Abstract
The rat proximal tubule epithelium is represented as well-stirred, compliant cellular and paracellular compartments bounded by mucosal and serosal bathing solutions. With a uniform pCO2 throughout the epithelium, the model variables include the concentrations of Na, K, Cl, HCO3, H2PO4, HPO4, and H, as well as hydrostatic pressure and electrical potential. Except for a metabolically driven Na-K exchanger at the basolateral cell membrane, all membrane transport within the epithelium is passive and is represented by the linear equations of nonequilibrium thermodynamics. In particular, this includes the cotransport of Na-Cl and Na-H2PO4 and countertransport of Na-H at the apical cell membrane. Experimental constraints on the choice of ionic conductivities are satisfied by allowing K-Cl cotransport at the basolateral membrane. The model equations include those for mass balance of the nonreacting species, as well as chemical equilibrium for the acidification reactions. Time-dependent terms are retained to permit the study of transient phenomena. In the steady state the energy dissipation is computed and verified equal to the sum of input from the Na-K exchanger plus the Gibbs free energy of mass addition to the system. The parameter dependence of coupled water transport is studied and shown to be consistent with the predictions of previous analytical models of the lateral intercellular space. Water transport in the presence of an end-proximal (HCO3-depleted) luminal solution is investigated. Here the lower permeability and higher reflection coefficient of HCO3 enhance net sodium and water transport. Due to enhanced flux across the tight junction, this process may permit proximal tubule Na transport to proceed with diminished energy dissipation.
