Abstract
Alamethicin, a 20-amino acid peptide, has been studied for a number of years as a model for voltage-gated channels. Recently both the x-ray structure of alamethicin in crystal and an NMR solution structure have been published (Fox and Richards, 1982. Bannerjee et al., 1983). Both structures show that the amino end of the molecule forms a stable alpha-helix nine or 10 residues in length and that the COOH-terminal ends exhibits a variable hydrogen bonding pattern. We have used synthetic analogues of alamethicin to test various hypotheses of its mode of action. As a result of these studies we propose a channel structure in which the COOH-terminal residues bond together as a beta-barrel, leaving the alpha- helices free to rotate under the influence of the electric field and gate the channel. Though the number of monomers per channel varies with experimental conditions, the gating charge per monomer stays close to that expected from an alpha-helical gate. We can also alter the sign of the voltage which turns on a channel by varying the charge on the alamethicin analogue. Channels are always slightly cation-selective even though formed by monomers with negative, positive, or zero formal charge. Channels are less stable in low ionic strength solutions than high. Finally, alamethicin conductance parameters vary systematically with changes in membrane thickness. We show how these results and others in the literature can be explained by a fairly detailed structural model. The model can be easily generalized to a form more suited to high molecular weight single-peptide-chain proteins.
Full text
