Abstract
The shapes of the photocurrent responses of rat rods, recorded with microelectrodes from the receptor layer of small pieces of isolated retinas, have been investigated as a function of temperature and of stimulus energy. Between 27 and 37°C the responses to short flashes can be described formally as the output of a chain of at least four linear low-pass filters with time constants in the range 50-100 msec. The output of the filter chain is then distorted by a nonlinear amplitude-limiting process with a hyperbolic saturation characteristic. Flashes producing ∼30 photons absorbed per rod yield responses of half-maximal size independently of temperature. The maximum response amplitude is that just sufficient to cancel the dark current. The rate of rise of a response is proportional to flash energy up to the level of 105 photons absorbed per rod, where hyperbolic rate saturation ensues. The responses continue to increase in duration with even more intense flashes until, at the level of 107 photons absorbed per rod, they last longer than 50 min. The time-courses of the photocurrent and of the excitatory disturbance in the rod system are very similar. The stimulus intensity at which amplitude saturation of the photocurrent responses begins is near that where psychophysical “rod saturation” is seen. An analysis of these properties leads to the following conclusions about the mechanism of rod excitation. (a) The kinetics of the photocurrent bear no simple relation to the formation or decay of any of the spectroscopic intermediates so far detected during the photolysis of rhodopsin. (b) The forms of