Full text
PDF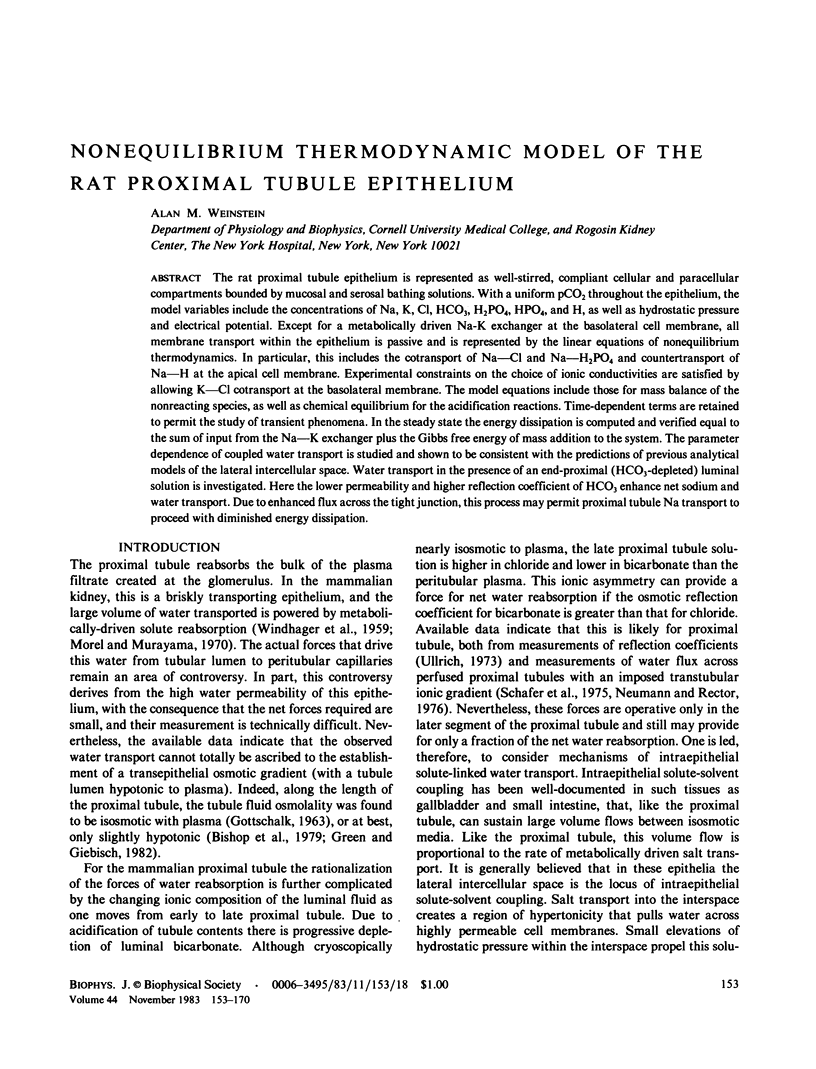
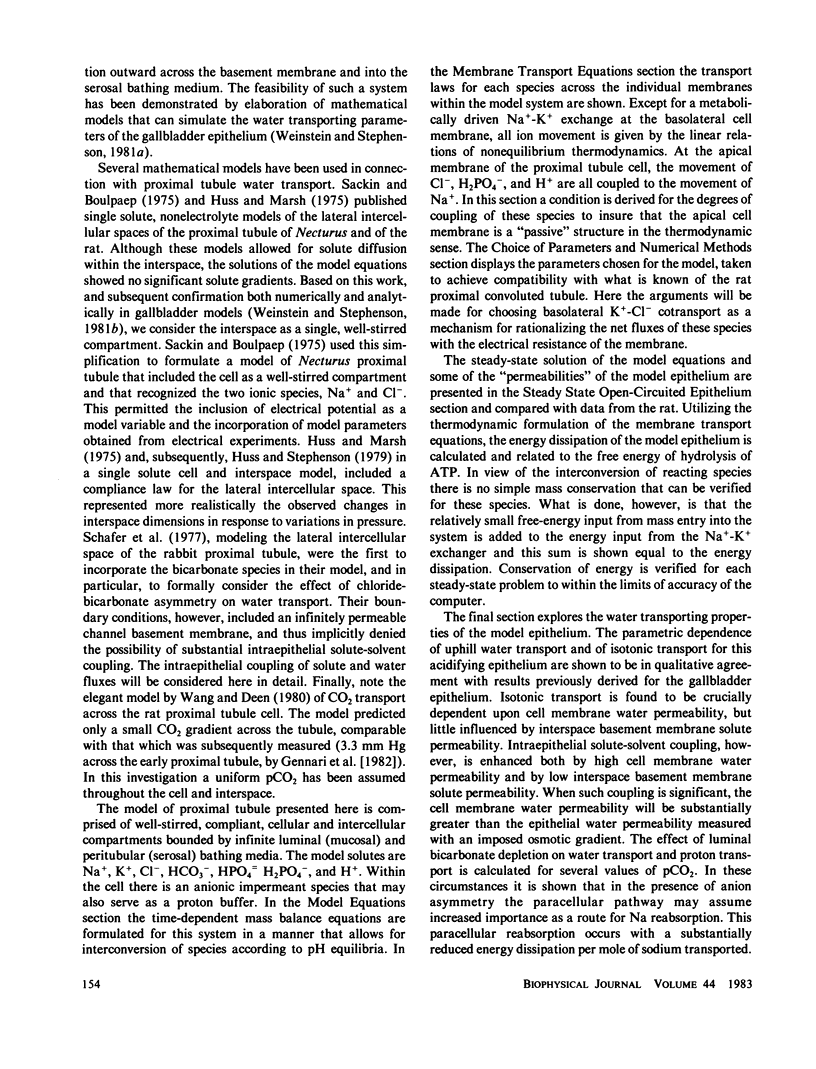
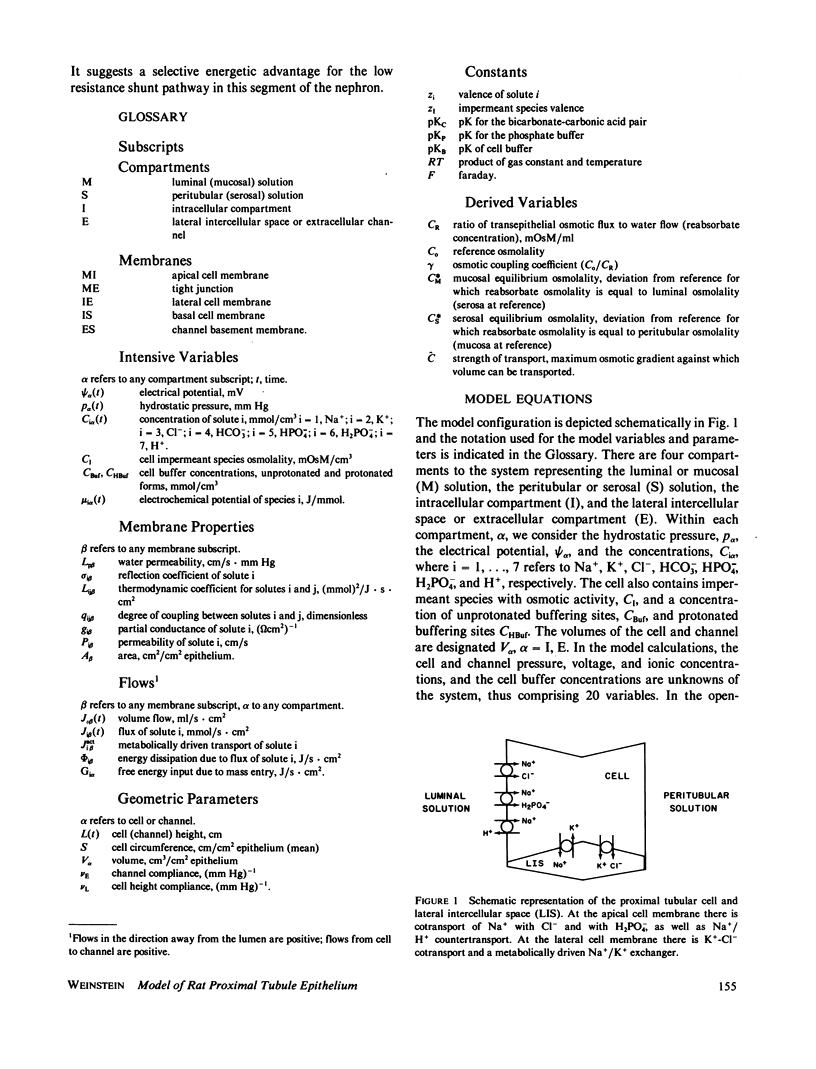
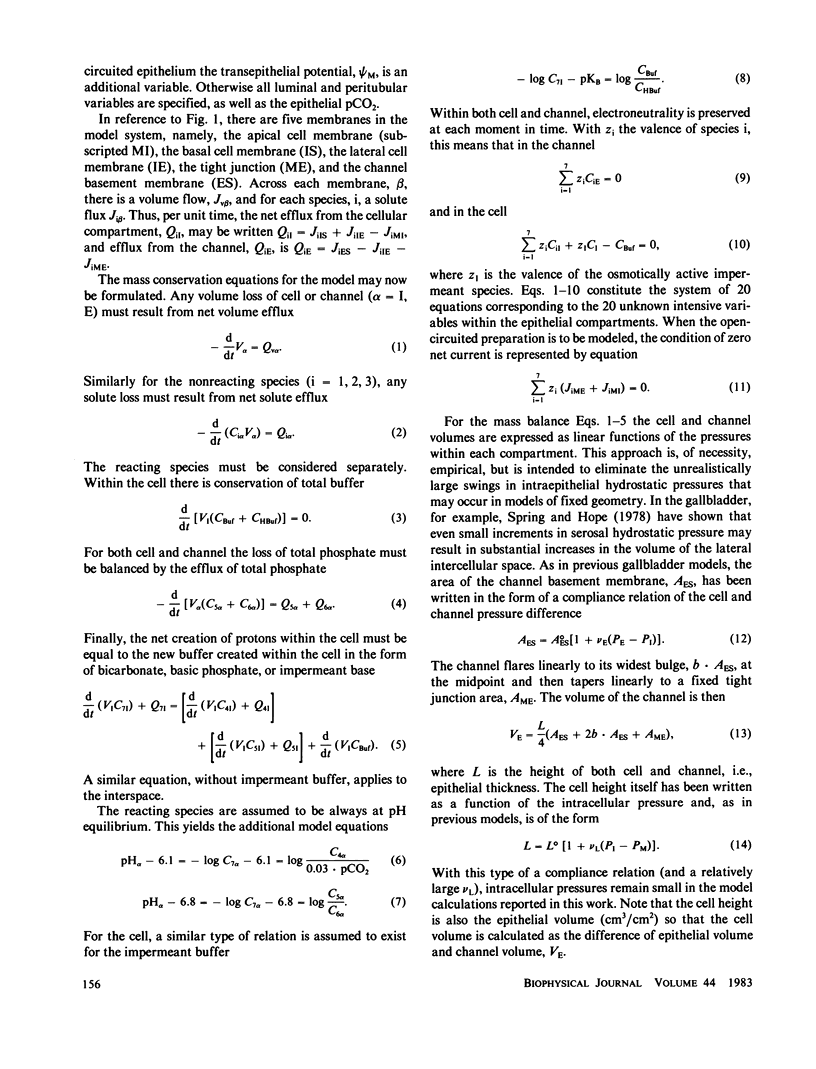
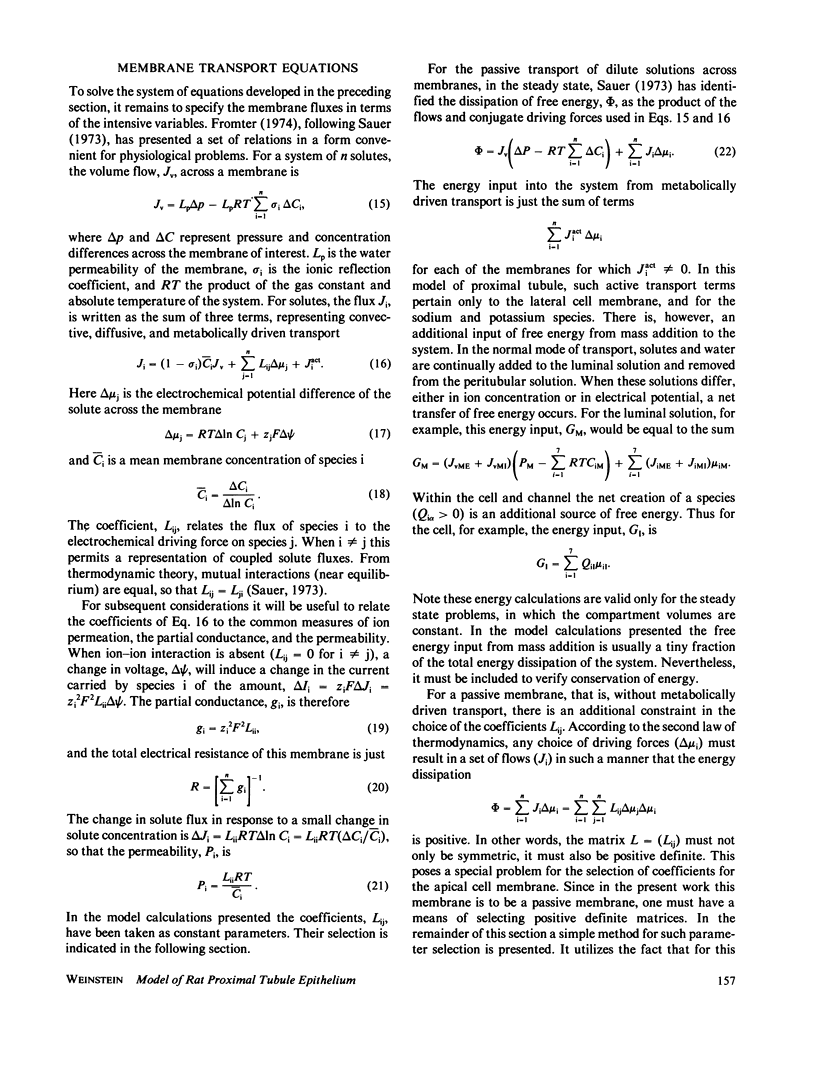
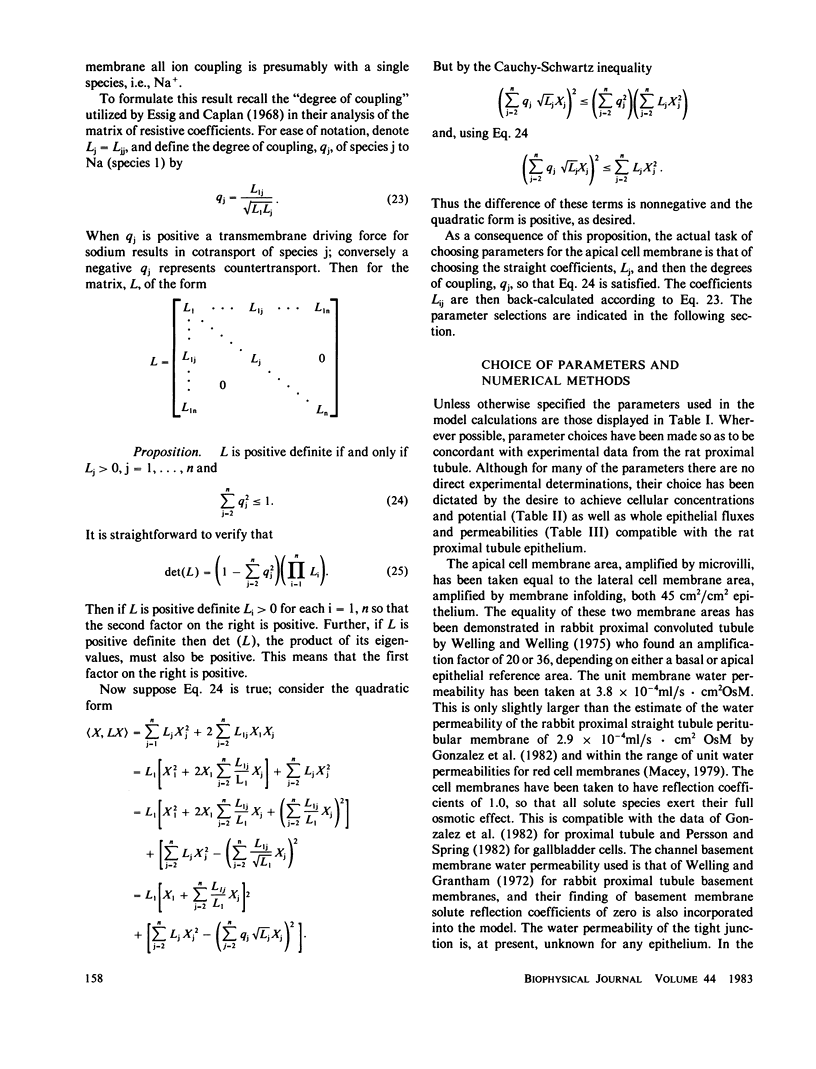
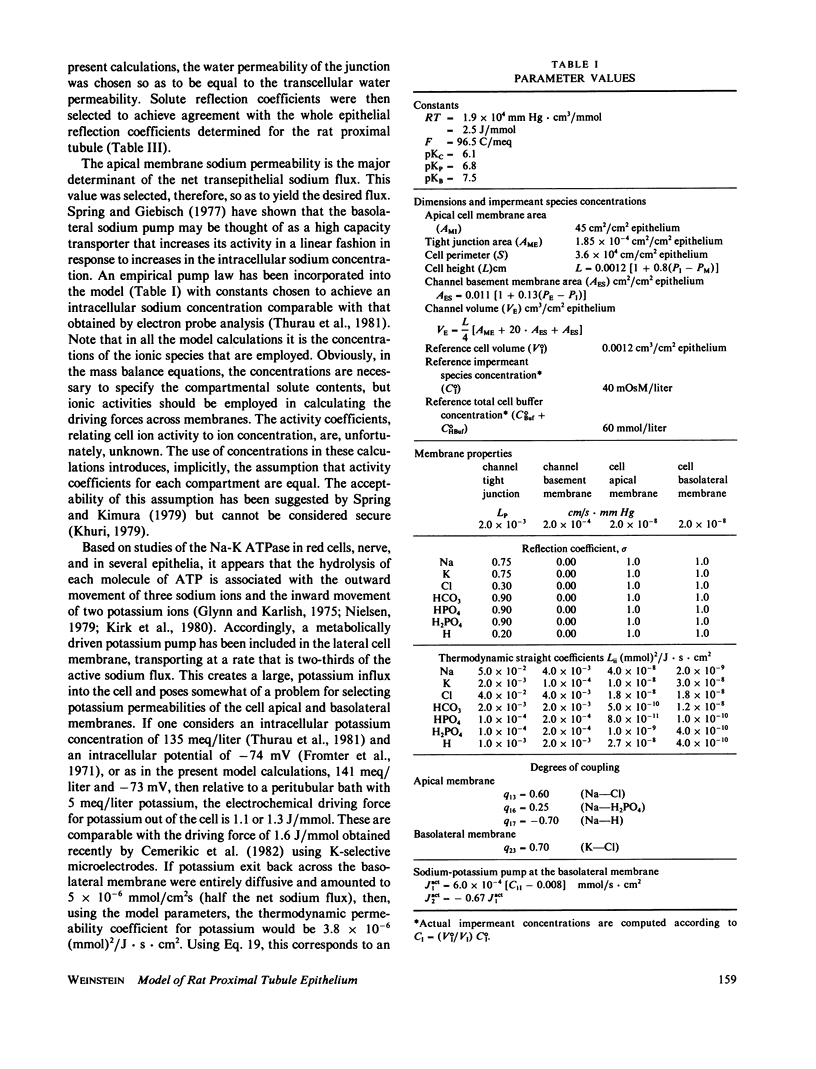
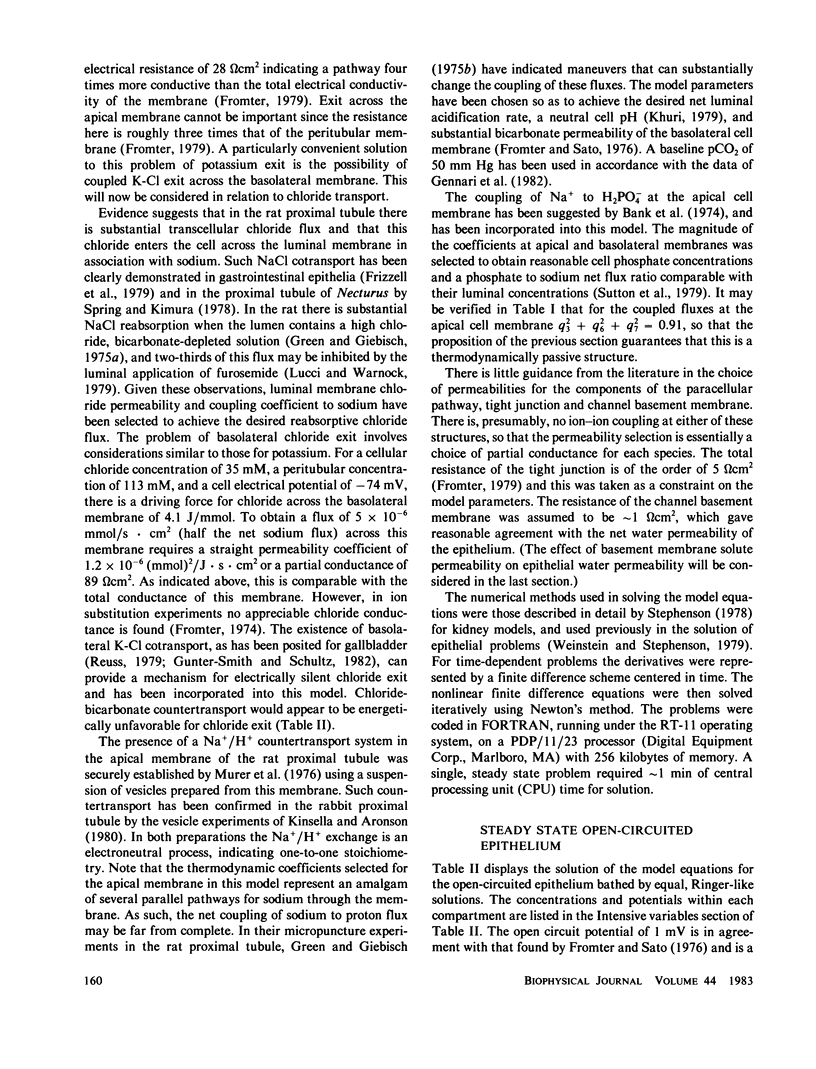
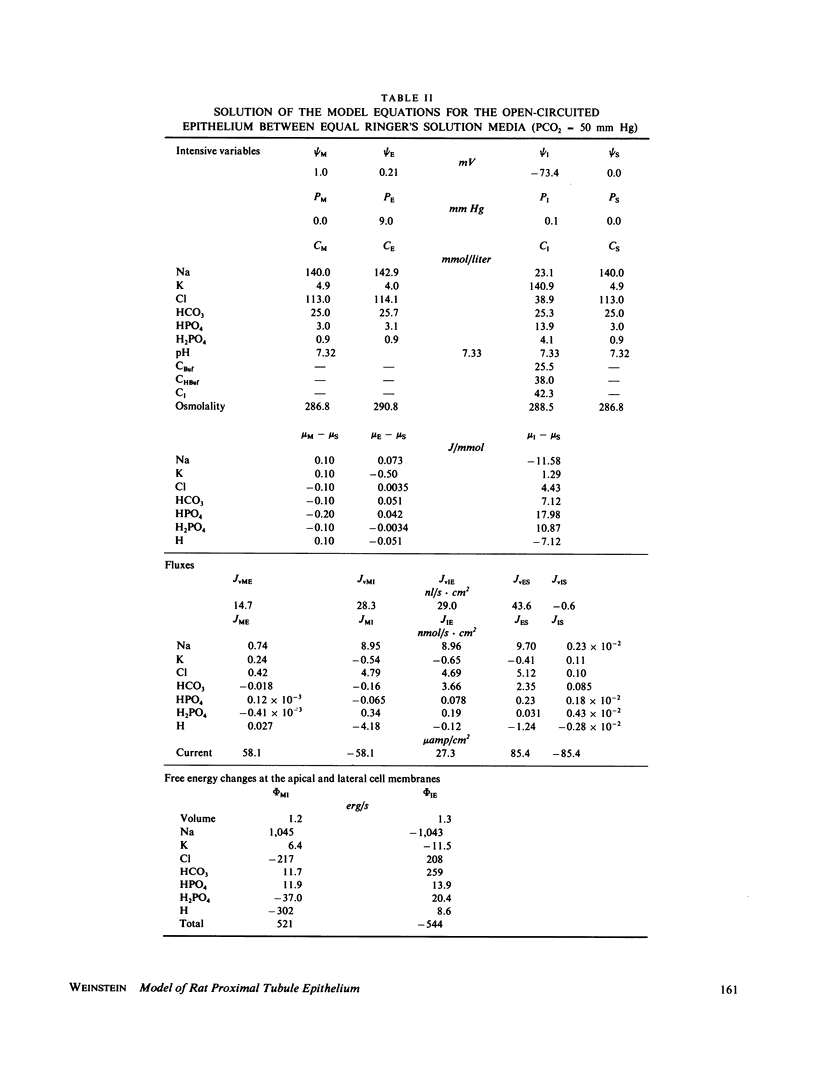
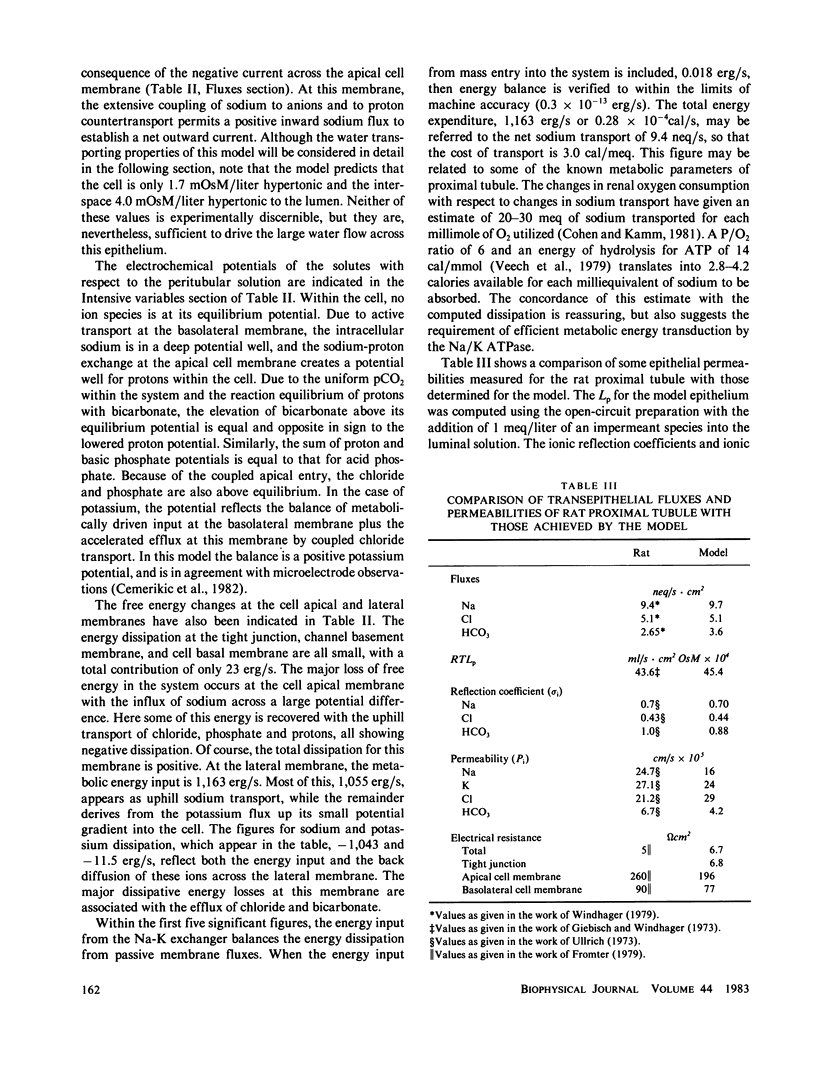
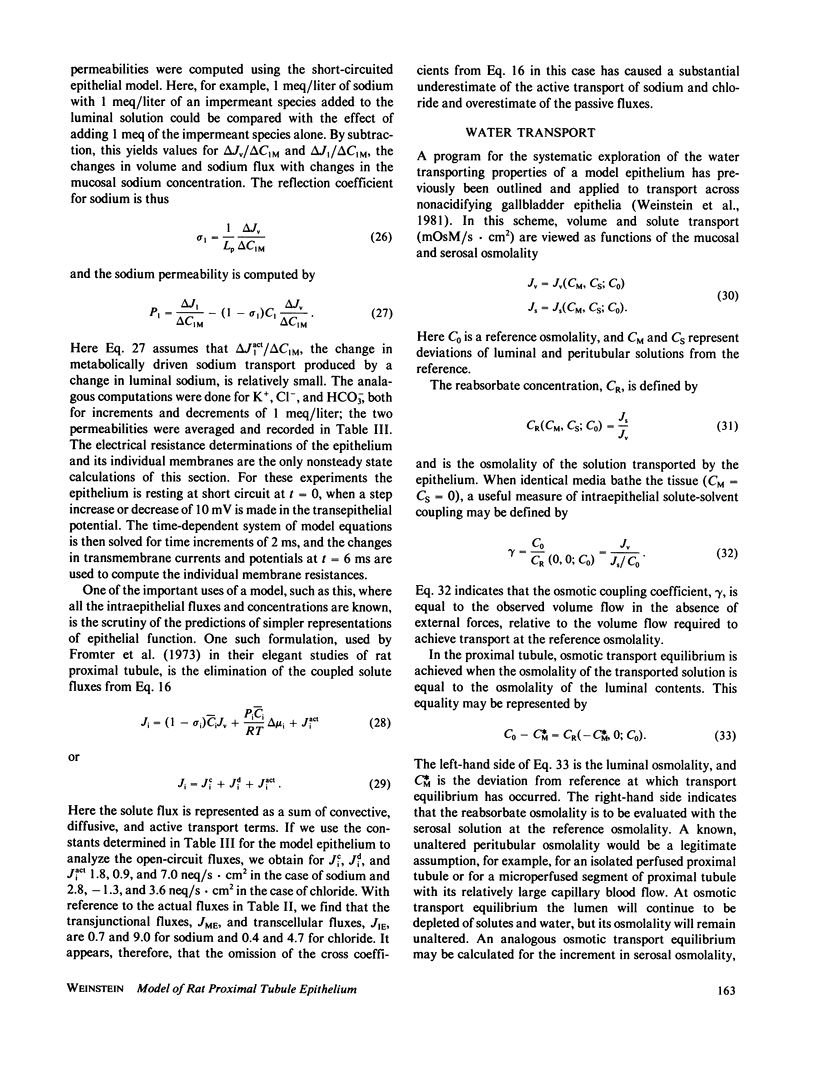
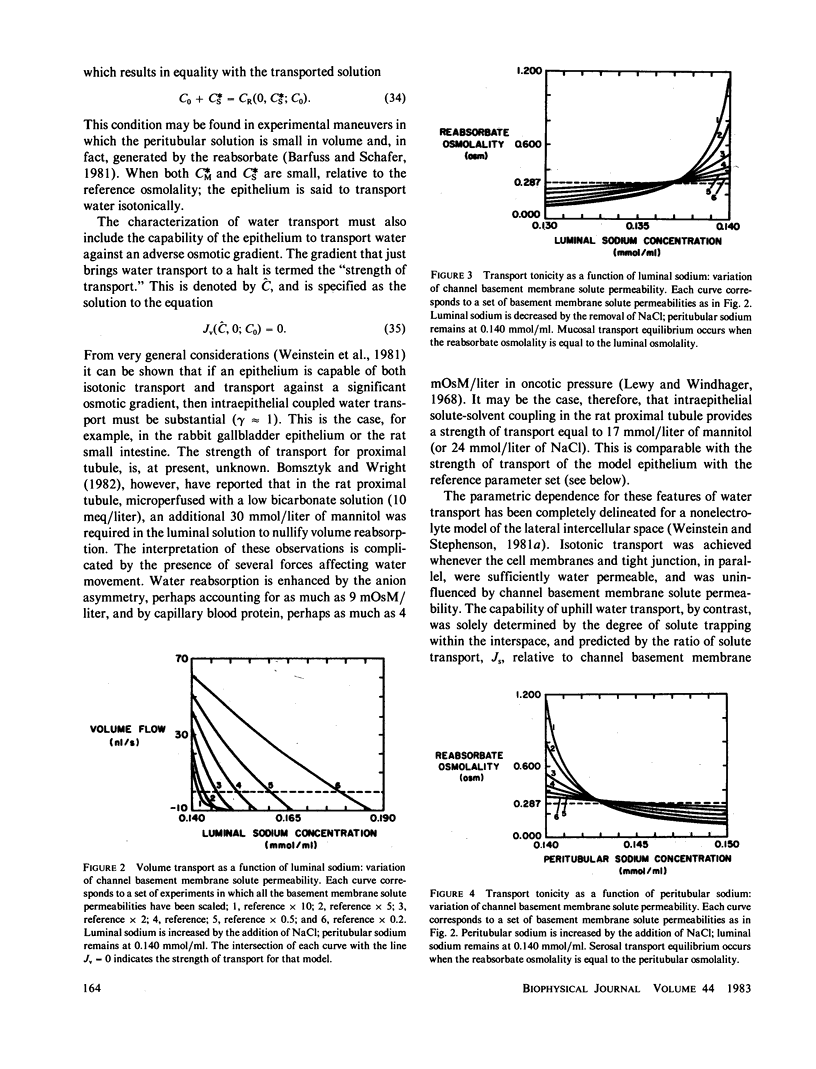
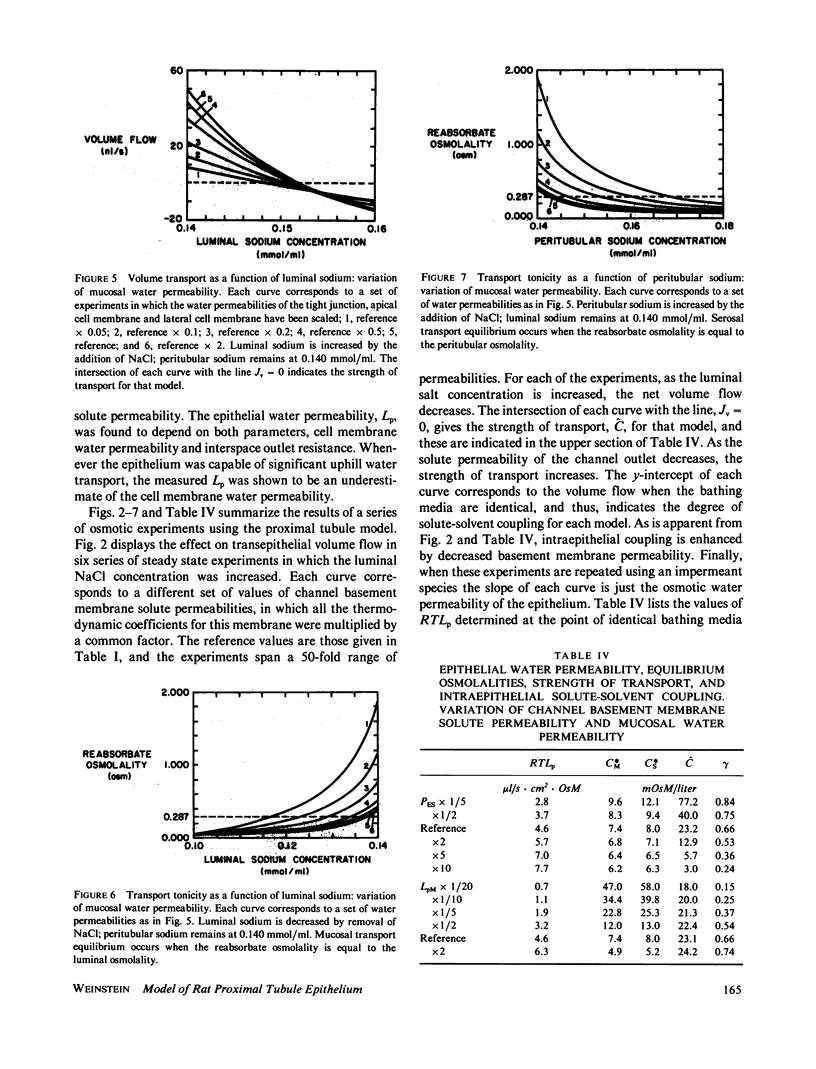
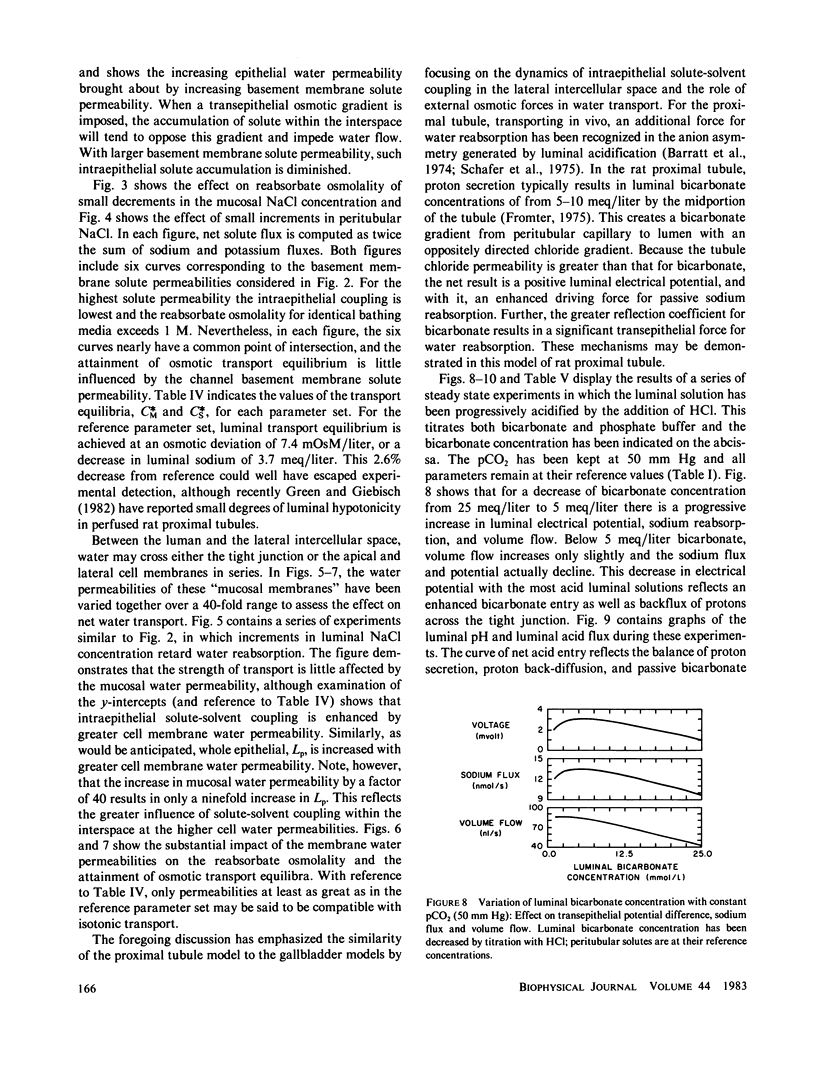
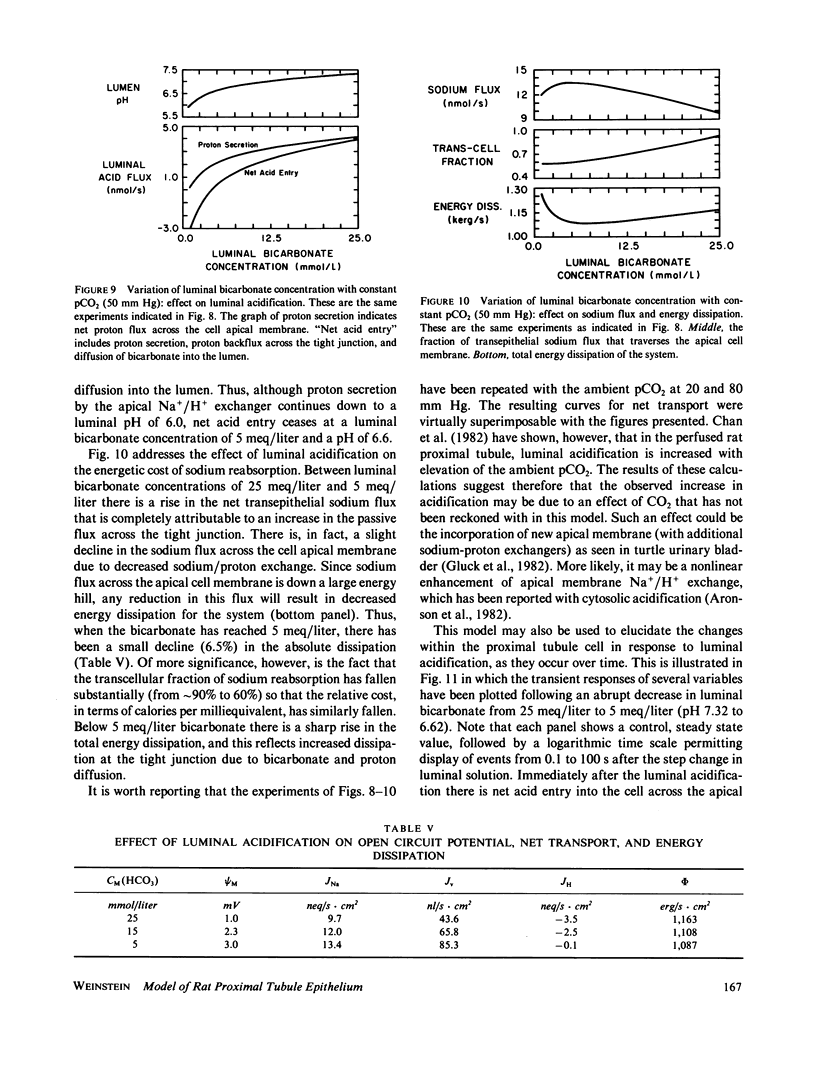
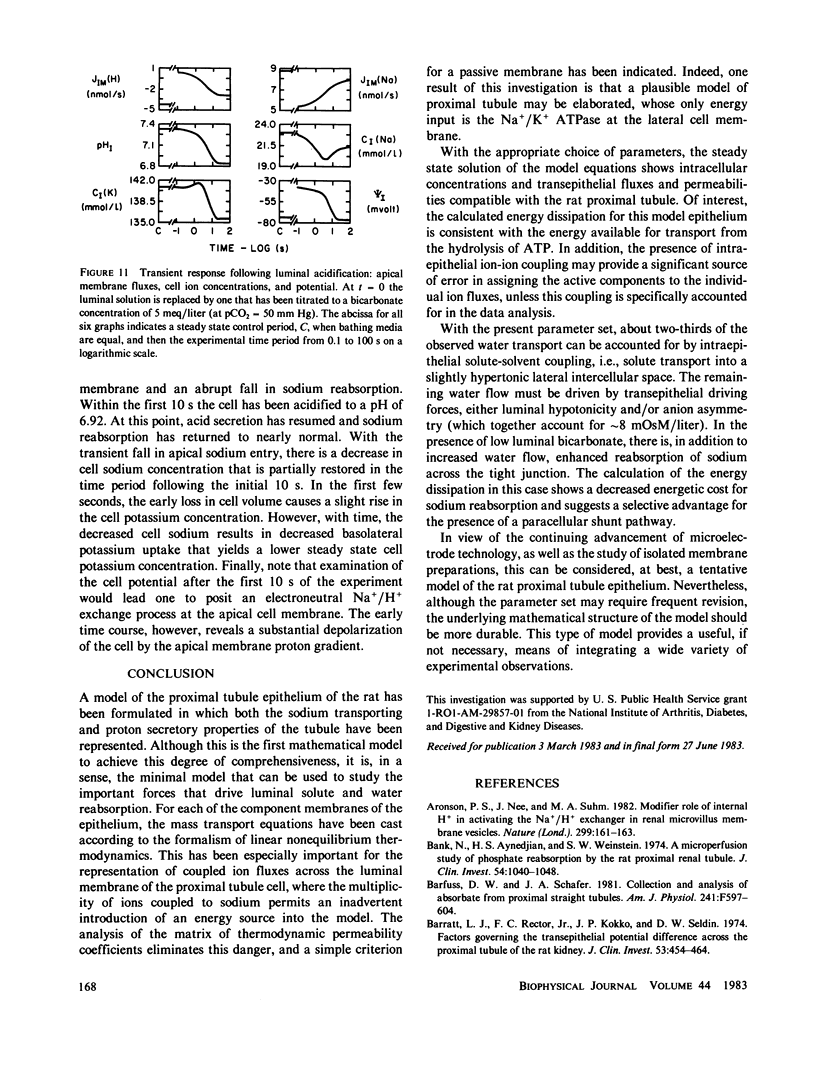
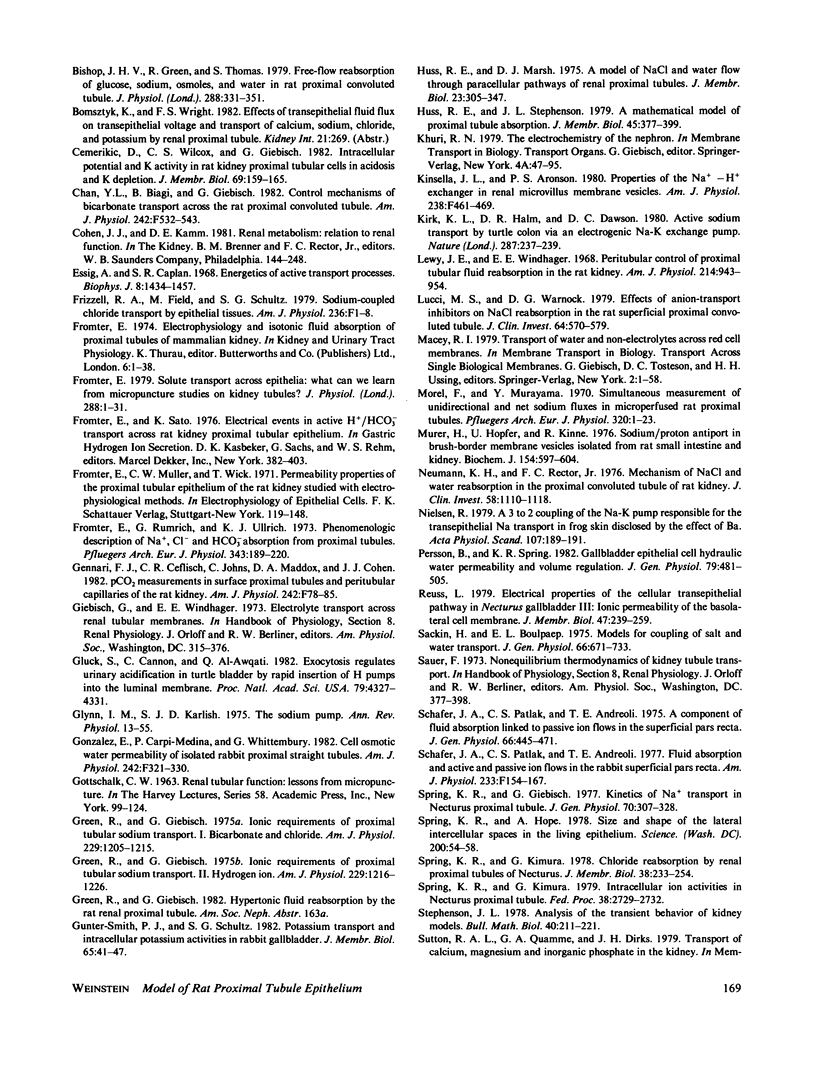
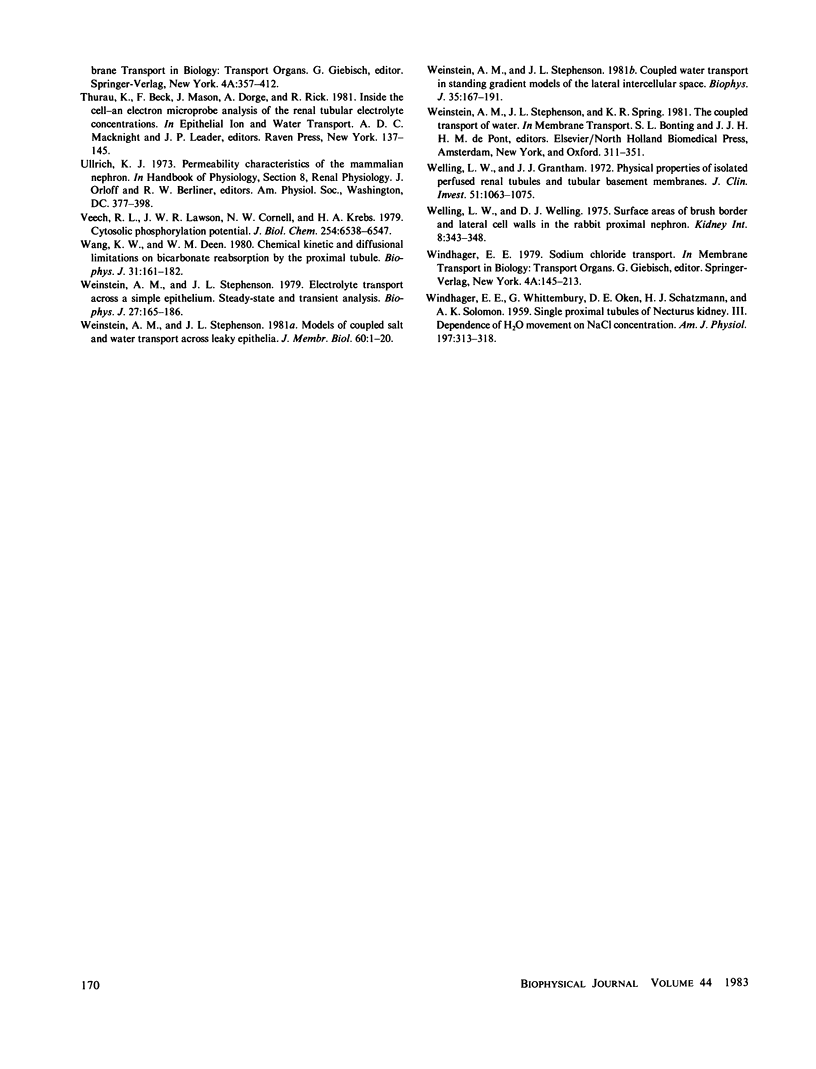
Selected References
These references are in PubMed. This may not be the complete list of references from this article.
- Aronson P. S., Nee J., Suhm M. A. Modifier role of internal H+ in activating the Na+-H+ exchanger in renal microvillus membrane vesicles. Nature. 1982 Sep 9;299(5879):161–163. doi: 10.1038/299161a0. [DOI] [PubMed] [Google Scholar]
- Bank N., Aynedjian H. S., Weinstein S. W. A microperfusion study of phosphate reabsorption by the rat proximal renal tubule. Effect of parathyroid hormone. J Clin Invest. 1974 Nov;54(5):1040–1048. doi: 10.1172/JCI107847. [DOI] [PMC free article] [PubMed] [Google Scholar]