PDF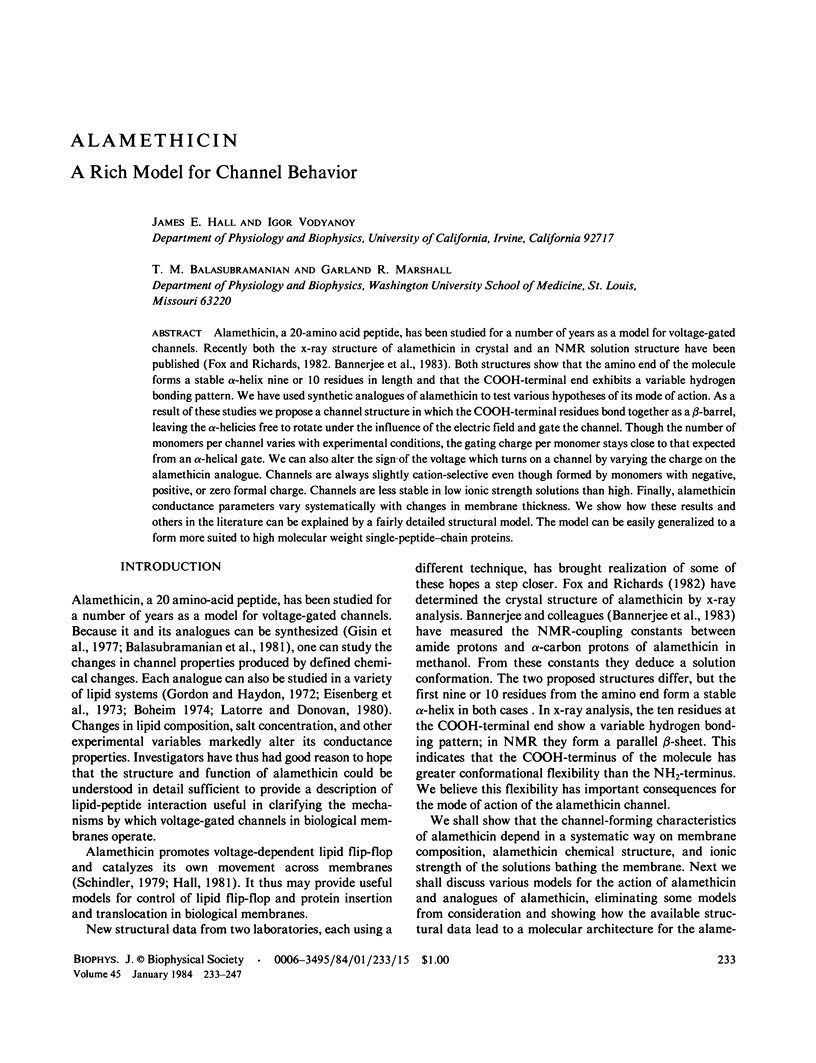
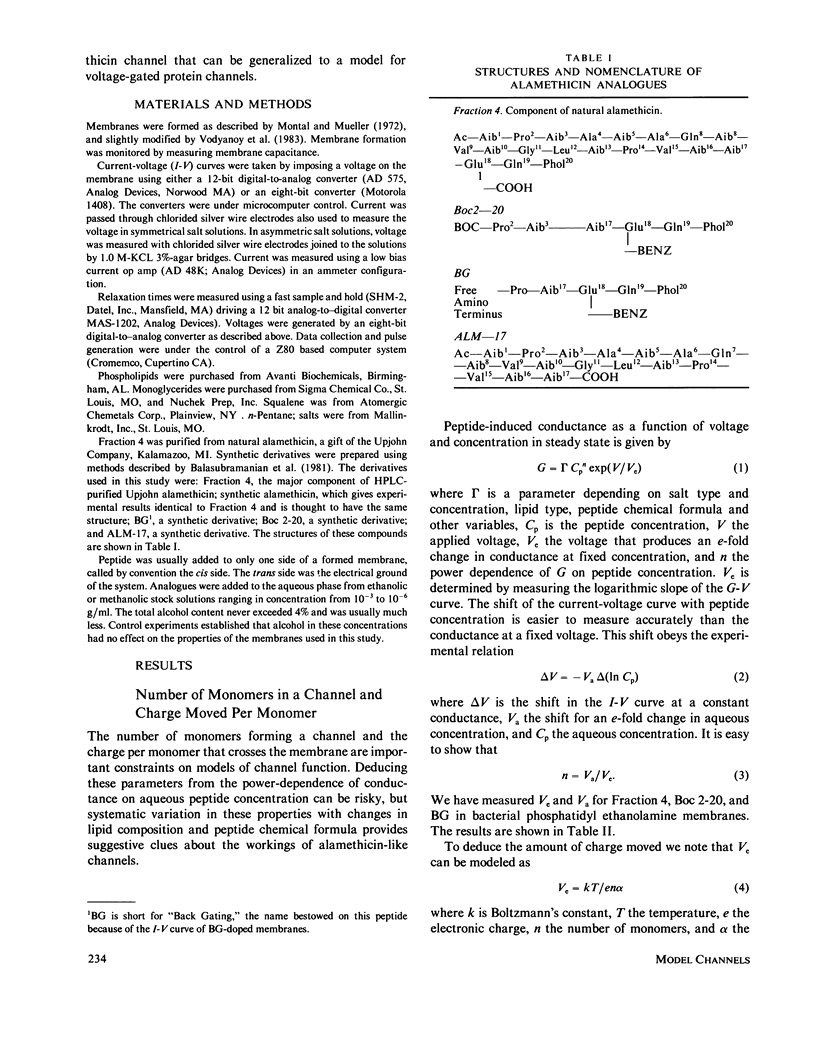
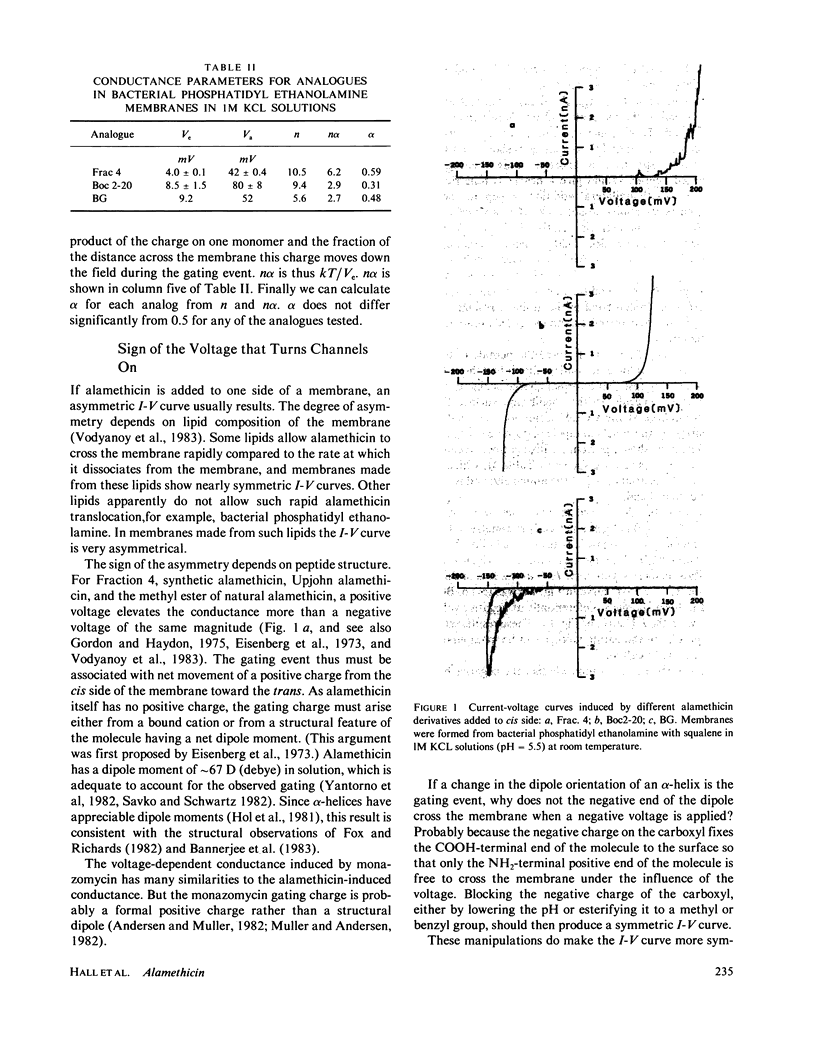
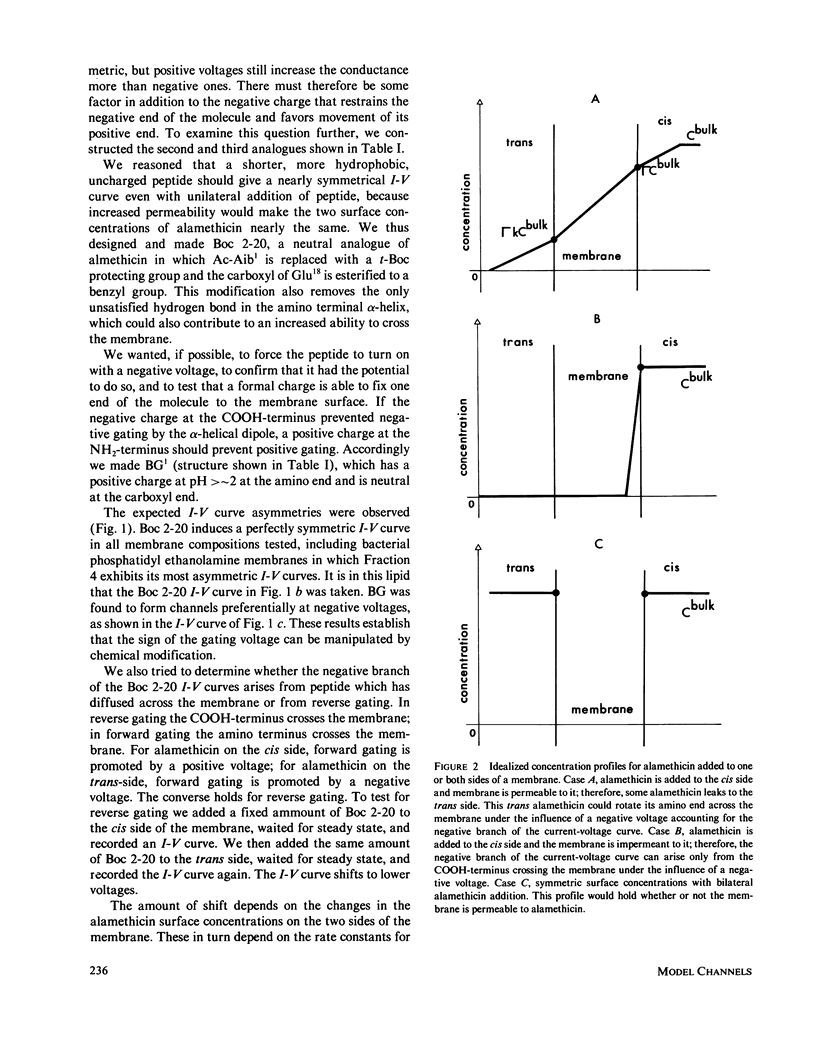
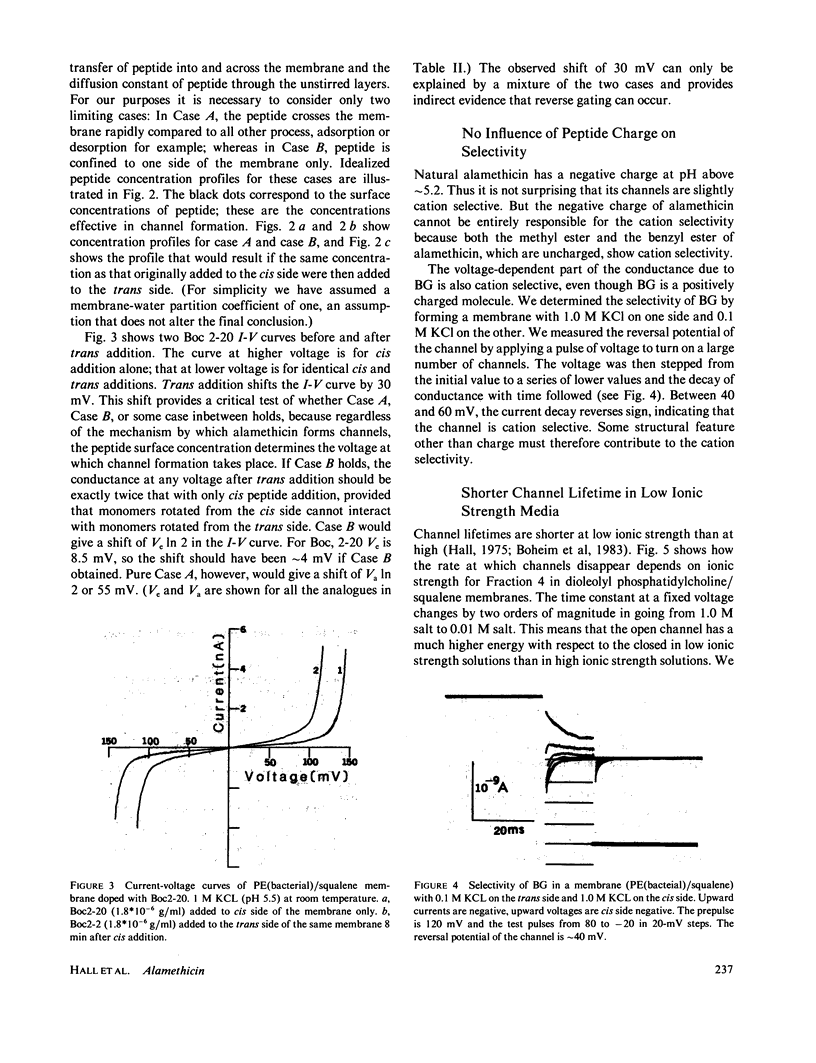
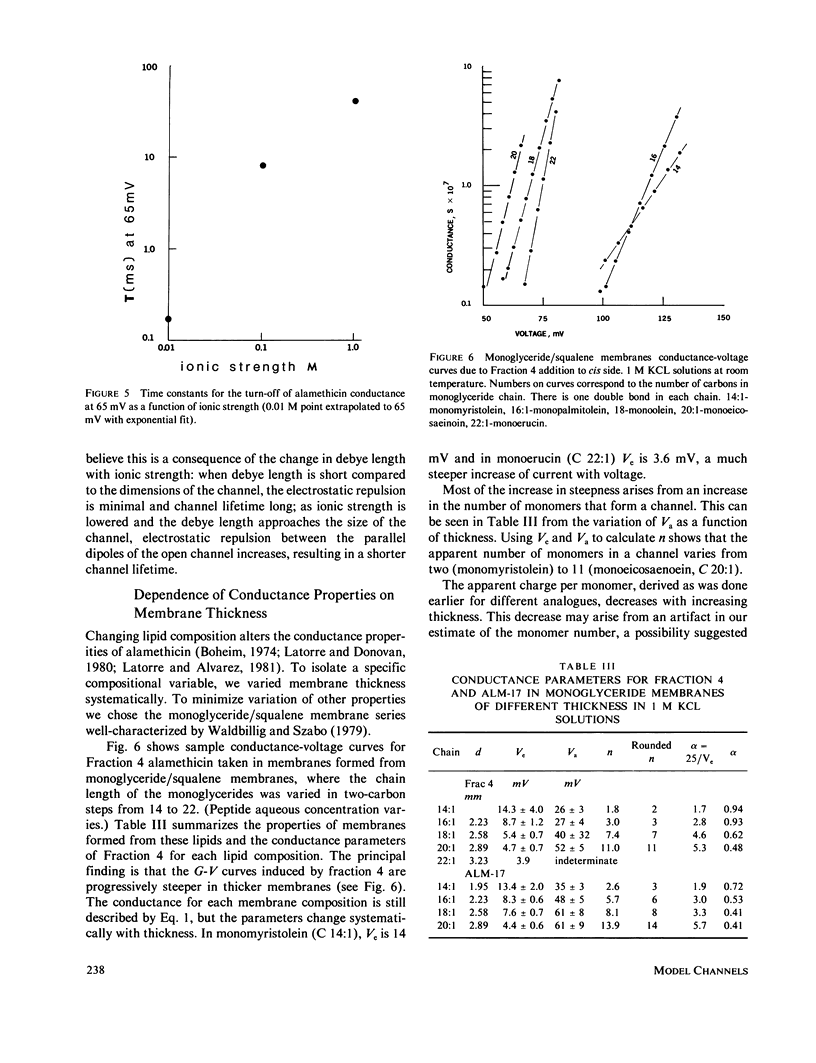
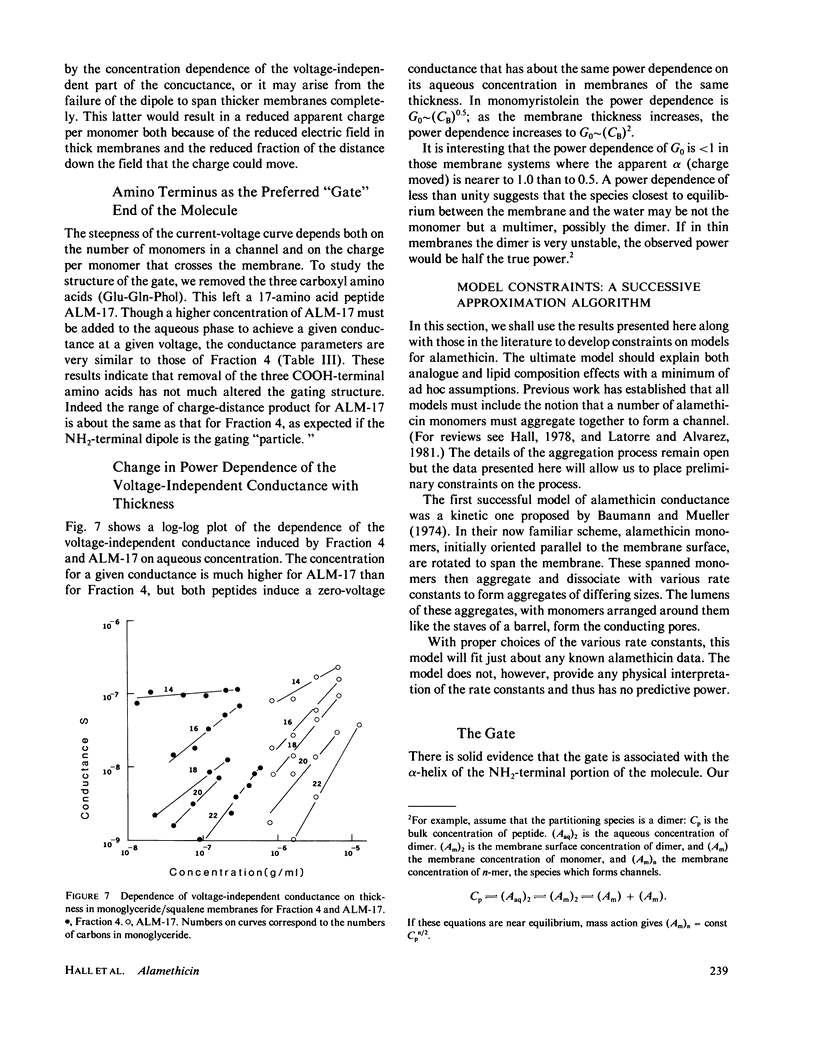
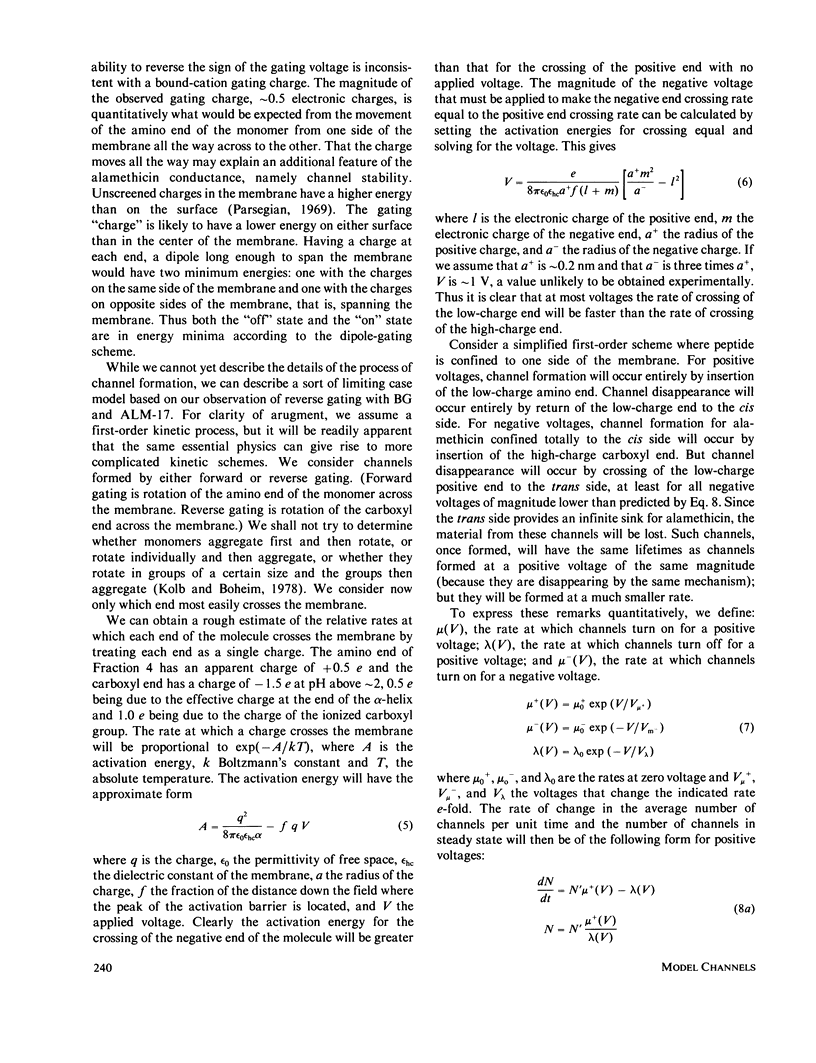
