Google Scholar]
- HUTTER O. F., NOBLE D. The chloride conductance of frog skeletal muscle. J Physiol. 1960 Apr;151:89–102. [PMC free article] [PubMed] [Google Scholar]
- HUTTER O. F., PADSHA S. M. Effect of nitrate and other anions on the membrane resistance of frog skeletal muscle. J Physiol. 1959 Apr 23;146(1):117–132. doi: 10.1113/jphysiol.1959.sp006182. [DOI] [PMC free article] [PubMed] [Google Scholar]
- Hille B. The selective inhibition of delayed potassium currents in nerve by tetraethylammonium ion. J Gen Physiol. 1967 May;50(5):1287–1302. doi: 10.1085/jgp.50.5.1287. [DOI] [PMC free article] [PubMed] [Google Scholar]
- Hutter O. F., Warner A. E. Action of some foreign cations and anions on the chloride permeability of frog muscle. J Physiol. 1967 Apr;189(3):445–460. doi: 10.1113/jphysiol.1967.sp008178. [DOI] [PMC free article] [PubMed] [Google Scholar]
- Hutter O. F., Warner A. E. Rectifier properties of the chloride conductance of skeletal muscle at different pH. J Physiol. 1969 Jan;200(1):82P–83P. [PubMed] [Google Scholar]
- Hutter O. F., Warner A. E. The effect of pH on the 36-Cl efflux from frog skeletal muscle. J Physiol. 1967 Apr;189(3):427–443. doi: 10.1113/jphysiol.1967.sp008177. [DOI] [PMC free article] [PubMed] [Google Scholar]
- Hutter O. F., Warner A. E. The pH sensitivity of the chloride conductance of frog skeletal muscle. J Physiol. 1967 Apr;189(3):403–425. doi: 10.1113/jphysiol.1967.sp008176. [DOI] [PMC free article] [PubMed] [Google Scholar]
- ISAACSON A., SANDOW A. Effects of zinc on responses of skeletal muscle. J Gen Physiol. 1963 Mar;46:655–677. doi: 10.1085/jgp.46.4.655. [DOI] [PMC free article] [PubMed] [Google Scholar]
- Kandel E. R., Tauc L. Anomalous rectification in the metacerebral giant cells and its consequences for synaptic transmission. J Physiol. 1966 Mar;183(2):287–304. doi: 10.1113/jphysiol.1966.sp007867. [DOI] [PMC free article] [PubMed] [Google Scholar]
- MASHIMA H., WASHIO H. THE EFFECT OF ZINC ON THE ELECTRICAL PROPERTIES OF MEMBRANE AND THE TWITCH TENSION IN FROG MUSCLE FIBRES. Jpn J Physiol. 1964 Oct 15;14:538–550. doi: 10.2170/jjphysiol.14.538. [DOI] [PubMed] [Google Scholar]
- Nelson P. G., Frank K. Anomalous rectification in cat spinal motoneurons and effect of polarizing currents on excitatory postsynaptic potential. J Neurophysiol. 1967 Sep;30(5):1097–1113. doi: 10.1152/jn.1967.30.5.1097. [DOI] [PubMed] [Google Scholar]
- Noble D., Tsien R. W. The kinetics and rectifier properties of the slow potassium current in cardiac Purkinje fibres. J Physiol. 1968 Mar;195(1):185–214. doi: 10.1113/jphysiol.1968.sp008454. [DOI] [PMC free article] [PubMed] [Google Scholar]
- Peachey L. D. The sarcoplasmic reticulum and transverse tubules of the frog's sartorius. J Cell Biol. 1965 Jun;25(3 Suppl):209–231. doi: 10.1083/jcb.25.3.209. [DOI] [PubMed] [Google Scholar]
- Schmidt H., Stämpfli R. Die Wirkung von Tetraäthylammoniumchlorid auf den einzelnen Ranvierschen Schnürring. Pflugers Arch Gesamte Physiol Menschen Tiere. 1966;287(4):311–325. [PubMed] [Google Scholar]
- Stanfield P. R. The effect of the tetraethylammonium ion on the delayed currents of frog skeletal muscle. J Physiol. 1970 Jul;209(1):209–229. doi: 10.1113/jphysiol.1970.sp009163. [DOI] [PMC free article] [PubMed] [Google Scholar]
- Stanfield P. R. The effect of the tetraethylammonium ion on the inwardly rectifying potassium channel of frog sartorius muscle. J Physiol. 1969 Jan;200(1):2P–3P. [PubMed] [Google Scholar]
- TASAKI I., HAGIWAR A. S. Demonstration of two stable potential states in the squid giant axon under tetraethylammonium chloride. J Gen Physiol. 1957 Jul 20;40(6):859–885. doi: 10.1085/jgp.40.6.859. [DOI] [PMC free article] [PubMed] [Google Scholar]
- WASHIO H., MASHIMA H. EFFECTS OF SOME ANIONS AND CATIONS ON THE MEMBRANE RESISTANCE AND TWITCH TENSION OF FROG MUSCLE FIBRE. Jpn J Physiol. 1963 Dec 15;13:617–629. doi: 10.2170/jjphysiol.13.617. [DOI] [PubMed] [Google Scholar]
- WERMAN R., GRUNDFEST H. Graded and all-or-none electrogenesis in arthropod muscle. II. The effects of alkali-earth and onium ions on lobster muscle fibers. J Gen Physiol. 1961 May;44:997–1027. doi: 10.1085/jgp.44.5.997. [DOI] [PMC free article] [PubMed] [Google Scholar]