- Barfuss D. W., Schafer J. A. Collection and analysis of absorbate from proximal straight tubules. Am J Physiol. 1981 Dec;241(6):F597–F604. doi: 10.1152/ajprenal.1981.241.6.F597. [DOI] [PubMed] [Google Scholar]
- Barratt L. J., Rector F. C., Jr, Kokko J. P., Seldin D. W. Factors governing the transepithelial potential difference across the proximal tubule of the rat kidney. J Clin Invest. 1974 Feb;53(2):454–464. doi: 10.1172/JCI107579. [DOI] [PMC free article] [PubMed] [Google Scholar]
- Bishop J. H., Green R., Thomas S. Free-flow reabsorption of glucose, sodium, osmoles and water in rat proximal convoluted tubule. J Physiol. 1979 Mar;288:331–351. [PMC free article] [PubMed] [Google Scholar]
- Cemerikić D., Wilcox C. S., Giebisch G. Intracellular potential and K+ activity in rat kidney proximal tubular cells in acidosis and K+ depletion. J Membr Biol. 1982;69(2):159–165. doi: 10.1007/BF01872275. [DOI] [PubMed] [Google Scholar]
- Chan Y. L., Biagi B., Giebisch G. Control mechanisms of bicarbonate transport across the rat proximal convoluted tubule. Am J Physiol. 1982 May;242(5):F532–F543. doi: 10.1152/ajprenal.1982.242.5.F532. [DOI] [PubMed] [Google Scholar]
- Essig A., Caplan S. R. Energetics of active transport processes. Biophys J. 1968 Dec;8(12):1434–1457. doi: 10.1016/S0006-3495(68)86565-8. [DOI] [PMC free article] [PubMed] [Google Scholar]
- Frizzell R. A., Field M., Schultz S. G. Sodium-coupled chloride transport by epithelial tissues. Am J Physiol. 1979 Jan;236(1):F1–F8. doi: 10.1152/ajprenal.1979.236.1.F1. [DOI] [PubMed] [Google Scholar]
