Abstract
Many local anesthetics promote reduction in sodium current during repetitive stimulation of excitable membranes. Use-, frequency-, and voltage-dependent responses describe patterns of peak INa when pulse width, pulse frequency, and pulse amplitude are varied. Such responses can be viewed as reflecting voltage-sensitive shifts in equilibrium between conducting, unblocked channels and nonconducting, blocked channels. The modulated-receptor hypothesis postulates shifts in equilibrium as the result of a variable-affinity receptor and modified inactivation gate kinetics in drug-complexed channels. An alternative view considers drug blocking in the absence of these two features. We propose that drug binds to a constant-affinity channel receptor where receptor access is regulated by the channel gates. Specifically, we view channel binding sites as guarded by the channel gate conformation, so that unlike receptors where ligands have continuous access, blocking agent access is variable during the course of an action potential. During the course of an action potential, the m and h gates change conformation in response to transmembrane potential. Conducting channels with both gates open leave the binding site unguarded and thus accessible to drug, whereas nonconducting channels, with gates in the closed conformation, act to restrict drug access to unbound receptors and possibly to trap drug in drug-complexed channels. We develop analytical expressions characterizing guarded receptors as "apparently" variable-affinity binding sites and predicting shifts in "apparent" channel inactivation in the hyperpolarizing direction. These results were confirmed with computer simulations. Furthermore, these results are in quantitative agreement with recent investigations of lidocaine binding in cardiac sodium channels.
Full text
PDF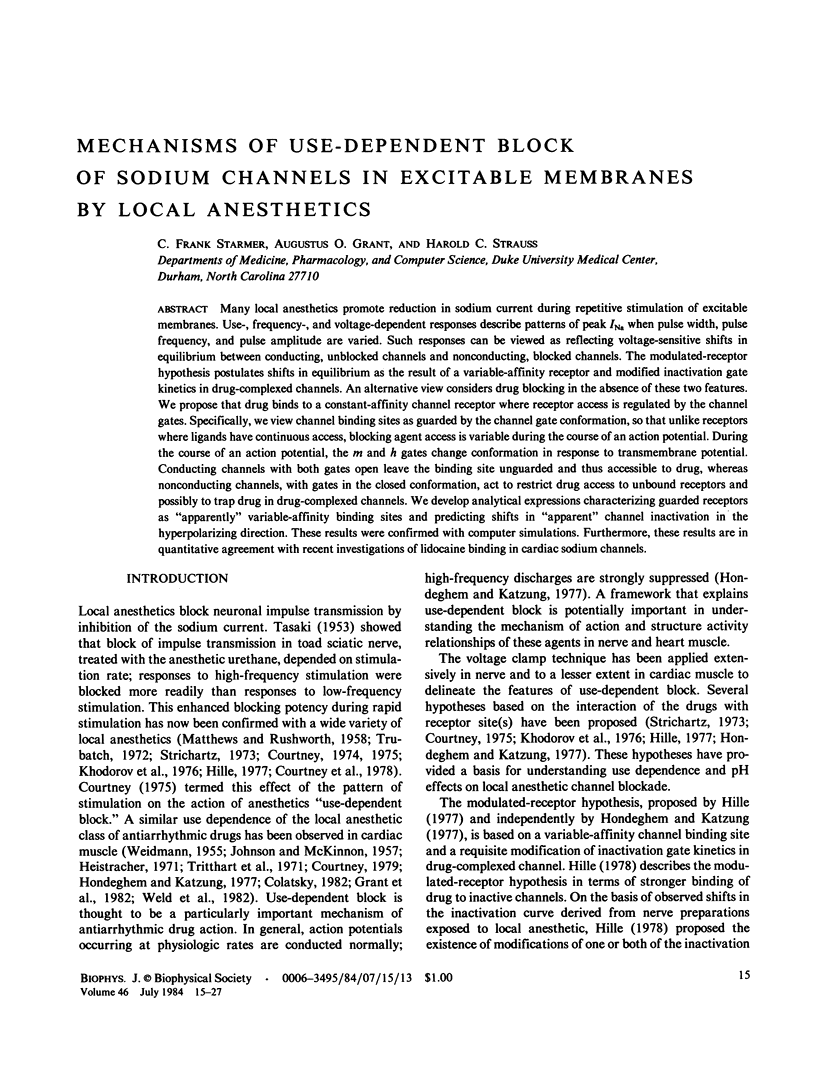
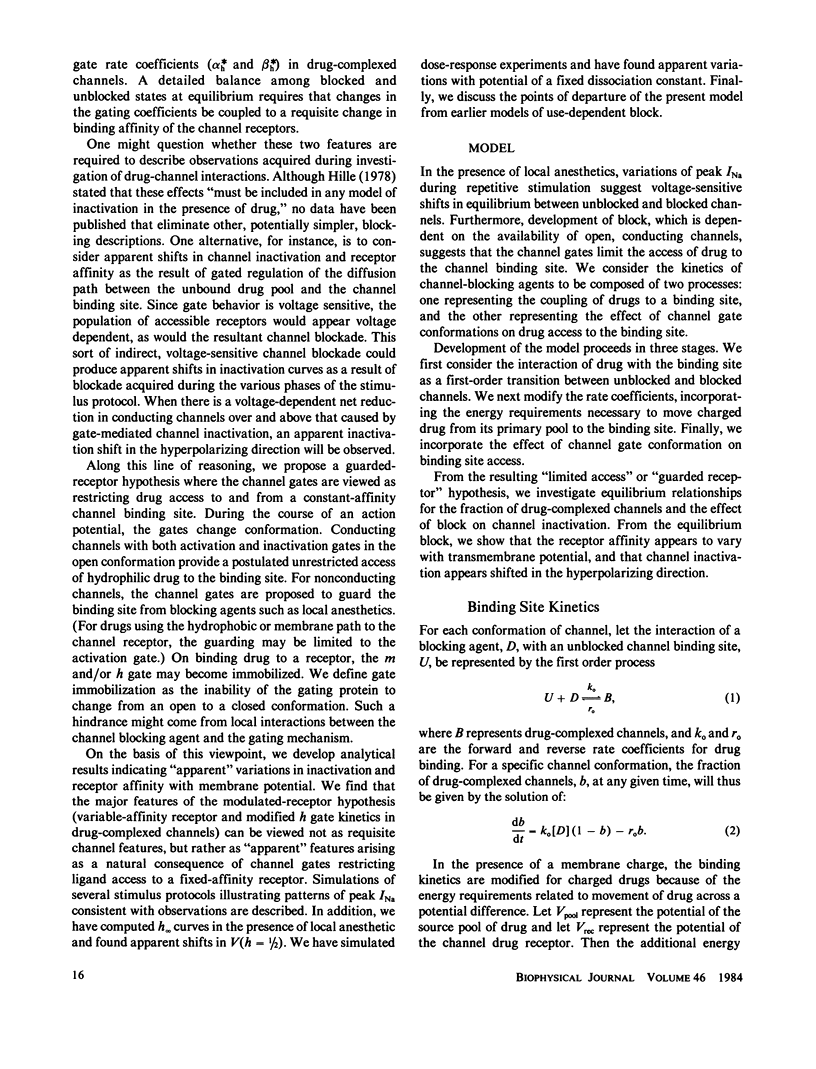
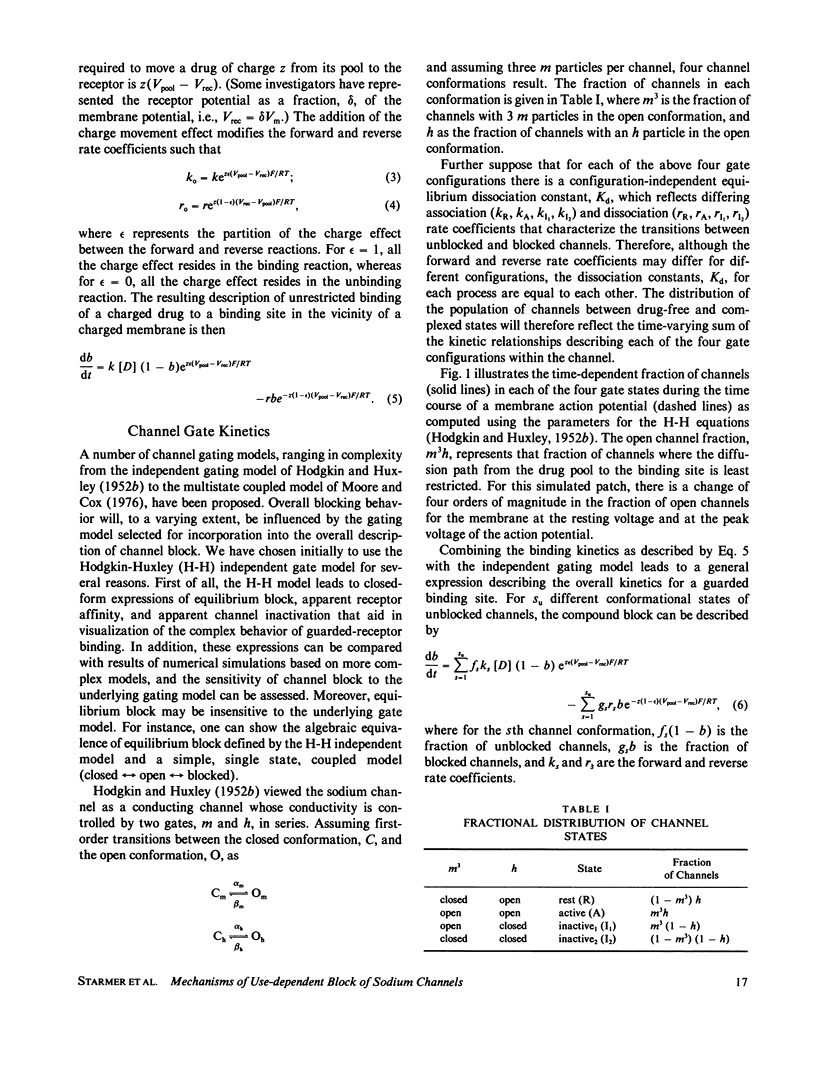
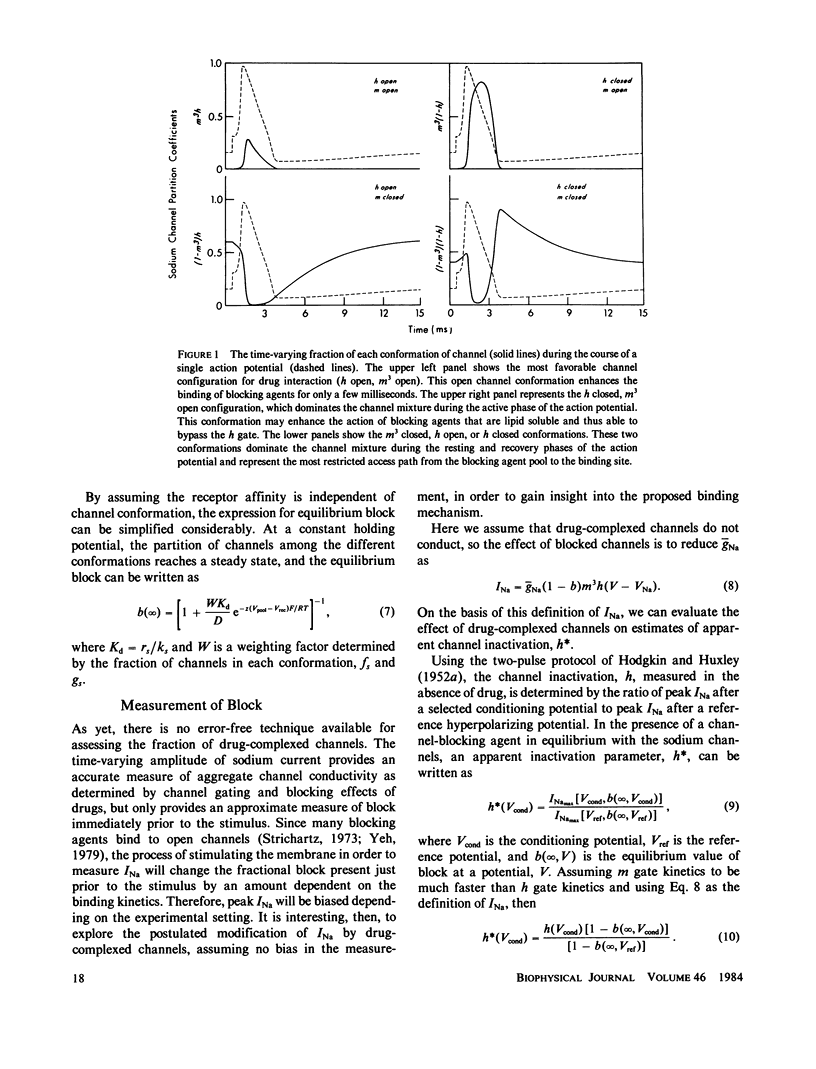
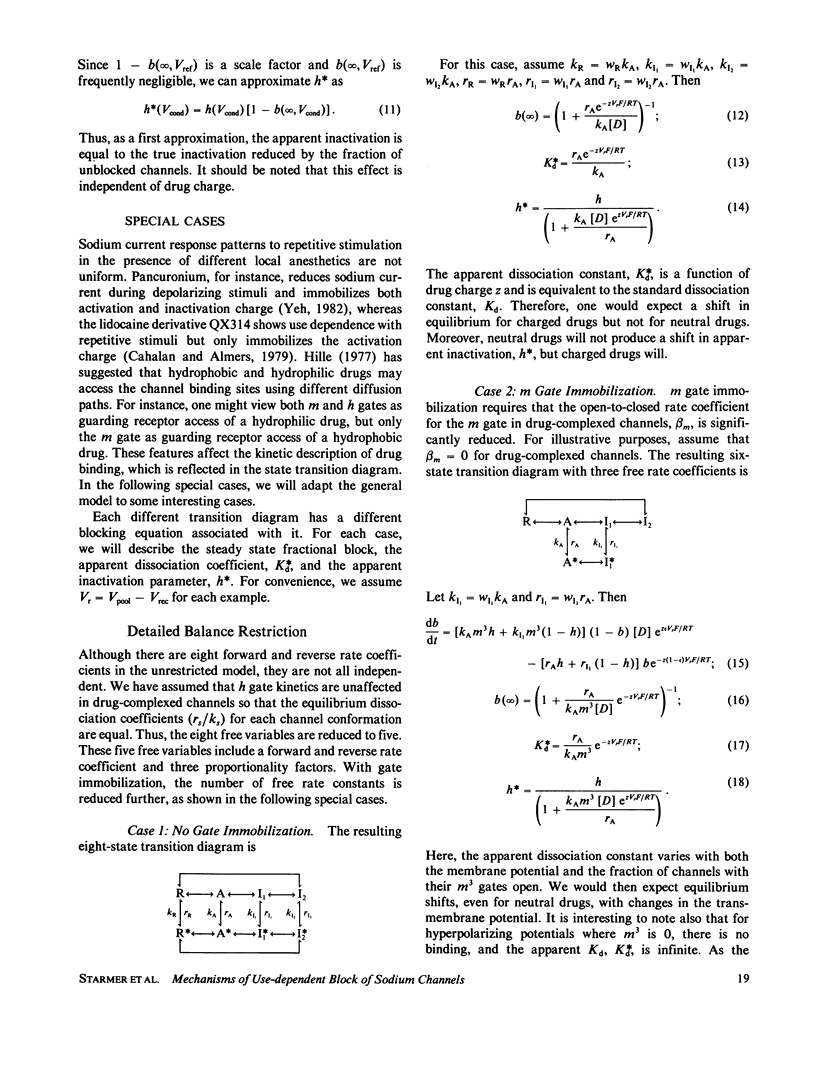
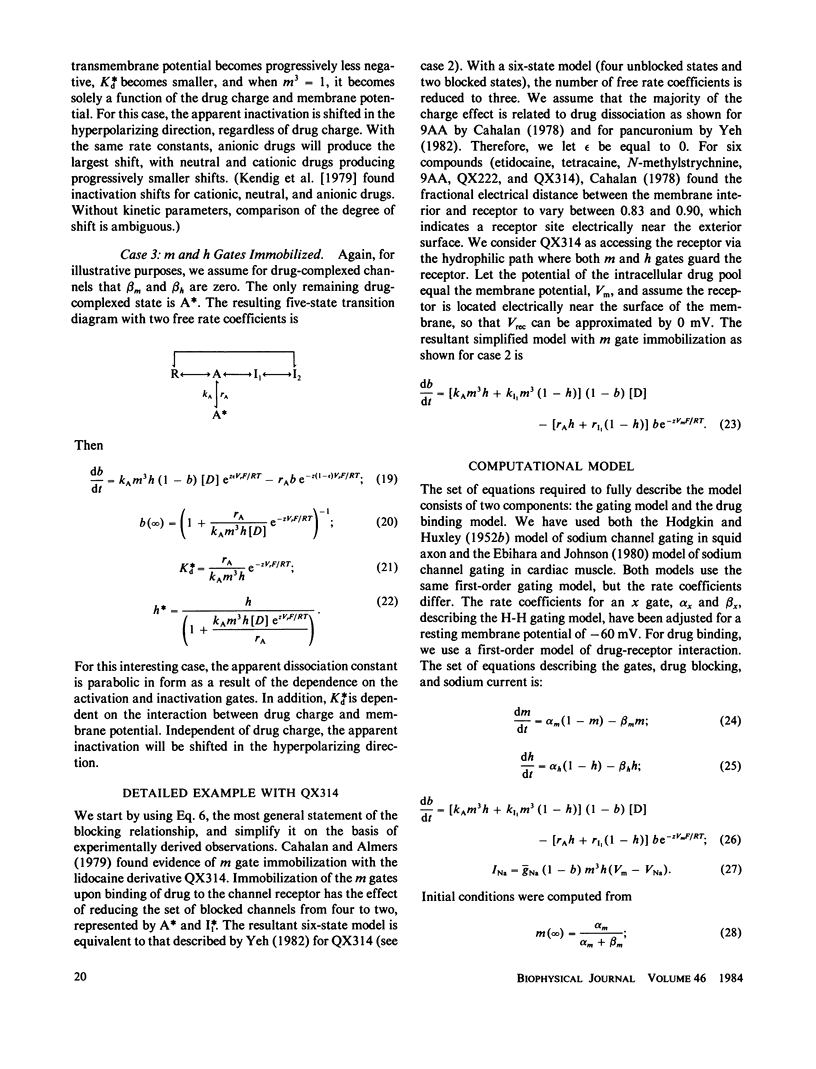
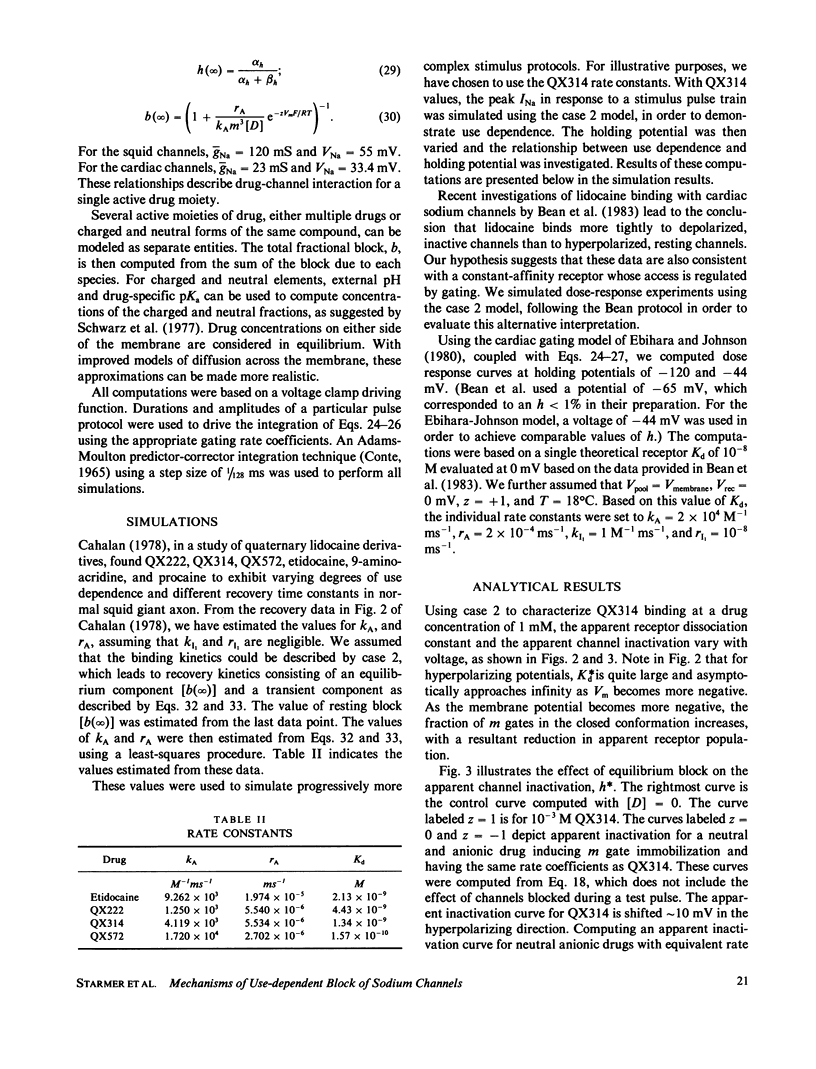
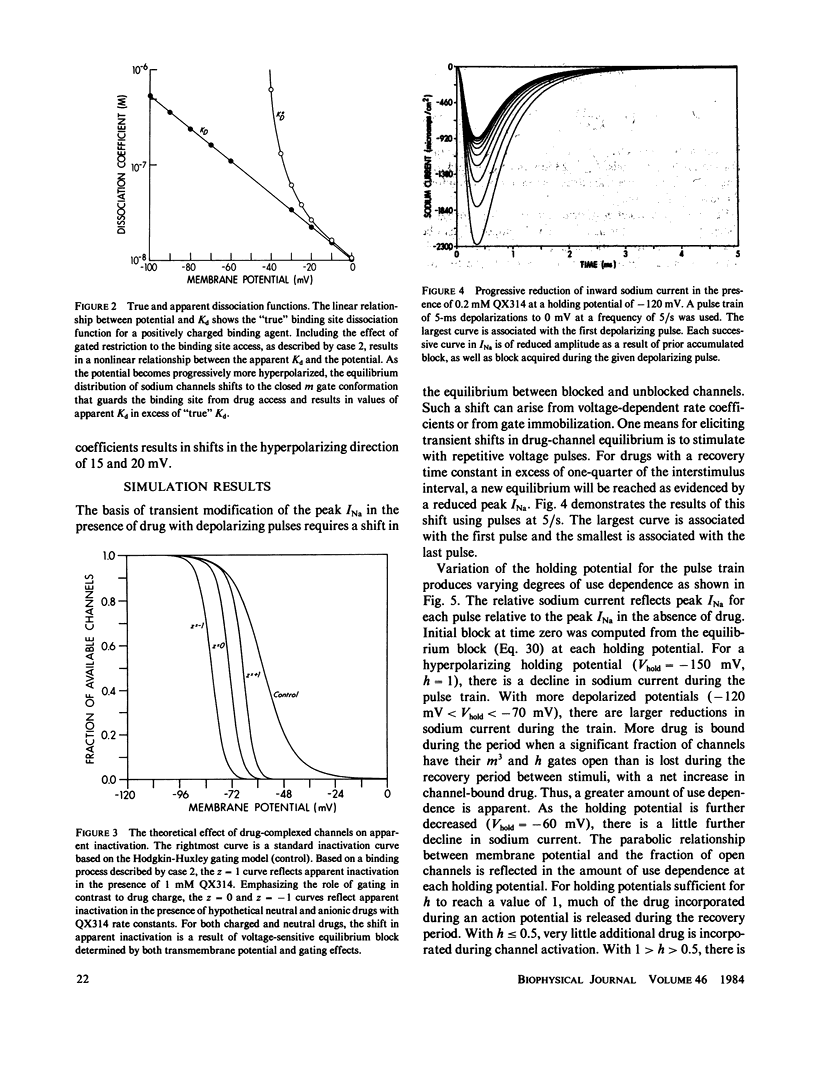
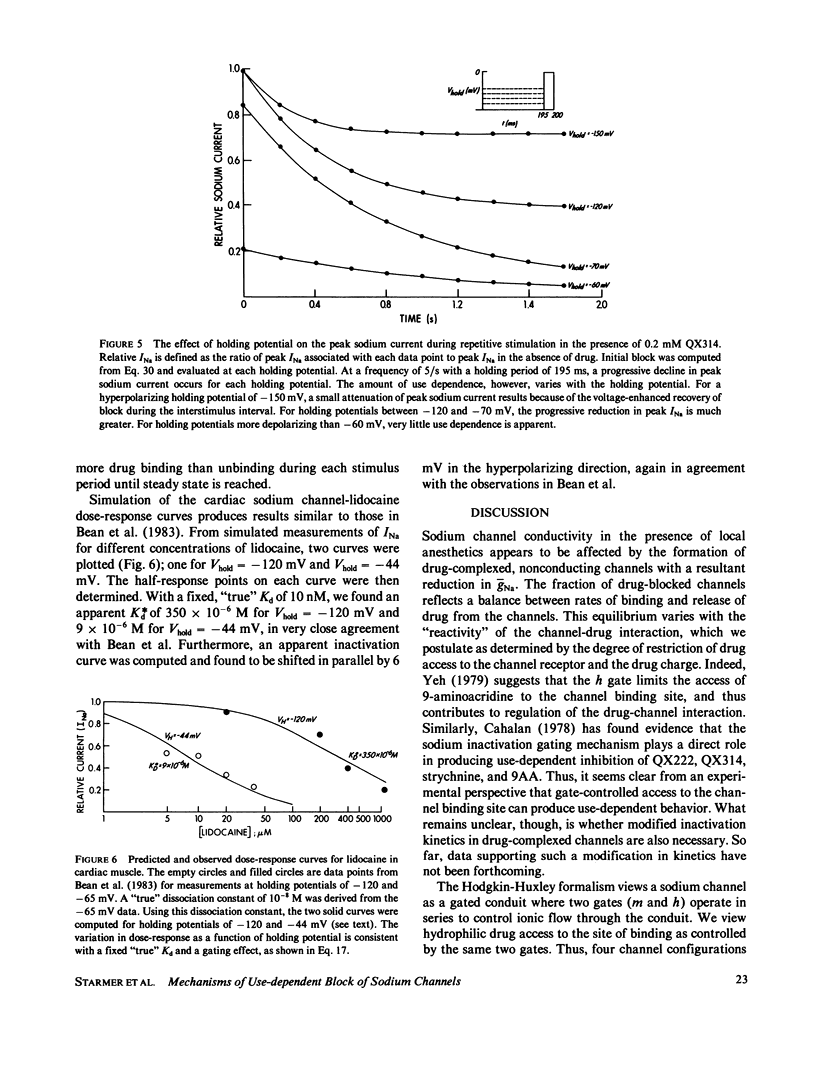
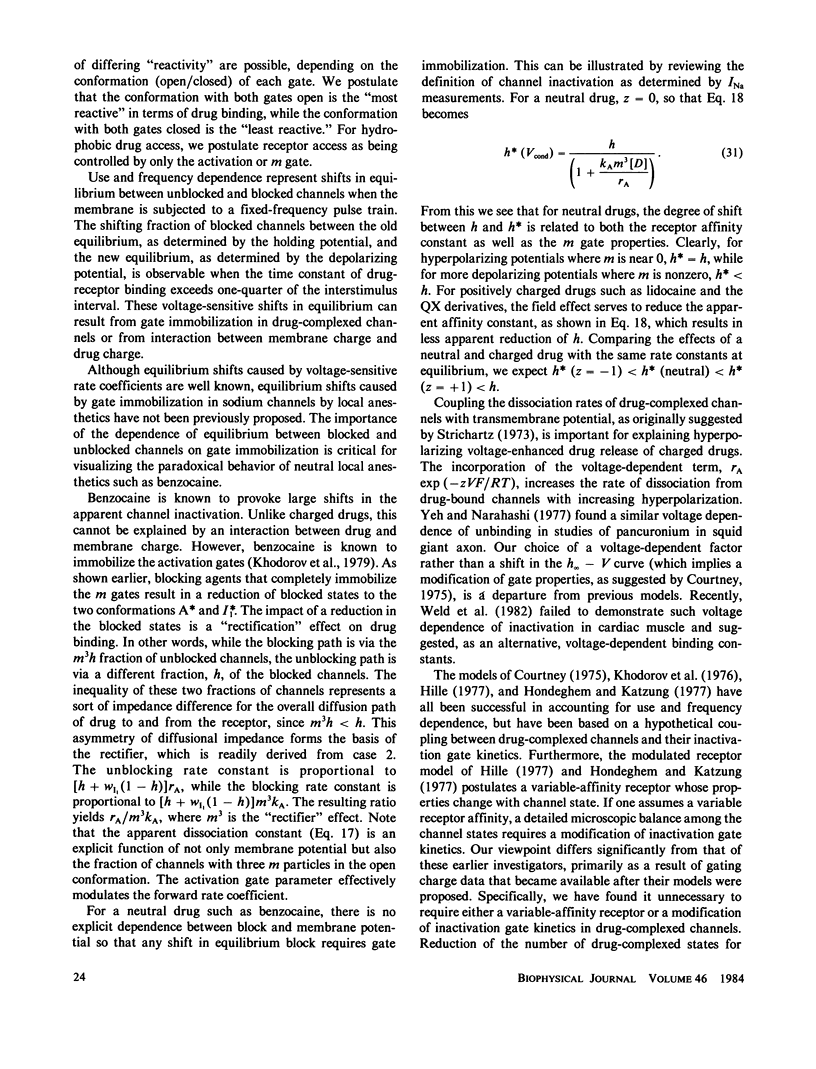
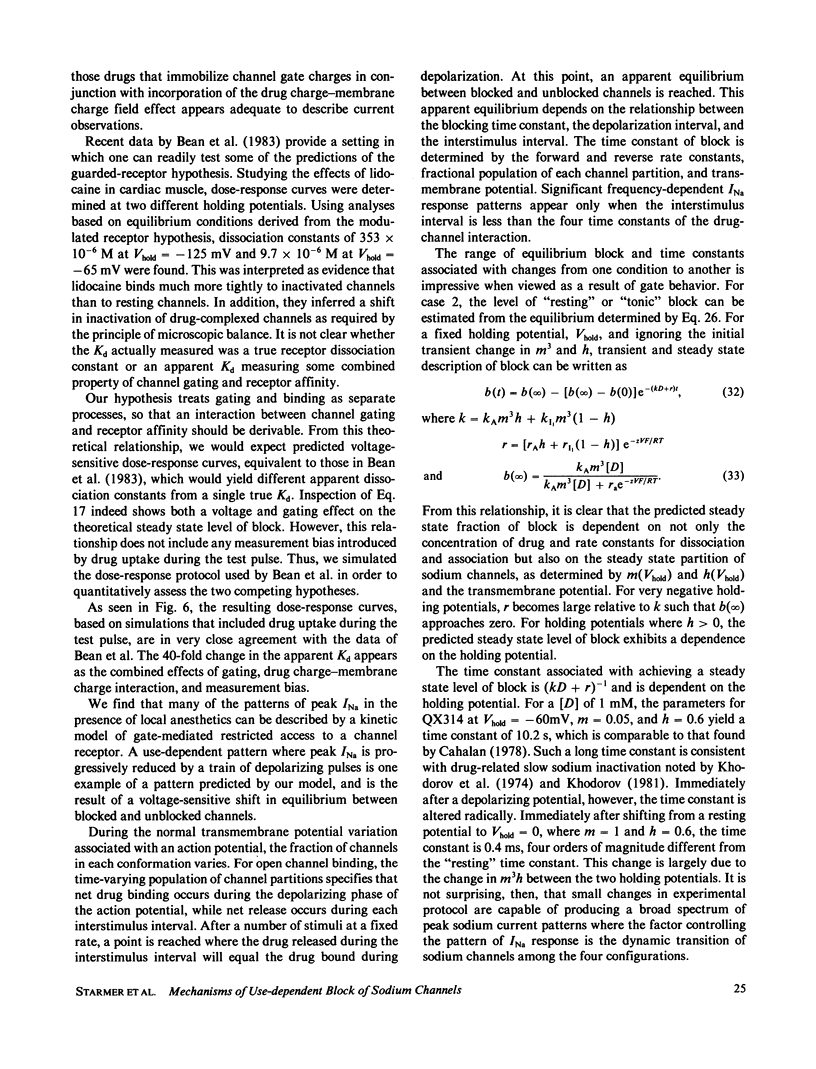
