Abstract
We present a perturbation method for analyzing nucleation-controlled polymerization augmented by a secondary pathway for polymer growth. With this method, the solution to the kinetic equations assumes a simple analytic closed form that can easily be used in fitting data. So long as the formation of polymers by the secondary pathway depends linearly on the concentration of monomers polymerized, the form of the solutions is the same. This permits the analysis of augmented growth models with a minimum number of modeling assumptions, and thus makes it readily possible to distinguish between a variety of secondary processes (heterogeneous nucleation, lateral growth, and fragmentation). In addition, the parameters of the homogeneous process, such as the homogeneous nucleus size, can be determined independent of the nature of the secondary mechanism. We describe applications of this method to the polymerization of actin, collagen, and sickle hemoglobin. We present an extensive analysis of data on actin polymerization (Wegner, A., and P. Savko, 1982, Biochemistry, 21:1909-1913) to illustrate the use of the method. Although our conclusions generally agree with theirs, we find that lateral growth describes the secondary pathway better than the fragmentation model originally proposed. We also show how this method can be used to study the degree of polymerization, the parentage of polymers, and the behavior of polymers in cycling experiments.
Full text
PDF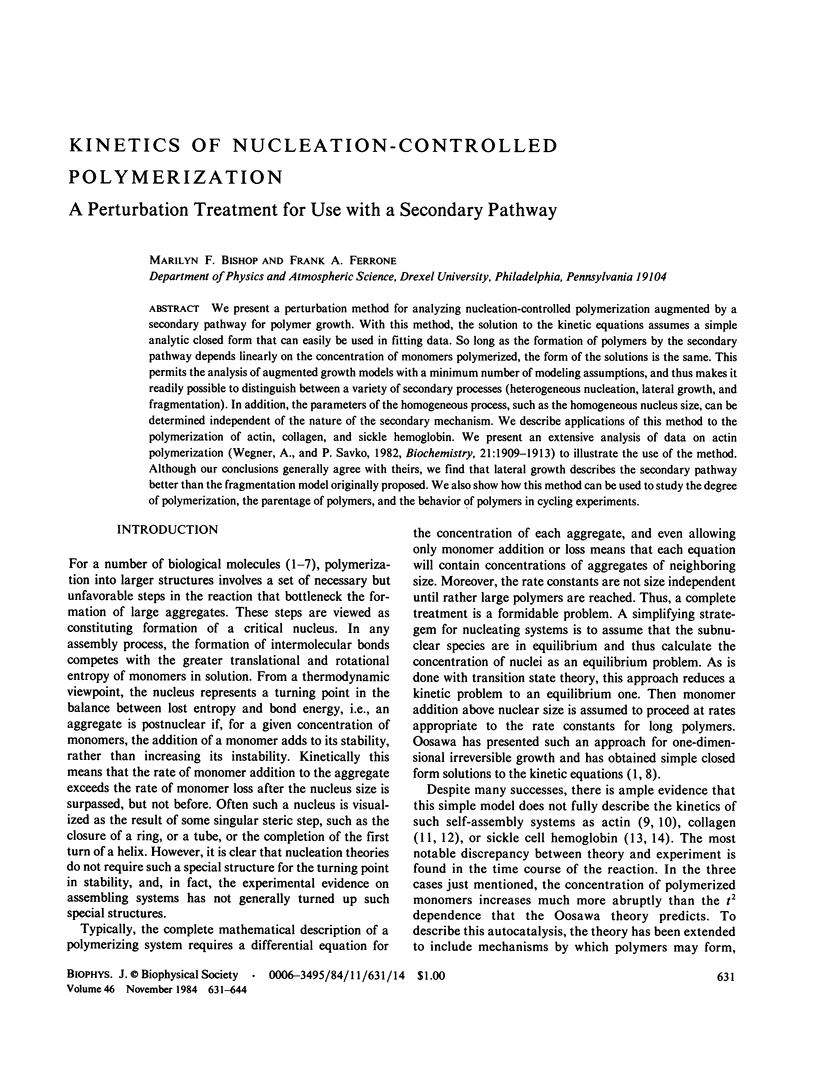
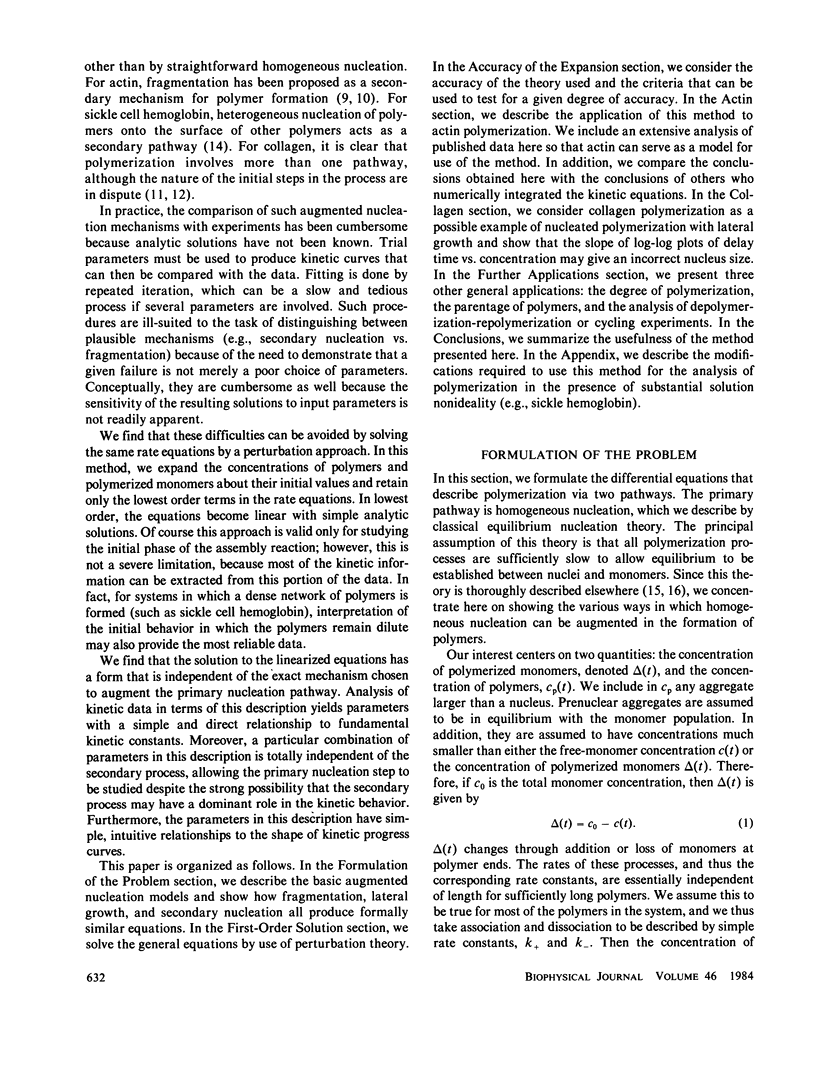