- Frömter E., Rumrich G., Ullrich K. J. Phenomenologic description of Na+, Cl- and HCO-3 absorption from proximal tubules of rat kidney. Pflugers Arch. 1973 Oct 22;343(3):189–220. doi: 10.1007/BF00586045. [DOI] [PubMed] [Google Scholar]
- Frömter E. The Feldberg Lecture 1976. Solute transport across epithelia: what can we learn from micropuncture studies in kidney tubules? J Physiol. 1979 Mar;288:1–31. [PMC free article] [PubMed] [Google Scholar]
- GOTTSCHALK C. W. RENAL TUBULAR FUNCTION: LESSONS FROM MICROPUNCTURE. Harvey Lect. 1963;58:99–124. [PubMed] [Google Scholar]
- Gluck S., Cannon C., Al-Awqati Q. Exocytosis regulates urinary acidification in turtle bladder by rapid insertion of H+ pumps into the luminal membrane. Proc Natl Acad Sci U S A. 1982 Jul;79(14):4327–4331. doi: 10.1073/pnas.79.14.4327. [DOI] [PMC free article] [PubMed] [Google Scholar]
- Glynn I. M., Karlish S. J. The sodium pump. Annu Rev Physiol. 1975;37:13–55. doi: 10.1146/annurev.ph.37.030175.000305. [DOI] [PubMed] [Google Scholar]
- González E., Carpi-Medina P., Whittembury G. Cell osmotic water permeability of isolated rabbit proximal straight tubules. Am J Physiol. 1982 Apr;242(4):F321–F330. doi: 10.1152/ajprenal.1982.242.4.F321. [DOI] [PubMed] [Google Scholar]
- Green R., Giebisch G. Ionic requirements of proximal tubular sodium transport. I. Bicarbonate and chloride. Am J Physiol. 1975 Nov;229(5):1205–1215. doi: 10.1152/ajplegacy.1975.229.5.1205. [DOI] [PubMed] [Google Scholar]
- Green R., Giebisch G. Ionic requirements of proximal tubular sodium transport. II. Hydrogen ion. Am J Physiol. 1975 Nov;229(5):1216–1226. doi: 10.1152/ajplegacy.1975.229.5.1216. [DOI] [PubMed] [Google Scholar]