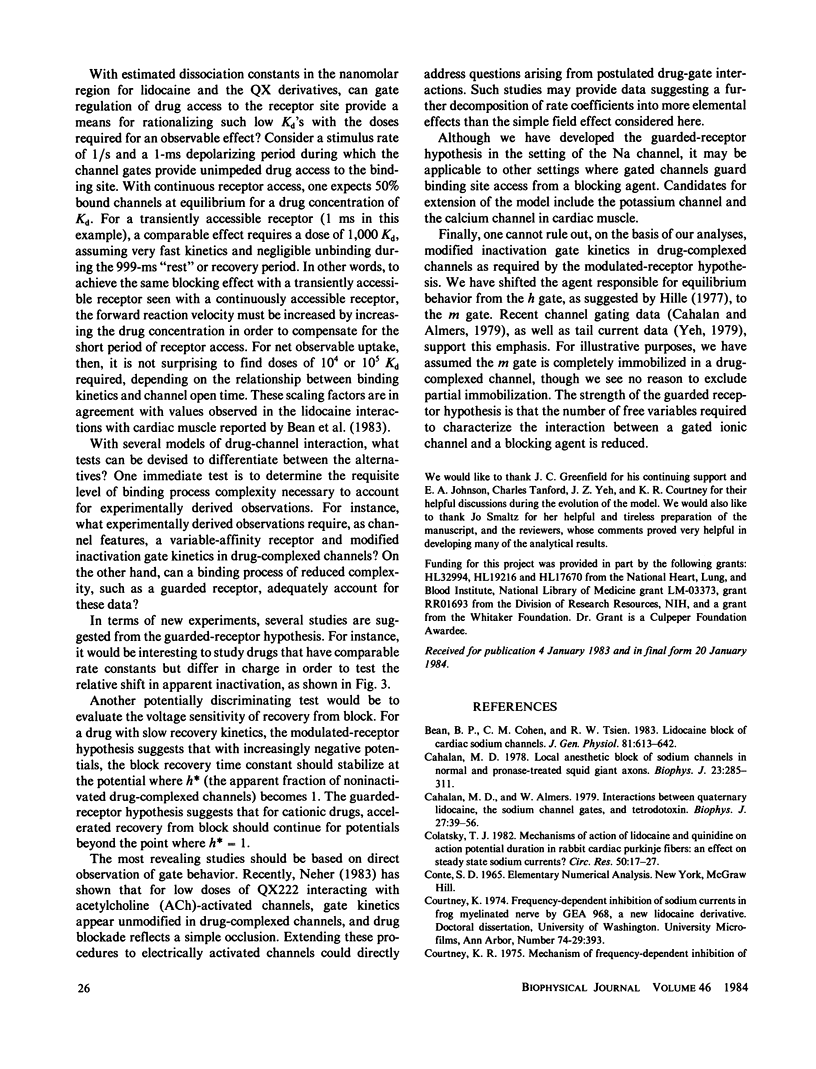
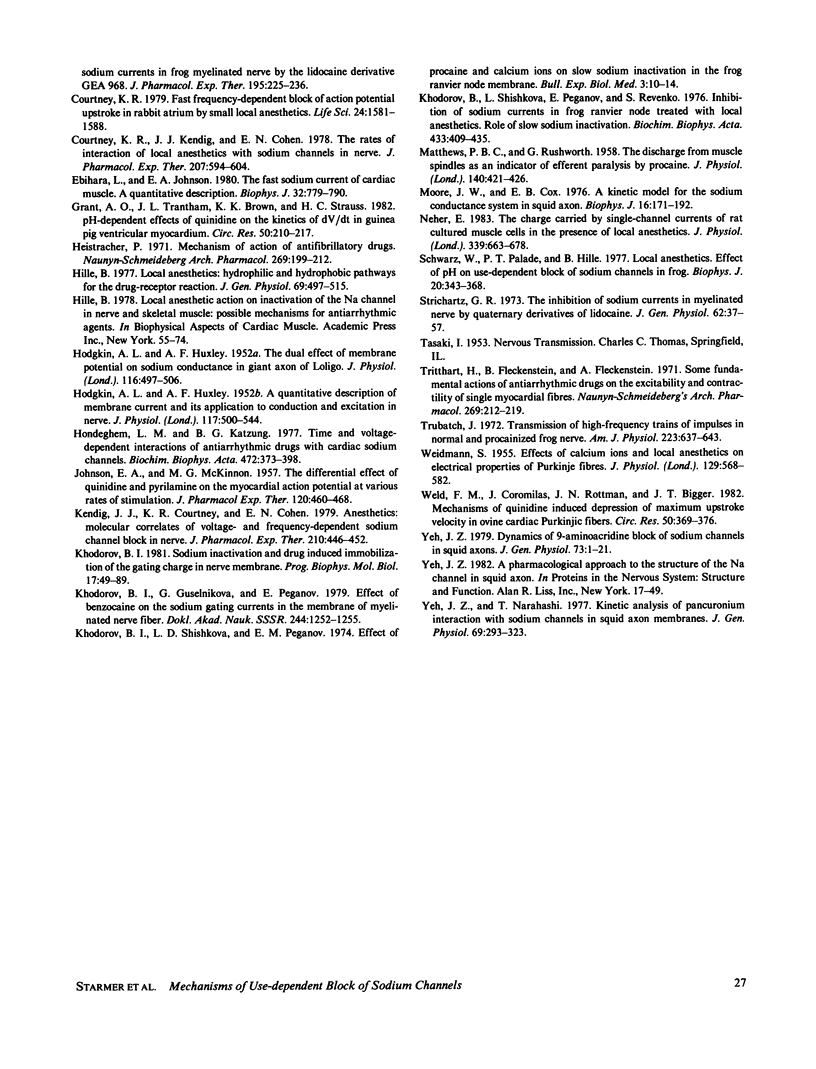
Selected References
These references are in PubMed. This may not be the complete list of references from this article.
- Bean B. P., Cohen C. J., Tsien R. W. Lidocaine block of cardiac sodium channels. J Gen Physiol. 1983 May;81(5):613–642. doi: 10.1085/jgp.81.5.613. [DOI] [PMC free article] [PubMed] [Google Scholar]
- Cahalan M. D., Almers W. Interactions between quaternary lidocaine, the sodium channel gates, and tetrodotoxin. Biophys J. 1979 Jul;27(1):39–55. doi: 10.1016/S0006-3495(79)85201-7. [DOI] [PMC free article] [PubMed] [Google Scholar]
- Cahalan M. D. Local anesthetic block of sodium channels in normal and pronase-treated squid giant axons. Biophys J. 1978 Aug;23(2):285–311. doi: 10.1016/S0006-3495(78)85449-6. [DOI] [PMC free article] [PubMed] [Google Scholar]
- Colatsky T. J. Mechanisms of action of lidocaine and quinidine on action potential duration in rabbit cardiac Purkinje fibers. An effect on steady state sodium currents? Circ Res. 1982 Jan;50(1):17–27. doi: 10.1161/01.res.50.1.17. [DOI] [PubMed] [Google Scholar]
