Abstract
1. Evoked electrical activity was observed in slices from guinea-pig olfactory cortex maintained in vitro. This evoked activity was studied in saline solutions containing various concentrations of Ca2+ and Mg2+.
2. The evoked potentials recorded from the surface of the prepiriform cortex comprised a negative wave (N-wave) of about 10-15 msec duration upon which a variable number of short duration positive notches were superimposed. The N-wave was identified as a population excitatory post-synaptic potential (EPSP) of the olfactory cortex neurones and the positive notches were identified with synchronous discharge of cortical neurones.
3. The relation between the EPSP amplitude and saline Ca2+ concentration was sigmoid. The N-wave amplitude (EPSP) was proportional to [Ca2+]on. When saline Ca2+ was between 0·4 and 1 mM n averaged 2·5 (range 1·5-4·3).
4. The EPSP amplitude was reduced by increasing saline [Mg2+]. High Mg2+ salines (about 10 mM) abolished the EPSP.
5. Both Ca2+ and Mg2+ in high concentrations depressed the positive notches.
6. Salines containing little or no Ca2+ and Mg2+ caused the preparation to show signs of hyperexcitability. Under these conditions the evoked potentials were very variable and unstable. `Ca2+-free' salines caused a reversible loss of excitability.
7. The results indicated that Ca2+ and Mg2+ had antagonistic effects on the release of the transmitter substance(s). Increased Ca2+ concentrations increased the output of transmitter, increased Mg2+ concentrations reduced the output of transmitter. Both Ca2+ and Mg2+ in increasing concentration increased the threshold for action potential generation.
Full text
PDF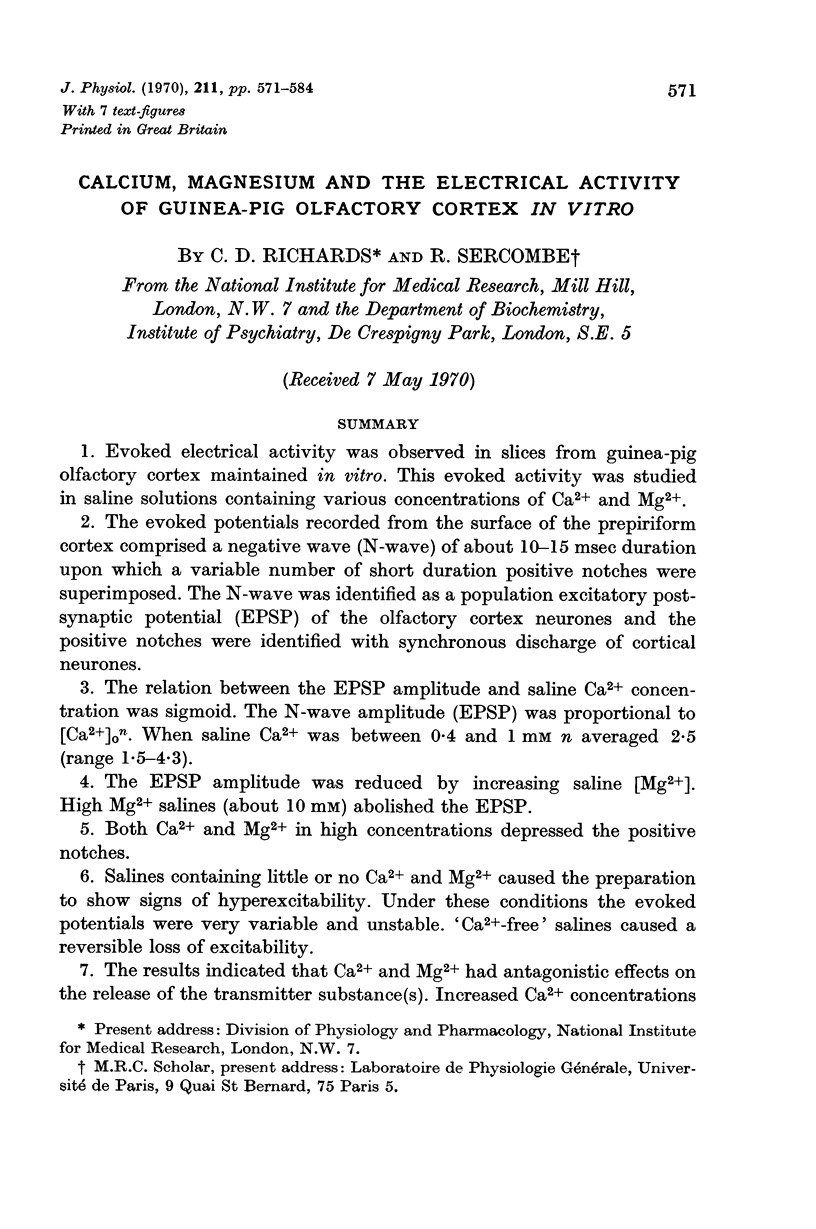
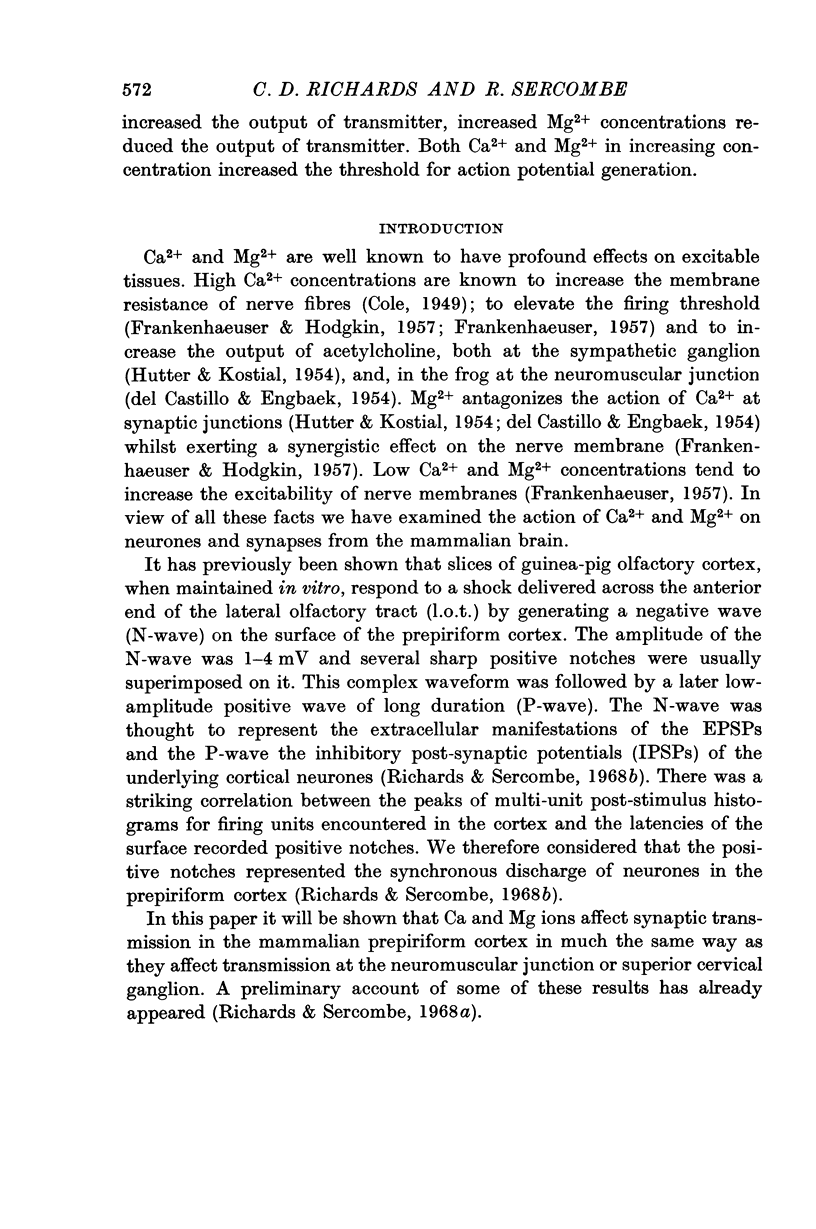
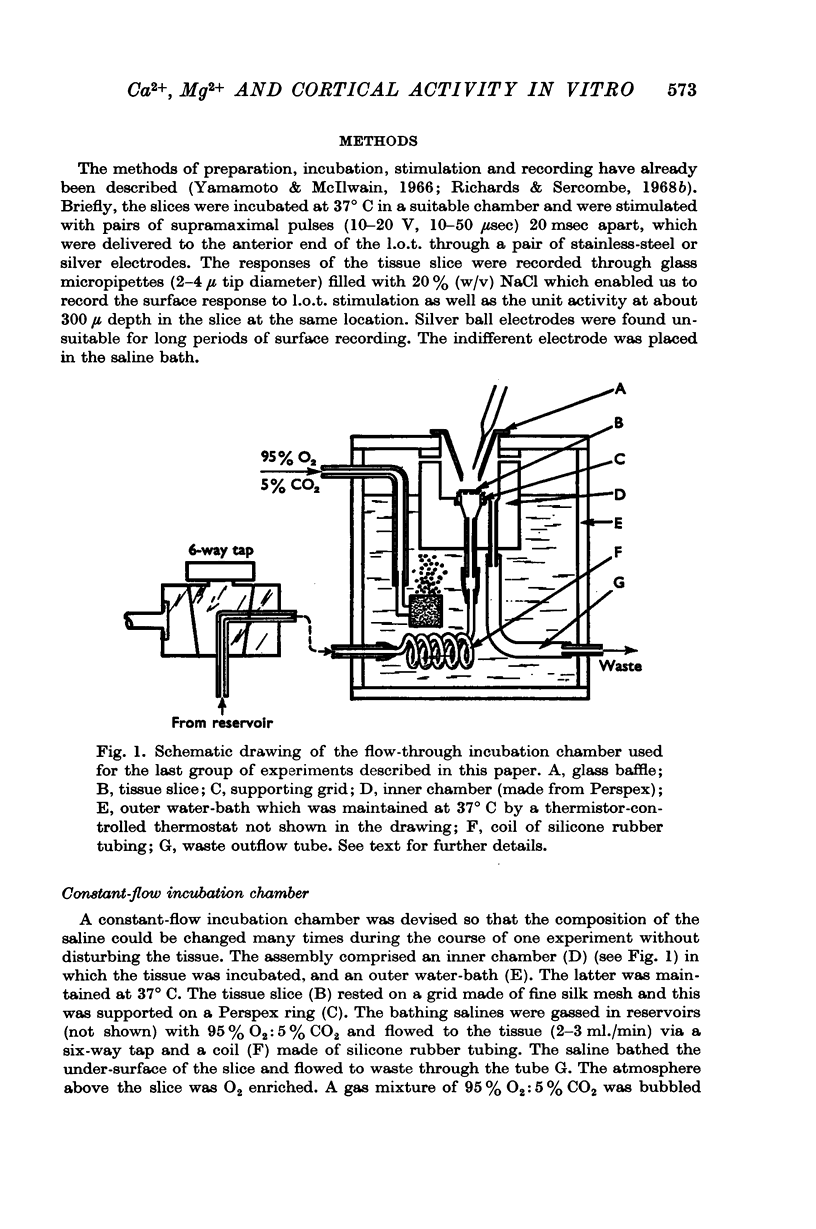
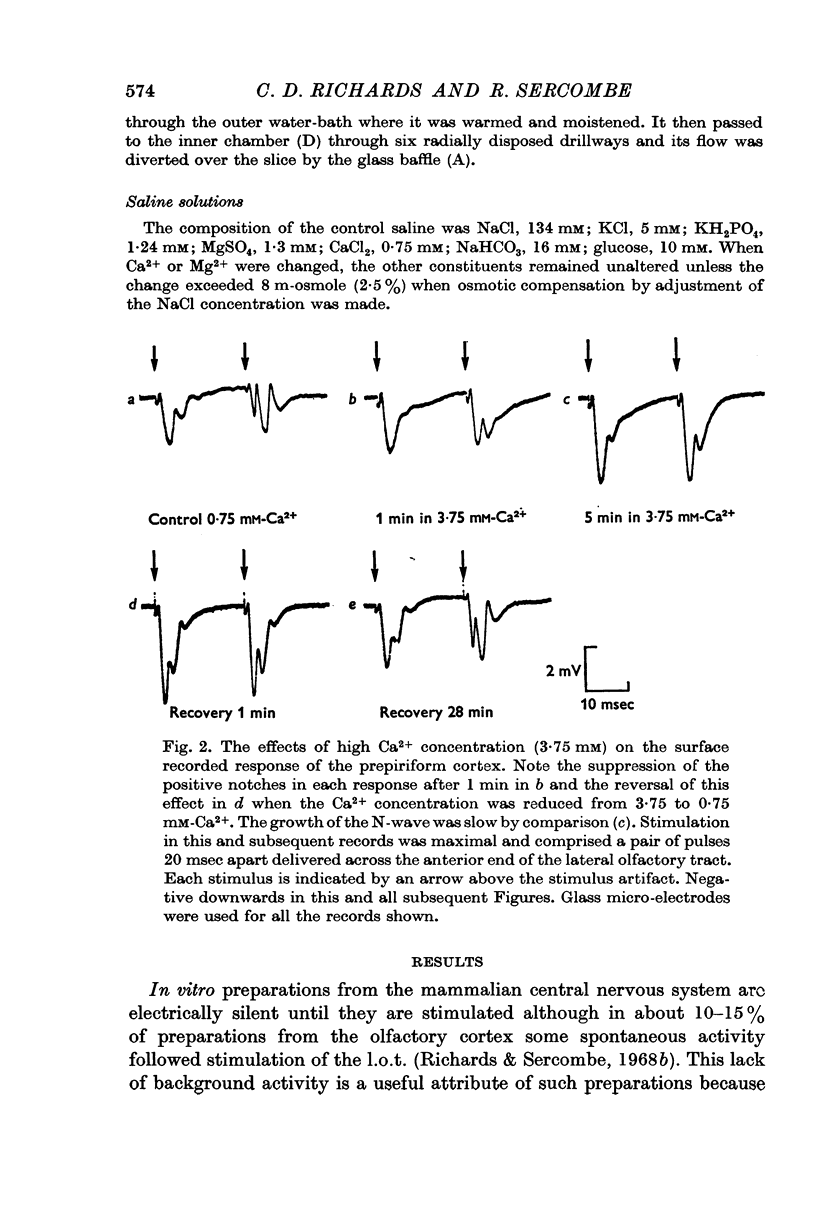
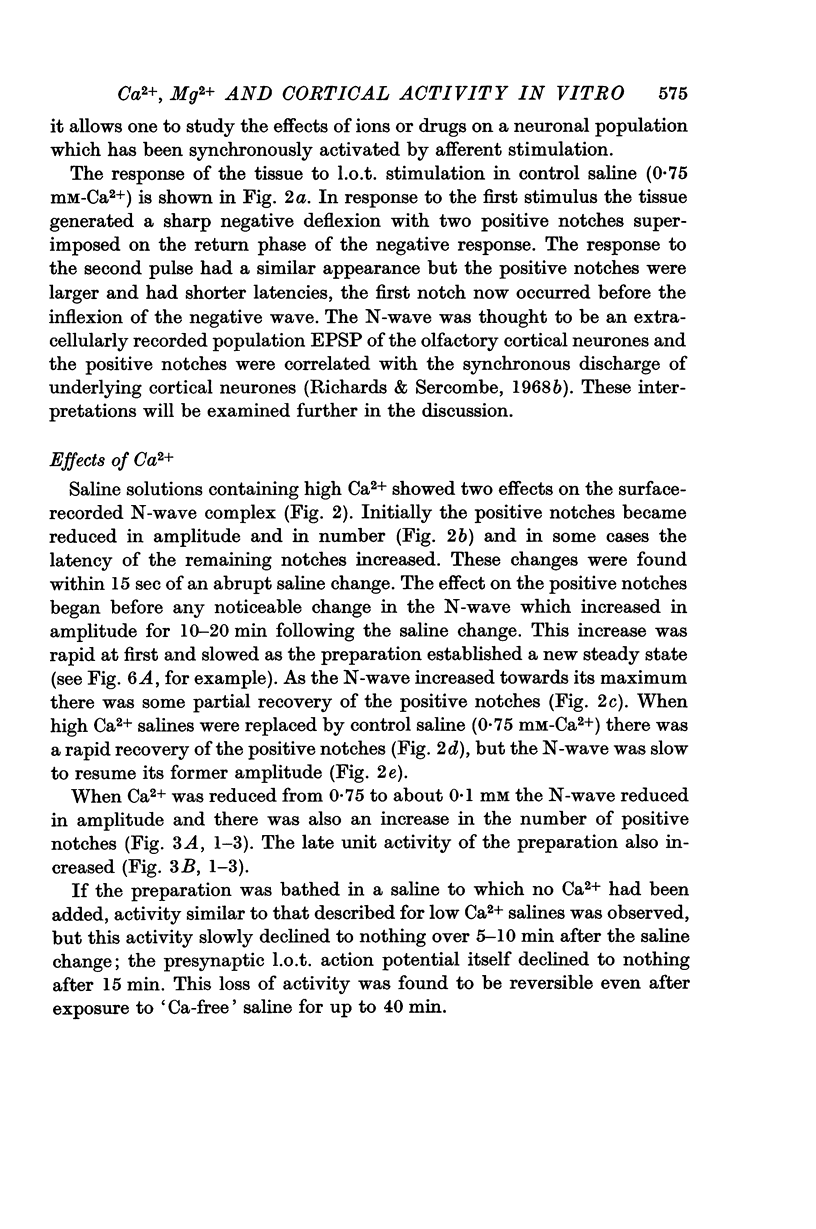
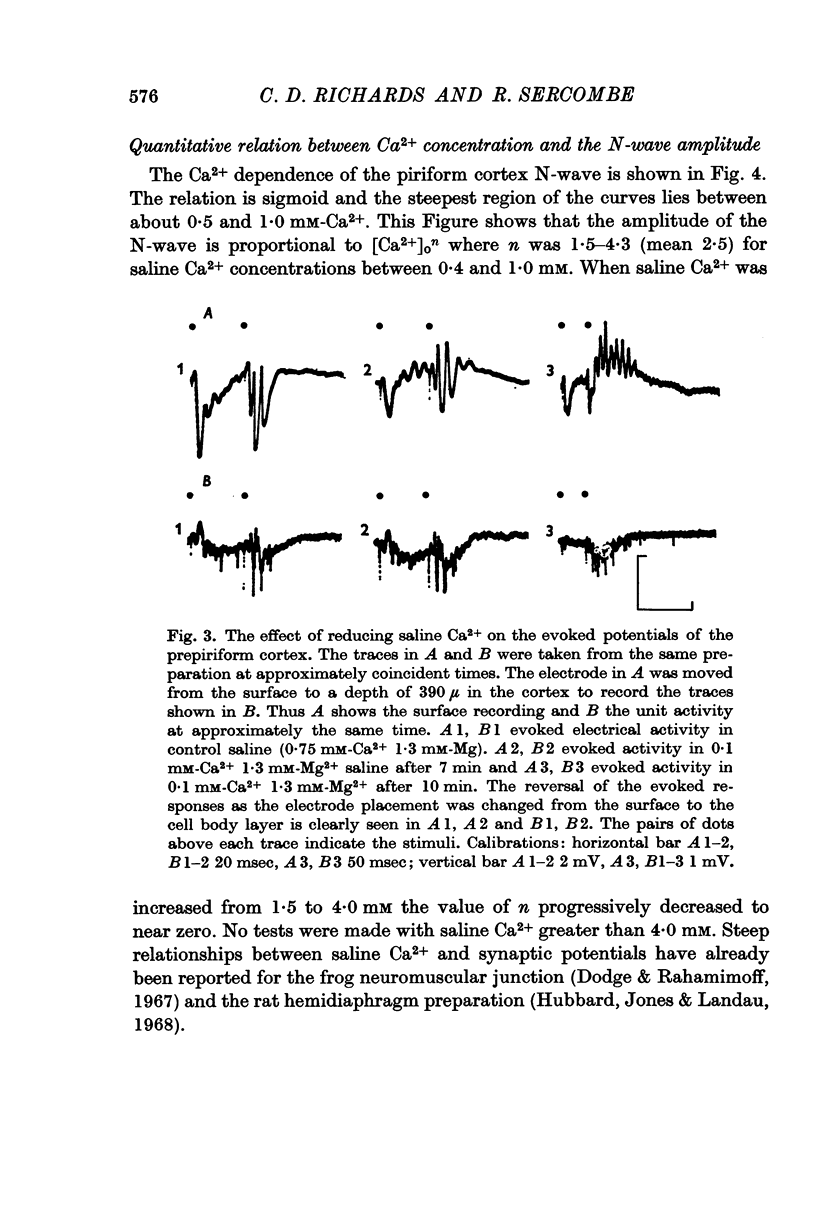
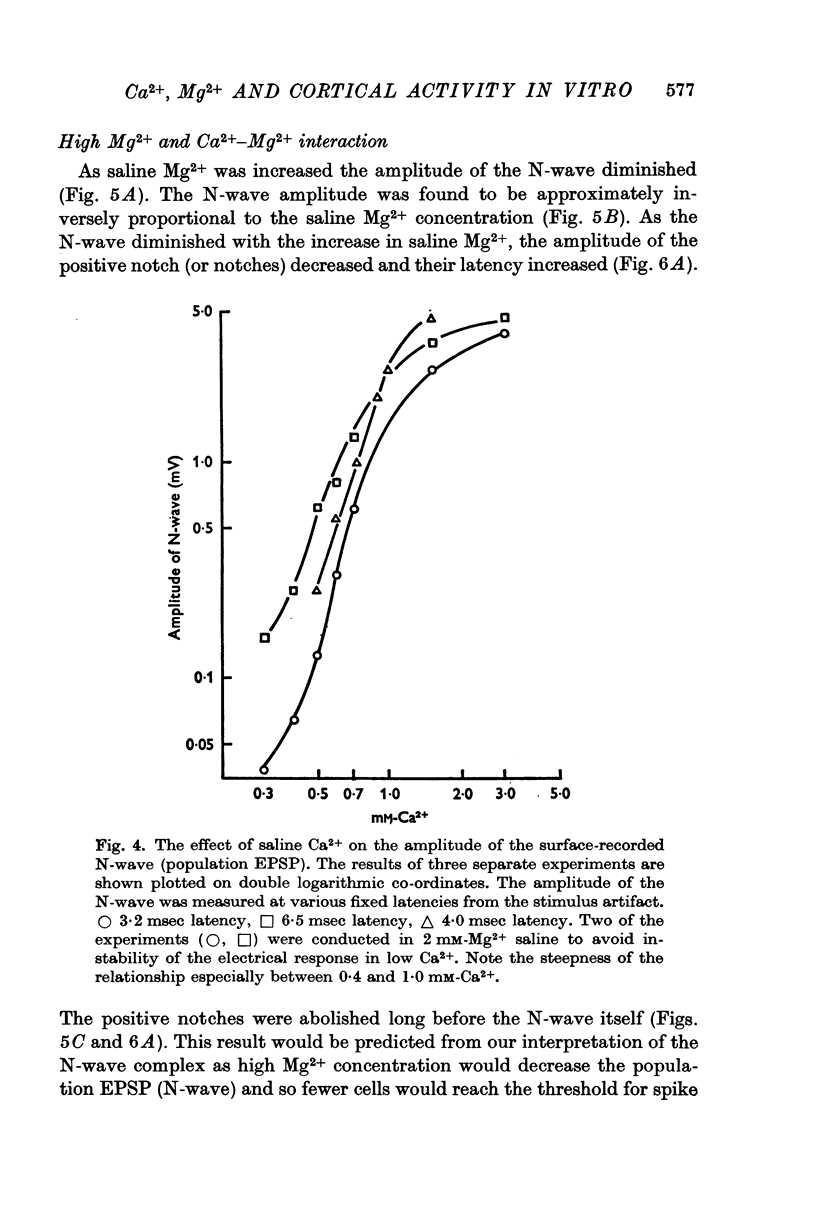