- Gunter-Smith P. J., Schultz S. G. Potassium transport and intracellular potassium activities in rabbit gallbladder. J Membr Biol. 1982;65(1-2):41–47. doi: 10.1007/BF01870467. [DOI] [PubMed] [Google Scholar]
- Huss R. E., Marsh D. J. A model of NaCl and water flow through paracellular pathways of renal proximal tubules. J Membr Biol. 1975;23(3-4):305–347. doi: 10.1007/BF01870256. [DOI] [PubMed] [Google Scholar]
- Huss R. E., Stephenson J. L. A mathematical model of proximal tubule absorption. J Membr Biol. 1979 Jun 7;47(4):377–399. doi: 10.1007/BF01869745. [DOI] [PubMed] [Google Scholar]
- Kinsella J. L., Aronson P. S. Properties of the Na+-H+ exchanger in renal microvillus membrane vesicles. Am J Physiol. 1980 Jun;238(6):F461–F469. doi: 10.1152/ajprenal.1980.238.6.F461. [DOI] [PubMed] [Google Scholar]
- Kirk K. L., Halm D. R., Dawson D. C. Active sodium transport by turtle colon via an electrogenic Na-K exchange pump. Nature. 1980 Sep 18;287(5779):237–239. doi: 10.1038/287237a0. [DOI] [PubMed] [Google Scholar]
- Lewy J. E., Windhager E. E. Peritubular control of proximal tubular fluid reabsorption in the rat kidney. Am J Physiol. 1968 May;214(5):943–954. doi: 10.1152/ajplegacy.1968.214.5.943. [DOI] [PubMed] [Google Scholar]
- Lucci M. S., Warnock D. G. Effects of anion-transport inhibitors on NaCl reabsorption in the rat superficial proximal convoluted tubule. J Clin Invest. 1979 Aug;64(2):570–579. doi: 10.1172/JCI109495. [DOI] [PMC free article] [PubMed] [Google Scholar]
