Abstract
We report here the first observations of the effects of elevated hydrostatic pressure on the kinetics of bilayer membrane conductance induced by the pore-forming antibiotic, alamethicin. Bacterial phosphatidylethanolamine-squalene bilayer membranes were formed by the apposition of lipid monolayers in a vessel capable of sustaining hydrostatic pressures in the range, 0.1-100 MPa (1-1,000 atm). Principal observations were (a) the lifetimes of discrete conductance states were lengthened with increasing pressure, (b) both the onset and decay of alamethicin conductance accompanying application and removal of supra-threshold voltage pulses were slowed with increasing pressure, (c) the onset of alamethicin conductance at elevated pressure became distinctly sigmoidal, suggesting an electrically silent intermediate state of channel assembly, (d) the magnitudes of the discrete conductance levels observed did not change with pressure, and, (e) the voltage threshold for the onset of alamethicin conductance was not altered by pressure. Apparent activation volumes for both the formation and decay of conducting states were positive and of comparable magnitude, namely, approximately 100 A3/event. Observation d indicates that channel geometry and the kinetics of ion transport through open channels were not affected by pressure in the range employed. The remaining observations indicate that, while the relative positions of free-energy minima characterizing individual conducting states at a given voltage were not modified by pressure, the heights of intervening potential maxima were increased by its application.
Full text
PDF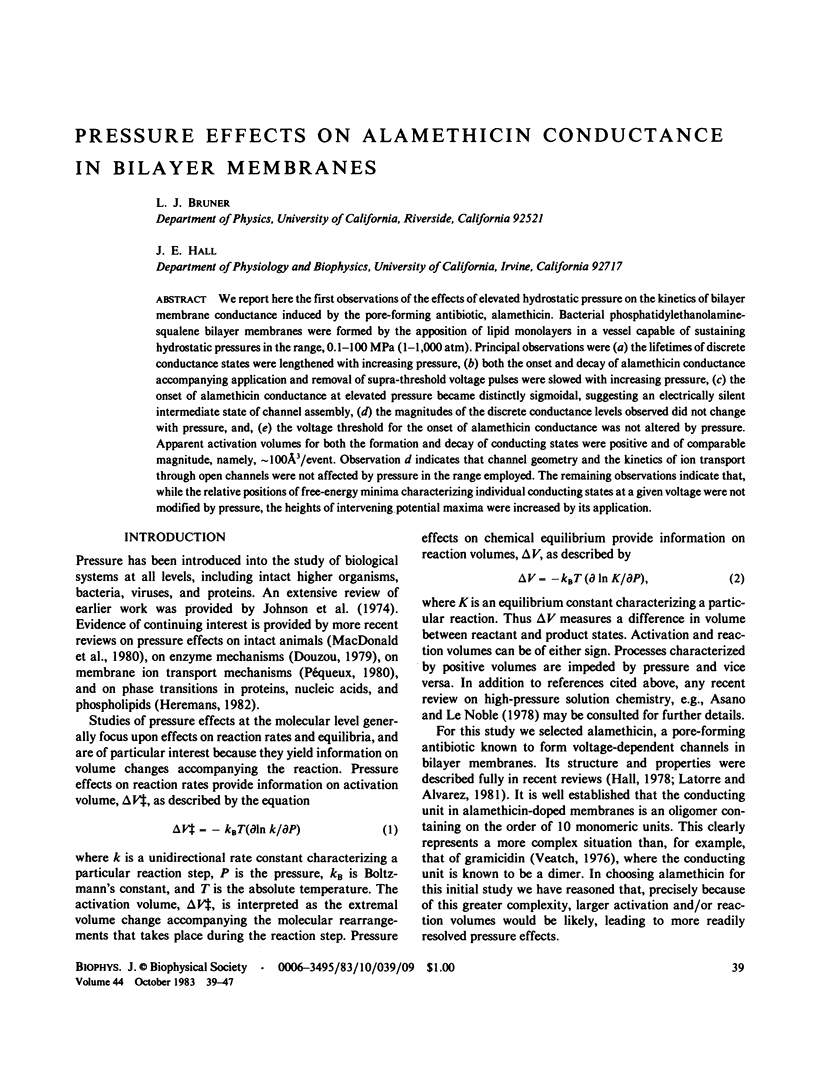
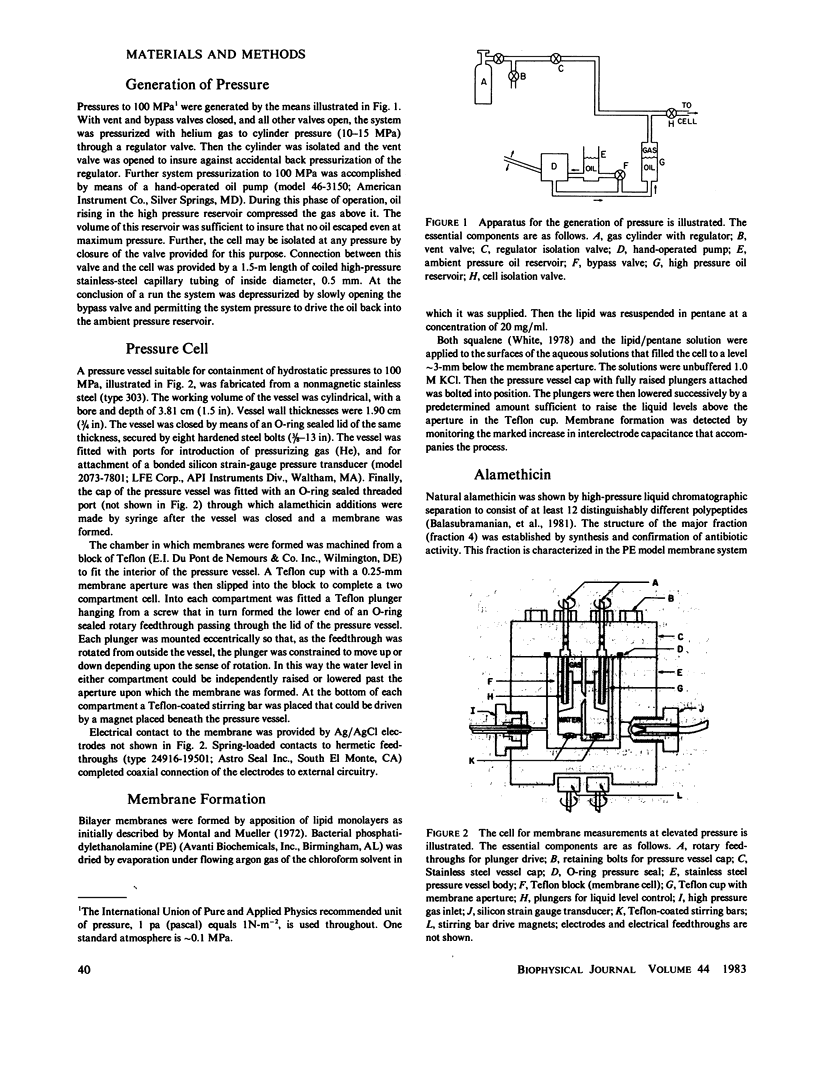
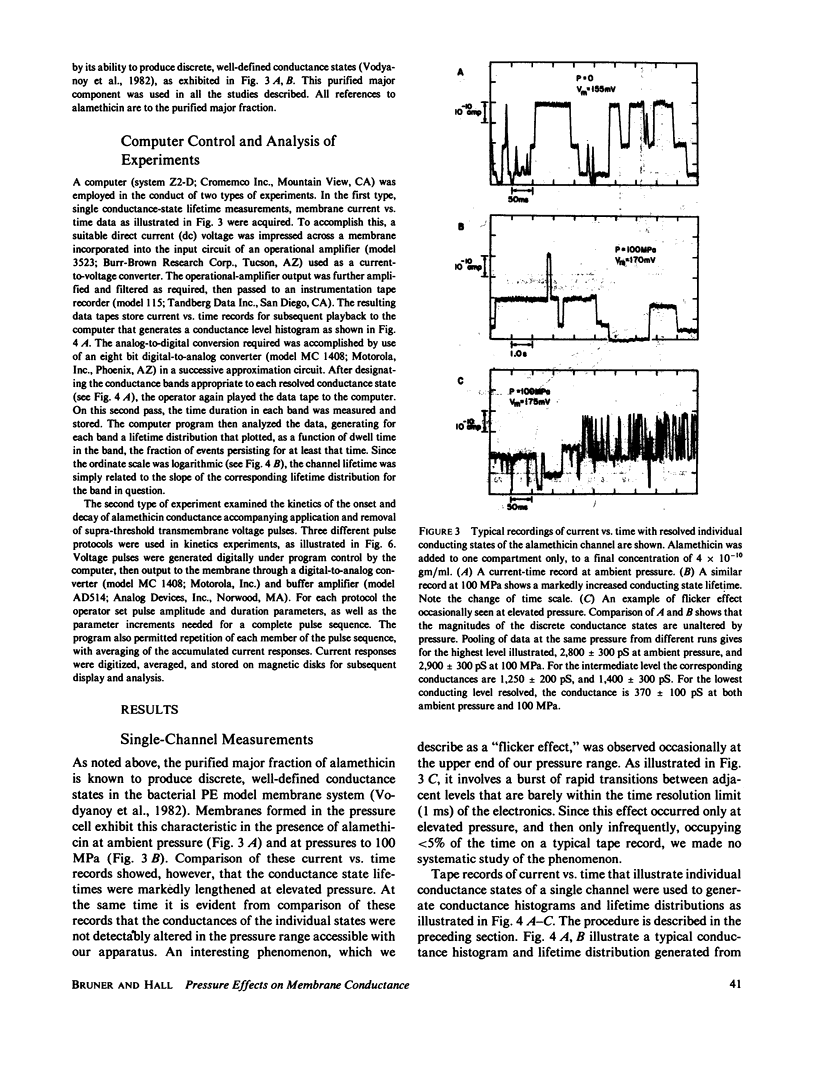
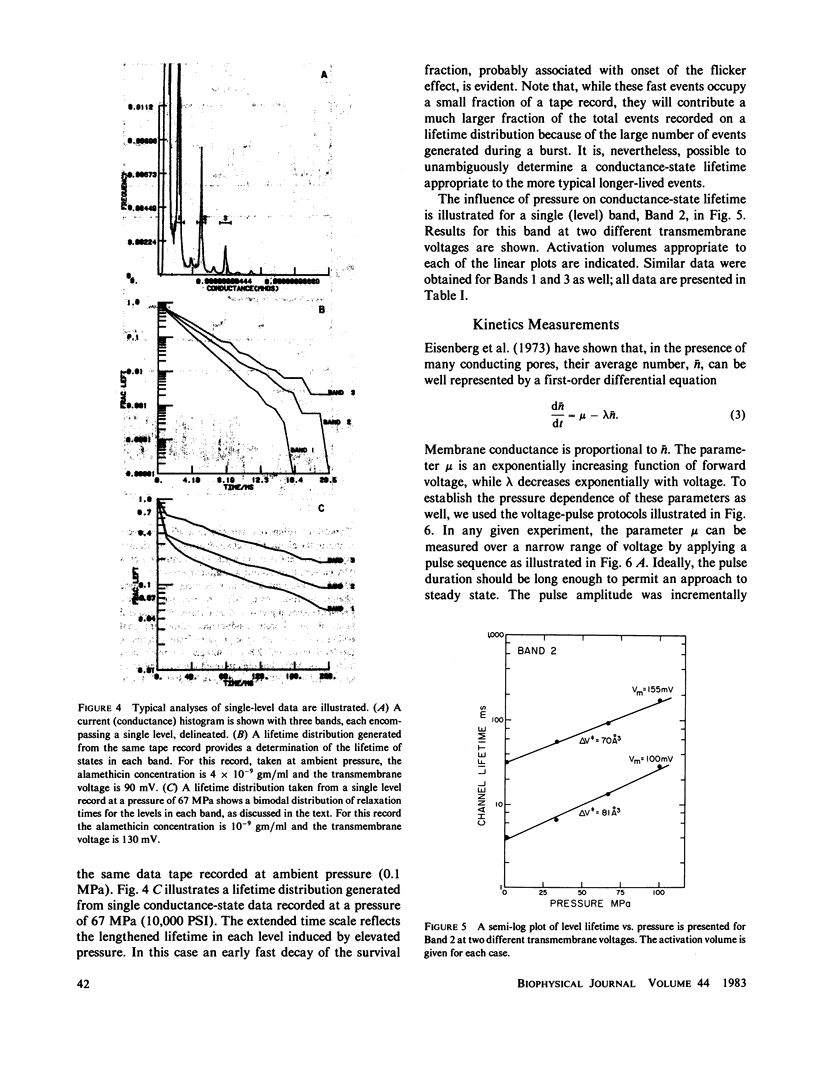