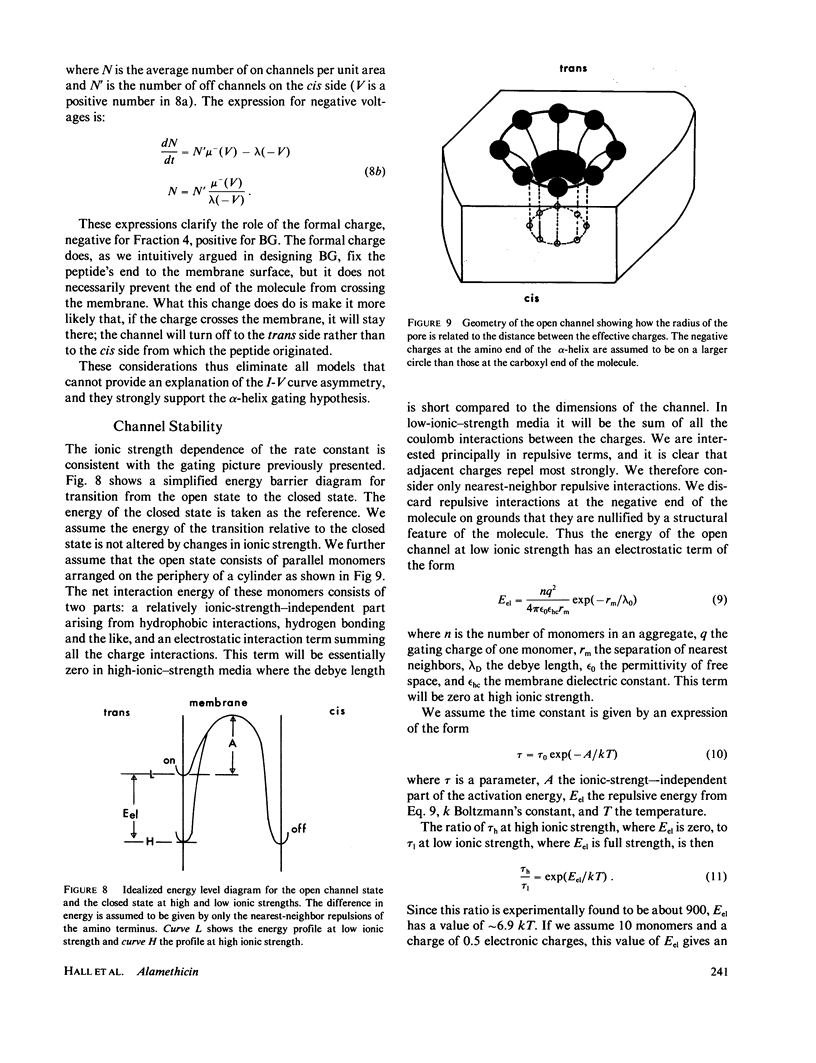
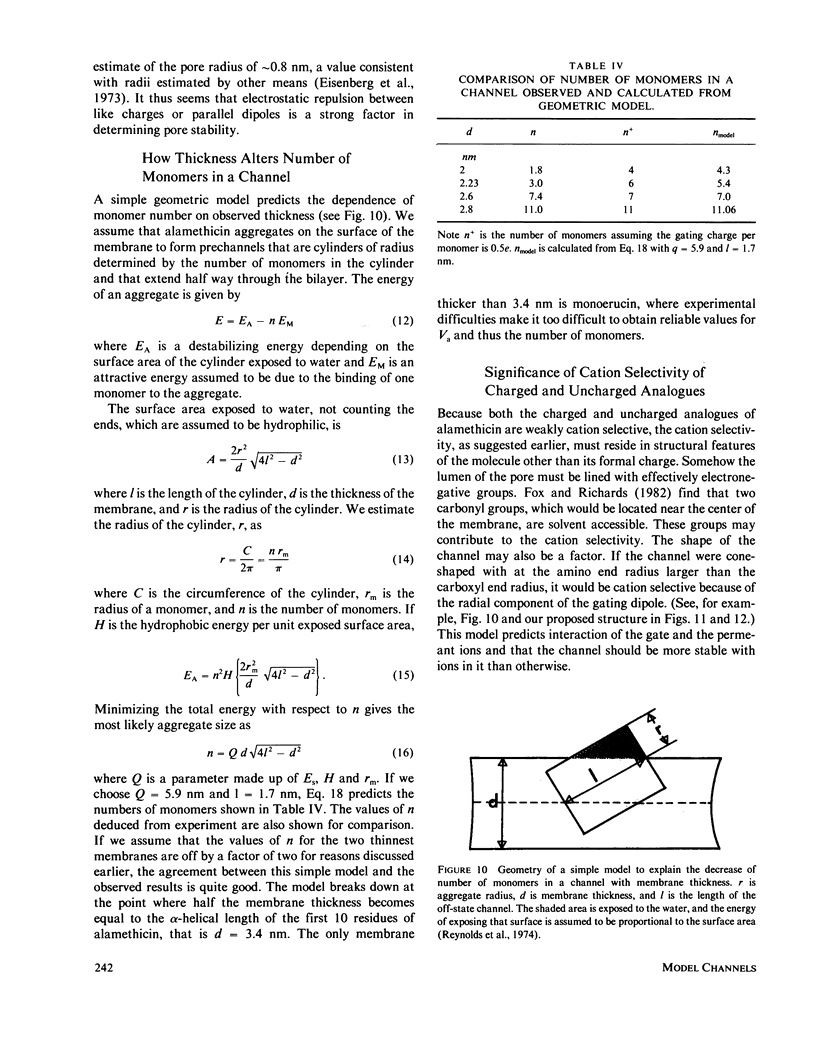
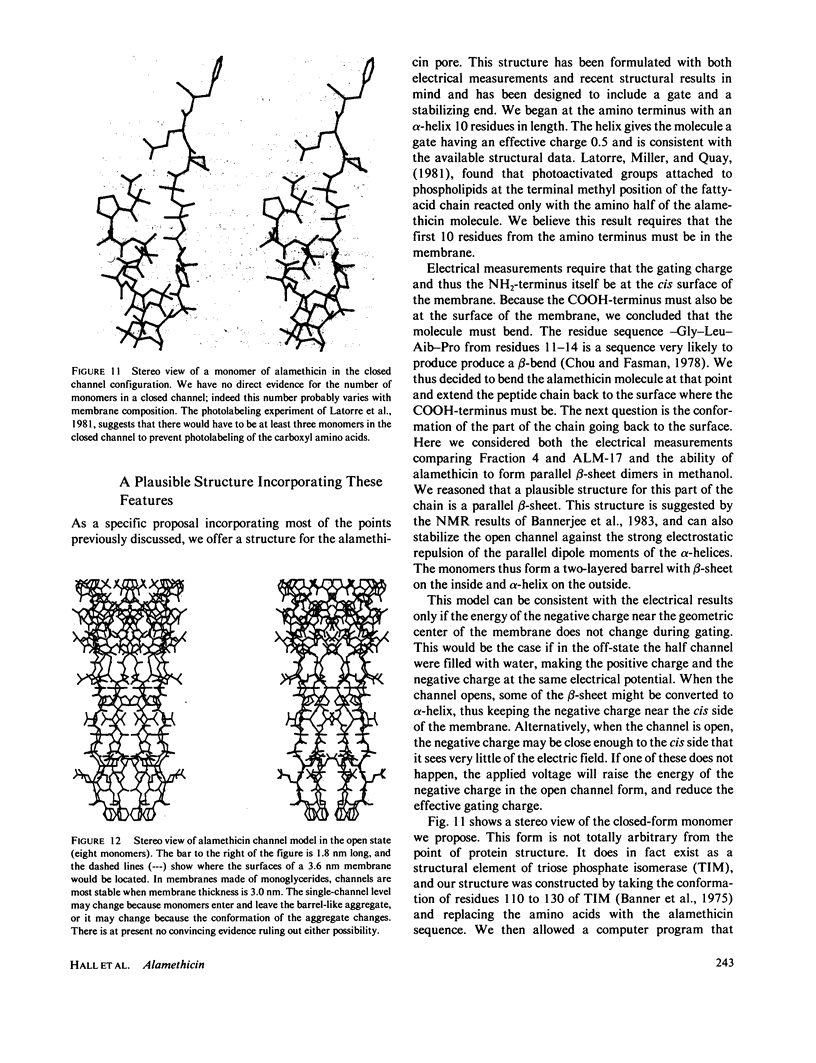
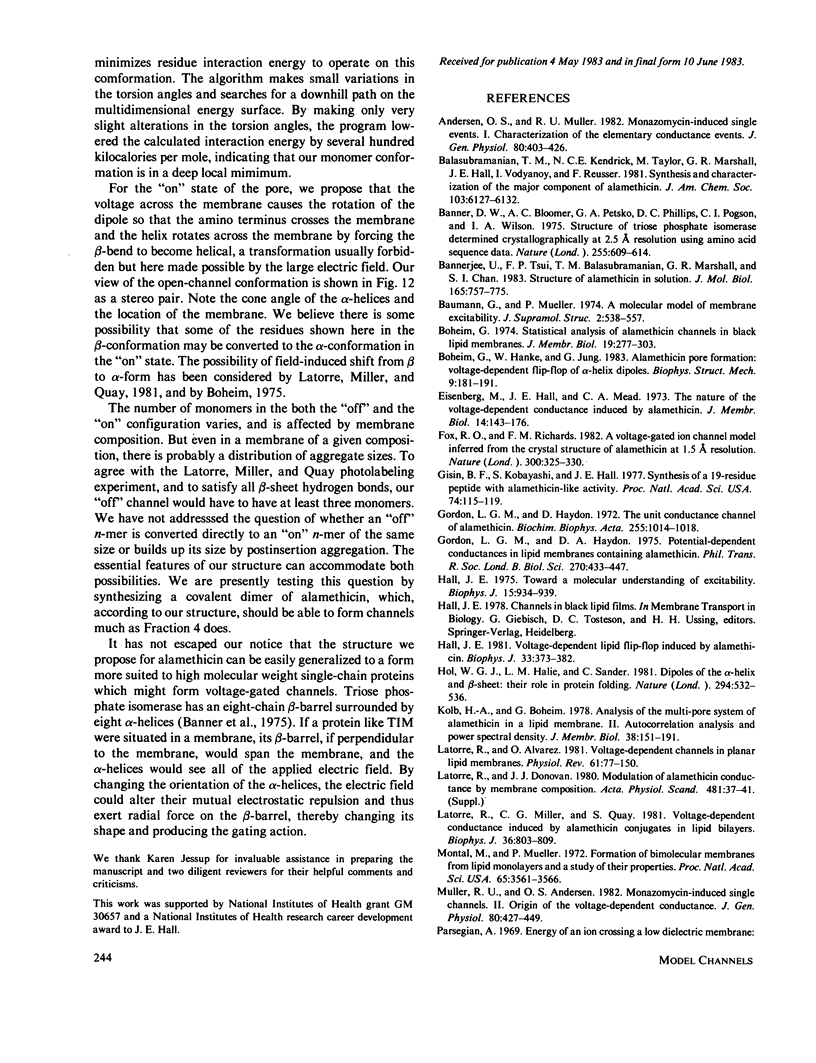
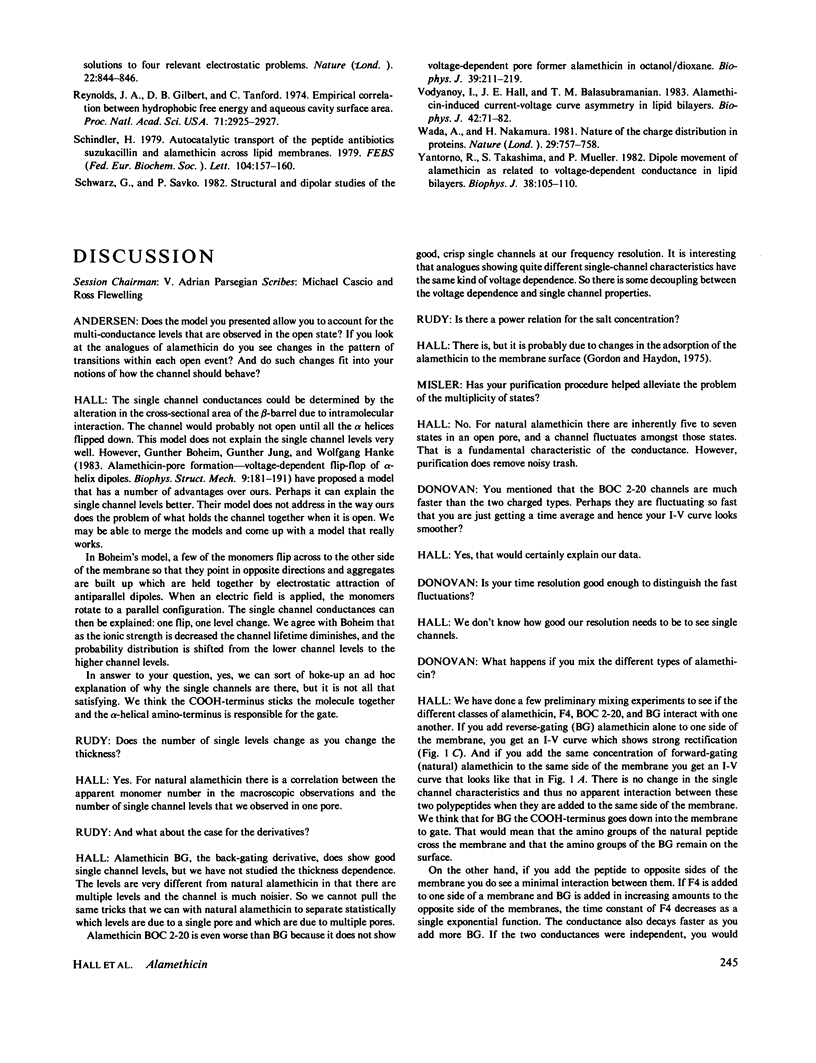
Selected References
These references are in PubMed. This may not be the complete list of references from this article.
