Abstract
It has been repeatedly postulated that the high heat resistance of bacterial spores is due to stabilization of biopolymers in the spore interior by a solid deposit of protective cement consisting of coordination complexes of ligands with divalent metal ions. This report presents data on metal-binding characteristics of some of the ligands related to spores as determined by means of potentiometric equilibrium measurements under conditions of temperature and ionic strength (t = 25.0°C; μ = 1.0 KNO3) identical with those reported earlier by the authors in order to facilitate correlation by using comparable data. The spore ligands investigated in this study included 2,6-pyridinedicarboxylic acid (DPA), α,ε-diaminopimelic acid, D-glutamic acid, and D-alanine in a ratio of 1:1 with metal ions which are known to play a role in heat resistance of spores. Stability constants of the chelates of these spore ligands with metal ions such as Ca(II), Mg(II), Cu(II), Ni(II), Zn(II), Co(II), and Mn(II) have been determined. In general the metal chelates of DPA exhibited the greatest stability. On the basis of a consideration of the stability data together with the known configurations of the ligand and the coordination requirements of the metal ions, possible structures indicating the coordinate binding of the spore ligands with the metal ions are presented. All the metal chelates except those of Ca(II) were found to undergo hydrolysis and separation of solid phase in the pH range 7-8.5. The relatively greater hydrolytic stability of Ca(II) chelates and the high affinity of DPA for metal ions appear to be of biological significance insofar as these two spore components are more widely associated with the heat resistance of bacterial spores.
Full text
PDF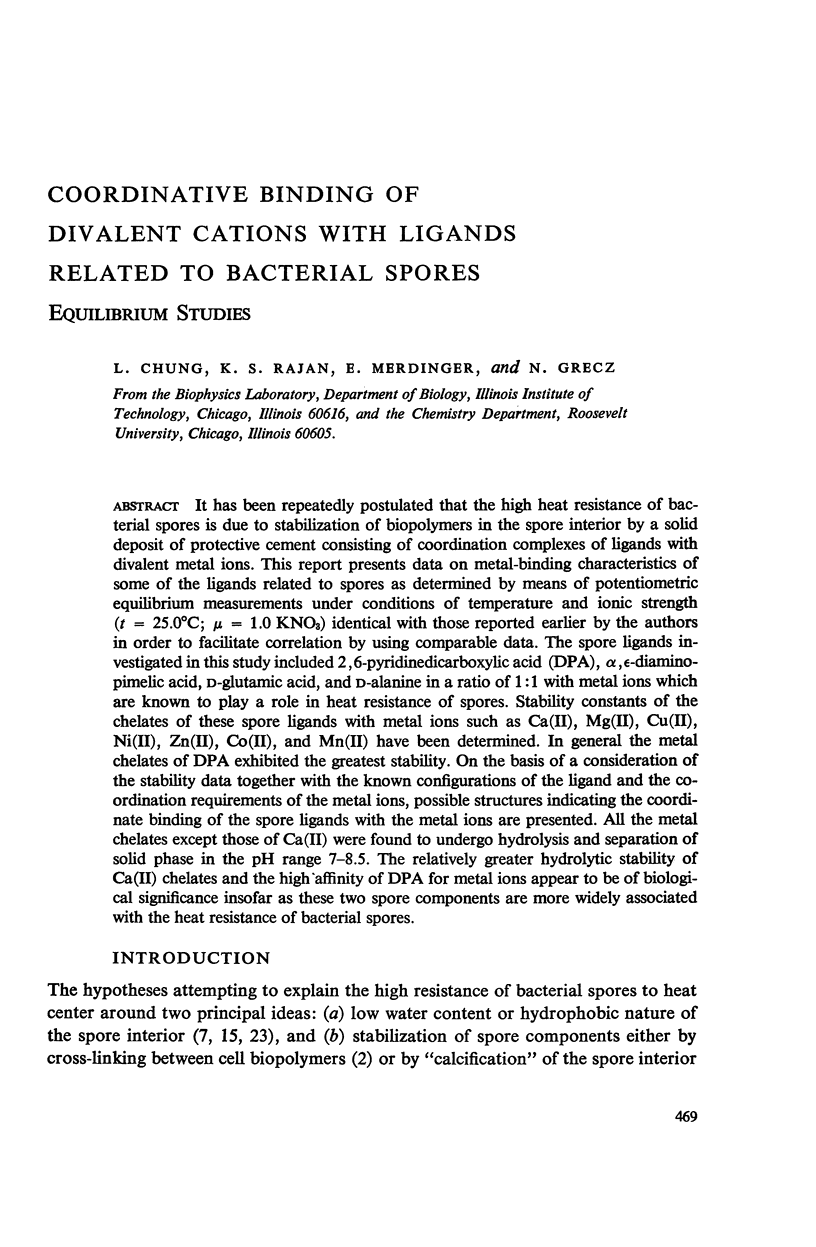
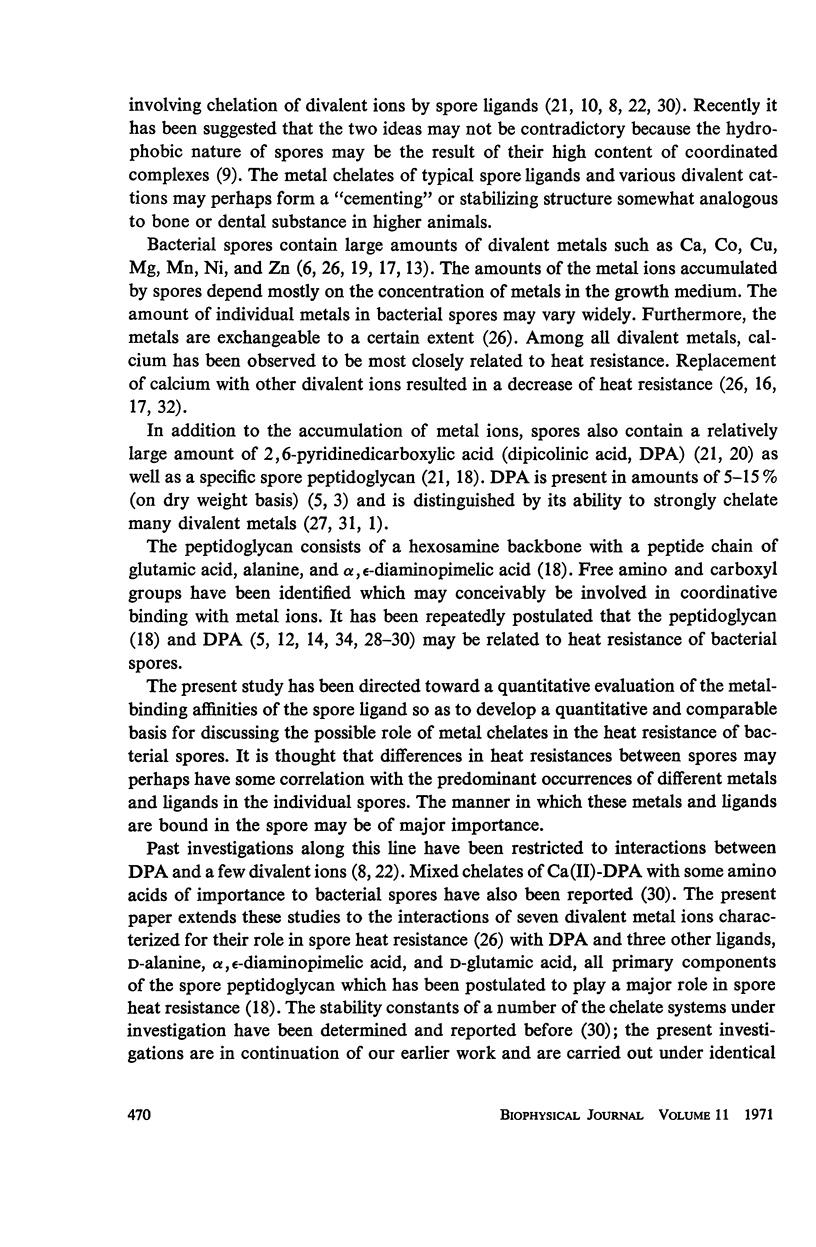
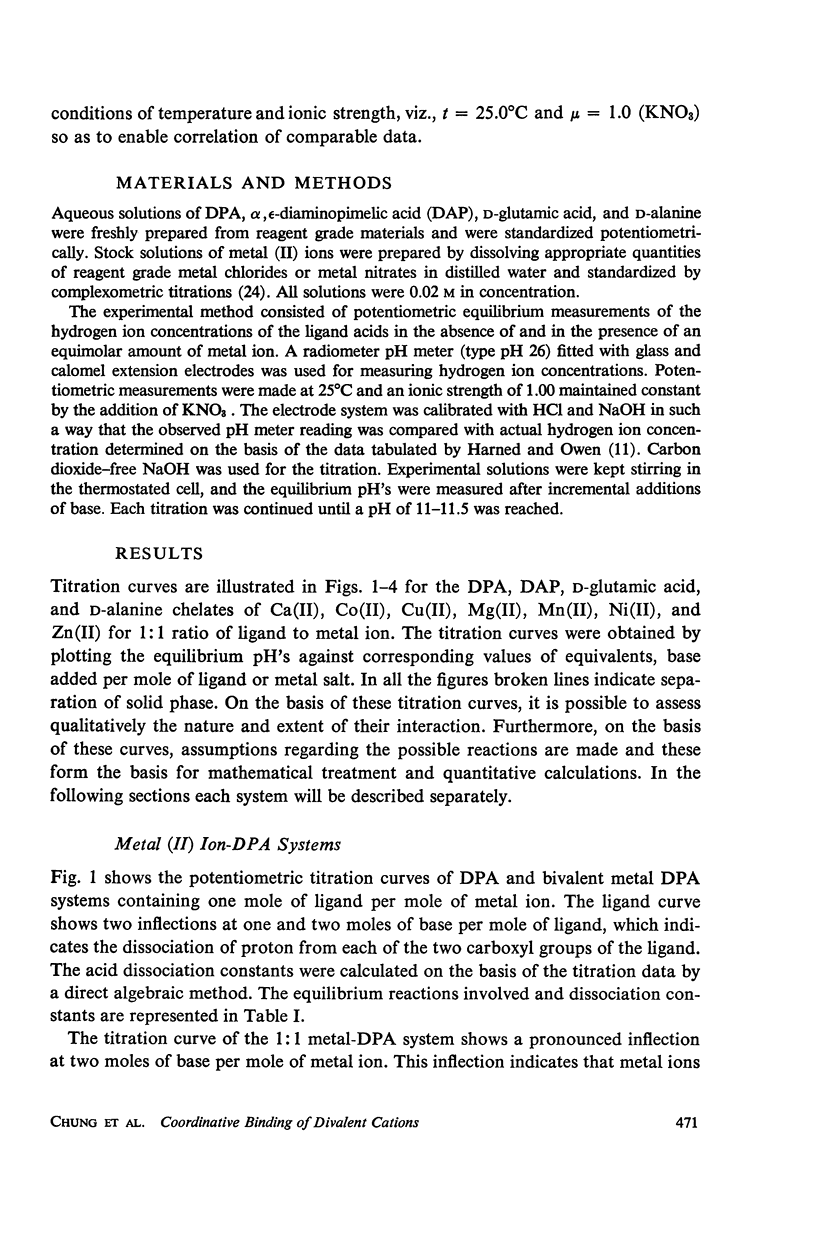
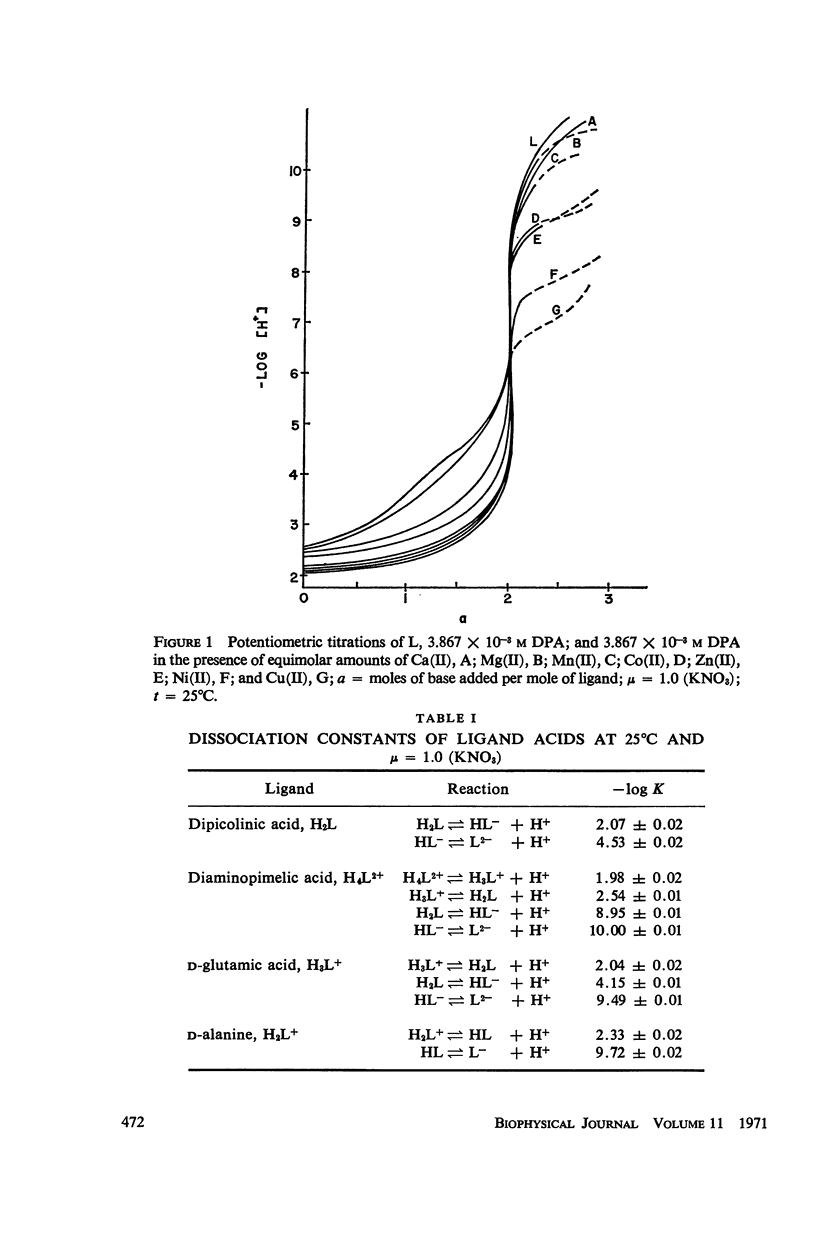
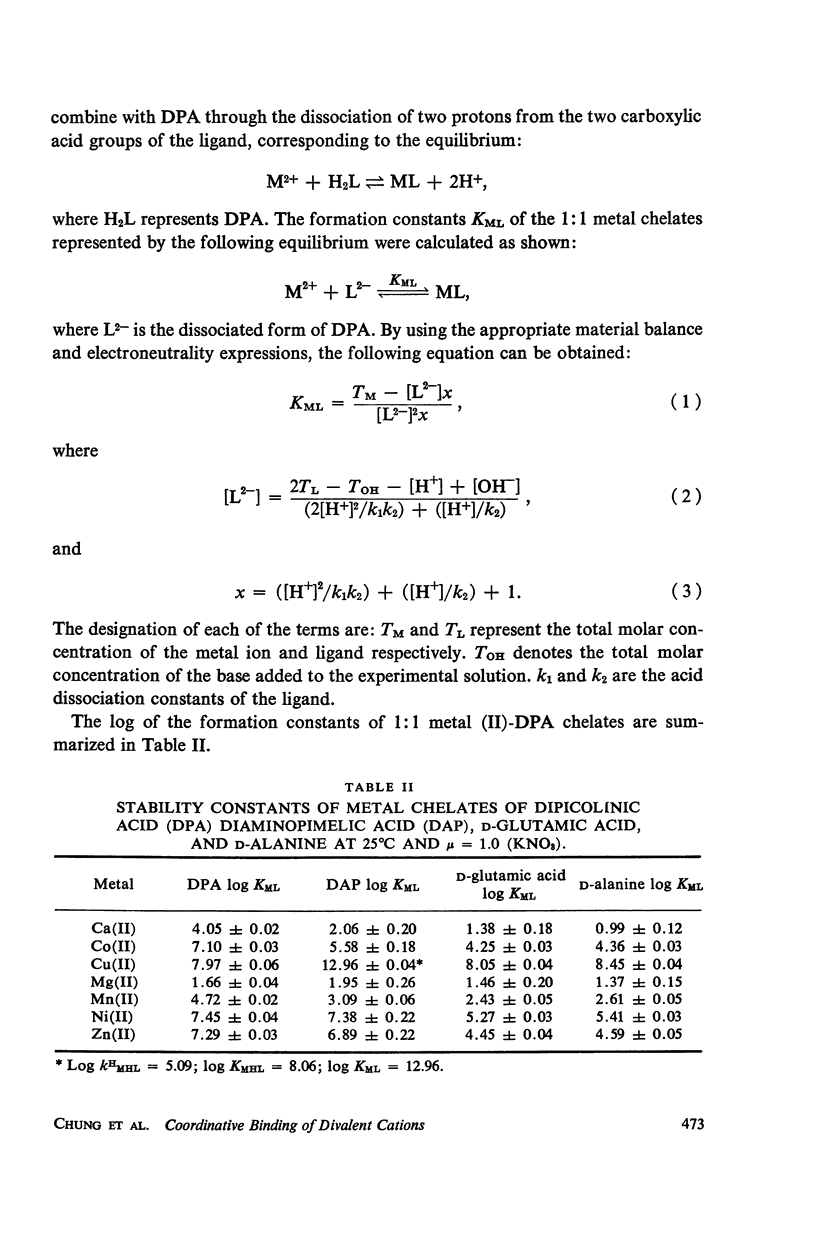
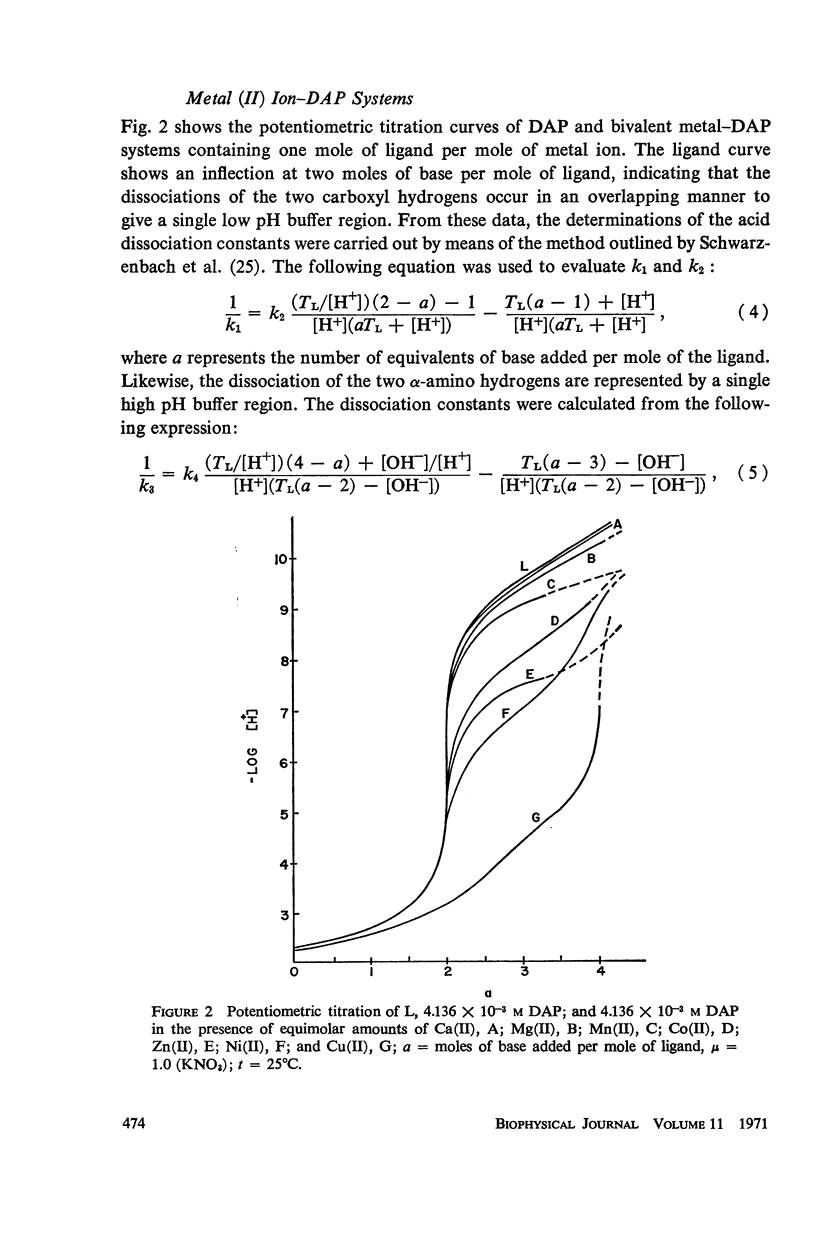
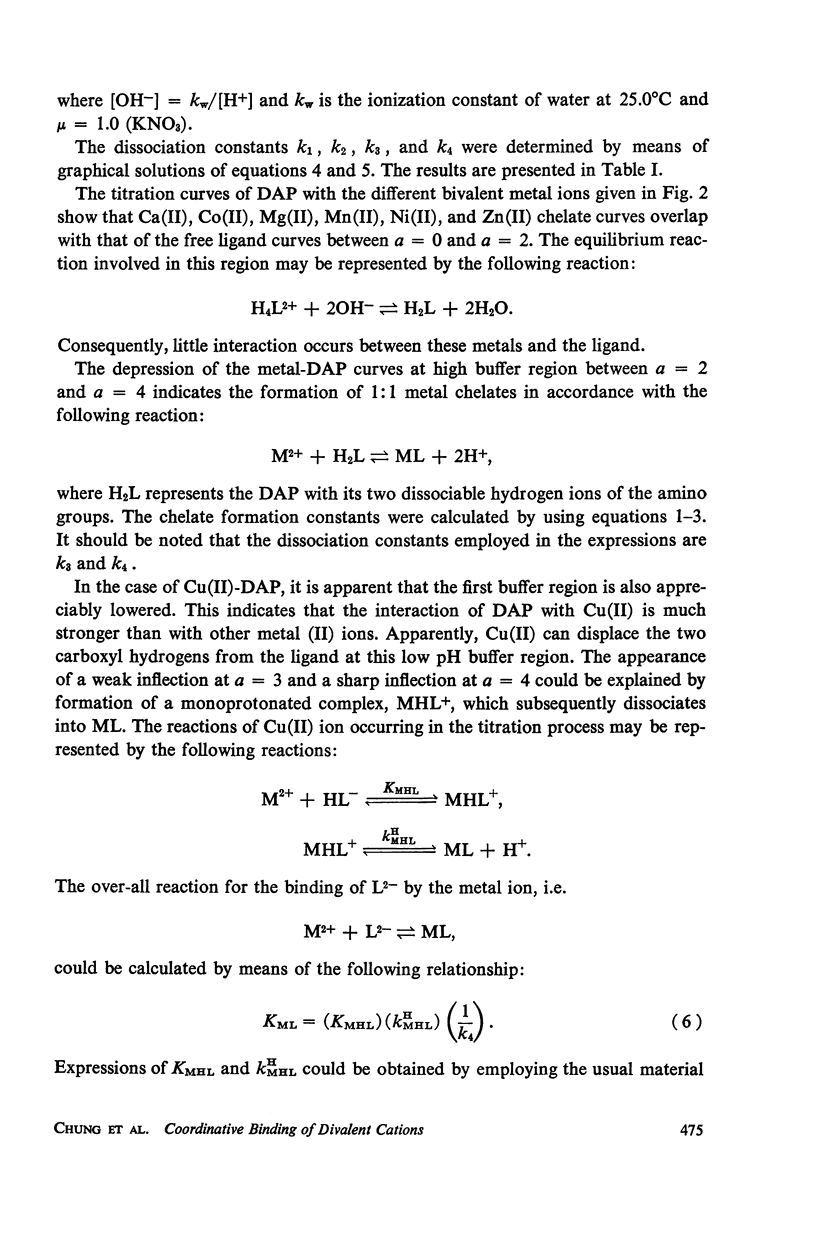
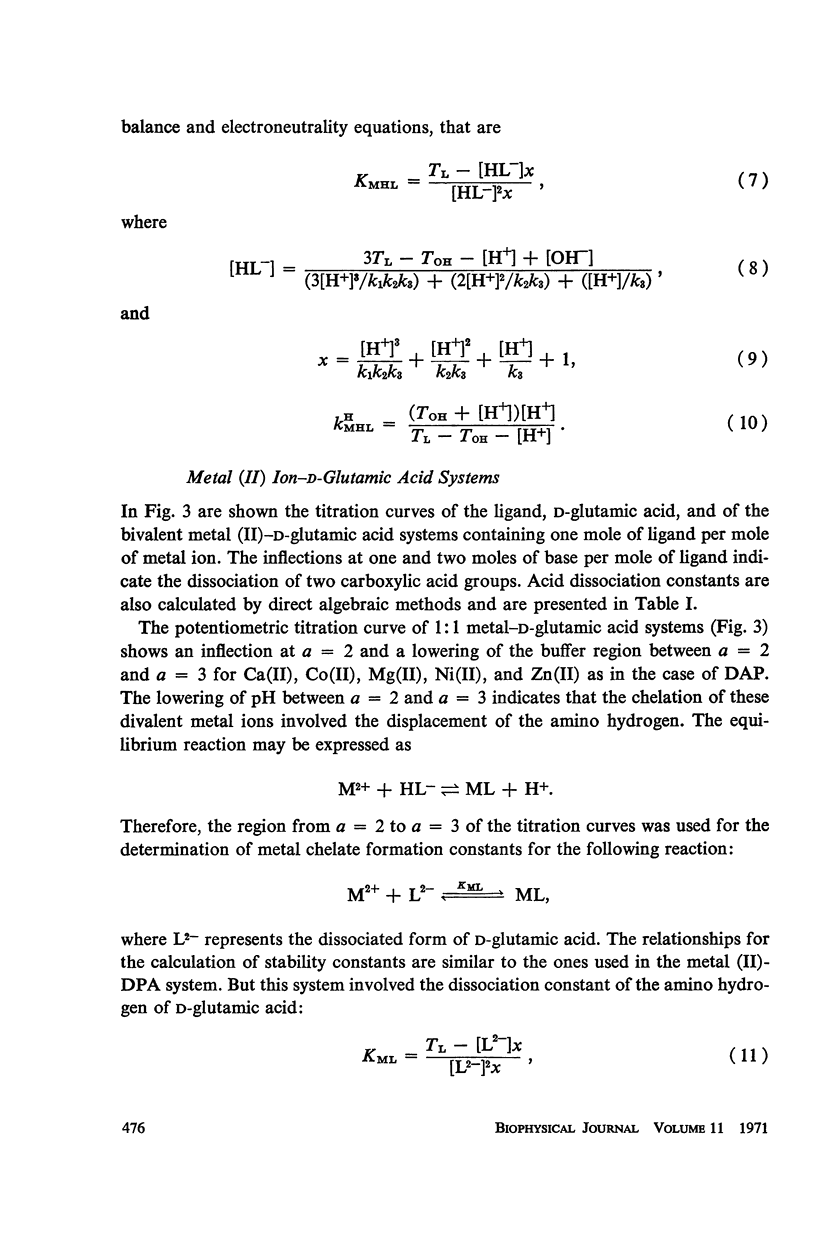
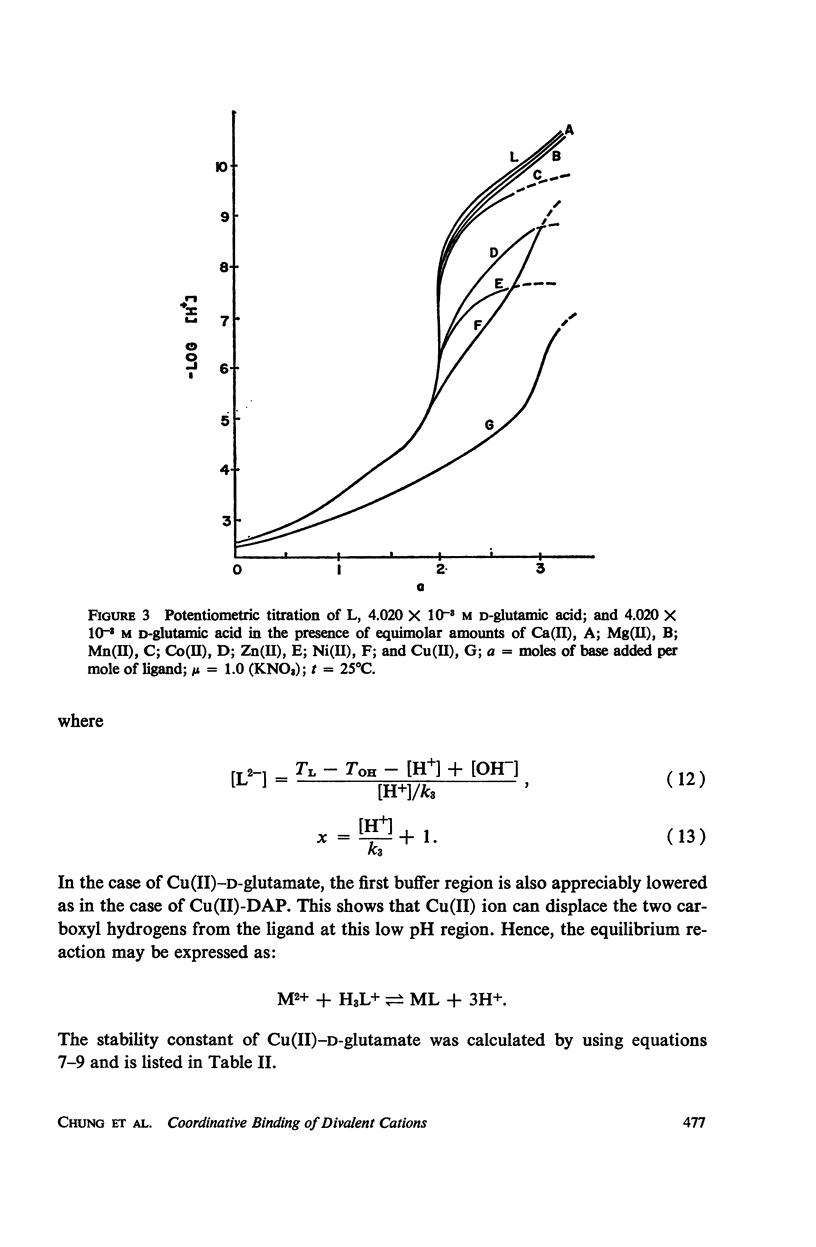
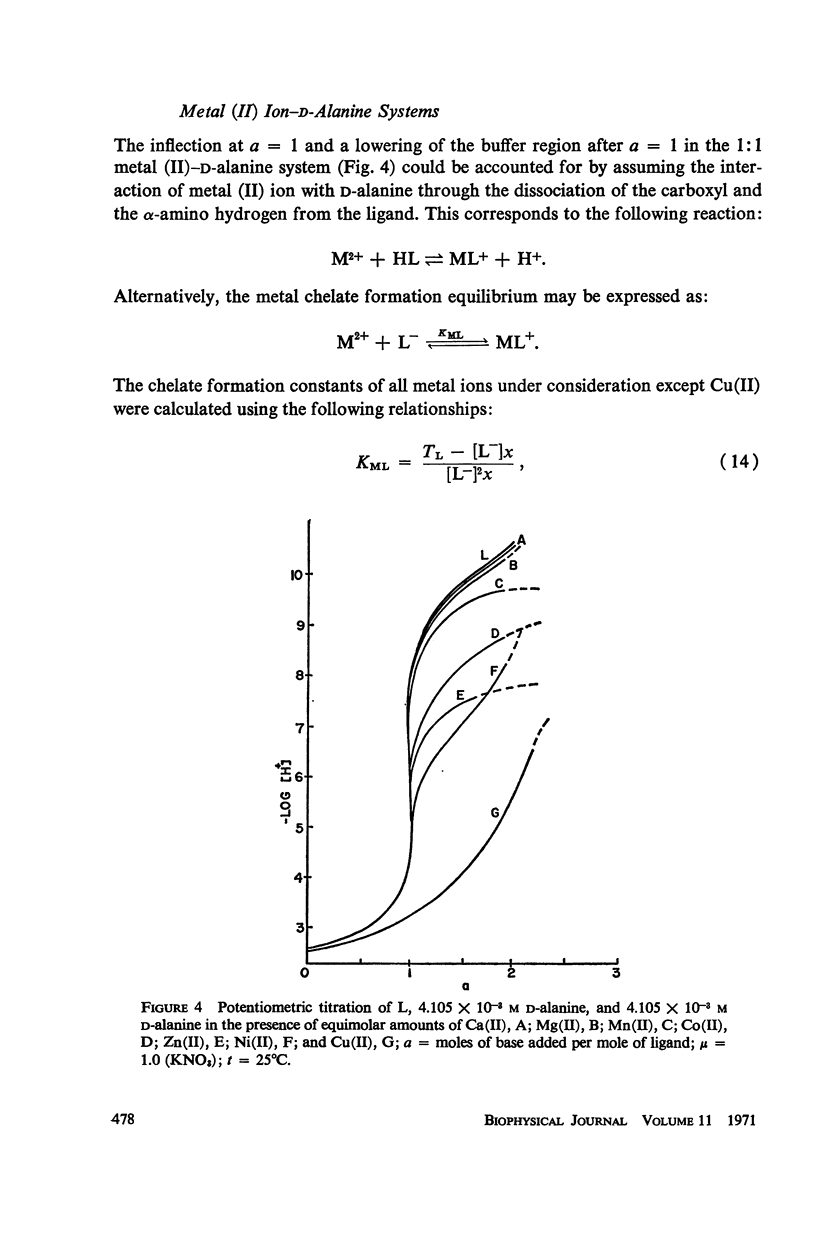