- Courtney K. R. Fast frequency-dependent block of action potential upstroke in rabbit atrium by small local anesthetics. Life Sci. 1979 Apr 23;24(17):1581–1588. doi: 10.1016/0024-3205(79)90019-5. [DOI] [PubMed] [Google Scholar]
- Courtney K. R., Kendig J. J., Cohen E. N. The rates of interaction of local anesthetics with sodium channels in nerve. J Pharmacol Exp Ther. 1978 Nov;207(2):594–604. [PubMed] [Google Scholar]
- Courtney K. R. Mechanism of frequency-dependent inhibition of sodium currents in frog myelinated nerve by the lidocaine derivative GEA. J Pharmacol Exp Ther. 1975 Nov;195(2):225–236. [PubMed] [Google Scholar]
- Ebihara L., Johnson E. A. Fast sodium current in cardiac muscle. A quantitative description. Biophys J. 1980 Nov;32(2):779–790. doi: 10.1016/S0006-3495(80)85016-8. [DOI] [PMC free article] [PubMed] [Google Scholar]
- Grant A. O., Trantham J. L., Brown K. K., Strauss H. C. PH-Dependent effects of quinidine on the kinetics of dV/dtmax in guinea pig ventricular myocardium. Circ Res. 1982 Feb;50(2):210–217. doi: 10.1161/01.res.50.2.210. [DOI] [PubMed] [Google Scholar]