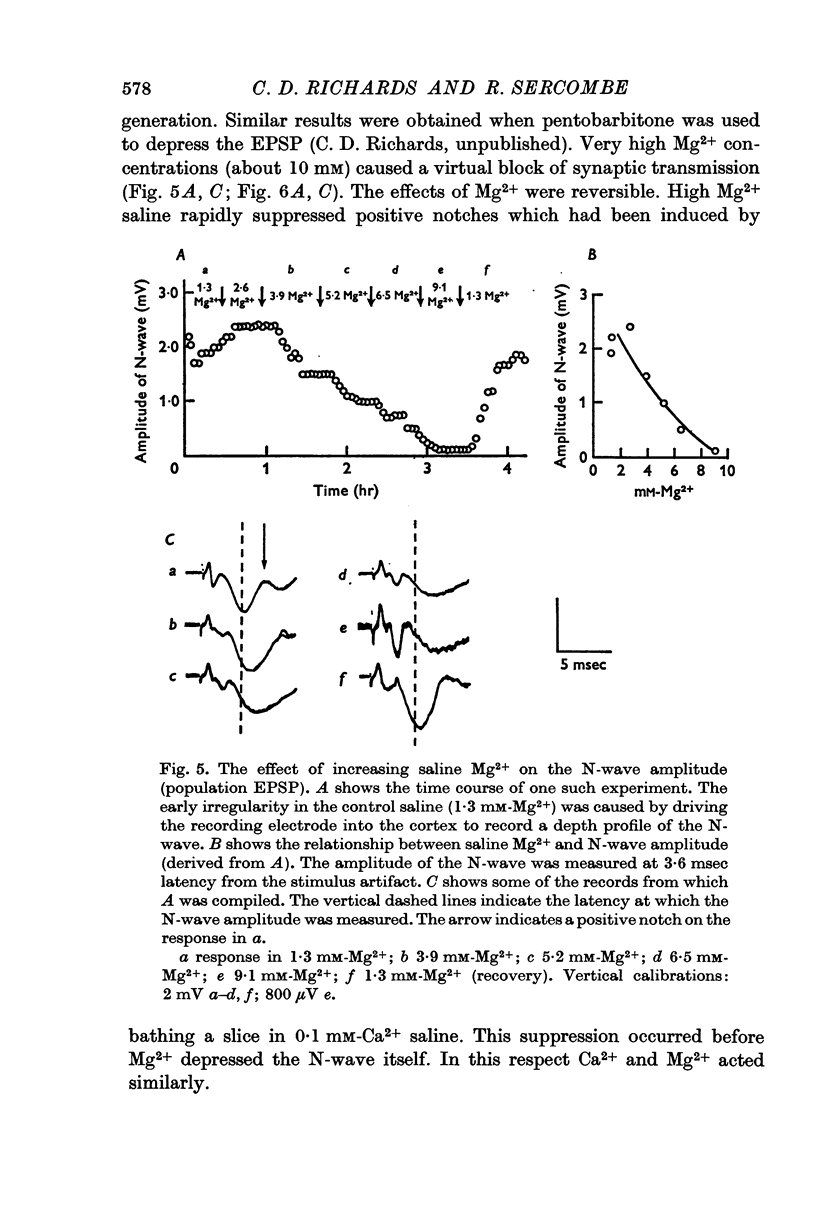
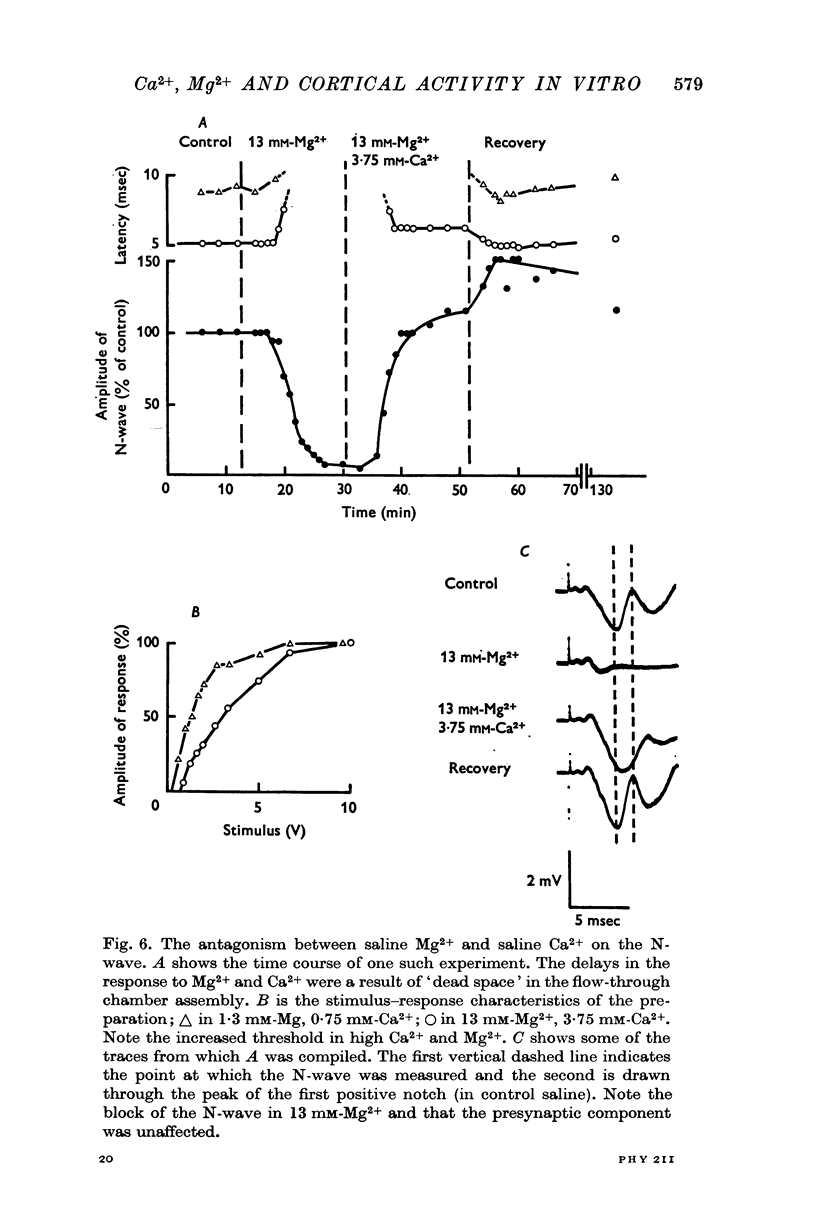
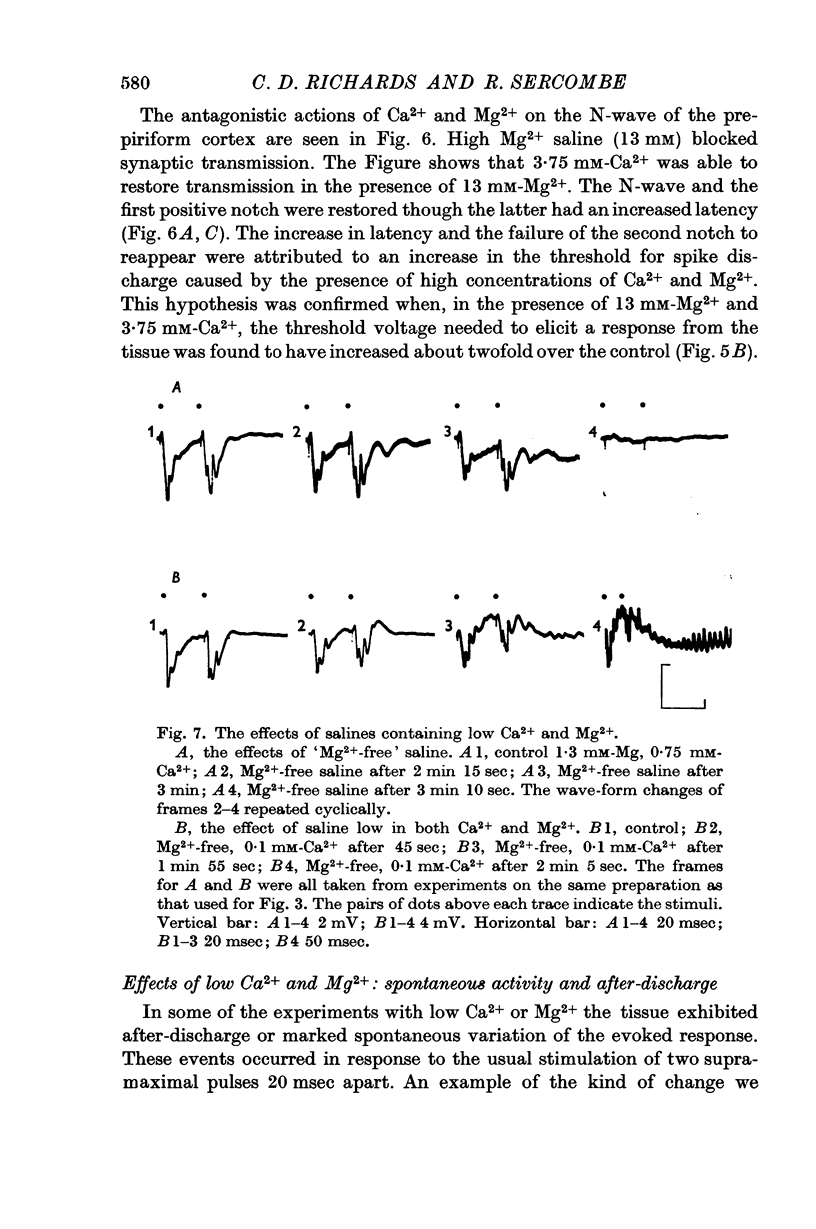
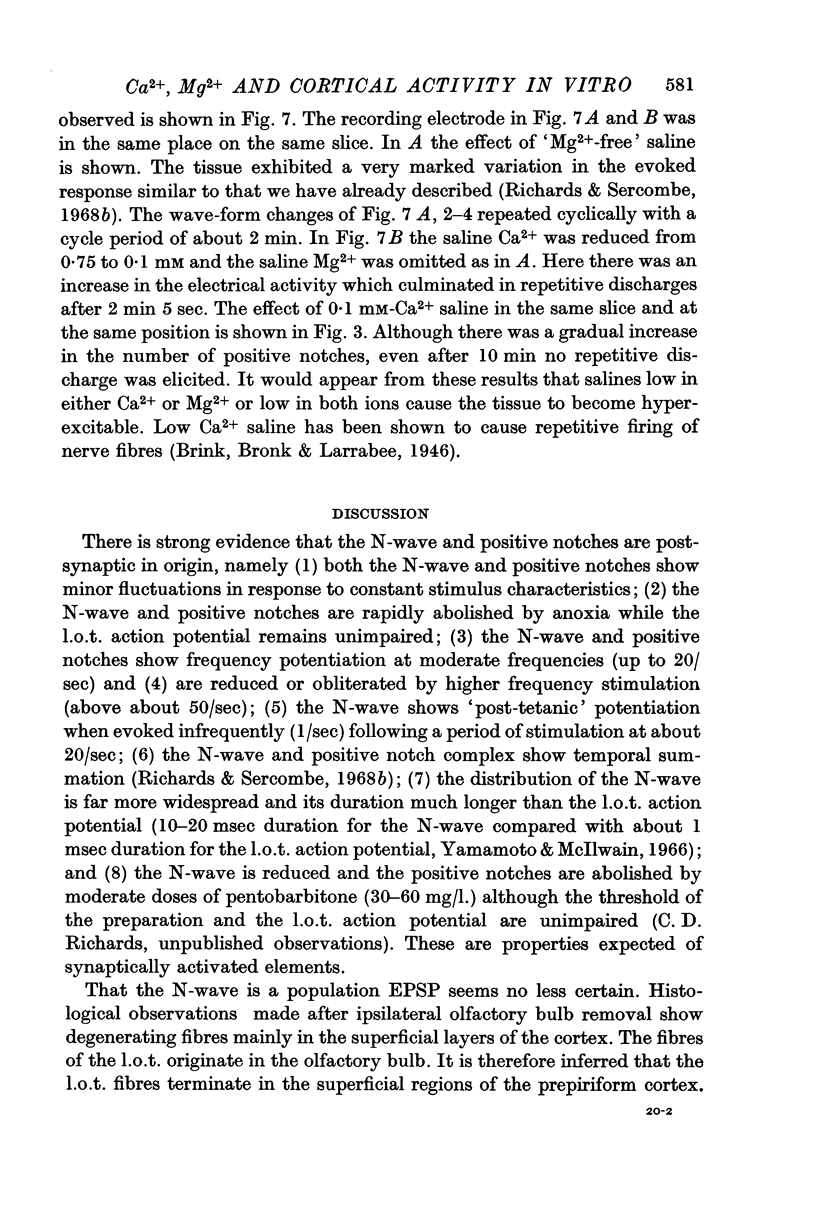
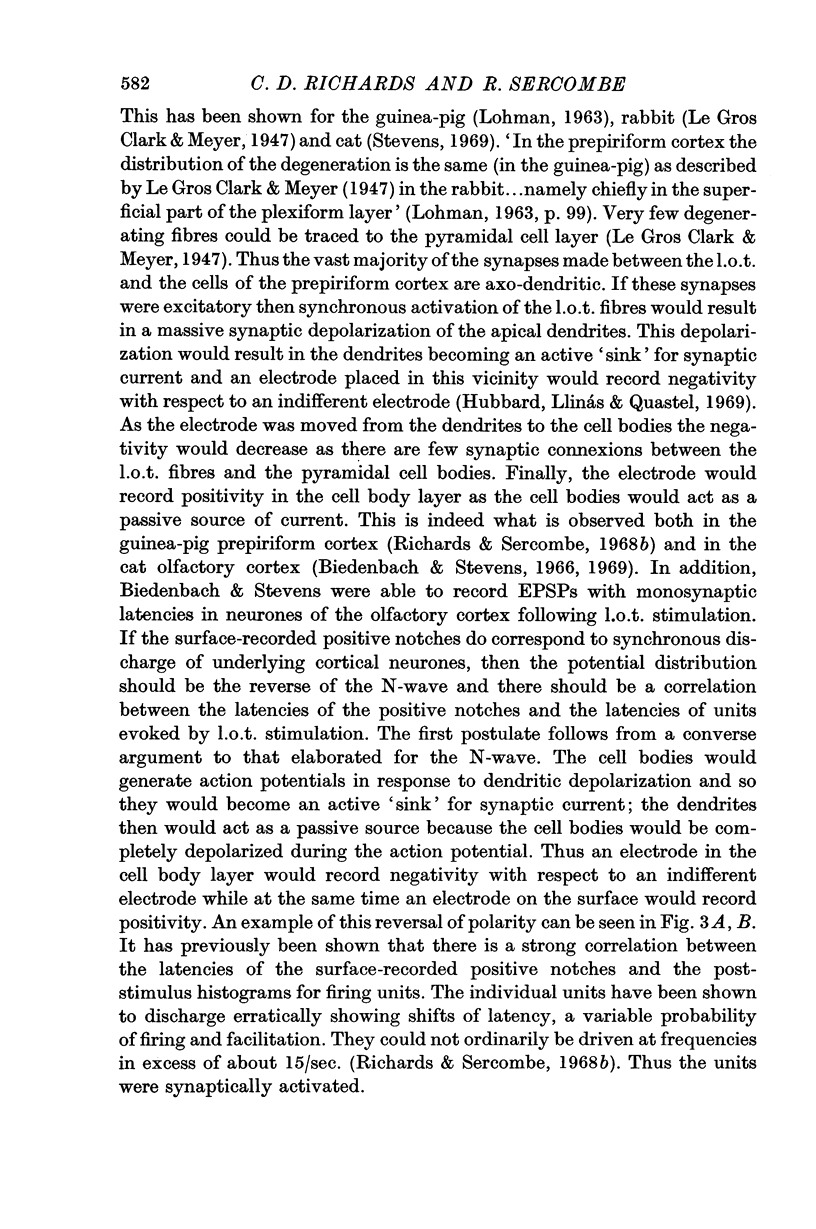
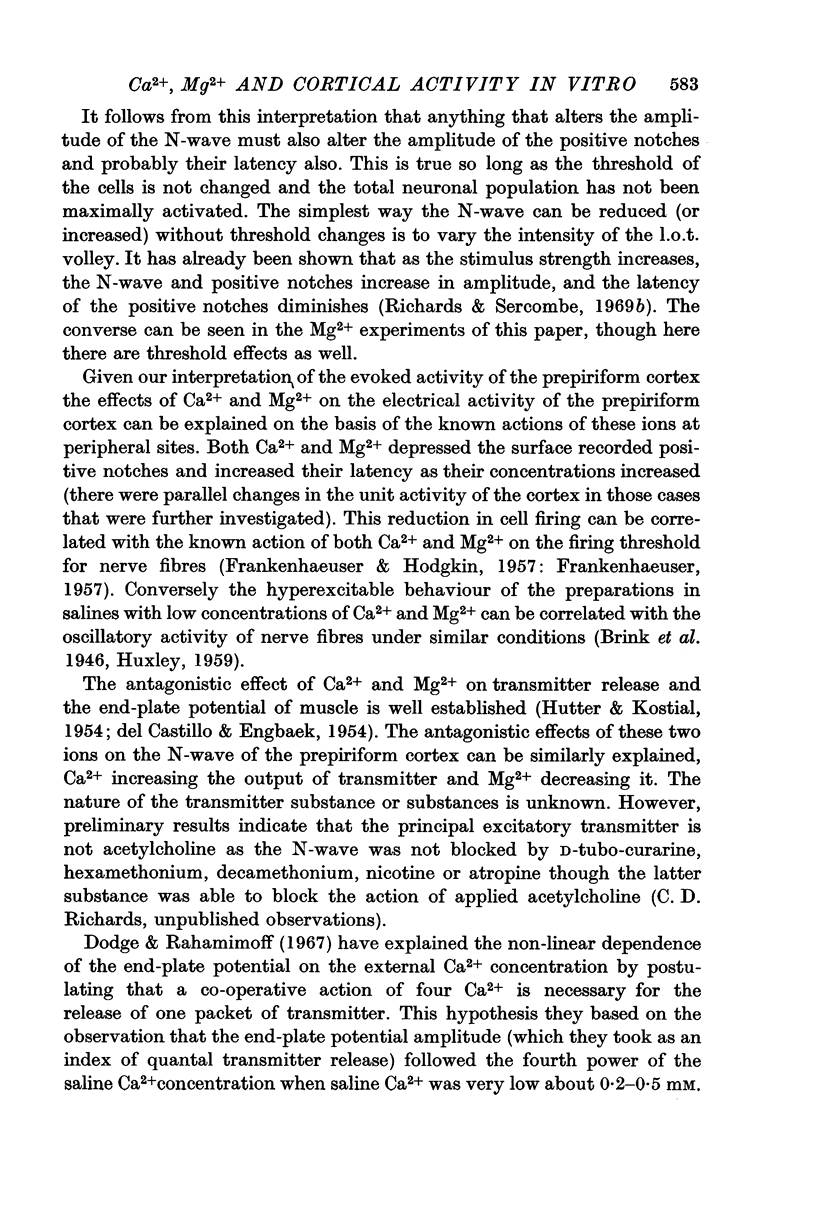
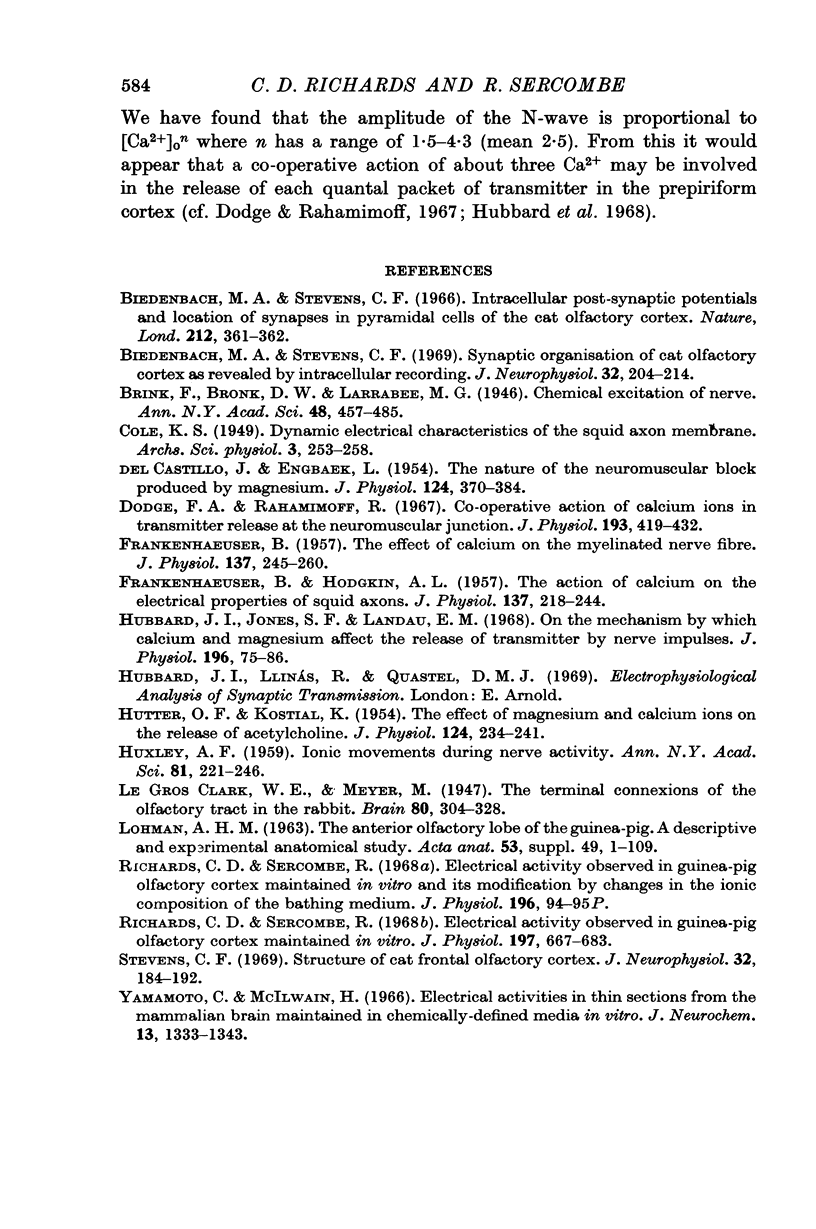
Selected References
These references are in PubMed. This may not be the complete list of references from this article.
- Biedenbach M. A., Stevens C. F. Intracellular postsynaptic potentials and location of synapses in pyramidal cells of the cat olfactory cortex. Nature. 1966 Oct 22;212(5060):361–362. doi: 10.1038/212361a0. [DOI] [PubMed] [Google Scholar]