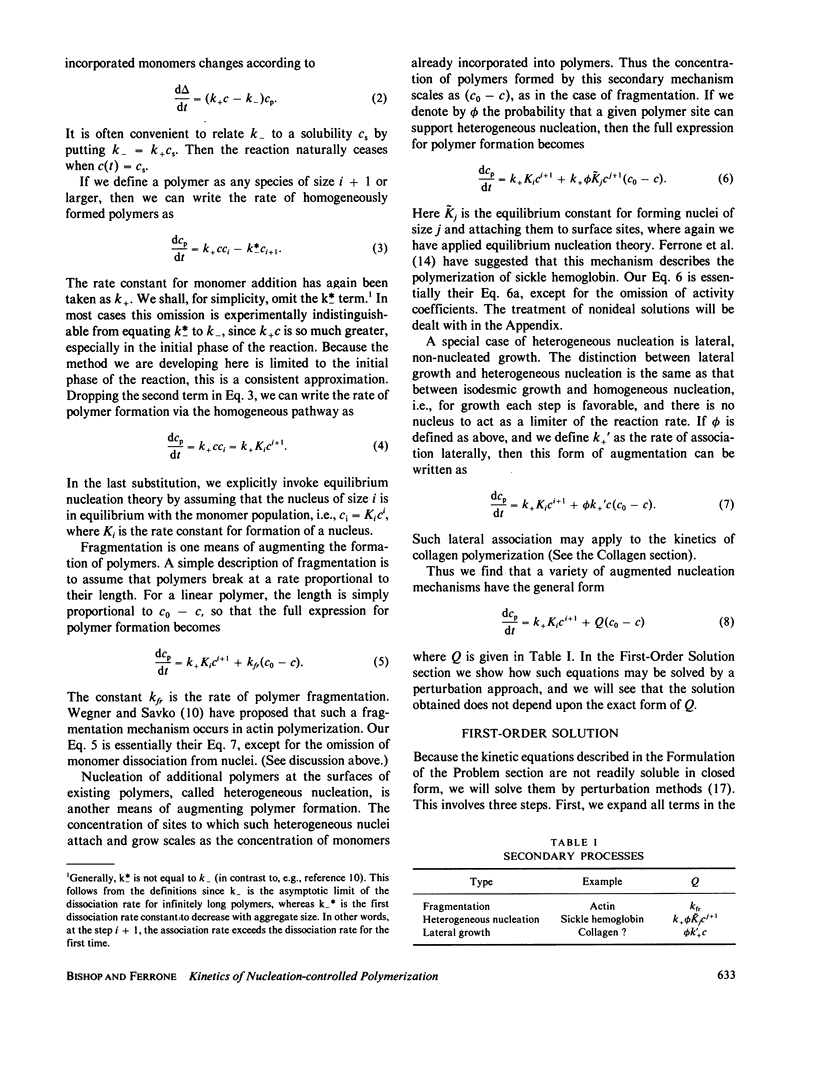
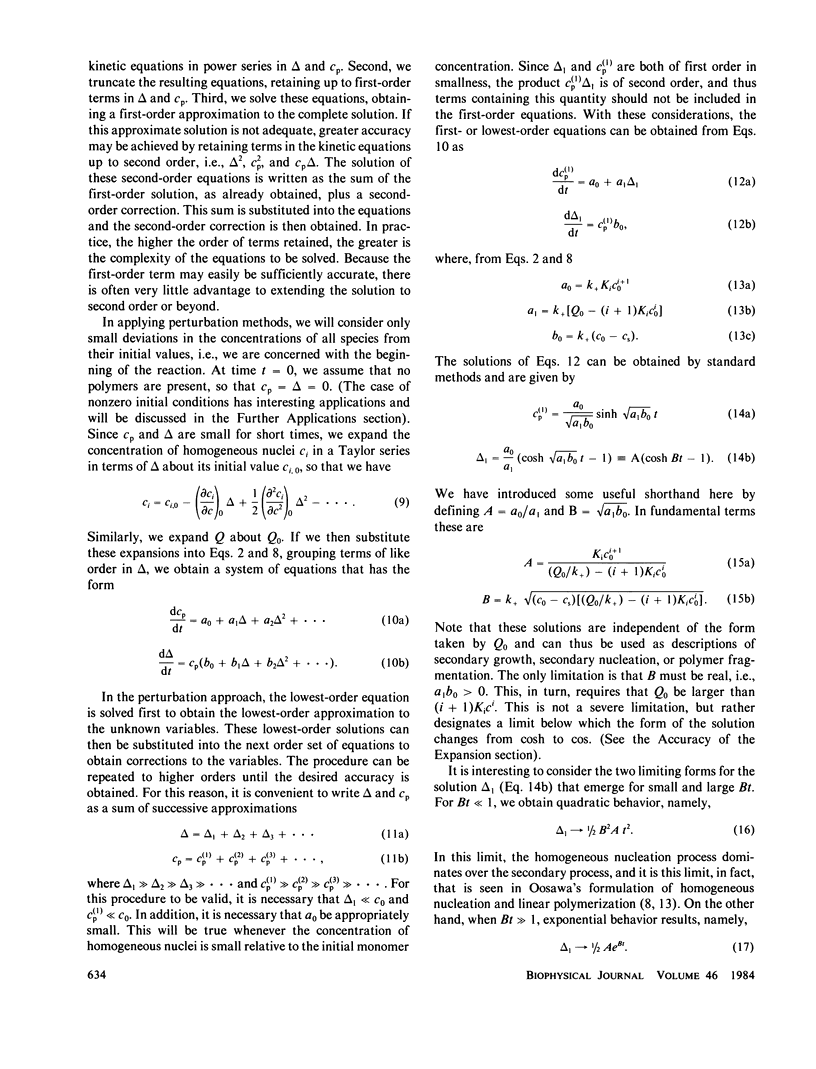
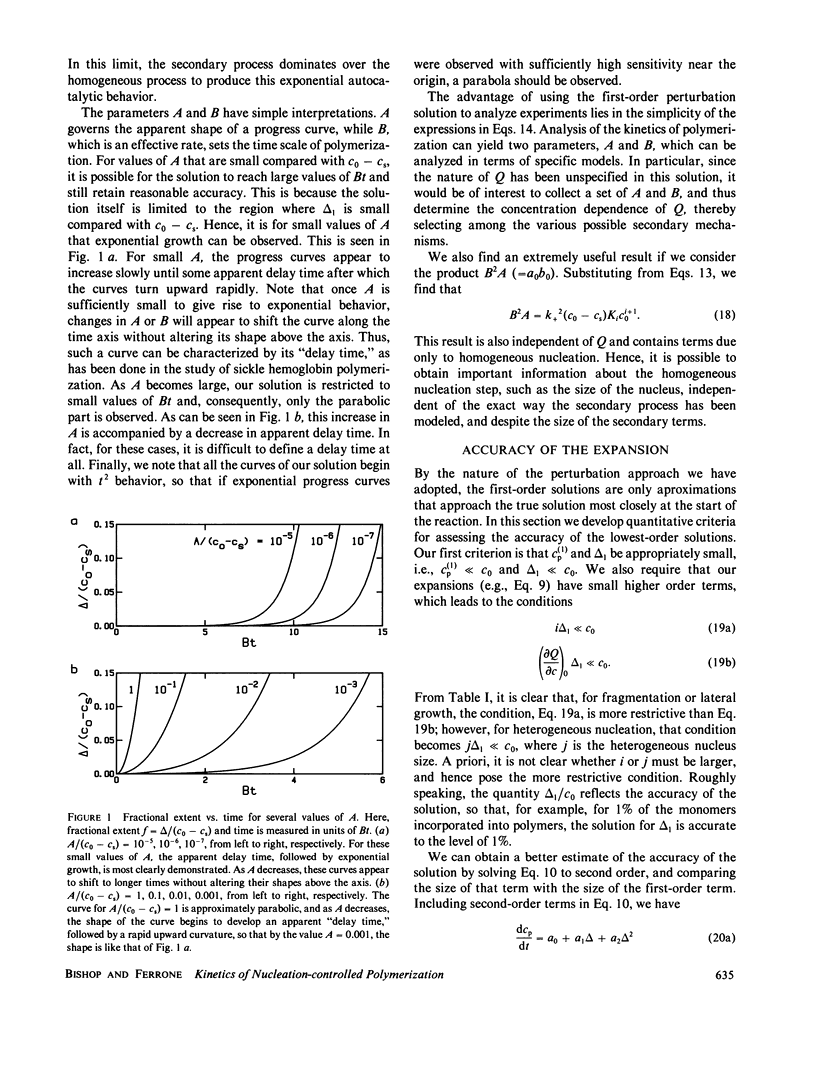
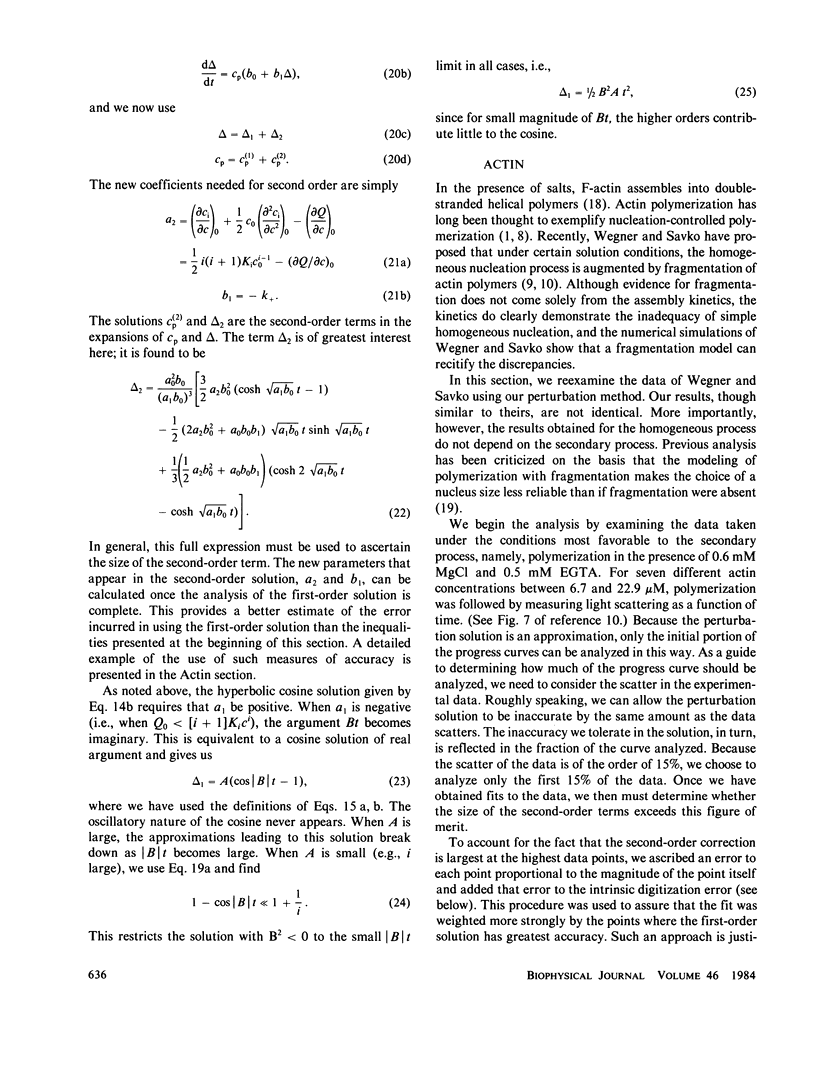
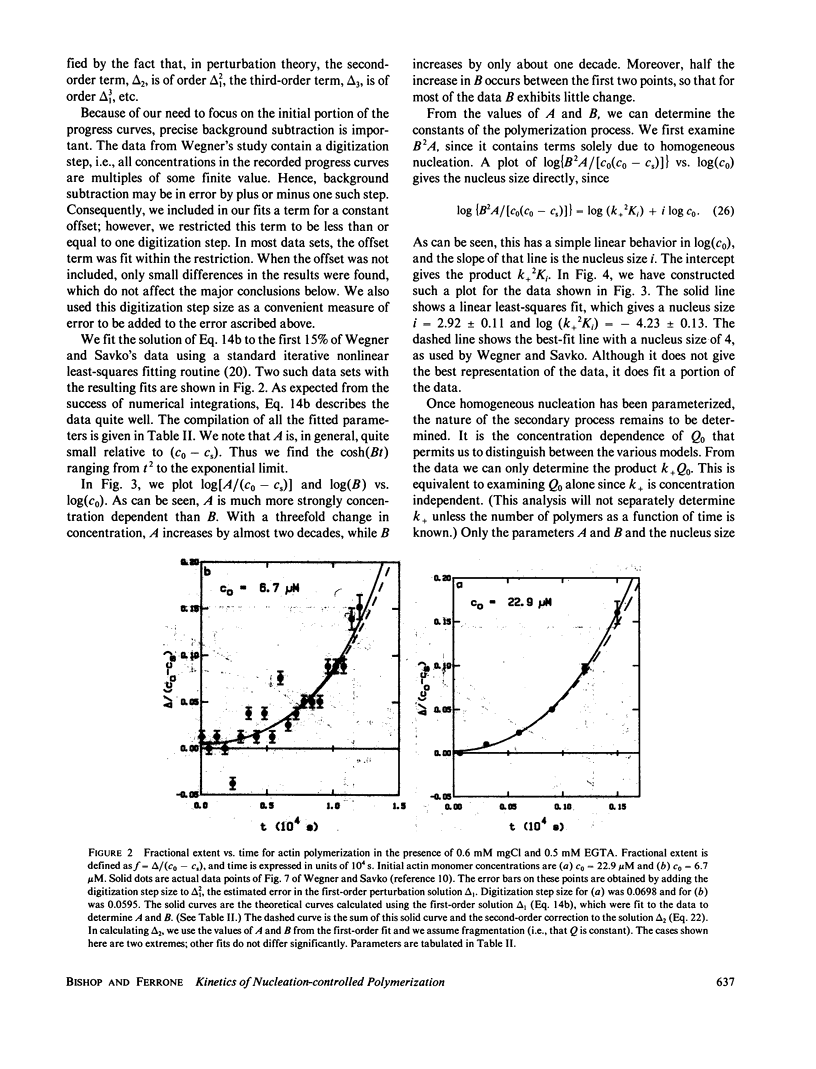
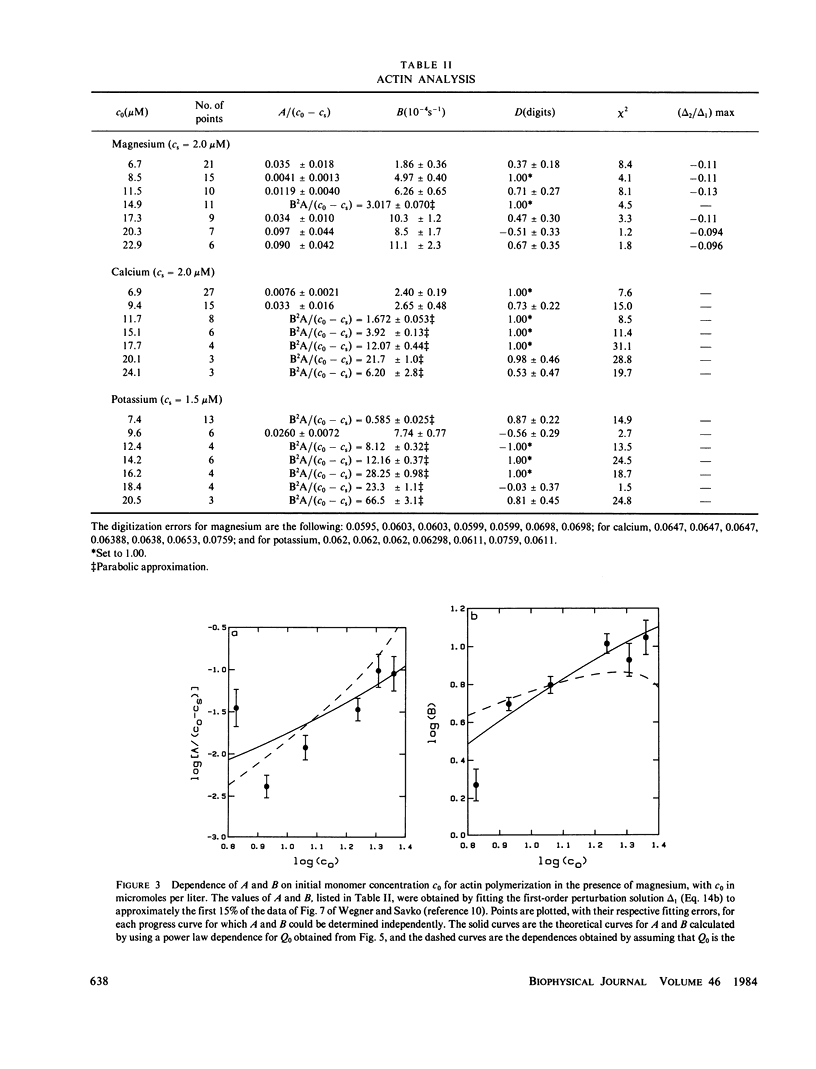
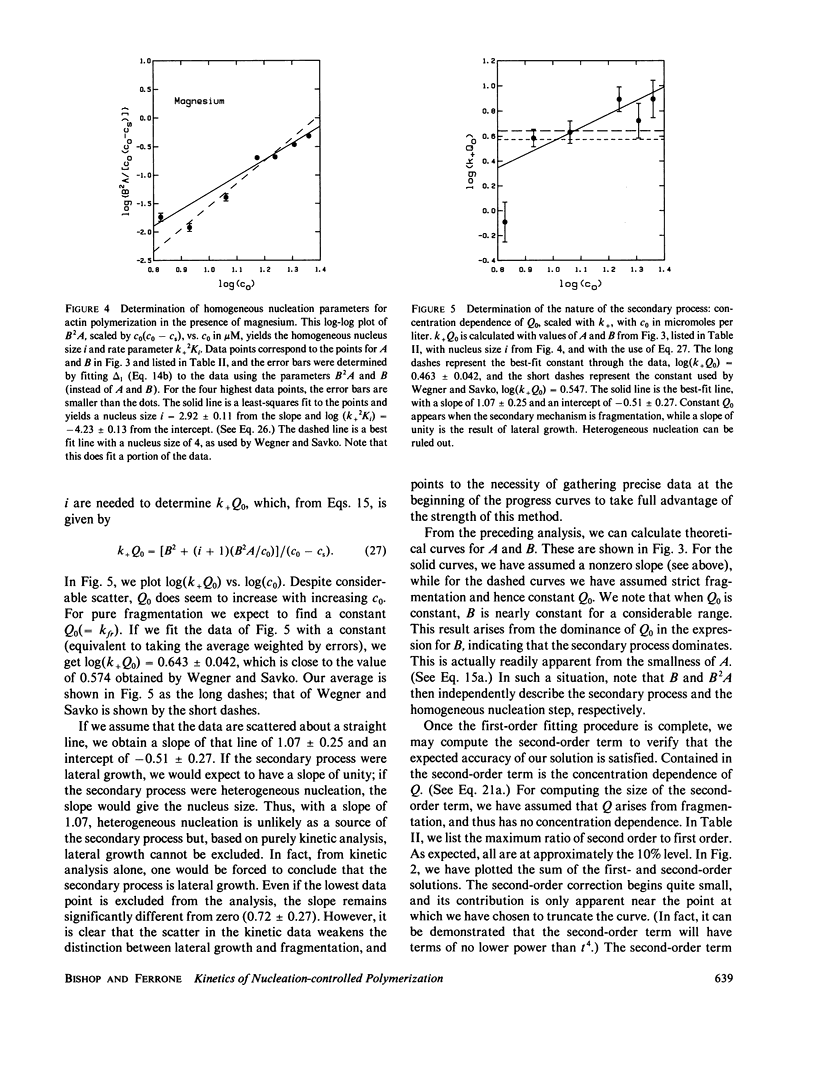