- Morel F., Murayama Y. Simultaneous measurement of undirectional and net sodium fluxes in microperfused rat proximal tubules. Pflugers Arch. 1970;320(1):1–23. doi: 10.1007/BF00588454. [DOI] [PubMed] [Google Scholar]
- Murer H., Hopfer U., Kinne R. Sodium/proton antiport in brush-border-membrane vesicles isolated from rat small intestine and kidney. Biochem J. 1976 Mar 15;154(3):597–604. [PMC free article] [PubMed] [Google Scholar]
- Neumann K. H., Rector F. C., Jr Mechanism of NaCl and water reabsorption in the proximal convoluted tubule of rat kidney. J Clin Invest. 1976 Nov;58(5):1110–1118. doi: 10.1172/JCI108563. [DOI] [PMC free article] [PubMed] [Google Scholar]
- Nielsen R. A 3 to 2 coupling of the Na-K pump responsible for the transepithelial Na transport in frog skin disclosed by the effect of Ba. Acta Physiol Scand. 1979 Oct;107(2):189–191. doi: 10.1111/j.1748-1716.1979.tb06462.x. [DOI] [PubMed] [Google Scholar]
- Persson B. E., Spring K. R. Gallbladder epithelial cell hydraulic water permeability and volume regulation. J Gen Physiol. 1982 Mar;79(3):481–505. doi: 10.1085/jgp.79.3.481. [DOI] [PMC free article] [PubMed] [Google Scholar]
- Reuss L. Electrical properties of the cellular transepithelial pathway in Necturus gallbladder: III. Ionic permeability of the basolateral cell membrane. J Membr Biol. 1979 May 25;47(3):239–259. doi: 10.1007/BF01869080. [DOI] [PubMed] [Google Scholar]
- Sackin H., Boulpaep E. L. Models for coupling of salt and water transport; Proximal tubular reabsorption in Necturus kidney. J Gen Physiol. 1975 Dec;66(6):671–733. doi: 10.1085/jgp.66.6.671. [DOI] [PMC free article] [PubMed] [Google Scholar]