- Biedenbach M. A., Stevens C. F. Synaptic organization of cat olfactory cortex as revealed by intracellular recording. J Neurophysiol. 1969 Mar;32(2):204–214. doi: 10.1152/jn.1969.32.2.204. [DOI] [PubMed] [Google Scholar]
- DEL CASTILLO J., ENGBAEK L. The nature of the neuromuscular block produced by magnesium. J Physiol. 1954 May 28;124(2):370–384. doi: 10.1113/jphysiol.1954.sp005114. [DOI] [PMC free article] [PubMed] [Google Scholar]
- Dodge F. A., Jr, Rahamimoff R. Co-operative action a calcium ions in transmitter release at the neuromuscular junction. J Physiol. 1967 Nov;193(2):419–432. doi: 10.1113/jphysiol.1967.sp008367. [DOI] [PMC free article] [PubMed] [Google Scholar]
- FRANKENHAEUSER B., HODGKIN A. L. The action of calcium on the electrical properties of squid axons. J Physiol. 1957 Jul 11;137(2):218–244. doi: 10.1113/jphysiol.1957.sp005808. [DOI] [PMC free article] [PubMed] [Google Scholar]
- FRANKENHAEUSER B. The effect of calcium on the myelinated nerve fibre. J Physiol. 1957 Jul 11;137(2):245–260. doi: 10.1113/jphysiol.1957.sp005809. [DOI] [PMC free article] [PubMed] [Google Scholar]
- HUTTER O. F., KOSTIAL K. Effect of magnesium and calcium ions on the release of acetylcholine. J Physiol. 1954 May 28;124(2):234–241. doi: 10.1113/jphysiol.1954.sp005102. [DOI] [PMC free article] [PubMed] [Google Scholar]