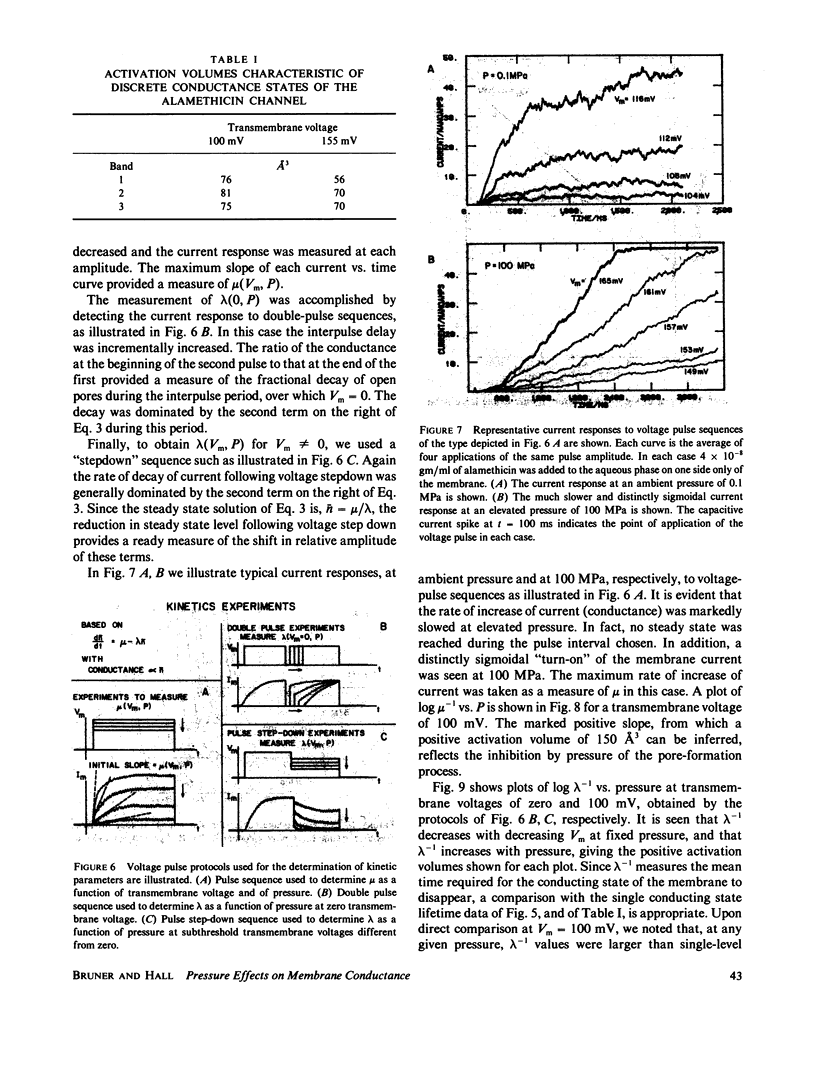
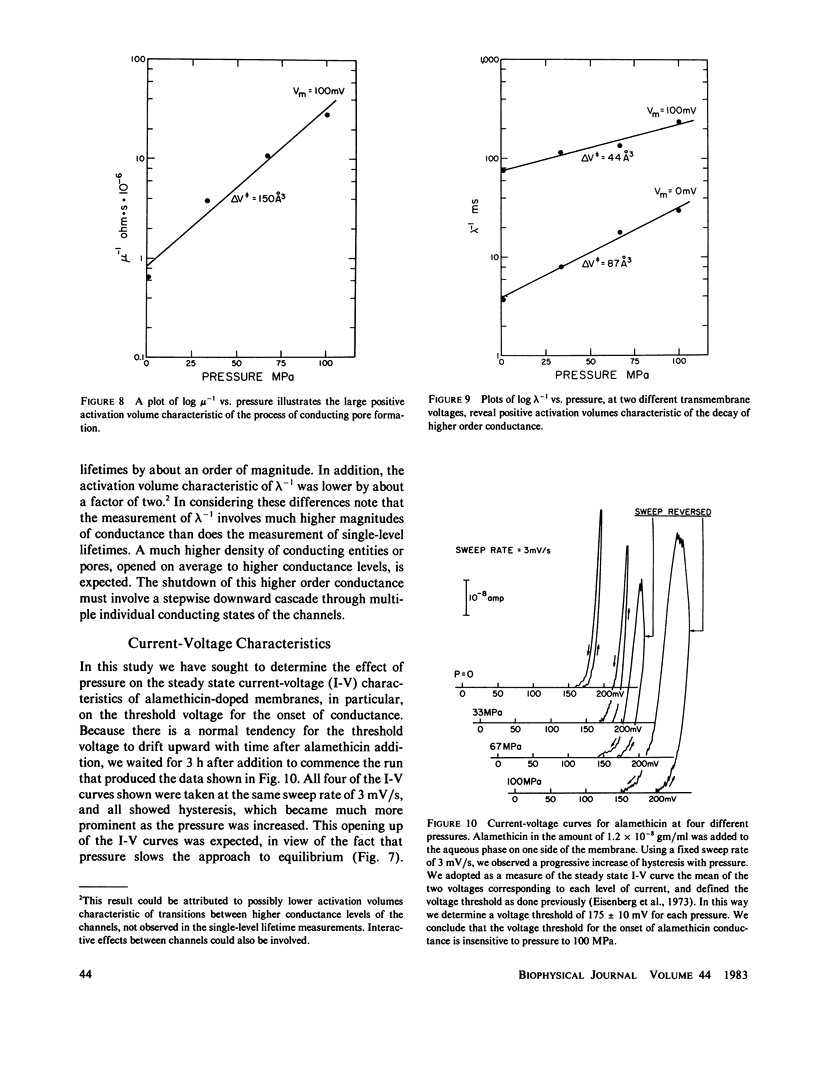
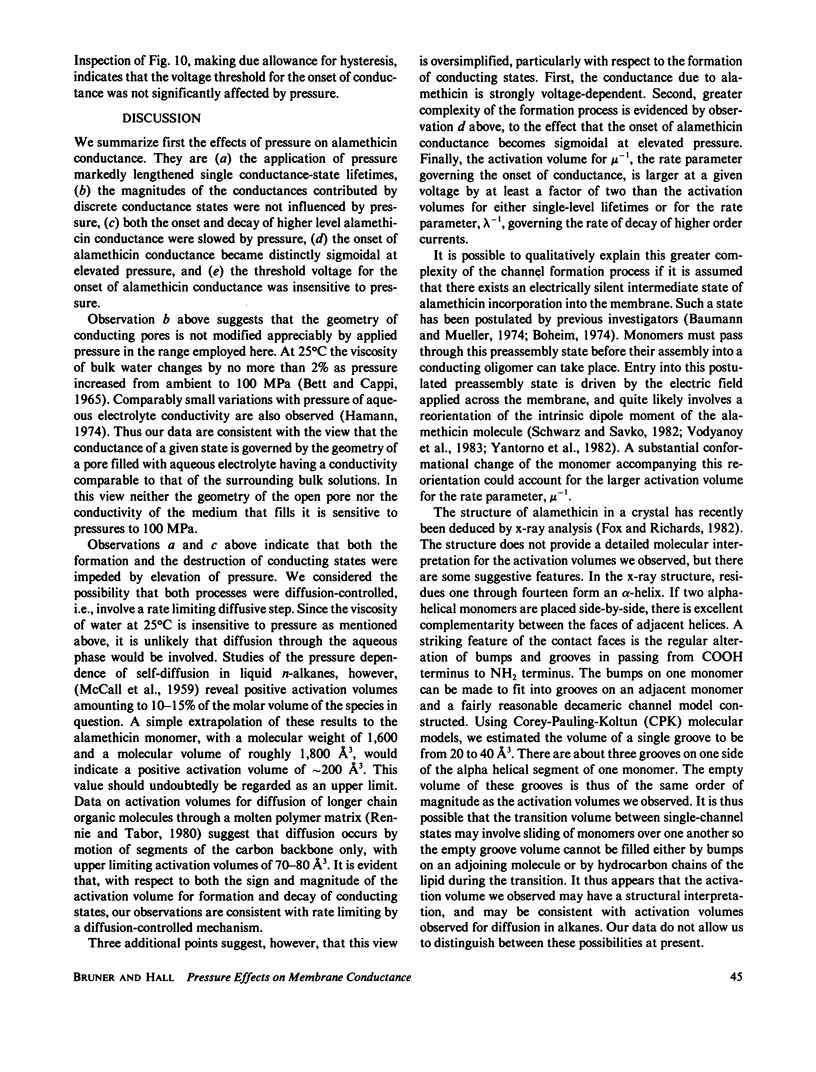
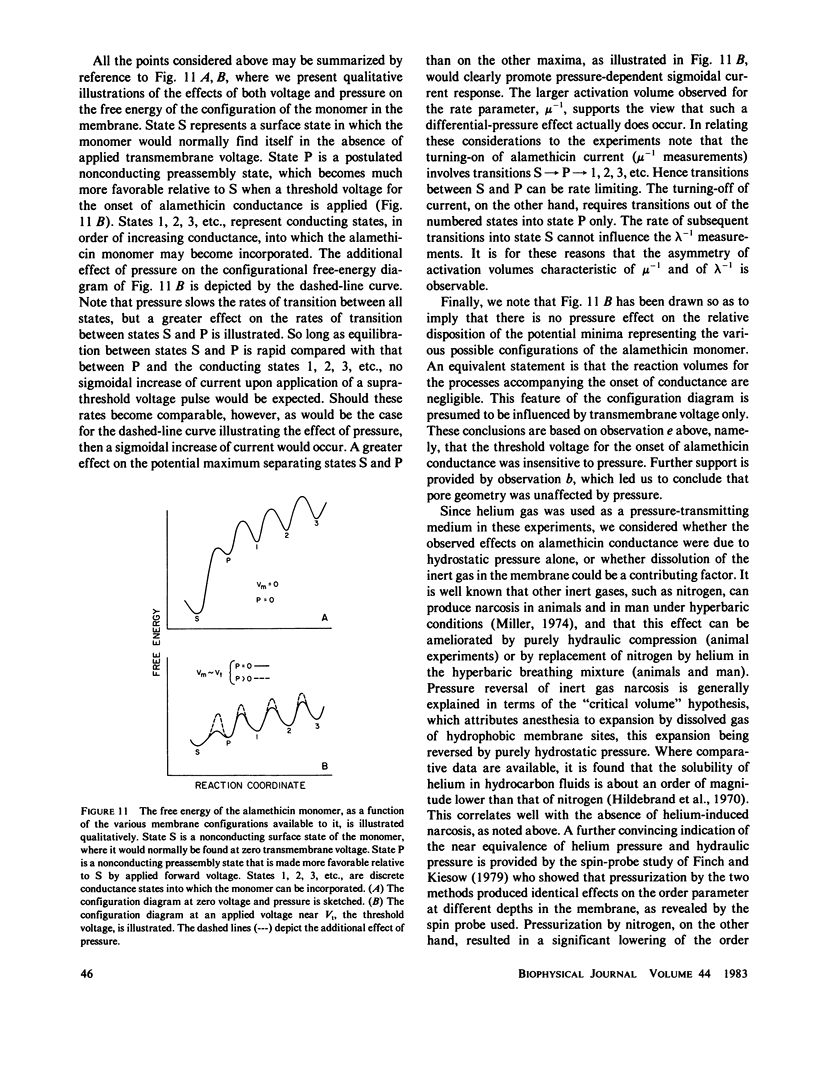
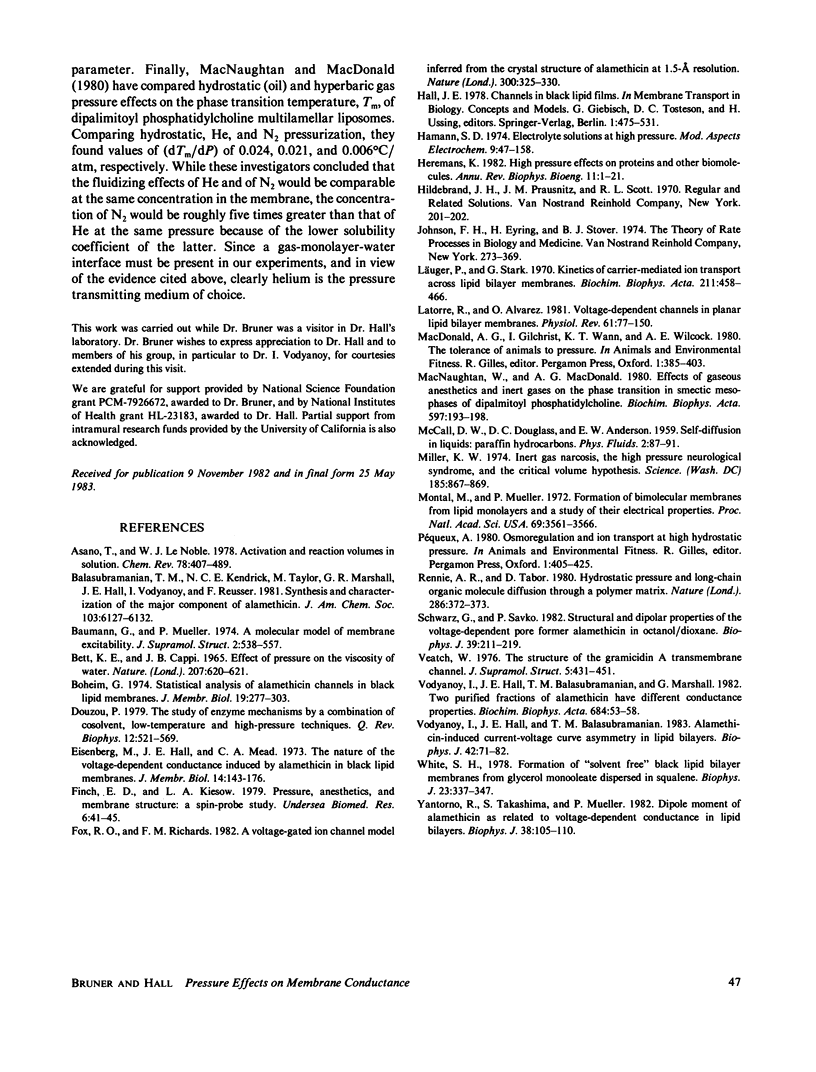
Selected References
These references are in PubMed. This may not be the complete list of references from this article.