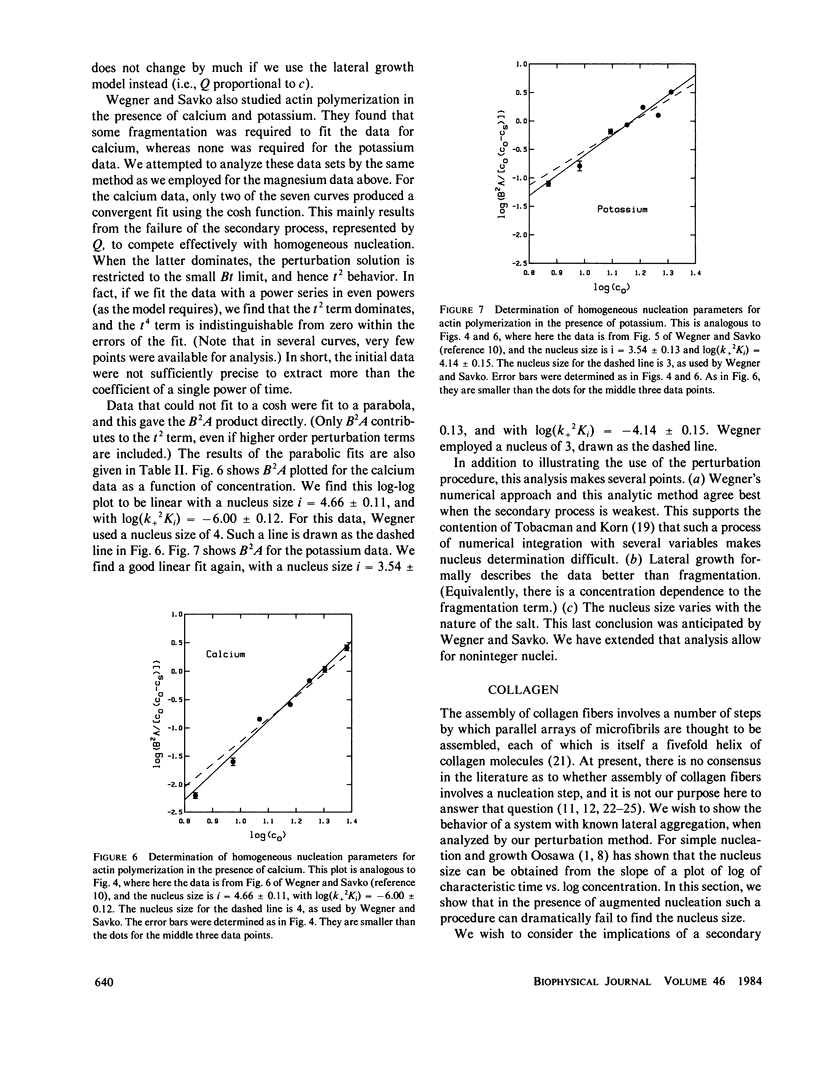
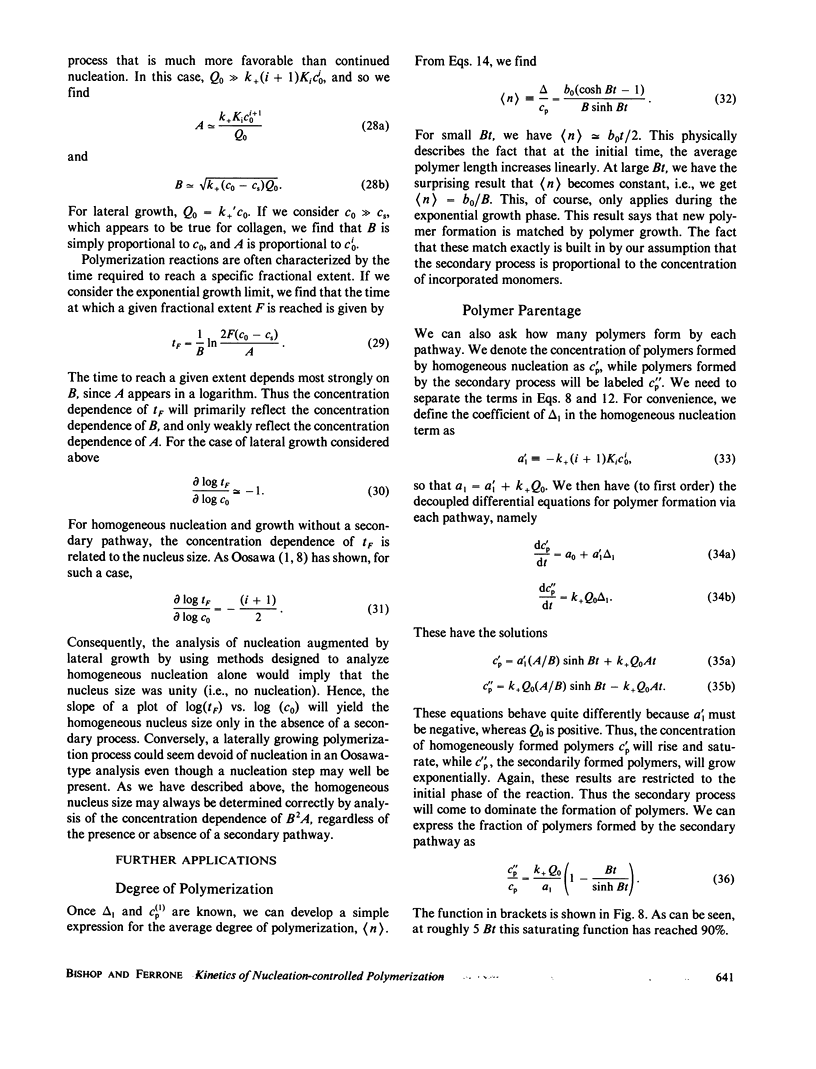
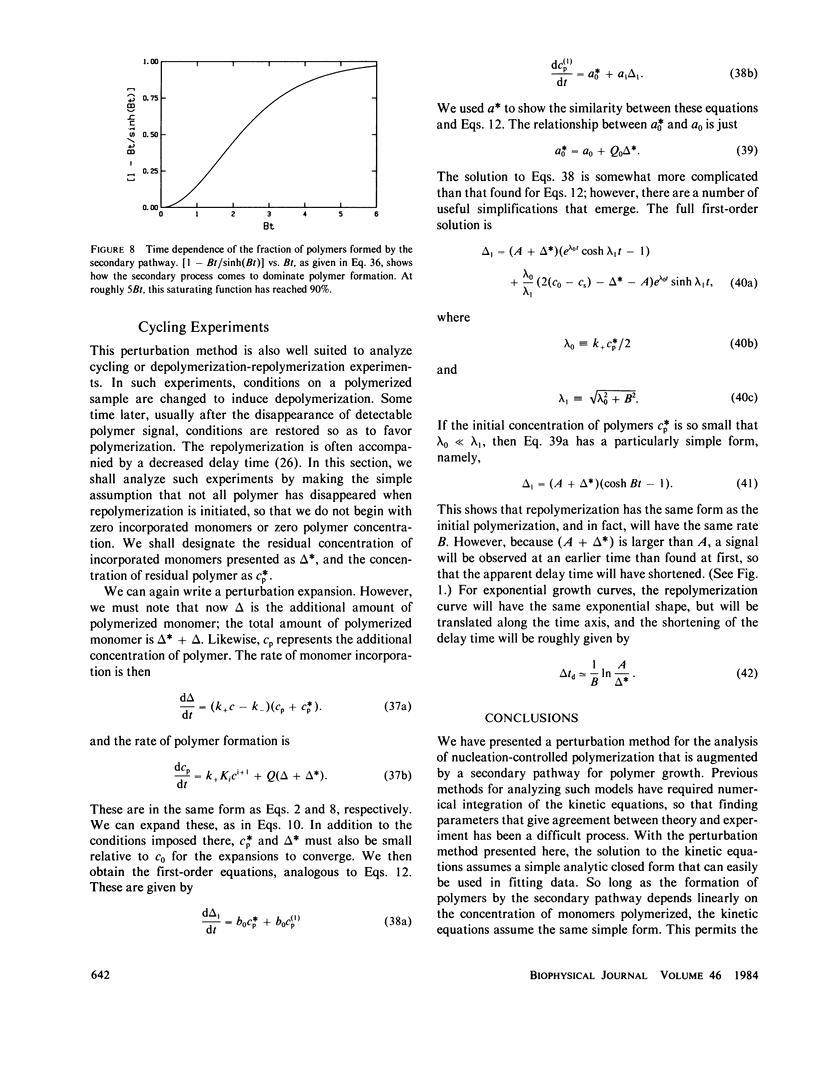
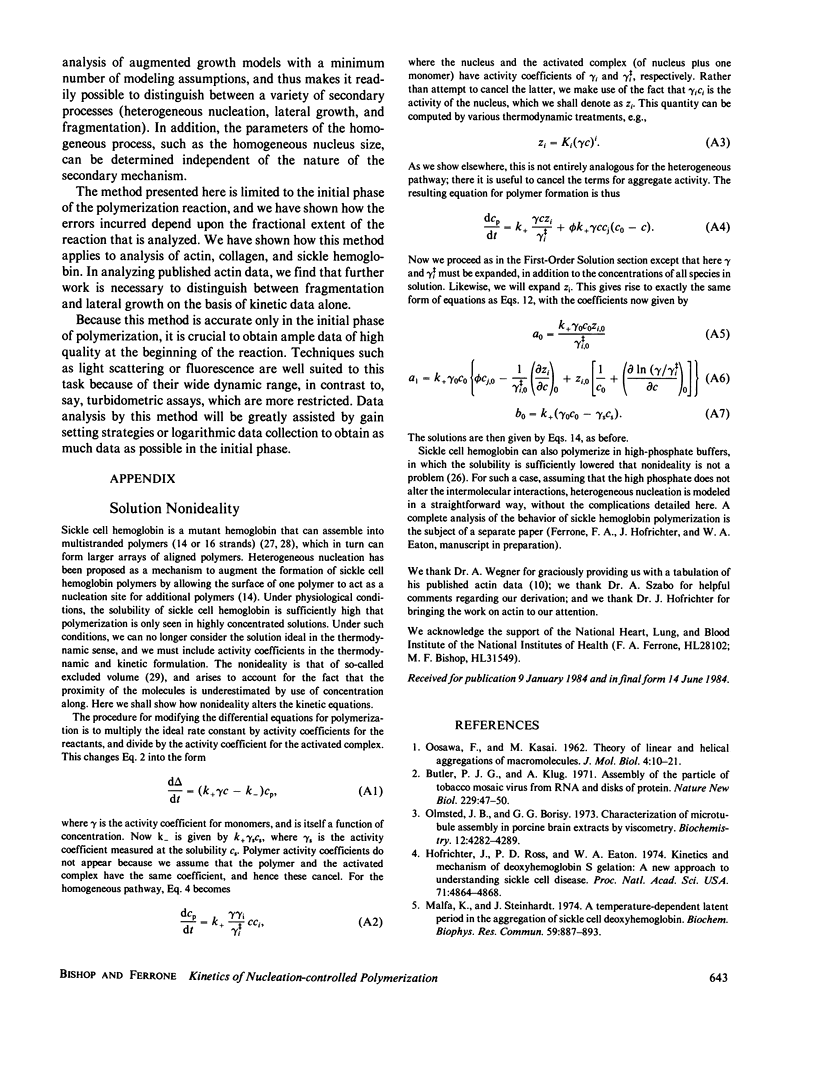
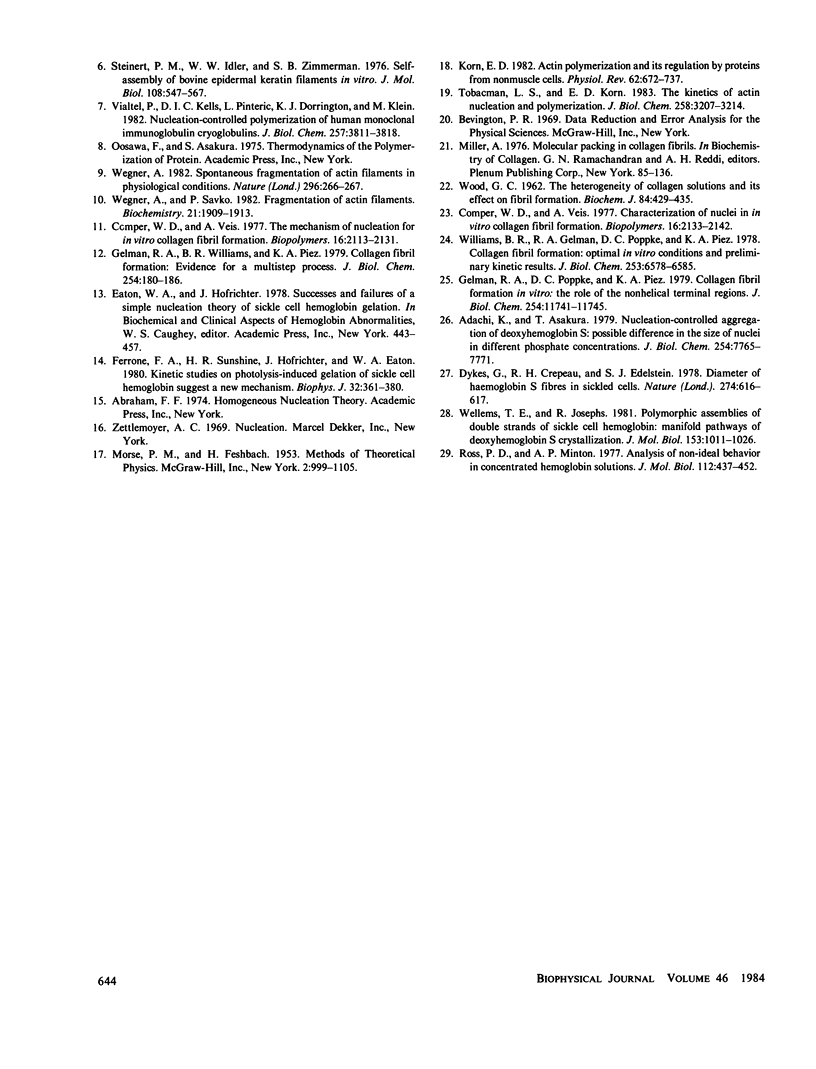
Selected References
These references are in PubMed. This may not be the complete list of references from this article.
