Abstract
In wavelength-resolved steady state spectra we observe three different kinds of emission from histone H1, a class A protein with only a single tyrosine residue. Unfolded H1 emissions that peak at approximately 300 and 340 nm can both be excited maximally at approximately 280 nm. Another, peaking much further to the red at approximately 400 nm, can be excited maximally at approximately 320 nm. The 300-nm fluorescence can be resolved by lifetime measurements into three components with decay times of approximately 1, 2, and 4 ns. On sodium-chloride-induced refolding of H1, simplification of the emission properties occurs. The 340 and 400-nm components disappear while the two shorter lifetime components of the 300-nm band diminish in amplitude and are replaced by the 4-ns decay. We believe that the 340-nm emission is tyrosinate fluorescence resulting from excited-state proton transfer. The origin of the 400-nm emission remains uncertain. We assign the 1 and 2-ns components of the 300-nm emission to two states of tyrosine in denatured H1 and the 4-ns decay to fluorescence of the single tyrosine residue in the globular region of refolded H1. Our results support the contention that salt induced folding of H1 is a cooperative two state process, and permit us to better understand the previously reported increases in fluorescence intensity and anisotropy on salt-induced folding.
Full text
PDF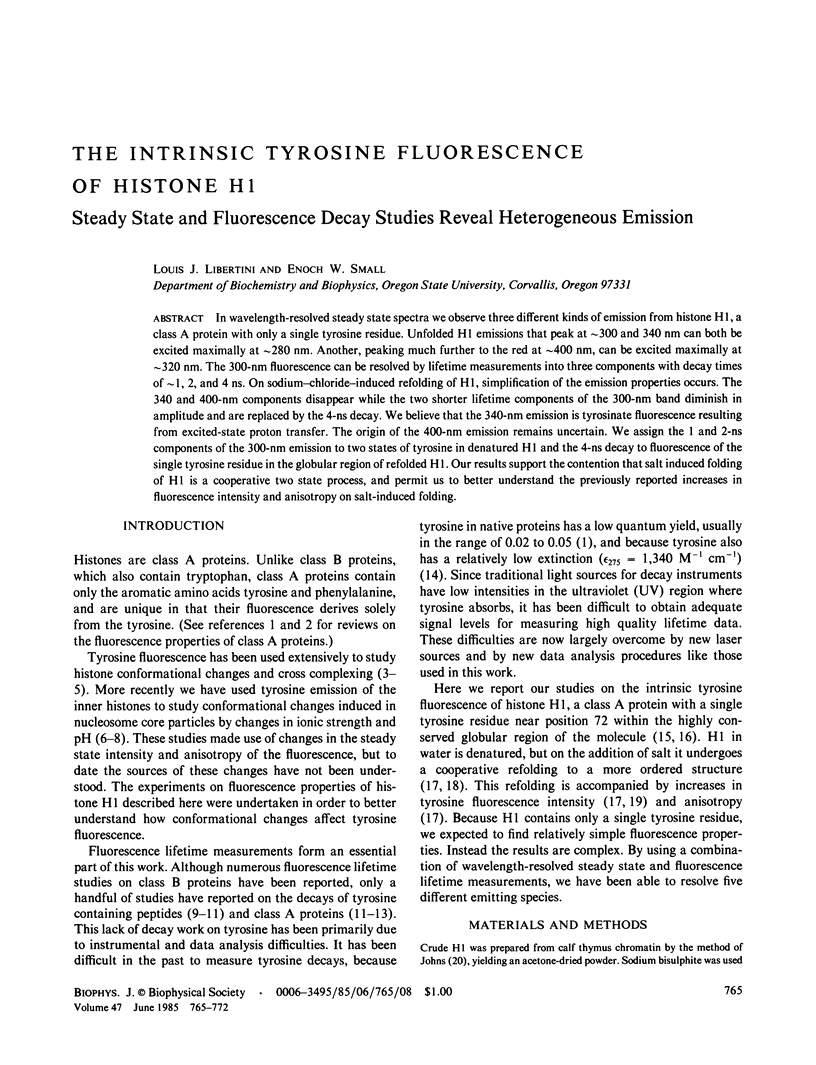
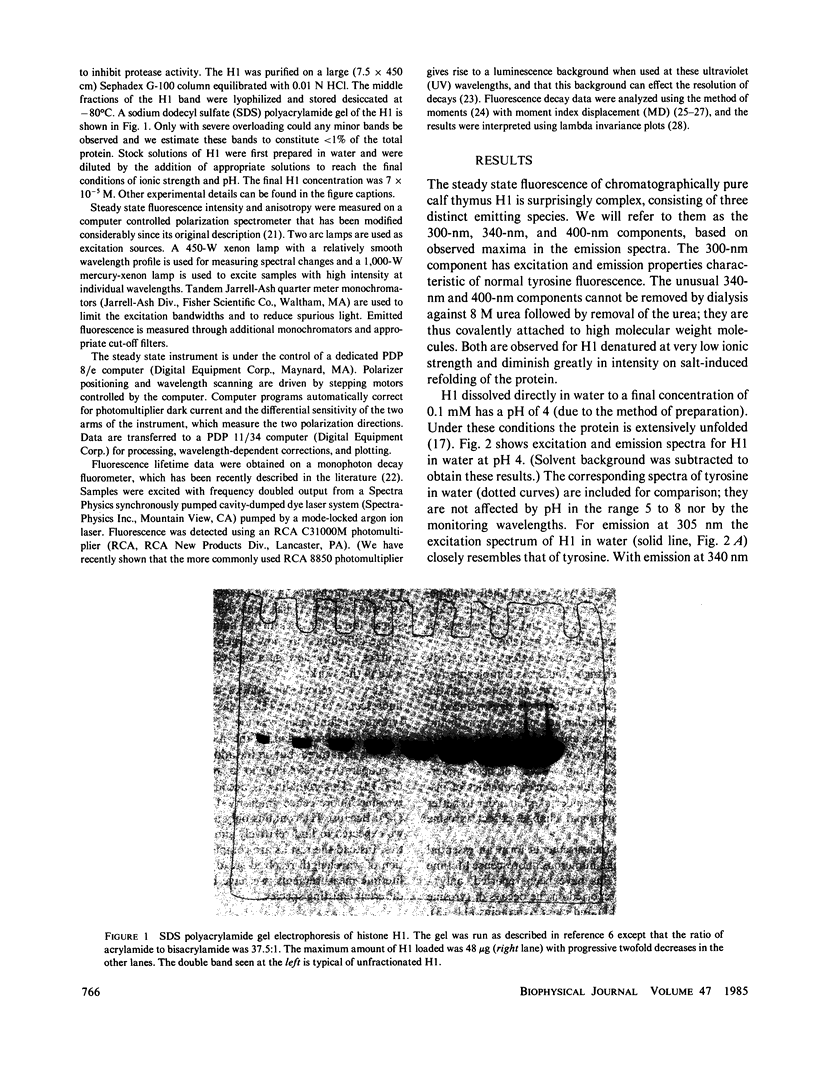
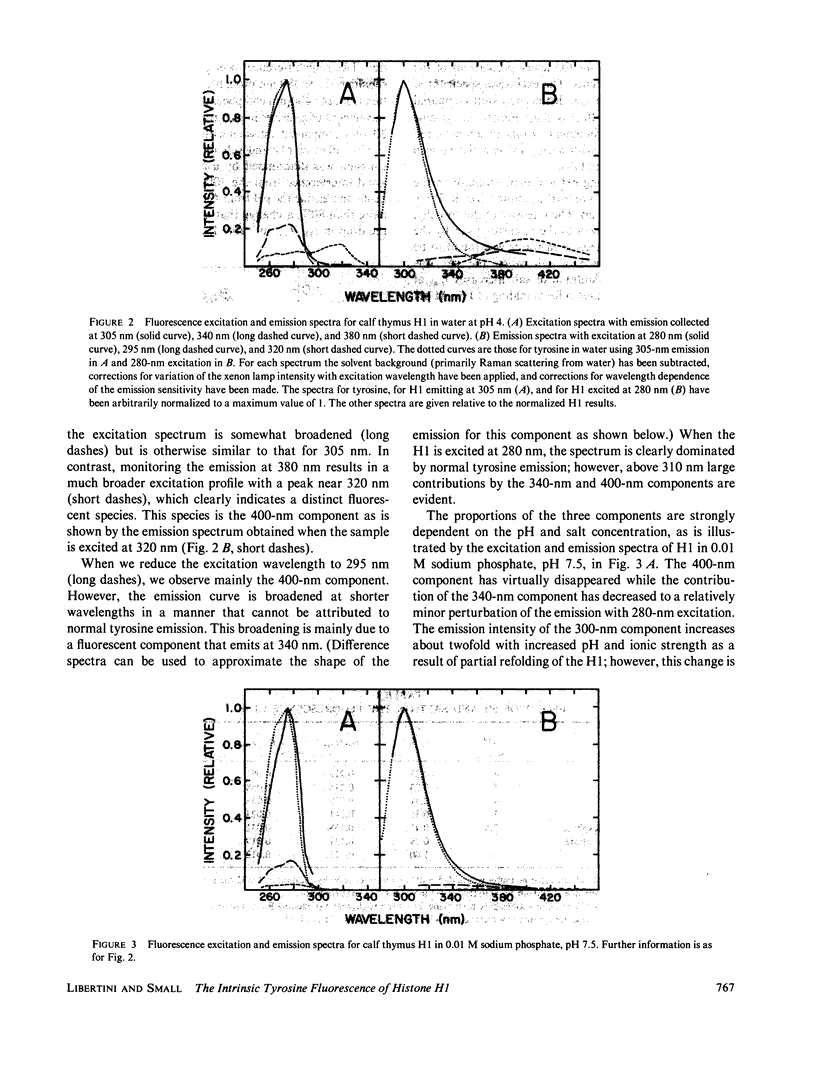
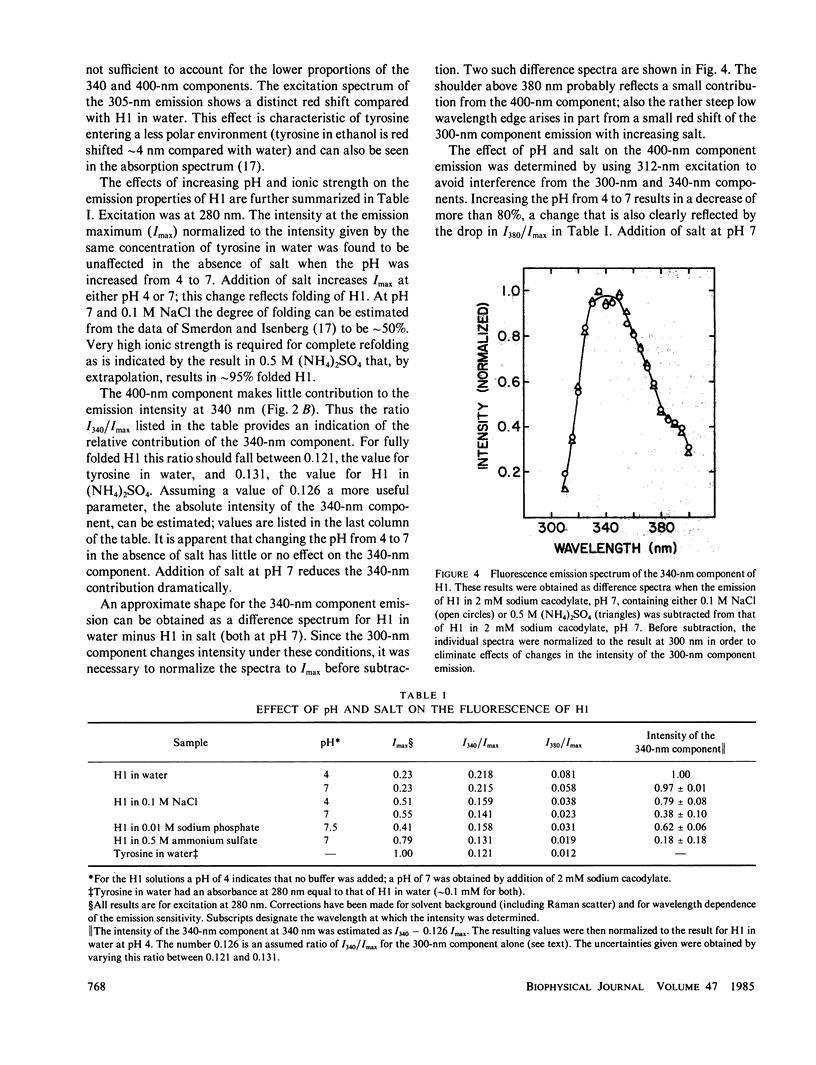