- Schafer J. A., Patlak C. S., Andreoli T. E. A component of fluid absorption linked to passive ion flows in the superficial pars recta. J Gen Physiol. 1975 Oct;66(4):445–471. doi: 10.1085/jgp.66.4.445. [DOI] [PMC free article] [PubMed] [Google Scholar]
- Schafer J. A., Patlak C. S., Andreoli T. E. Fluid absorption and active and passive ion flows in the rabbit superficial pars recta. Am J Physiol. 1977 Aug;233(2):F154–F167. doi: 10.1152/ajprenal.1977.233.2.F154. [DOI] [PubMed] [Google Scholar]
- Spring K. R., Giebisch G. Kinetics of Na+ transport in Necturus proximal tubule. J Gen Physiol. 1977 Sep;70(3):307–328. doi: 10.1085/jgp.70.3.307. [DOI] [PMC free article] [PubMed] [Google Scholar]
- Spring K. R., Hope A. Size and shape of the lateral intercellular spaces in a living epithelium. Science. 1978 Apr 7;200(4337):54–58. doi: 10.1126/science.635571. [DOI] [PubMed] [Google Scholar]
- Spring K. R., Kimura G. Chloride reabsorption by renal proximal tubules of Necturus. J Membr Biol. 1978 Jan 18;38(3):233–254. doi: 10.1007/BF01871924. [DOI] [PubMed] [Google Scholar]
- Spring K. R., Kimura G. Intracellular ion activities in Necturus proximal tubule. Fed Proc. 1979 Dec;38(13):2729–2732. [PubMed] [Google Scholar]
- Stephenson J. L. Analysis of the transient behavior of kidney models. Bull Math Biol. 1978;40(2):211–221. doi: 10.1007/BF02461436. [DOI] [PubMed] [Google Scholar]
- Veech R. L., Lawson J. W., Cornell N. W., Krebs H. A. Cytosolic phosphorylation potential. J Biol Chem. 1979 Jul 25;254(14):6538–6547. [PubMed] [Google Scholar]