- HODGKIN A. L., HUXLEY A. F. A quantitative description of membrane current and its application to conduction and excitation in nerve. J Physiol. 1952 Aug;117(4):500–544. doi: 10.1113/jphysiol.1952.sp004764. [DOI] [PMC free article] [PubMed] [Google Scholar]
- HODGKIN A. L., HUXLEY A. F. The dual effect of membrane potential on sodium conductance in the giant axon of Loligo. J Physiol. 1952 Apr;116(4):497–506. doi: 10.1113/jphysiol.1952.sp004719. [DOI] [PMC free article] [PubMed] [Google Scholar]
- Heistracher P. Mechanism of action of antifibrillatory drugs. Naunyn Schmiedebergs Arch Pharmakol. 1971;269(2):199–212. doi: 10.1007/BF01003037. [DOI] [PubMed] [Google Scholar]
- Hille B. Local anesthetics: hydrophilic and hydrophobic pathways for the drug-receptor reaction. J Gen Physiol. 1977 Apr;69(4):497–515. doi: 10.1085/jgp.69.4.497. [DOI] [PMC free article] [PubMed] [Google Scholar]
- Hondeghem L. M., Katzung B. G. Time- and voltage-dependent interactions of antiarrhythmic drugs with cardiac sodium channels. Biochim Biophys Acta. 1977 Nov 14;472(3-4):373–398. doi: 10.1016/0304-4157(77)90003-x. [DOI] [PubMed] [Google Scholar]