- Andersen O. S., Muller R. U. Monazomycin-induced single channels. I. Characterization of the elementary conductance events. J Gen Physiol. 1982 Sep;80(3):403–426. doi: 10.1085/jgp.80.3.403. [DOI] [PMC free article] [PubMed] [Google Scholar]
- Banerjee U., Tsui F. P., Balasubramanian T. N., Marshall G. R., Chan S. I. Structure of Alamethicin in solution. One- and two-dimensional 1H nuclear magnetic resonance studies at 500 MHz. J Mol Biol. 1983 Apr 25;165(4):757–775. doi: 10.1016/s0022-2836(83)80279-4. [DOI] [PubMed] [Google Scholar]
- Banner D. W., Bloomer A. C., Petsko G. A., Phillips D. C., Pogson C. I., Wilson I. A., Corran P. H., Furth A. J., Milman J. D., Offord R. E. Structure of chicken muscle triose phosphate isomerase determined crystallographically at 2.5 angstrom resolution using amino acid sequence data. Nature. 1975 Jun 19;255(5510):609–614. doi: 10.1038/255609a0. [DOI] [PubMed] [Google Scholar]
- Baumann G., Mueller P. A molecular model of membrane excitability. J Supramol Struct. 1974;2(5-6):538–557. doi: 10.1002/jss.400020504. [DOI] [PubMed] [Google Scholar]
- Boheim G. Statistical analysis of alamethicin channels in black lipid membranes. J Membr Biol. 1974;19(3):277–303. doi: 10.1007/BF01869983. [DOI] [PubMed] [Google Scholar]
- Eisenberg M., Hall J. E., Mead C. A. The nature of the voltage-dependent conductance induced by alamethicin in black lipid membranes. J Membr Biol. 1973 Dec 31;14(2):143–176. doi: 10.1007/BF01868075. [DOI] [PubMed] [Google Scholar]
- Fox R. O., Jr, Richards F. M. A voltage-gated ion channel model inferred from the crystal structure of alamethicin at 1.5-A resolution. Nature. 1982 Nov 25;300(5890):325–330. doi: 10.1038/300325a0. [DOI] [PubMed] [Google Scholar]
- Gisin B. F., Kobayashi S., Hall J. E. Synthesis of a 19-residue peptide with alamethicin-like activity. Proc Natl Acad Sci U S A. 1977 Jan;74(1):115–119. doi: 10.1073/pnas.74.1.115. [DOI] [PMC free article] [PubMed] [Google Scholar]
- Gordon L. G., Haydon D. A. Potential-dependent conductances in lipid membranes containing alamethicin. Philos Trans R Soc Lond B Biol Sci. 1975 Jun 10;270(908):433–447. doi: 10.1098/rstb.1975.0021. [DOI] [PubMed] [Google Scholar]
- Gordon L. G., Haydon D. A. The unit conductance channel of alamethicin. Biochim Biophys Acta. 1972 Mar 17;255(3):1014–1018. doi: 10.1016/0005-2736(72)90415-4. [DOI] [PubMed] [Google Scholar]
- Hall J. E. Toward a molecular understanding of excitability. Alamethicin in black lipid films. Biophys J. 1975 Sep;15(9):934–939. doi: 10.1016/S0006-3495(75)85869-3. [DOI] [PMC free article] [PubMed] [Google Scholar]
- Hall J. E. Voltage-dependent lipid flip-flop induced by alamethicin. Biophys J. 1981 Mar;33(3):373–381. doi: 10.1016/S0006-3495(81)84901-6. [DOI] [PMC free article] [PubMed] [Google Scholar]
- Hol W. G., Halie L. M., Sander C. Dipoles of the alpha-helix and beta-sheet: their role in protein folding. Nature. 1981 Dec 10;294(5841):532–536. doi: 10.1038/294532a0. [DOI] [PubMed] [Google Scholar]
- Latorre R., Alvarez O. Voltage-dependent channels in planar lipid bilayer membranes. Physiol Rev. 1981 Jan;61(1):77–150. doi: 10.1152/physrev.1981.61.1.77. [DOI] [PubMed] [Google Scholar]
- Latorre R., Donovan J. J. Modulation of alamethicin-induced conductance by membrane composition. Acta Physiol Scand Suppl. 1980;481:37–45. [PubMed] [Google Scholar]
- Latorre R., Miller C. G., Quay S. Voltage-dependent conductance induced by alamethicin-phospholipid conjugates in lipid bilayers. Biophys J. 1981 Dec;36(3):803–809. doi: 10.1016/S0006-3495(81)84767-4. [DOI] [PMC free article] [PubMed] [Google Scholar]
- Montal M., Mueller P. Formation of bimolecular membranes from lipid monolayers and a study of their electrical properties. Proc Natl Acad Sci U S A. 1972 Dec;69(12):3561–3566. doi: 10.1073/pnas.69.12.3561. [DOI] [PMC free article] [PubMed] [Google Scholar]
- Muller R. U., Andersen O. S. Monazomycin-induced single channels. II. Origin of the voltage dependence of the macroscopic conductance. J Gen Physiol. 1982 Sep;80(3):427–449. doi: 10.1085/jgp.80.3.427. [DOI] [PMC free article] [PubMed] [Google Scholar]
- Reynolds J. A., Gilbert D. B., Tanford C. Empirical correlation between hydrophobic free energy and aqueous cavity surface area. Proc Natl Acad Sci U S A. 1974 Aug;71(8):2925–2927. doi: 10.1073/pnas.71.8.2925. [DOI] [PMC free article] [PubMed] [Google Scholar]
- Schindler H. Autocatalytic transport of the peptide antibiotics suzukacillin and alamethicin across lipid membranes. FEBS Lett. 1979 Aug 1;104(1):157–160. doi: 10.1016/0014-5793(79)81105-9. [DOI] [PubMed] [Google Scholar]
- Schwarz G., Savko P. Structural and dipolar properties of the voltage-dependent pore former alamethicin in octanol/dioxane. Biophys J. 1982 Aug;39(2):211–219. doi: 10.1016/S0006-3495(82)84510-4. [DOI] [PMC free article] [PubMed] [Google Scholar]
- Vodyanoy I., Hall J. E., Balasubramanian T. M. Alamethicin-induced current-voltage curve asymmetry in lipid bilayers. Biophys J. 1983 Apr;42(1):71–82. doi: 10.1016/S0006-3495(83)84370-7. [DOI] [PMC free article] [PubMed] [Google Scholar]
- Wada A., Nakamura H. Nature of the charge distribution in proteins. Nature. 1981 Oct 29;293(5835):757–758. doi: 10.1038/293757a0. [DOI] [PubMed] [Google Scholar]
- Yantorno R., Takashima S., Mueller P. Dipole moment of alamethicin as related to voltage-dependent conductance in lipid bilayers. Biophys J. 1982 May;38(2):105–110. doi: 10.1016/S0006-3495(82)84536-0. [DOI] [PMC free article] [PubMed] [Google Scholar]