- WINDHAGER E. E., WHITTEMBURY G., OKEN D. E., SCHATZMANN H. J., SOLOMON A. K. Single proximal tubules of the Necturus kidney. III. Dependence of H2O movement on NaCl concentration. Am J Physiol. 1959 Aug;197:313–318. doi: 10.1152/ajplegacy.1959.197.2.313. [DOI] [PubMed] [Google Scholar]
- Wang K. W., Deen W. M. Chemical kinetic and diffusional limitations on bicarbonate reabsorption by the proximal tubule. Biophys J. 1980 Aug;31(2):161–182. doi: 10.1016/S0006-3495(80)85048-X. [DOI] [PMC free article] [PubMed] [Google Scholar]
- Weinstein A. M., Stephenson J. L. Coupled water transport in standing gradient models of the lateral intercellular space. Biophys J. 1981 Jul;35(1):167–191. doi: 10.1016/S0006-3495(81)84781-9. [DOI] [PMC free article] [PubMed] [Google Scholar]
- Weinstein A. M., Stephenson J. L. Electrolyte transport across a simple epithelium. Steady-state and transient analysis. Biophys J. 1979 Aug;27(2):165–186. doi: 10.1016/S0006-3495(79)85209-1. [DOI] [PMC free article] [PubMed] [Google Scholar]
- Weinstein A. M., Stephenson J. L. Models of coupled salt and water transport across leaky epithelia. J Membr Biol. 1981 May 15;60(1):1–20. doi: 10.1007/BF01870828. [DOI] [PubMed] [Google Scholar]
- Welling L. W., Grantham J. J. Physical properties of isolated perfused renal tubules and tubular basement membranes. J Clin Invest. 1972 May;51(5):1063–1075. doi: 10.1172/JCI106898. [DOI] [PMC free article] [PubMed] [Google Scholar]
- Welling L. W., Welling D. J. Surface areas of brush border and lateral cell walls in the rabbit proximal nephron. Kidney Int. 1975 Dec;8(6):343–348. doi: 10.1038/ki.1975.125. [DOI] [PubMed] [Google Scholar]