- JOHNSON E. A., McKINNON M. G. The differential effect of quinidine and pyrilamine on the myocardial action potential at various rates of stimulation. J Pharmacol Exp Ther. 1957 Aug;120(4):460–468. [PubMed] [Google Scholar]
- Kendig J. J., Courtney K. R., Cohen E. N. Anesthetics: molecular correlates of voltage- and frequency-dependent sodium channel block in nerve. J Pharmacol Exp Ther. 1979 Sep;210(3):446–452. [PubMed] [Google Scholar]
- Khodorov B. I., Gusel'nikova G. G., Peganov E. M. Vliianie anestezina (benzokaina) na natrievye vorotnye toki membrany perekhvata Ran've. Dokl Akad Nauk SSSR. 1979;244(5):1252–1255. [PubMed] [Google Scholar]
- Khodorov B. I. Sodium inactivation and drug-induced immobilization of the gating charge in nerve membrane. Prog Biophys Mol Biol. 1981;37(2):49–89. doi: 10.1016/0079-6107(82)90020-7. [DOI] [PubMed] [Google Scholar]
- MATTHEWS P. B., RUSHWORTH G. The discharge from muscle spindles as an indicator of gamma efferent paralysis by procaine. J Physiol. 1958 Mar 11;140(3):421–426. [PMC free article] [PubMed] [Google Scholar]
- Moore J. W., Cox E. B. A kinetic model for the sodium conductance system in squid axon. Biophys J. 1976 Feb;16(2 Pt 1):171–192. doi: 10.1016/s0006-3495(76)85673-1. [DOI] [PMC free article] [PubMed] [Google Scholar]