- HUXLEY A. F. Ion movements during nerve activity. Ann N Y Acad Sci. 1959 Aug 28;81:221–246. doi: 10.1111/j.1749-6632.1959.tb49311.x. [DOI] [PubMed] [Google Scholar]
- Hubbard J. I., Jones S. F., Landau E. M. On the mechanism by which calcium and magnesium affect the release of transmitter by nerve impulses. J Physiol. 1968 May;196(1):75–86. doi: 10.1113/jphysiol.1968.sp008495. [DOI] [PMC free article] [PubMed] [Google Scholar]
- Richards C. D., Sercombe R. Electrical activity observed in guinea-pig olfactory cortex maintained in vitro and its modification by changes in the ionic composition of the bathing medium. J Physiol. 1968 May;196(2):94P–95P. [PubMed] [Google Scholar]
- Richards C. D., Sercombe R. Electrical activity observed in guinea-pig olfactory cortex maintained in vitro. J Physiol. 1968 Aug;197(3):667–683. doi: 10.1113/jphysiol.1968.sp008581. [DOI] [PMC free article] [PubMed] [Google Scholar]
- Stevens C. F. Structure of cat frontal olfactory cortex. J Neurophysiol. 1969 Mar;32(2):184–192. doi: 10.1152/jn.1969.32.2.184. [DOI] [PubMed] [Google Scholar]
- Yamamoto C., McIlwain H. Electrical activities in thin sections from the mammalian brain maintained in chemically-defined media in vitro. J Neurochem. 1966 Dec;13(12):1333–1343. doi: 10.1111/j.1471-4159.1966.tb04296.x. [DOI] [PubMed] [Google Scholar]