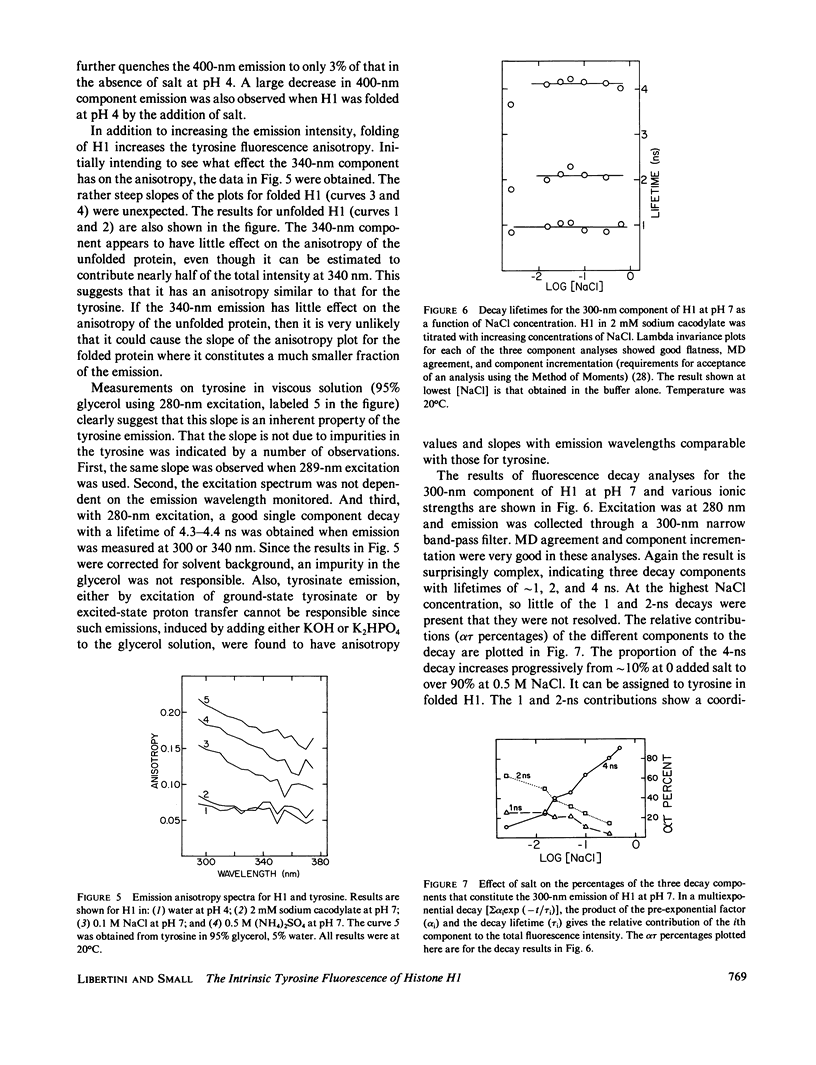
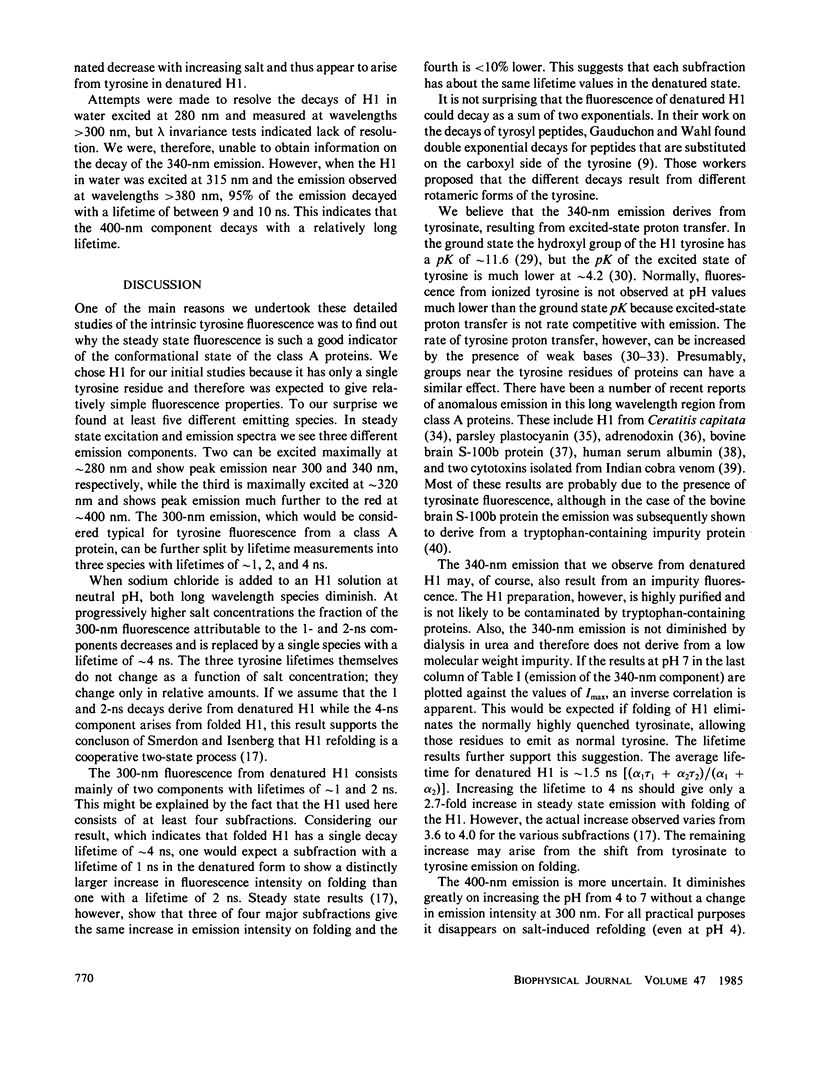
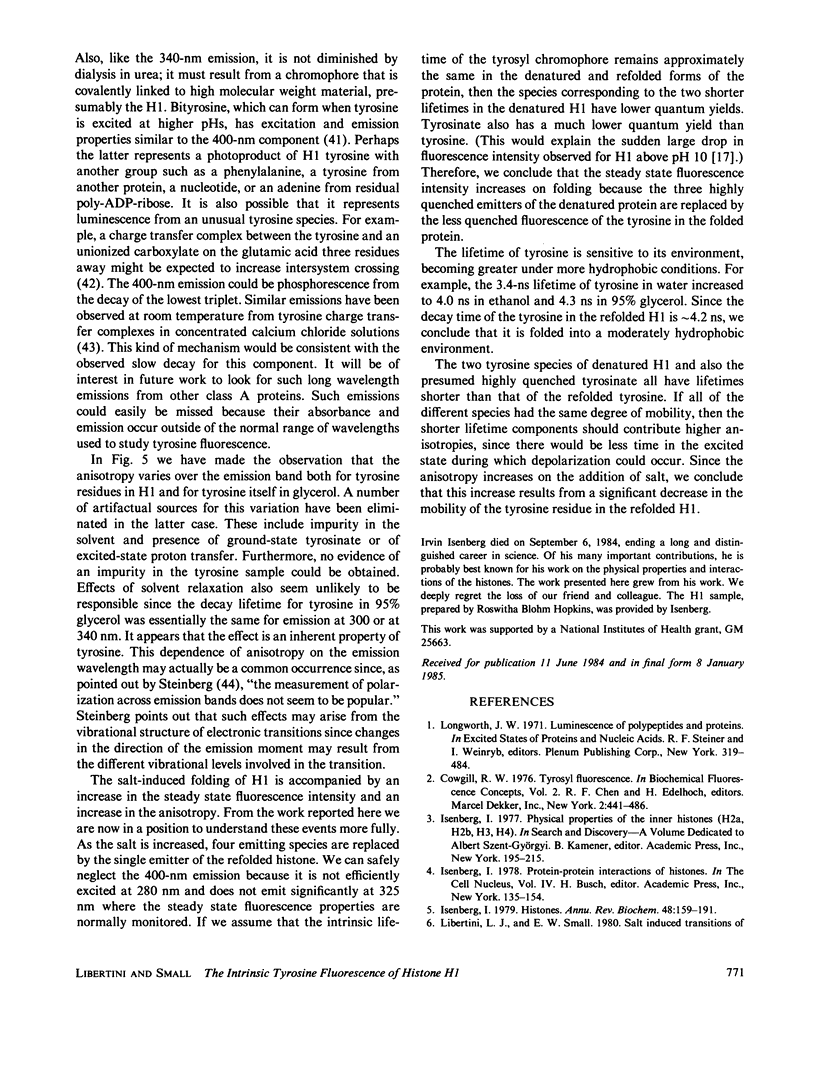
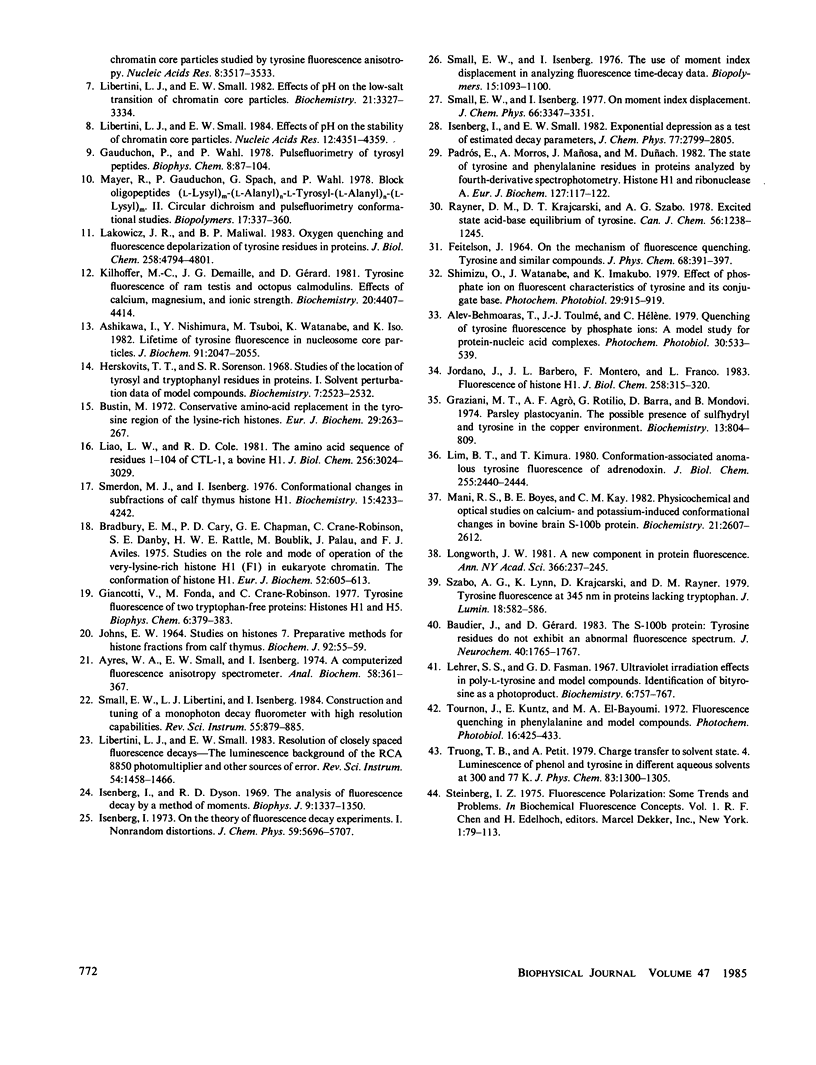
Images in this article
Selected References
These references are in PubMed. This may not be the complete list of references from this article.