- Neher E. The charge carried by single-channel currents of rat cultured muscle cells in the presence of local anaesthetics. J Physiol. 1983 Jun;339:663–678. doi: 10.1113/jphysiol.1983.sp014741. [DOI] [PMC free article] [PubMed] [Google Scholar]
- Schwarz W., Palade P. T., Hille B. Local anesthetics. Effect of pH on use-dependent block of sodium channels in frog muscle. Biophys J. 1977 Dec;20(3):343–368. doi: 10.1016/S0006-3495(77)85554-9. [DOI] [PMC free article] [PubMed] [Google Scholar]
- Strichartz G. R. The inhibition of sodium currents in myelinated nerve by quaternary derivatives of lidocaine. J Gen Physiol. 1973 Jul;62(1):37–57. doi: 10.1085/jgp.62.1.37. [DOI] [PMC free article] [PubMed] [Google Scholar]
- Tritthart H., Fleckenstein B., Fleckenstein A. Some fundamental actions of antiarrhythmic drugs on the excitability and the contractility of singel myocardial fibers. Naunyn Schmiedebergs Arch Pharmakol. 1971;269(2):212–219. doi: 10.1007/BF01003038. [DOI] [PubMed] [Google Scholar]
- Trubatch J. Transmission of high-frequency trains of impulses in normal and procainized frog nerve. Am J Physiol. 1972 Sep;223(3):637–643. doi: 10.1152/ajplegacy.1972.223.3.637. [DOI] [PubMed] [Google Scholar]