both the amplitude- and rate-limiting processes are not compatible with organization of rhodopsin into “photoreceptive units” containing more than 300 chromophores. Even at high stimulus intensities most rhodopsin chromophores remain connected to the excitatory apparatus of rods. (c) The maximum rate of rise of the photocurrent is too fast to be consistent with the infolded disks of a rod outer segment being attached to the overlying plasma membrane. Most of the disks behave electrically as if isolated within the cell. (d) Control of the photocurrent at the outer segment membrane is not achieved by segregation of the charge carriers of the current within the rod disks. Instead, it is likely to depend on control of the plasma membrane permeability by an agent released from the disks.
Full text
PDF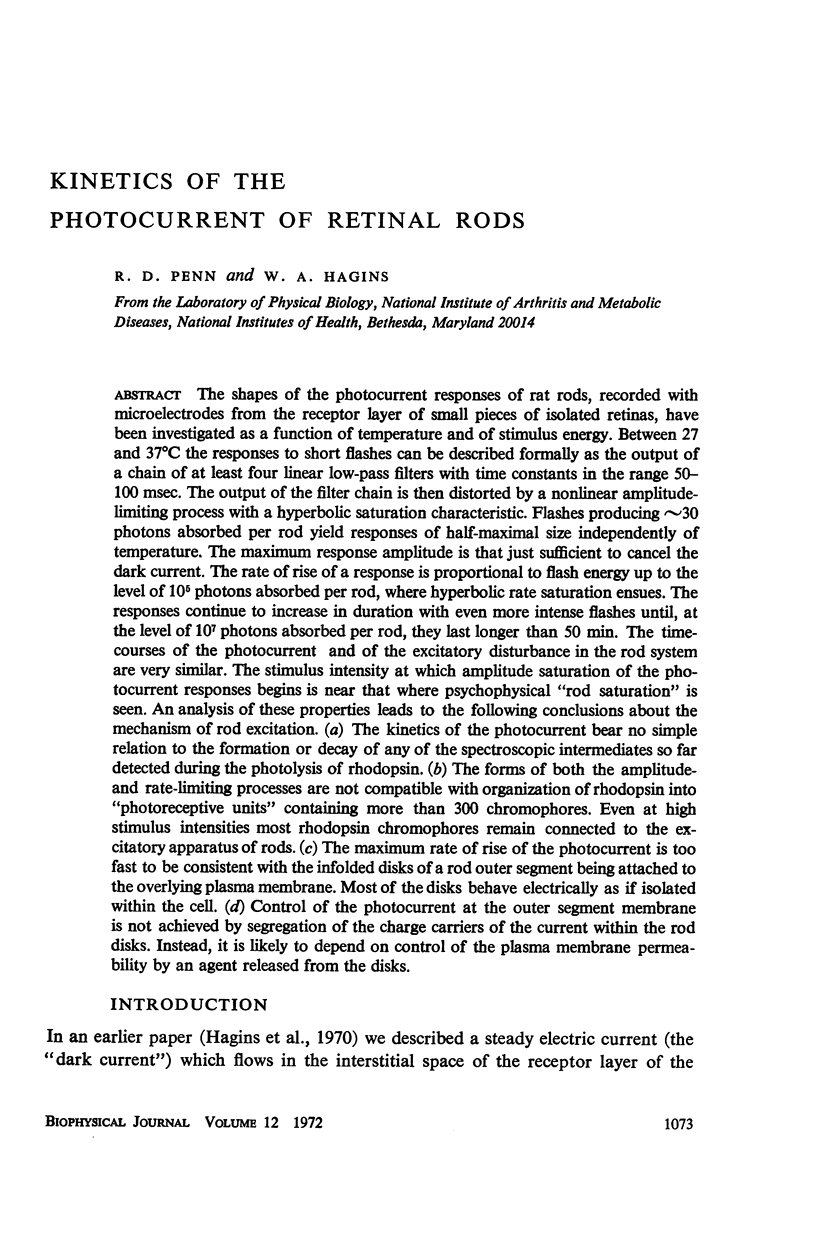
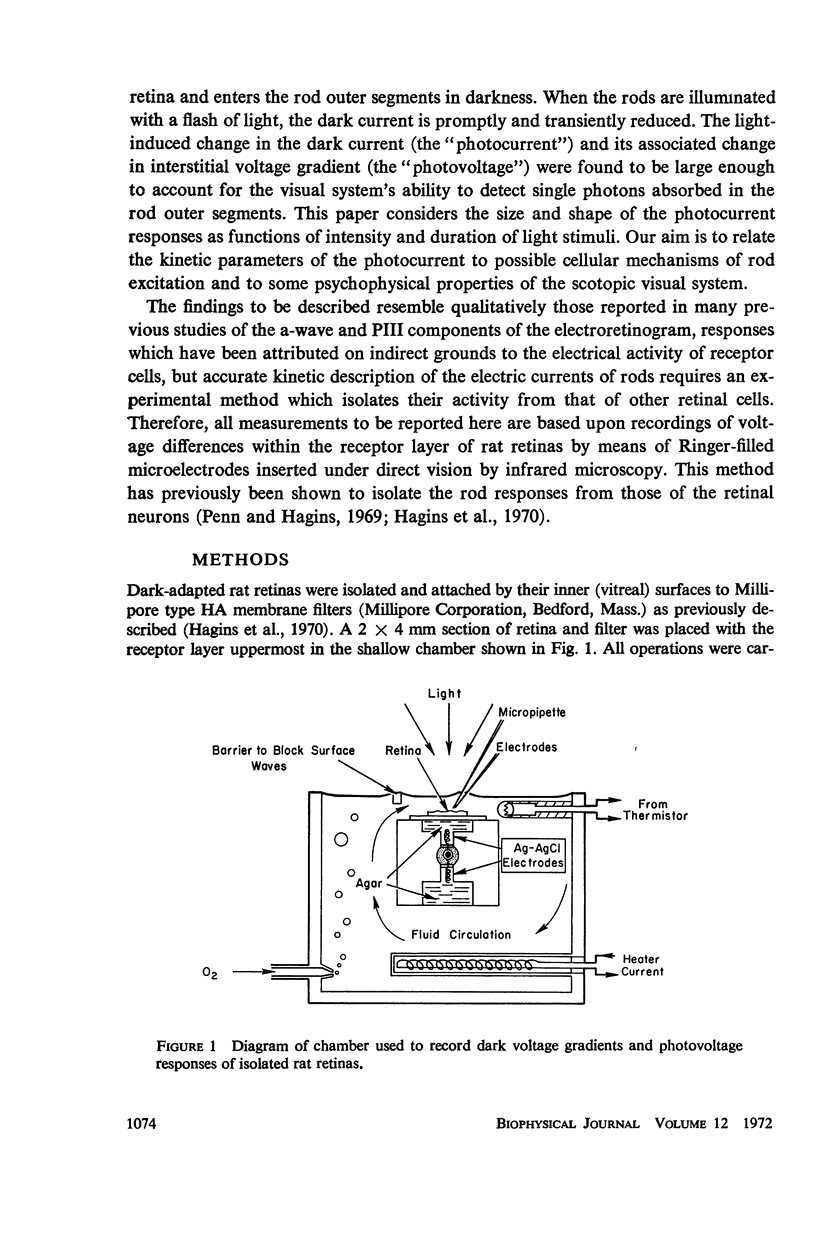
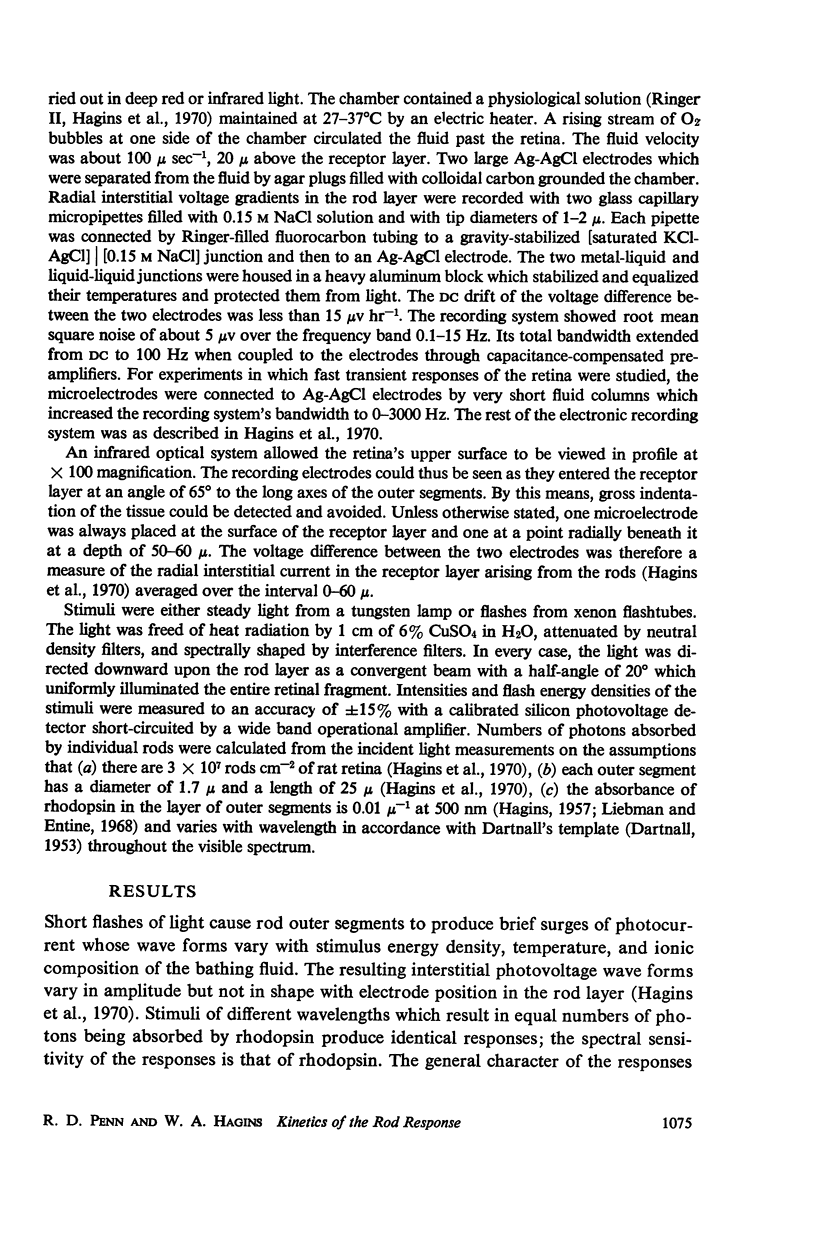
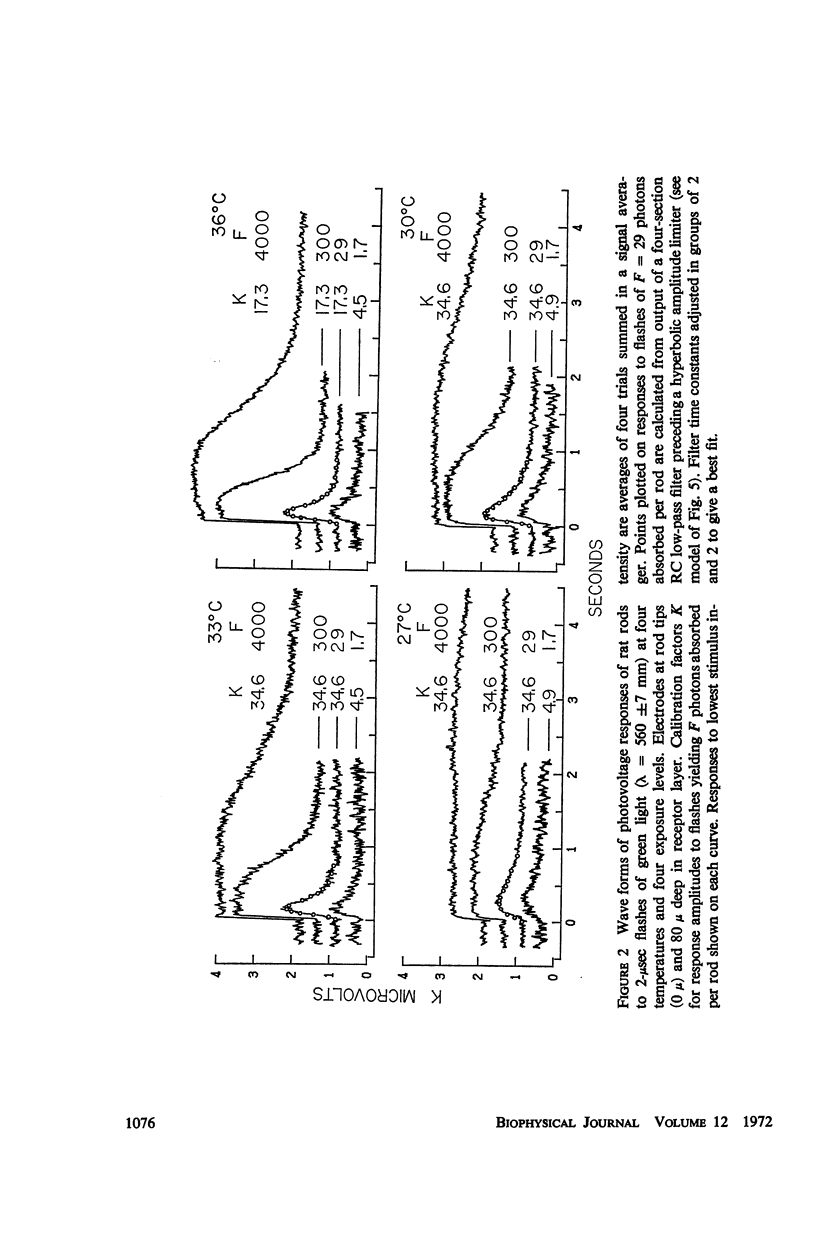
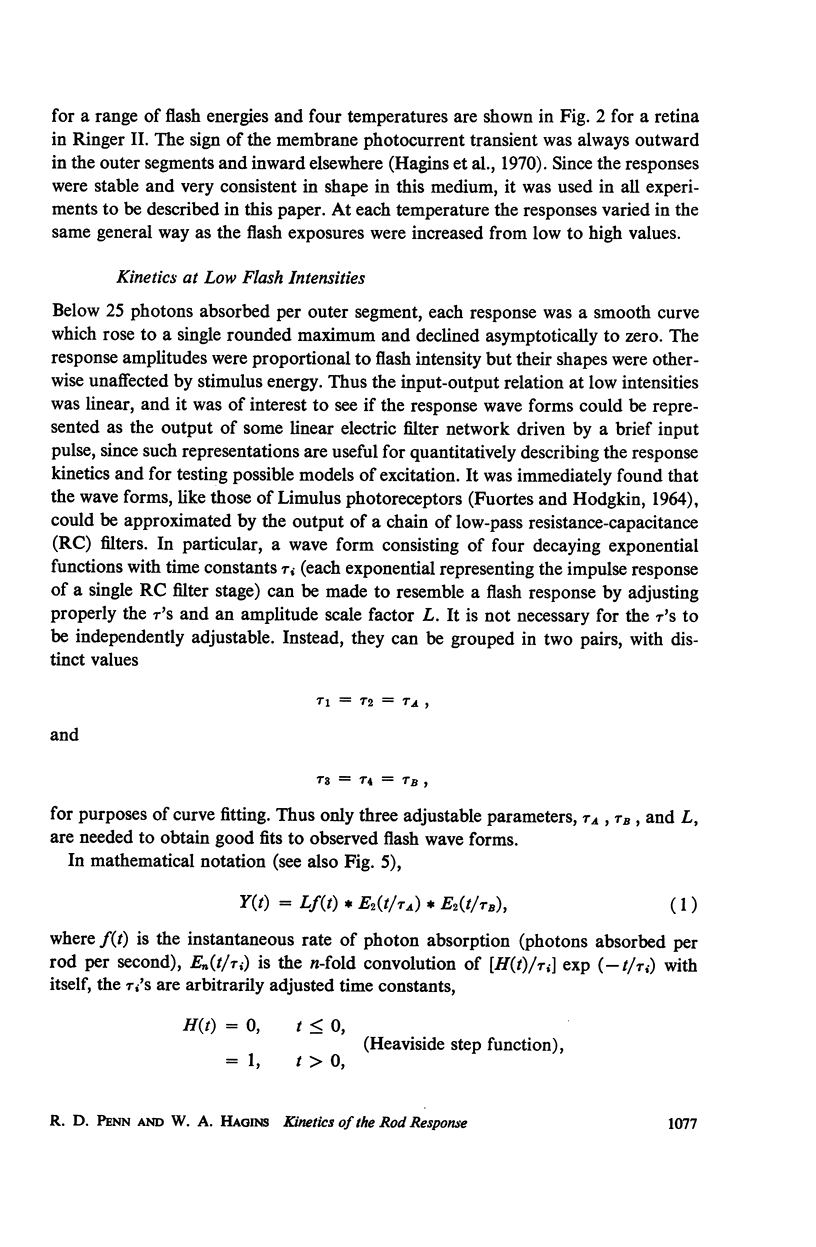
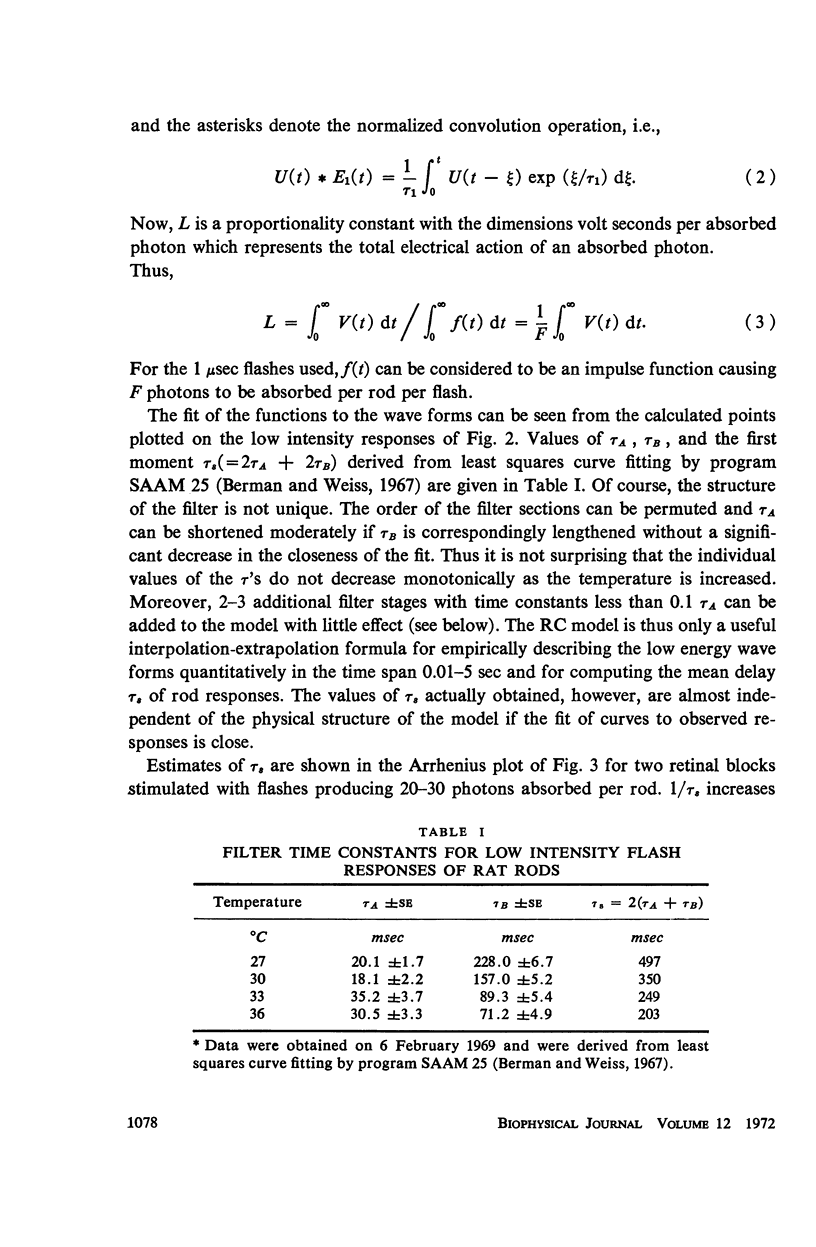
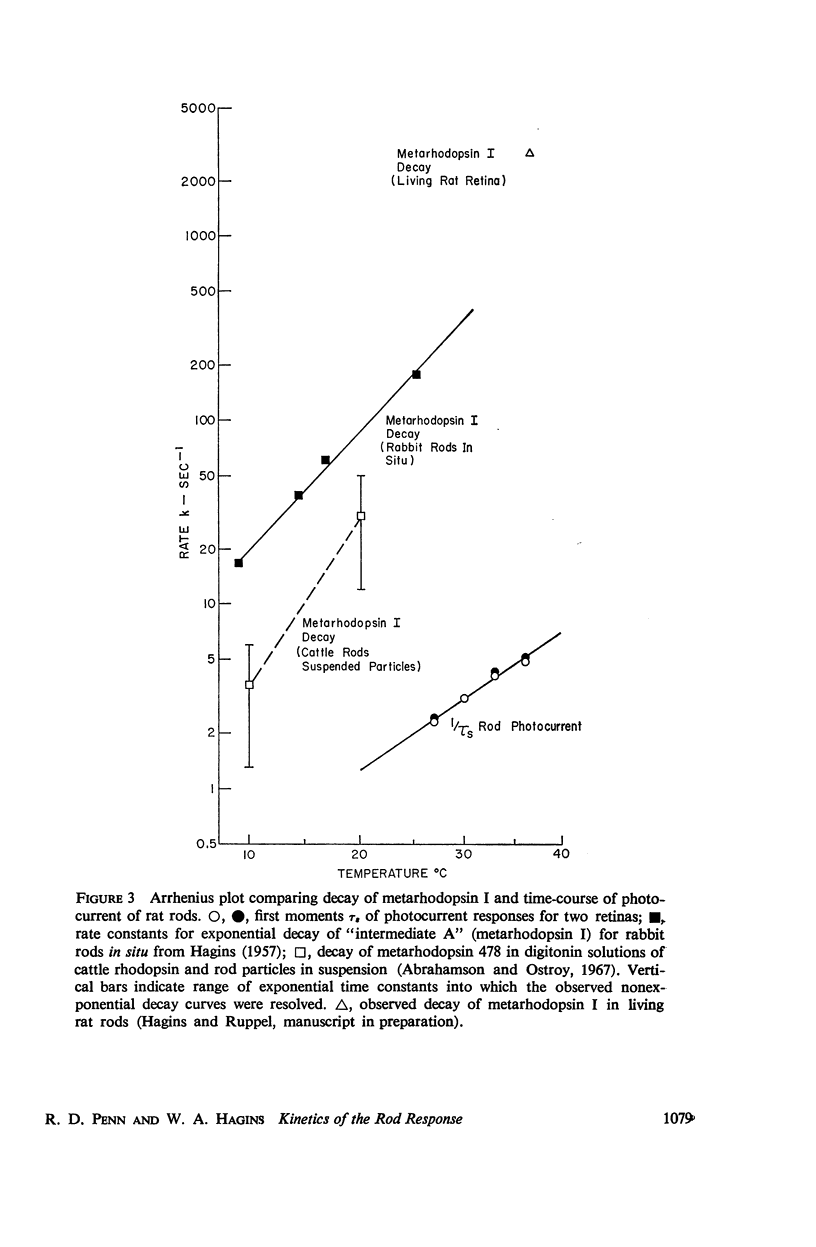
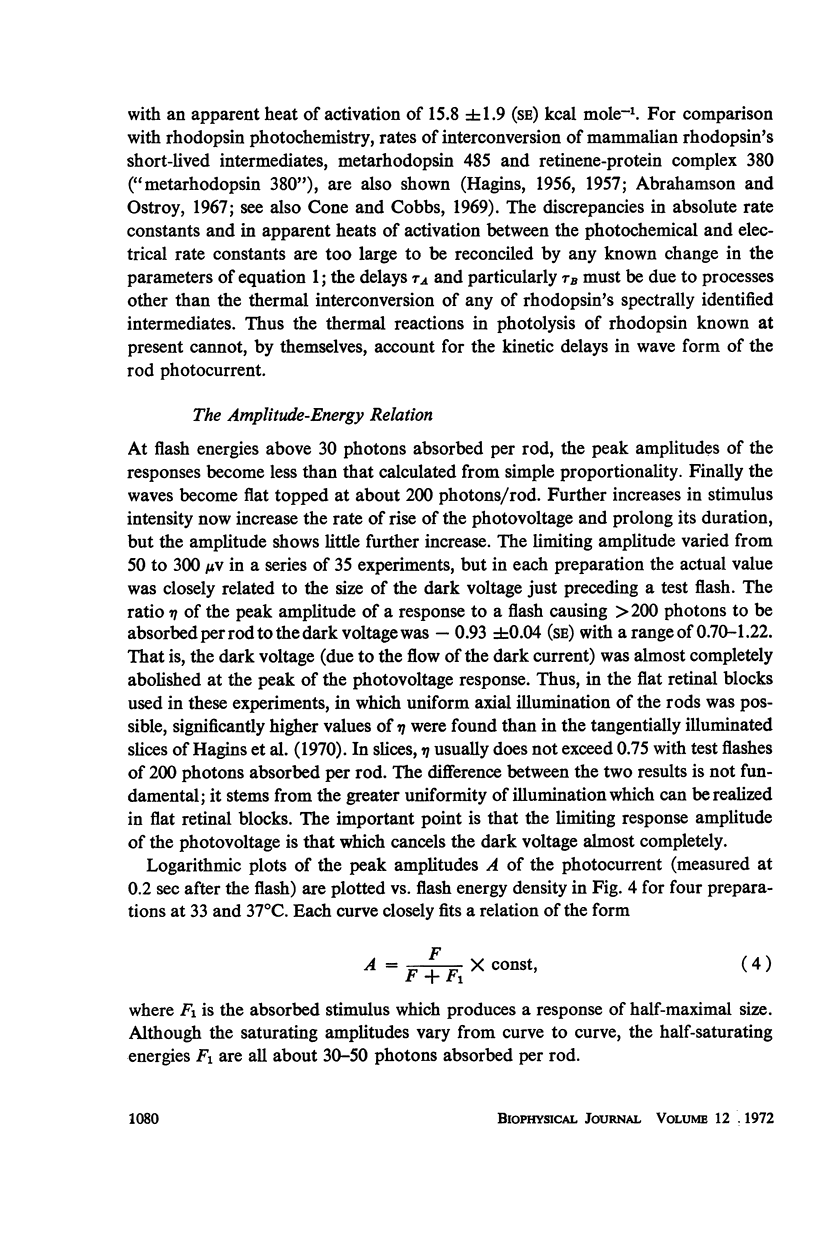
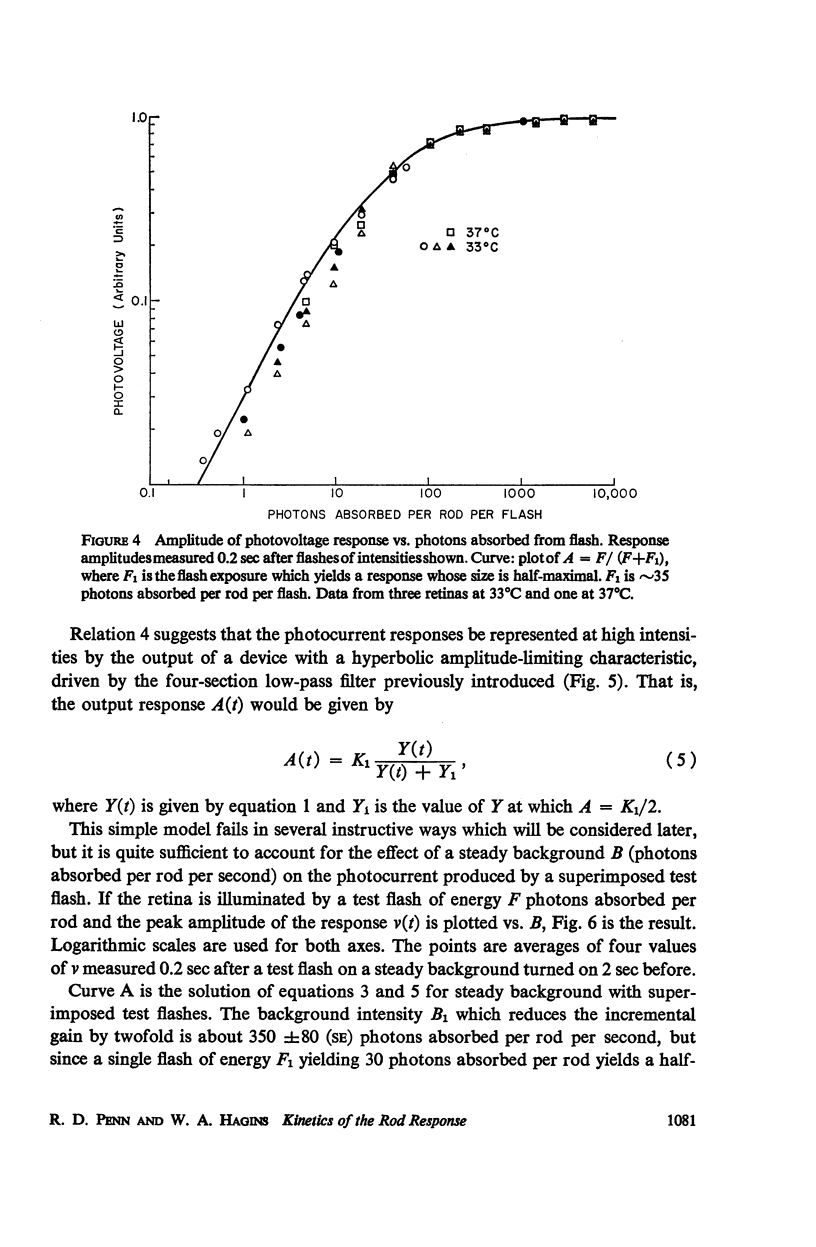
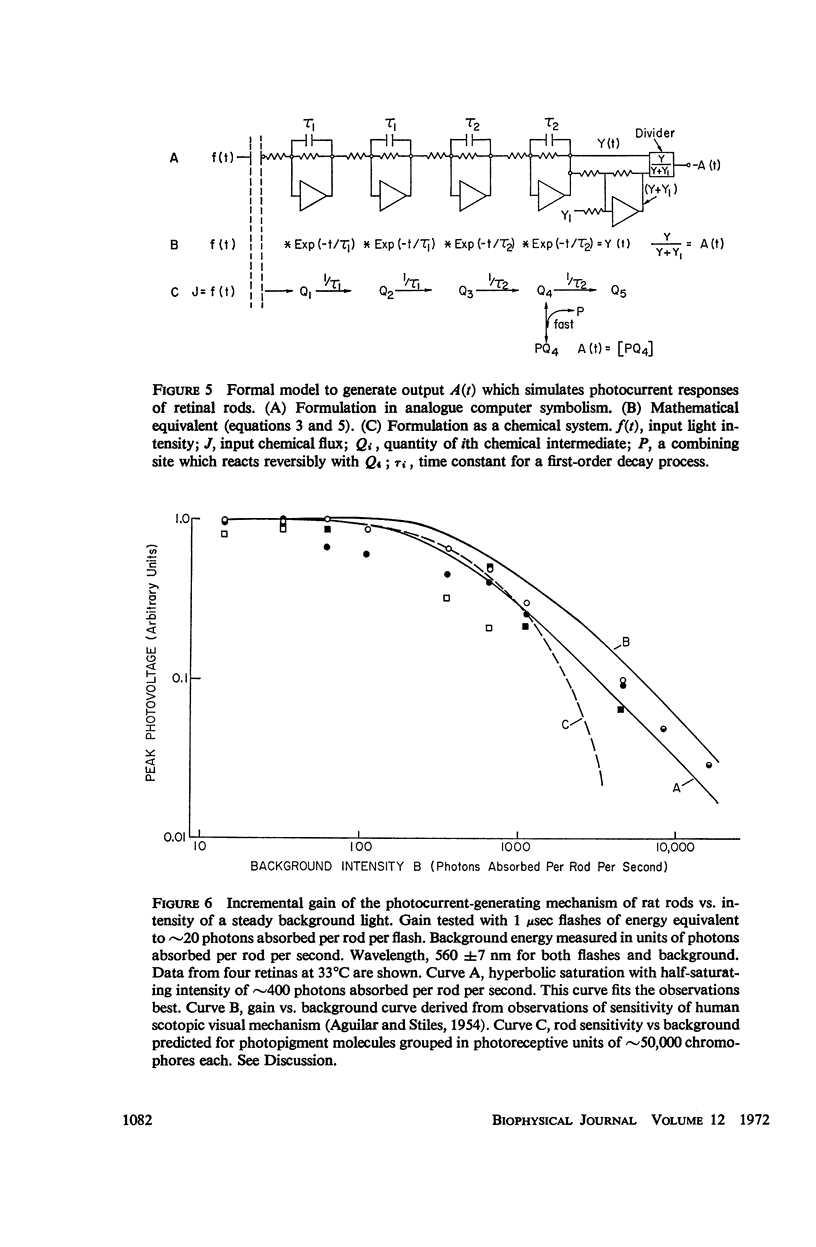