- Ashikawa I., Nishimura Y., Tsuboi M., Watanabe K., Iso K. Lifetime of tyrosine fluorescence in nucleosome core particles. J Biochem. 1982 Jun;91(6):2047–2055. doi: 10.1093/oxfordjournals.jbchem.a133898. [DOI] [PubMed] [Google Scholar]
- Ayres W. A., Small E. W., Isenberg I. A computerized fluorescence anisotropy spectrometer. Anal Biochem. 1974 Apr;58(2):361–367. doi: 10.1016/0003-2697(74)90203-6. [DOI] [PubMed] [Google Scholar]
- Baudier J., Gérard D. The S100-b protein: tyrosine residues do not exhibit an abnormal fluorescence spectrum. J Neurochem. 1983 Jun;40(6):1765–1767. doi: 10.1111/j.1471-4159.1983.tb08154.x. [DOI] [PubMed] [Google Scholar]
- Bradbury E. M., Cary P. D., Chapman G. E., Crane-Robinson C., Danby S. E., Rattle H. W., Boublik M., Palau J., Aviles F. J. Studies on the role and mode of operation of the very-lysine-rich histone H1 (F1) in eukaryote chromatin. The conformation of histone H1. Eur J Biochem. 1975 Apr 1;52(3):605–613. doi: 10.1111/j.1432-1033.1975.tb04032.x. [DOI] [PubMed] [Google Scholar]
- Bustin M. Conservative amino-acid replacement in the tyrosine region of the lysine-rich histones. Eur J Biochem. 1972 Sep 18;29(2):263–267. doi: 10.1111/j.1432-1033.1972.tb01983.x. [DOI] [PubMed] [Google Scholar]
- Gauduchon P., Wahl P. Pulsefluorimetry of tyrosyl peptides. Biophys Chem. 1978 Mar;8(1):87–104. doi: 10.1016/0301-4622(78)85026-1. [DOI] [PubMed] [Google Scholar]
- Giancotti V., Fonda M., Crane-Robinson C. Tyrosine fluorescence of two tryptophan-free proteins: histones H1 and H5. Biophys Chem. 1977 Apr;6(3):379–383. doi: 10.1016/0301-4622(77)85019-9. [DOI] [PubMed] [Google Scholar]
- Graziani M. T., Agrò A. F., Rotilio G., Barra D., Mondovi B. Parsley plastocyanin. The possible presence of sulfhydryl and tyrosine in the copper environment. Biochemistry. 1974 Feb 12;13(4):804–809. doi: 10.1021/bi00701a025. [DOI] [PubMed] [Google Scholar]
- Herskovits T. T., Sorensen M. Studies of the location of tyrosyl and tryptophyl residues in proteins. I. Solvent perturbation data of model compounds. Biochemistry. 1968 Jul;7(7):2523–2532. doi: 10.1021/bi00847a012. [DOI] [PubMed] [Google Scholar]
- Isenberg I., Dyson R. D. The analysis of fluorescence decay by a method of moments. Biophys J. 1969 Nov;9(11):1337–1350. doi: 10.1016/S0006-3495(69)86456-8. [DOI] [PMC free article] [PubMed] [Google Scholar]
- Isenberg I. Histones. Annu Rev Biochem. 1979;48:159–191. doi: 10.1146/annurev.bi.48.070179.001111. [DOI] [PubMed] [Google Scholar]
- Johns E. W. Studies on histones. 7. Preparative methods for histone fractions from calf thymus. Biochem J. 1964 Jul;92(1):55–59. doi: 10.1042/bj0920055. [DOI] [PMC free article] [PubMed] [Google Scholar]
- Jordano J., Barbero J. L., Montero F., Franco L. Fluorescence of histones H1. A tyrosinate-like fluorescence emission in Ceratitis capitata H1 at neutral pH values. J Biol Chem. 1983 Jan 10;258(1):315–320. [PubMed] [Google Scholar]