- WEIDMANN S. Effects of calcium ions and local anesthetics on electrical properties of Purkinje fibres. J Physiol. 1955 Sep 28;129(3):568–582. doi: 10.1113/jphysiol.1955.sp005379. [DOI] [PMC free article] [PubMed] [Google Scholar]
- Weld F. M., Coromilas J., Rottman J. N., Bigger J. T., Jr Mechanisms of quinidine-induced depression of maximum upstroke velocity in ovine cardiac Purkinje fibers. Circ Res. 1982 Mar;50(3):369–376. doi: 10.1161/01.res.50.3.369. [DOI] [PubMed] [Google Scholar]
- Yeh J. Z. A pharmacological approach to the structure of the Na channel in squid axon. Prog Clin Biol Res. 1982;79:17–49. [PubMed] [Google Scholar]
- Yeh J. Z. Dynamics of 9-aminoacridine block of sodium channels in squid axons. J Gen Physiol. 1979 Jan;73(1):1–21. doi: 10.1085/jgp.73.1.1. [DOI] [PMC free article] [PubMed] [Google Scholar]
- Yeh J. Z., Narahashi T. Kinetic analysis of pancuronium interaction with sodium channels in squid axon membranes. J Gen Physiol. 1977 Mar;69(3):293–323. doi: 10.1085/jgp.69.3.293. [DOI] [PMC free article] [PubMed] [Google Scholar]