- Adachi K., Asakura T. Nucleation-controlled aggregation of deoxyhemoglobin S. Possible difference in the size of nuclei in different phosphate concentrations. J Biol Chem. 1979 Aug 25;254(16):7765–7771. [PubMed] [Google Scholar]
- Butler P. J., Klug A. Assembly of the particle of tobacco mosaic virus from RNA and disks of protein. Nat New Biol. 1971 Jan 13;229(2):47–50. doi: 10.1038/newbio229047a0. [DOI] [PubMed] [Google Scholar]
- Comper W. D., Veis A. Characterization of nuclei in in vitro collagen fibril formation. Biopolymers. 1977 Oct;16(10):2133–2142. doi: 10.1002/bip.1977.360161005. [DOI] [PubMed] [Google Scholar]
- Comper W. D., Veis A. The mechanism of nucleation for in vitro collagen fibril formation. Biopolymers. 1977 Oct;16(10):2113–2131. doi: 10.1002/bip.1977.360161004. [DOI] [PubMed] [Google Scholar]
- Crepeau R. H., Dykes G., Garrell R., Edelstein S. J. Diameter of haemoglobin S fibres in sickled cells. Nature. 1978 Aug 10;274(5671):616–617. doi: 10.1038/274616a0. [DOI] [PubMed] [Google Scholar]
- Ferrone F. A., Hofrichter J., Sunshine H. R., Eaton W. A. Kinetic studies on photolysis-induced gelation of sickle cell hemoglobin suggest a new mechanism. Biophys J. 1980 Oct;32(1):361–380. doi: 10.1016/S0006-3495(80)84962-9. [DOI] [PMC free article] [PubMed] [Google Scholar]
- Gelman R. A., Poppke D. C., Piez K. A. Collagen fibril formation in vitro. The role of the nonhelical terminal regions. J Biol Chem. 1979 Nov 25;254(22):11741–11745. [PubMed] [Google Scholar]
- Gelman R. A., Williams B. R., Piez K. A. Collagen fibril formation. Evidence for a multistep process. J Biol Chem. 1979 Jan 10;254(1):180–186. [PubMed] [Google Scholar]
- Hofrichter J., Ross P. D., Eaton W. A. Kinetics and mechanism of deoxyhemoglobin S gelation: a new approach to understanding sickle cell disease. Proc Natl Acad Sci U S A. 1974 Dec;71(12):4864–4868. doi: 10.1073/pnas.71.12.4864. [DOI] [PMC free article] [PubMed] [Google Scholar]
- Korn E. D. Actin polymerization and its regulation by proteins from nonmuscle cells. Physiol Rev. 1982 Apr;62(2):672–737. doi: 10.1152/physrev.1982.62.2.672. [DOI] [PubMed] [Google Scholar]
- Malfa R., Steinhardt J. A temperature-dependent latent-period in the aggregation of sickle-cell deoxyhemoglobin. Biochem Biophys Res Commun. 1974 Aug 5;59(3):887–893. doi: 10.1016/s0006-291x(74)80062-8. [DOI] [PubMed] [Google Scholar]
- OOSAWA F., KASAI M. A theory of linear and helical aggregations of macromolecules. J Mol Biol. 1962 Jan;4:10–21. doi: 10.1016/s0022-2836(62)80112-0. [DOI] [PubMed] [Google Scholar]
- Olmsted J. B., Borisy G. G. Characterization of microtubule assembly in porcine brain extracts by viscometry. Biochemistry. 1973 Oct 9;12(21):4282–4289. doi: 10.1021/bi00745a037. [DOI] [PubMed] [Google Scholar]
- Ross P. D., Minton A. P. Analysis of non-ideal behavior in concentrated hemoglobin solutions. J Mol Biol. 1977 May 25;112(3):437–452. doi: 10.1016/s0022-2836(77)80191-5. [DOI] [PubMed] [Google Scholar]
- Steinert P. M., Idler W. W., Zimmerman S. B. Self-assembly of bovine epidermal keratin filaments in vitro. J Mol Biol. 1976 Dec 15;108(3):547–567. doi: 10.1016/s0022-2836(76)80136-2. [DOI] [PubMed] [Google Scholar]
- Tobacman L. S., Korn E. D. The kinetics of actin nucleation and polymerization. J Biol Chem. 1983 Mar 10;258(5):3207–3214. [PubMed] [Google Scholar]
- Vialtel P., Kells D. I., Pinteric L., Dorrington K. J., Klein M. Nucleation-controlled polymerization of human monoclonal immunoglobulin G cryoglobulins. J Biol Chem. 1982 Apr 10;257(7):3811–3818. [PubMed] [Google Scholar]
- WOOD G. C. The heterogeneity of collagen solutions and its effect on fibril formation. Biochem J. 1962 Aug;84:429–435. doi: 10.1042/bj0840429. [DOI] [PMC free article] [PubMed] [Google Scholar]
- Wegner A., Savko P. Fragmentation of actin filaments. Biochemistry. 1982 Apr 13;21(8):1909–1913. doi: 10.1021/bi00537a032. [DOI] [PubMed] [Google Scholar]
- Wegner A. Spontaneous fragmentation of actin filaments in physiological conditions. Nature. 1982 Mar 18;296(5854):266–267. doi: 10.1038/296266a0. [DOI] [PubMed] [Google Scholar]
- Wellems T. E., Vassar R. J., Josephs R. Polymorphic assemblies of double strands of sickle cell hemoglobin. Manifold pathways of deoxyhemoglobin S crystallization. J Mol Biol. 1981 Dec 25;153(4):1011–1026. doi: 10.1016/0022-2836(81)90464-2. [DOI] [PubMed] [Google Scholar]
- Williams B. R., Gelman R. A., Poppke D. C., Piez K. A. Collagen fibril formation. Optimal in vitro conditions and preliminary kinetic results. J Biol Chem. 1978 Sep 25;253(18):6578–6585. [PubMed] [Google Scholar]