- Kilhoffer M. C., Demaille J. G., Gérard D. Tyrosine fluorescence of ram testis and octopus calmodulins. Effects of calcium, magnesium, and ionic strength. Biochemistry. 1981 Jul 21;20(15):4407–4414. doi: 10.1021/bi00518a027. [DOI] [PubMed] [Google Scholar]
- Lakowicz J. R., Maliwal B. P. Oxygen quenching and fluorescence depolarization of tyrosine residues in proteins. J Biol Chem. 1983 Apr 25;258(8):4794–4801. [PubMed] [Google Scholar]
- Lehrer S. S., Fasman G. D. Ultraviolet irradiation effects in poly-L-tyrosine and model compounds. Identification of bityrosine as a photoproduct. Biochemistry. 1967 Mar;6(3):757–767. doi: 10.1021/bi00855a017. [DOI] [PubMed] [Google Scholar]
- Liao L. W., Cole R. D. The amino acid sequence of residues 1-104 of CTL-1, a bovine H1 histone. J Biol Chem. 1981 Mar 25;256(6):3024–3029. [PubMed] [Google Scholar]
- Libertini L. J., Small E. W. Effects of pH on low-salt transition of chromatin core particles. Biochemistry. 1982 Jul 6;21(14):3327–3334. doi: 10.1021/bi00257a013. [DOI] [PubMed] [Google Scholar]
- Libertini L. J., Small E. W. Effects of pH on the stability of chromatin core particles. Nucleic Acids Res. 1984 May 25;12(10):4351–4359. doi: 10.1093/nar/12.10.4351. [DOI] [PMC free article] [PubMed] [Google Scholar]
- Libertini L. J., Small E. W. Salt induced transitions of chromatin core particles studied by tyrosine fluorescence anisotropy. Nucleic Acids Res. 1980 Aug 25;8(16):3517–3534. doi: 10.1093/nar/8.16.3517. [DOI] [PMC free article] [PubMed] [Google Scholar]
- Lim B. T., Kimura T. Conformation-associated anomalous tyrosine fluorescence of adrenodoxin. J Biol Chem. 1980 Mar 25;255(6):2440–2444. [PubMed] [Google Scholar]
- Longworth J. W. A new component in protein fluorescence. Ann N Y Acad Sci. 1981;366:237–245. doi: 10.1111/j.1749-6632.1981.tb20757.x. [DOI] [PubMed] [Google Scholar]
- Mani R. S., Boyes B. E., Kay C. M. Physicochemical and optical studies on calcium- and potassium-induced conformational changes in bovine brain S-100b protein. Biochemistry. 1982 May 25;21(11):2607–2612. doi: 10.1021/bi00540a005. [DOI] [PubMed] [Google Scholar]
- Padrós E., Morros A., Mañosa J., Duñach M. The state of tyrosine and phenylalanine residues in proteins analyzed by fourth-derivative spectrophotometry. Histone H1 and ribonuclease A. Eur J Biochem. 1982 Sep;127(1):117–122. doi: 10.1111/j.1432-1033.1982.tb06844.x. [DOI] [PubMed] [Google Scholar]
- Smerdon M. J., Isenberg I. Conformational changes in subfractions of calf thymus histone H1. Biochemistry. 1976 Sep 21;15(19):4233–4242. doi: 10.1021/bi00664a016. [DOI] [PubMed] [Google Scholar]
- Tournon J., Kuntz E., el-Bayoumi M. A. Fluorescence quenching in phenylalanine and model compounds. Photochem Photobiol. 1972 Nov;16(5):425–433. doi: 10.1111/j.1751-1097.1972.tb06310.x. [DOI] [PubMed] [Google Scholar]