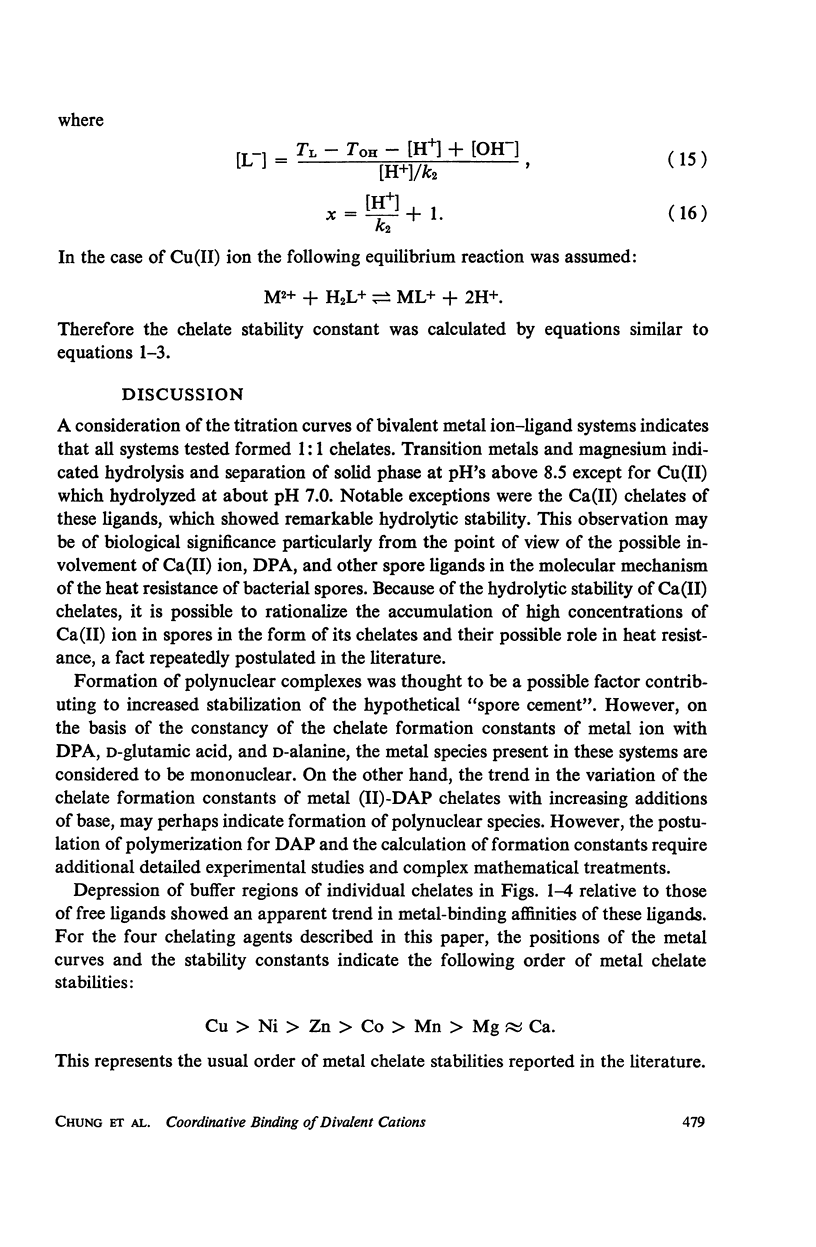
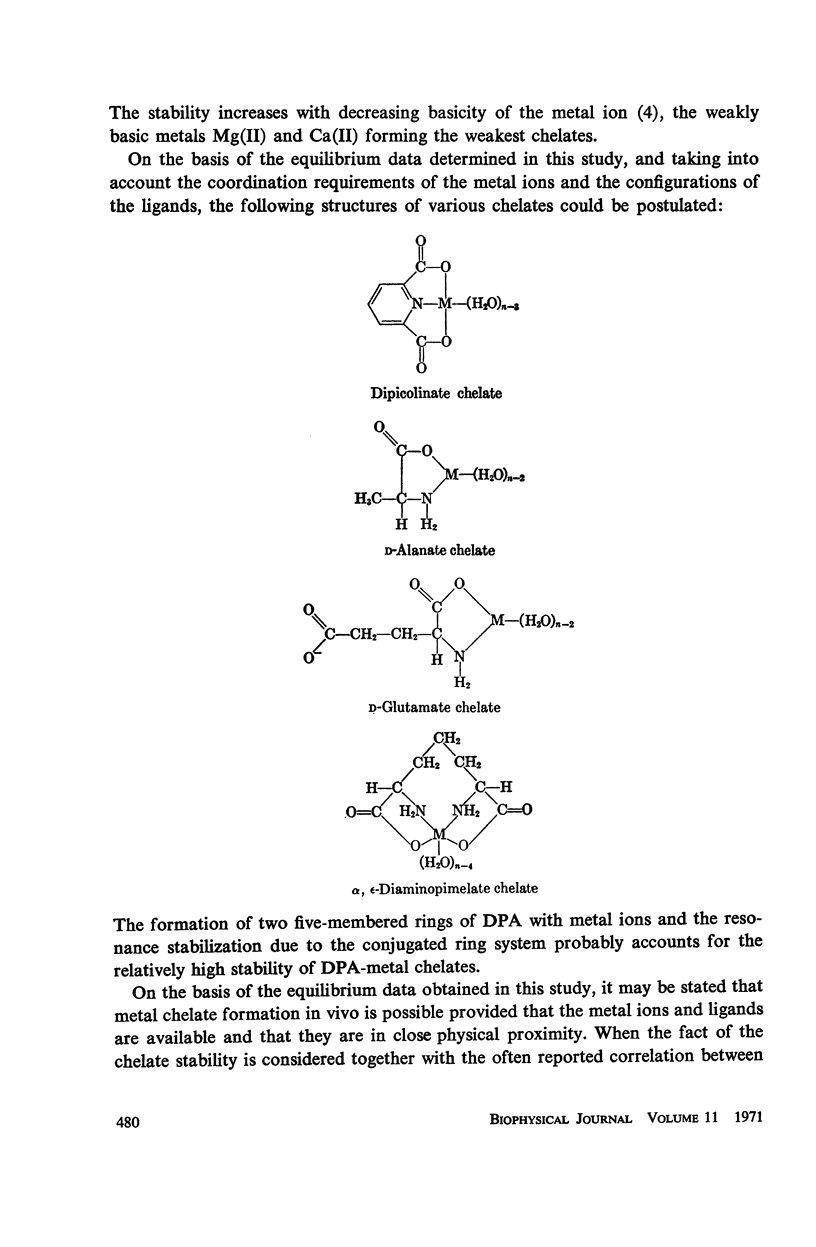
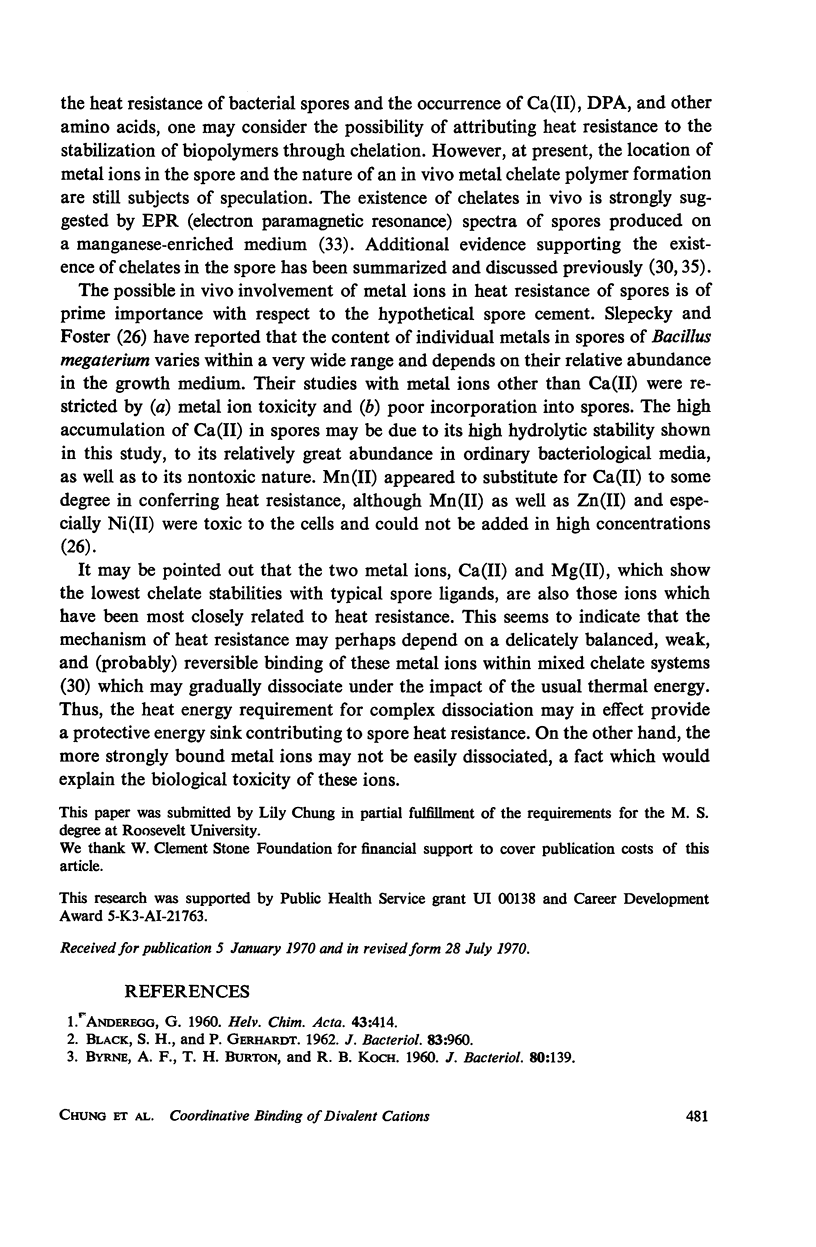
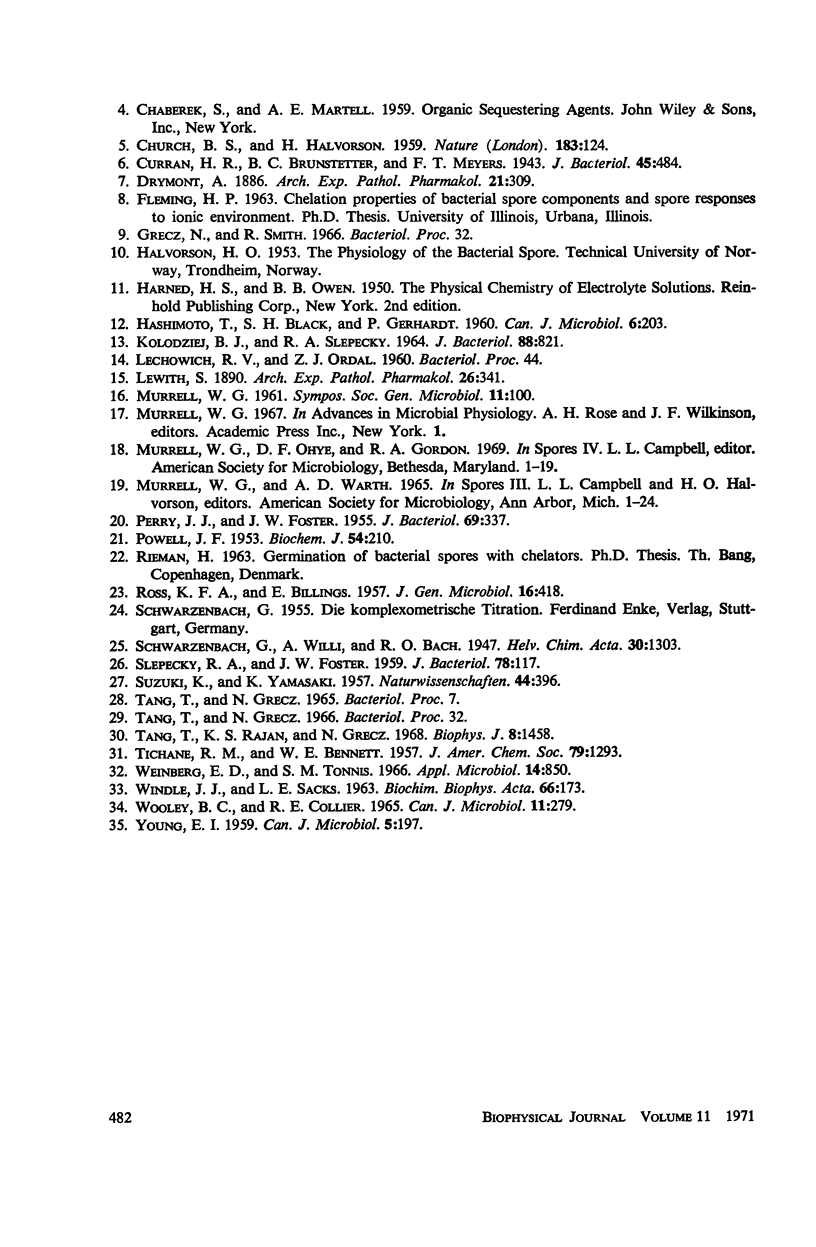
Selected References
These references are in PubMed. This may not be the complete list of references from this article.
- BLACK S. H., GERHARDT P. Permeability of bacterial spores. IV. Water content, uptake, and distribution. J Bacteriol. 1962 May;83:960–967. doi: 10.1128/jb.83.5.960-967.1962. [DOI] [PMC free article] [PubMed] [Google Scholar]
- BYRNE A. F., BURTON T. H., KOCH R. B. Relation of dipicolinic acid content of anaerobic bacterial endospores to their heat resistance. J Bacteriol. 1960 Jul;80:139–140. doi: 10.1128/jb.80.1.139-140.1960. [DOI] [PMC free article] [PubMed] [Google Scholar]