- Baumann G., Mueller P. A molecular model of membrane excitability. J Supramol Struct. 1974;2(5-6):538–557. doi: 10.1002/jss.400020504. [DOI] [PubMed] [Google Scholar]
- Boheim G. Statistical analysis of alamethicin channels in black lipid membranes. J Membr Biol. 1974;19(3):277–303. doi: 10.1007/BF01869983. [DOI] [PubMed] [Google Scholar]
- Eisenberg M., Hall J. E., Mead C. A. The nature of the voltage-dependent conductance induced by alamethicin in black lipid membranes. J Membr Biol. 1973 Dec 31;14(2):143–176. doi: 10.1007/BF01868075. [DOI] [PubMed] [Google Scholar]
- Fox R. O., Jr, Richards F. M. A voltage-gated ion channel model inferred from the crystal structure of alamethicin at 1.5-A resolution. Nature. 1982 Nov 25;300(5890):325–330. doi: 10.1038/300325a0. [DOI] [PubMed] [Google Scholar]
- Heremans K. High pressure effects on proteins and other biomolecules. Annu Rev Biophys Bioeng. 1982;11:1–21. doi: 10.1146/annurev.bb.11.060182.000245. [DOI] [PubMed] [Google Scholar]
- Latorre R., Alvarez O. Voltage-dependent channels in planar lipid bilayer membranes. Physiol Rev. 1981 Jan;61(1):77–150. doi: 10.1152/physrev.1981.61.1.77. [DOI] [PubMed] [Google Scholar]
- Läuger P., Stark G. Kinetics of carrier-mediated ion transport across lipid bilayer membranes. Biochim Biophys Acta. 1970 Sep 15;211(3):458–466. doi: 10.1016/0005-2736(70)90251-8. [DOI] [PubMed] [Google Scholar]
- MacNaughtan W., MacDonald A. G. Effects of gaseous anaesthetics and inert gases on the phase transition in smectic mesophases of dipalmitoyl phosphatidylcholine. Biochim Biophys Acta. 1980 Apr 10;597(2):193–198. doi: 10.1016/0005-2736(80)90098-x. [DOI] [PubMed] [Google Scholar]
- Miller K. W. Inert gas narcosis, the high pressure neurological syndrome, and the critical volume hypothesis. Science. 1974 Sep 6;185(4154):867–869. doi: 10.1126/science.185.4154.867. [DOI] [PubMed] [Google Scholar]
- Montal M., Mueller P. Formation of bimolecular membranes from lipid monolayers and a study of their electrical properties. Proc Natl Acad Sci U S A. 1972 Dec;69(12):3561–3566. doi: 10.1073/pnas.69.12.3561. [DOI] [PMC free article] [PubMed] [Google Scholar]
- Schwarz G., Savko P. Structural and dipolar properties of the voltage-dependent pore former alamethicin in octanol/dioxane. Biophys J. 1982 Aug;39(2):211–219. doi: 10.1016/S0006-3495(82)84510-4. [DOI] [PMC free article] [PubMed] [Google Scholar]
- Veatch W. The structure of the gramicidin A transmembrane channel. J Supramol Struct. 1976;5(4):431(35)–451(55). doi: 10.1002/jss.400050403. [DOI] [PubMed] [Google Scholar]
- Vodyanoy I., Hall J. E., Balasubramanian T. M. Alamethicin-induced current-voltage curve asymmetry in lipid bilayers. Biophys J. 1983 Apr;42(1):71–82. doi: 10.1016/S0006-3495(83)84370-7. [DOI] [PMC free article] [PubMed] [Google Scholar]
- White S. H. Formation of "solvent-free" black lipid bilayer membranes from glyceryl monooleate dispersed in squalene. Biophys J. 1978 Sep;23(3):337–347. doi: 10.1016/S0006-3495(78)85453-8. [DOI] [PMC free article] [PubMed] [Google Scholar]
- Yantorno R., Takashima S., Mueller P. Dipole moment of alamethicin as related to voltage-dependent conductance in lipid bilayers. Biophys J. 1982 May;38(2):105–110. doi: 10.1016/S0006-3495(82)84536-0. [DOI] [PMC free article] [PubMed] [Google Scholar]