- CHURCH B. D., HALVORSON H. Dependence of the heat resistance of bacterial endospores on their dipicolinic acid content. Nature. 1959 Jan 10;183(4654):124–125. doi: 10.1038/183124a0. [DOI] [PubMed] [Google Scholar]
- HASHIMOTO T., BLACK S. H., GERHARDT P. Development of fine structure, thermostability, and dipicolinate during sporogenesis in a bacillus. Can J Microbiol. 1960 Apr;6:203–212. doi: 10.1139/m60-022. [DOI] [PubMed] [Google Scholar]
- KOLODZIEJ B. J., SLEPECKY R. A. TRACE METAL REQUIREMENTS FOR SPORULATION OF BACILLUS MEGATERIUM. J Bacteriol. 1964 Oct;88:821–830. doi: 10.1128/jb.88.4.821-830.1964. [DOI] [PMC free article] [PubMed] [Google Scholar]
- PERRY J. J., FOSTER J. W. Studies on the biosynthesis of dipicolinic acid in spores of Bacillus cereus var. mycoides. J Bacteriol. 1955 Mar;69(3):337–346. doi: 10.1128/jb.69.3.337-346.1955. [DOI] [PMC free article] [PubMed] [Google Scholar]
- POWELL J. F. Isolation of dipicolinic acid (pyridine-2:6-dicarboxylic acid) from spores of Bacillus megatherium. Biochem J. 1953 May;54(2):210–211. doi: 10.1042/bj0540210. [DOI] [PMC free article] [PubMed] [Google Scholar]
- ROSS K. F., BILLING E. The water and solid content of living bacterial spores and vegetative cells as indicated by refractive index measurements. J Gen Microbiol. 1957 Apr;16(2):418–425. doi: 10.1099/00221287-16-2-418. [DOI] [PubMed] [Google Scholar]
- SLEPECKY R., FOSTER J. W. Alterations in metal content of spores of Bacillus megaterium and the effect on some spore properties. J Bacteriol. 1959 Jul;78(1):117–123. doi: 10.1128/jb.78.1.117-123.1959. [DOI] [PMC free article] [PubMed] [Google Scholar]
- Tang T., Rajan K. S., Grecz N. Mixed chelates of Ca(II)-pyridine-2,6-dicarboxylate with some amino acids related to bacterial spores. Biophys J. 1968 Dec;8(12):1458–1474. doi: 10.1016/S0006-3495(68)86566-X. [DOI] [PMC free article] [PubMed] [Google Scholar]
- WINDLE J. J., SACKS L. E. Electron paramagnetic resonance of managanese(II) and copper(II) in spores. Biochim Biophys Acta. 1963 Mar 19;66:173–179. doi: 10.1016/0006-3002(63)91183-1. [DOI] [PubMed] [Google Scholar]
- WOOLEY B. C., COLLIER R. E. CHANGES IN THERMORESISTANCE OF CLOSTRIDIUM ROSEUM AS RELATED TO THE INTRACELLULAR CONTENT OF CALCIUM AND DIPICOLINIC ACID. Can J Microbiol. 1965 Apr;11:279–285. doi: 10.1139/m65-034. [DOI] [PubMed] [Google Scholar]
- Weinberg E. D., Tonnis S. M. Action of chloramphenicol and its isomers on secondary biosynthetic processes of bacillus. Appl Microbiol. 1966 Nov;14(6):850–856. doi: 10.1128/am.14.6.850-856.1966. [DOI] [PMC free article] [PubMed] [Google Scholar]
- YOUNG I. E. A relationship between the free amino acid pool, dipicolinic acid, calcium from resting spores of Bacillus megaterium. Can J Microbiol. 1959 Apr;5(2):197–202. doi: 10.1139/m59-024. [DOI] [PubMed] [Google Scholar]