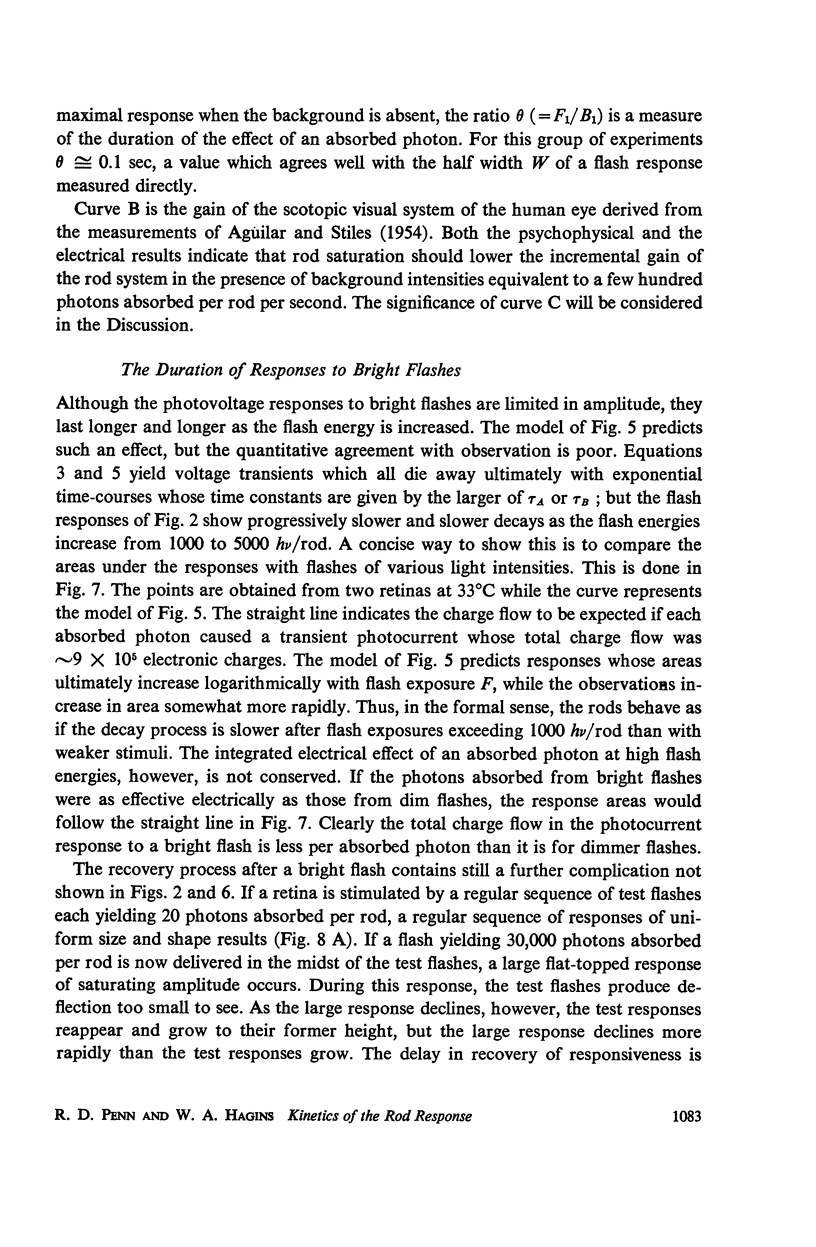
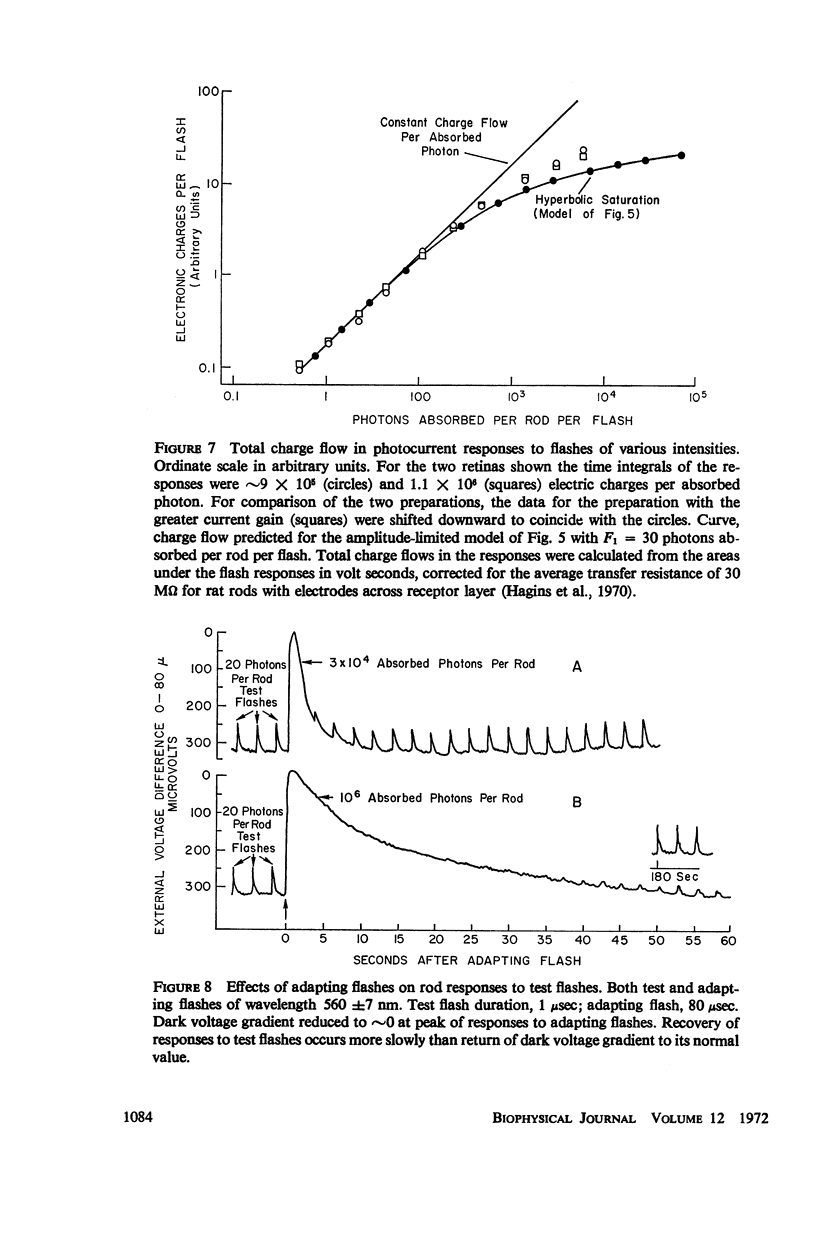
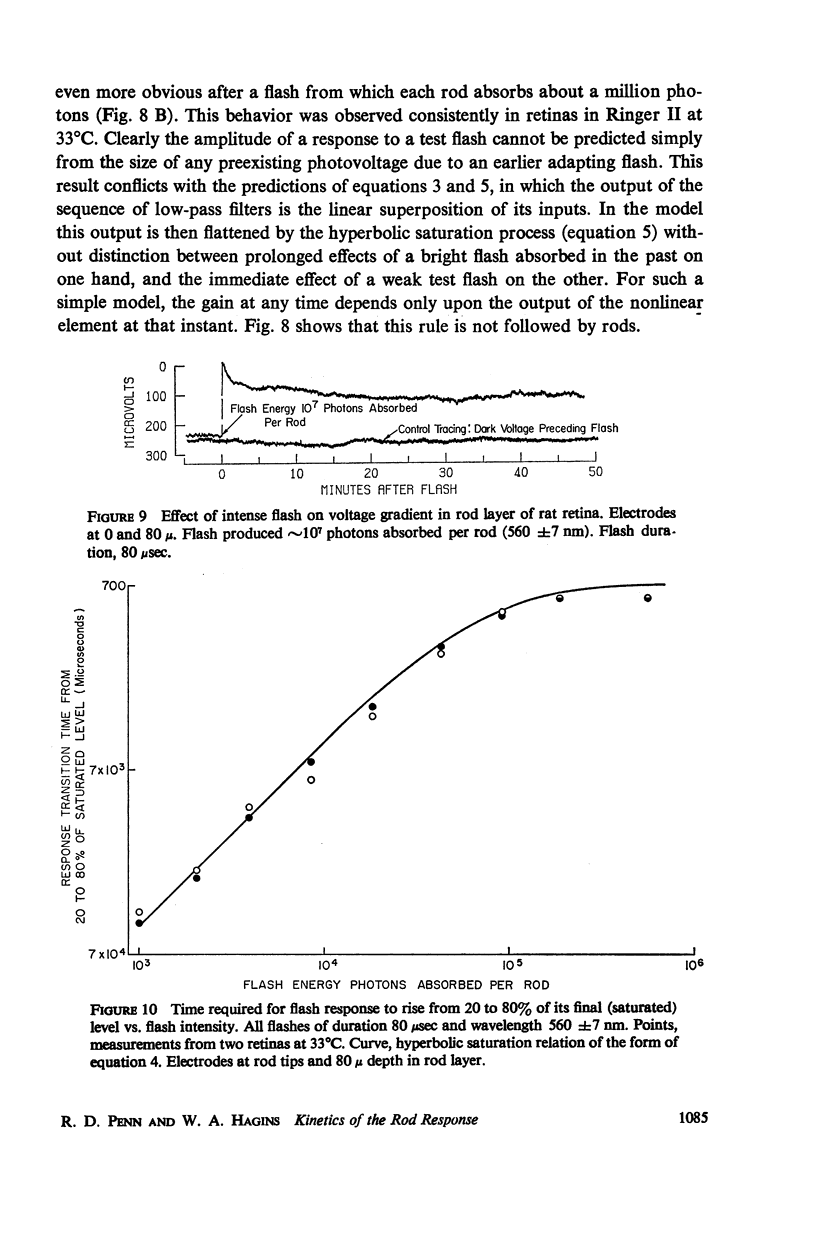
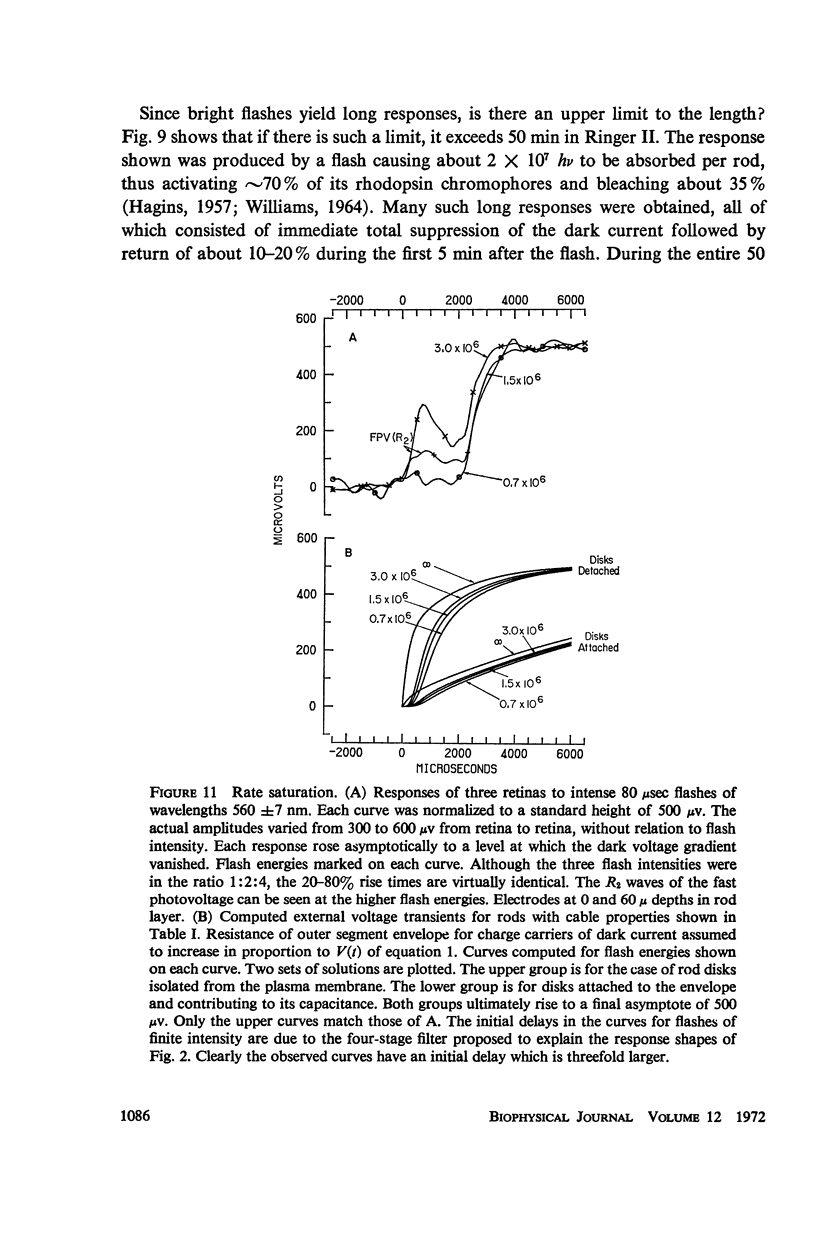
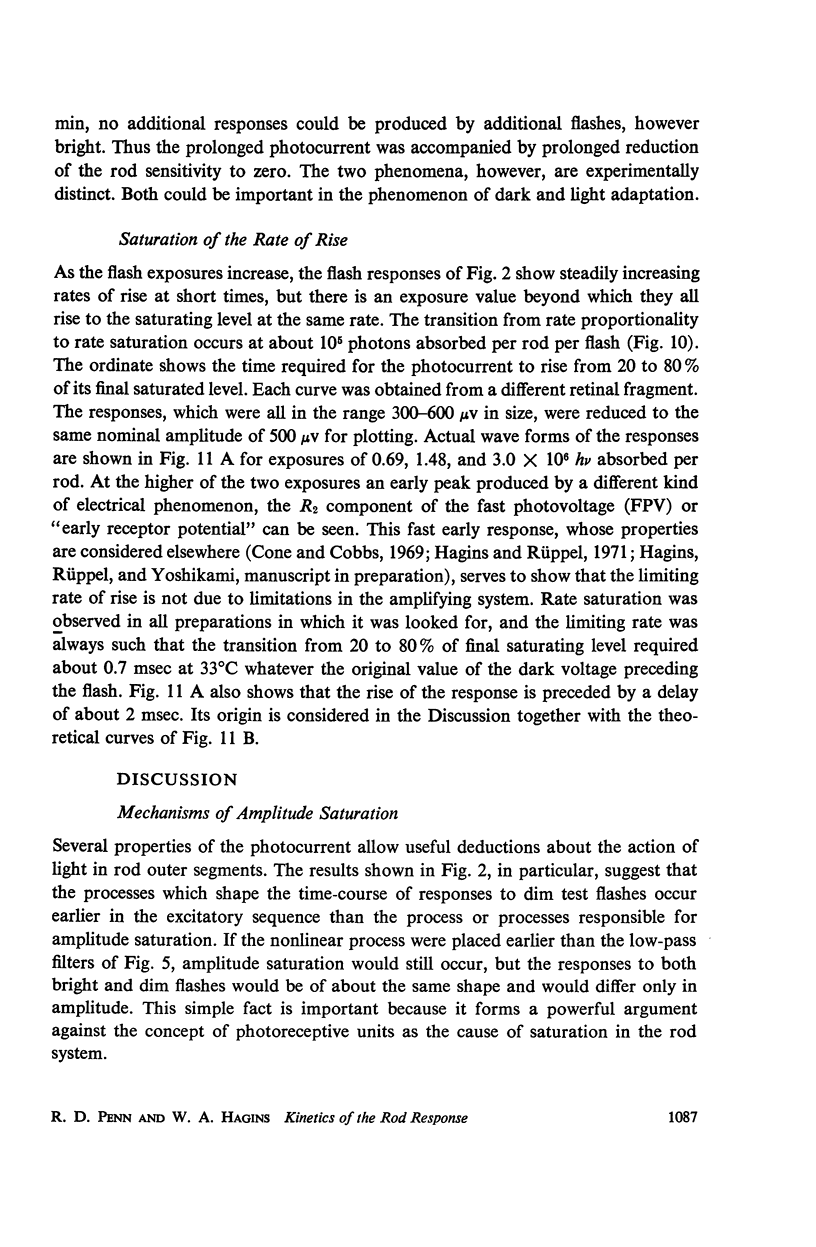
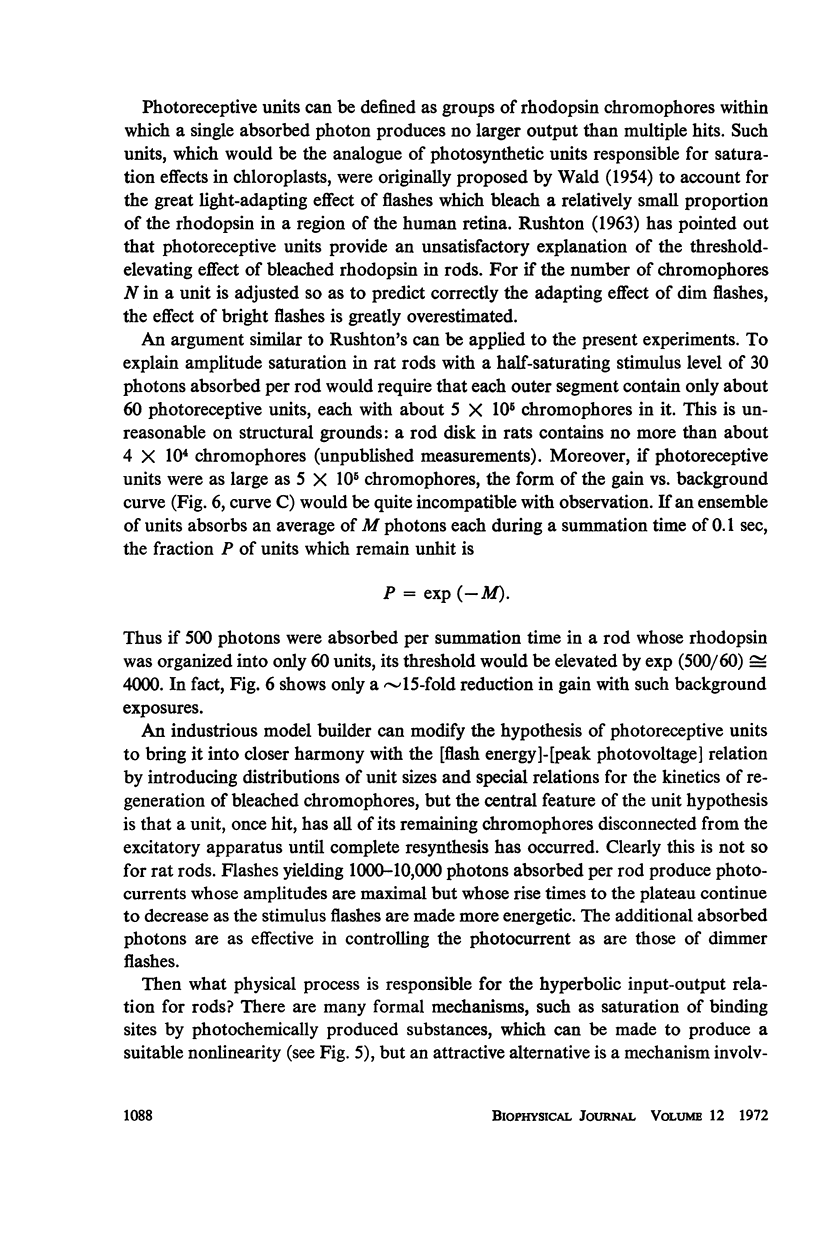
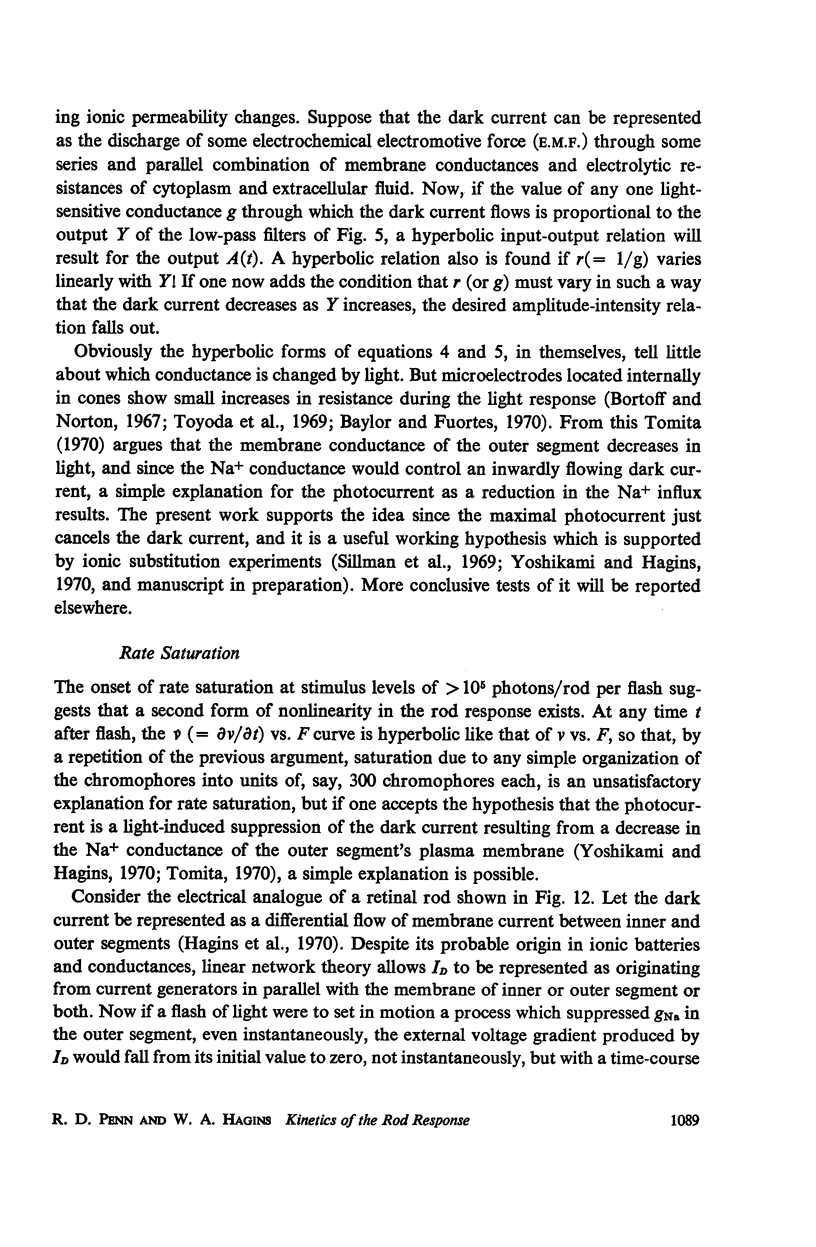
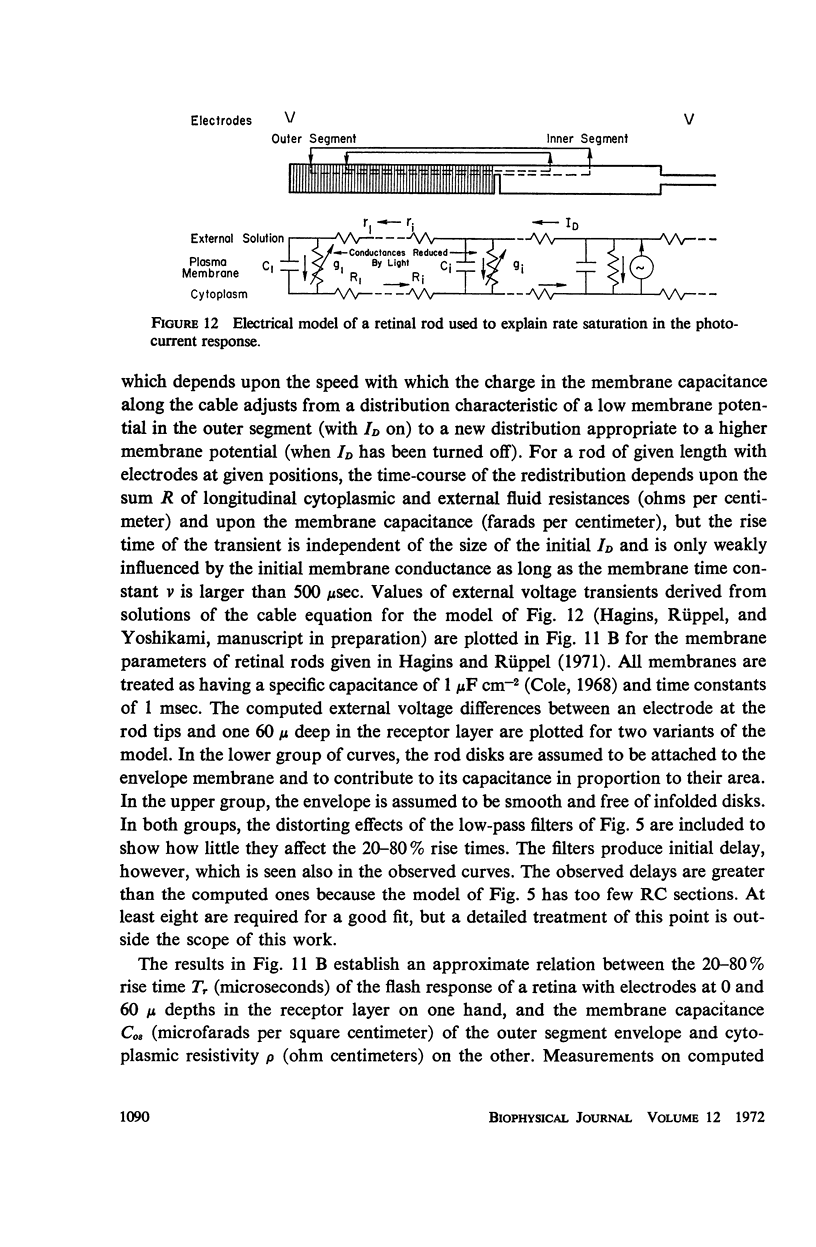
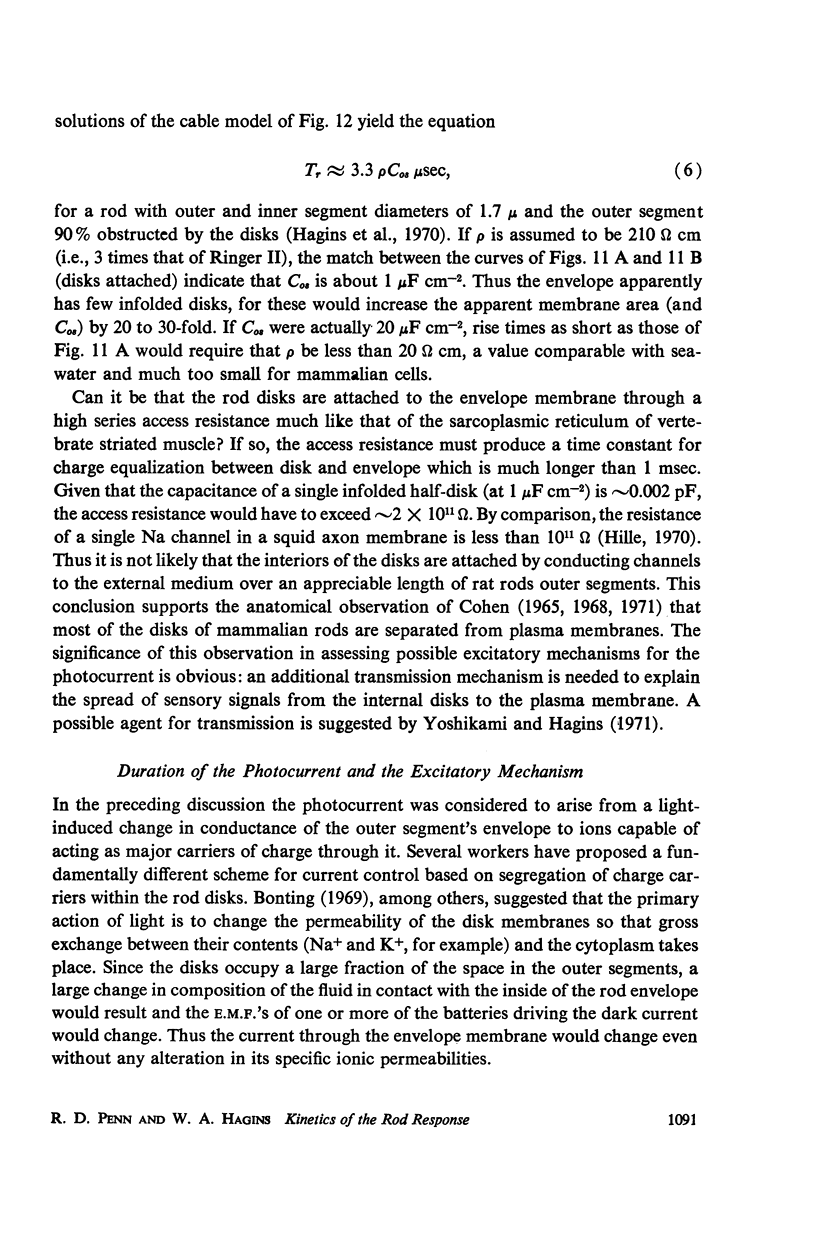
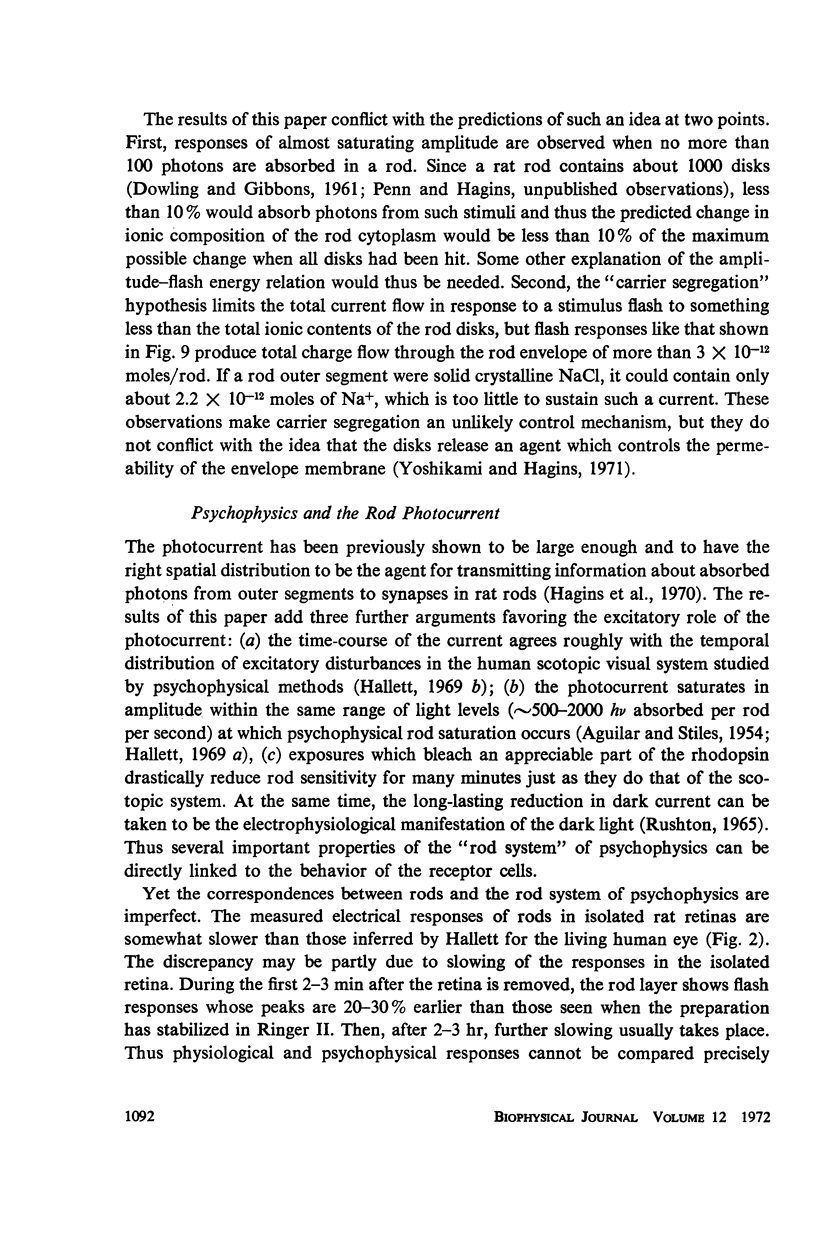
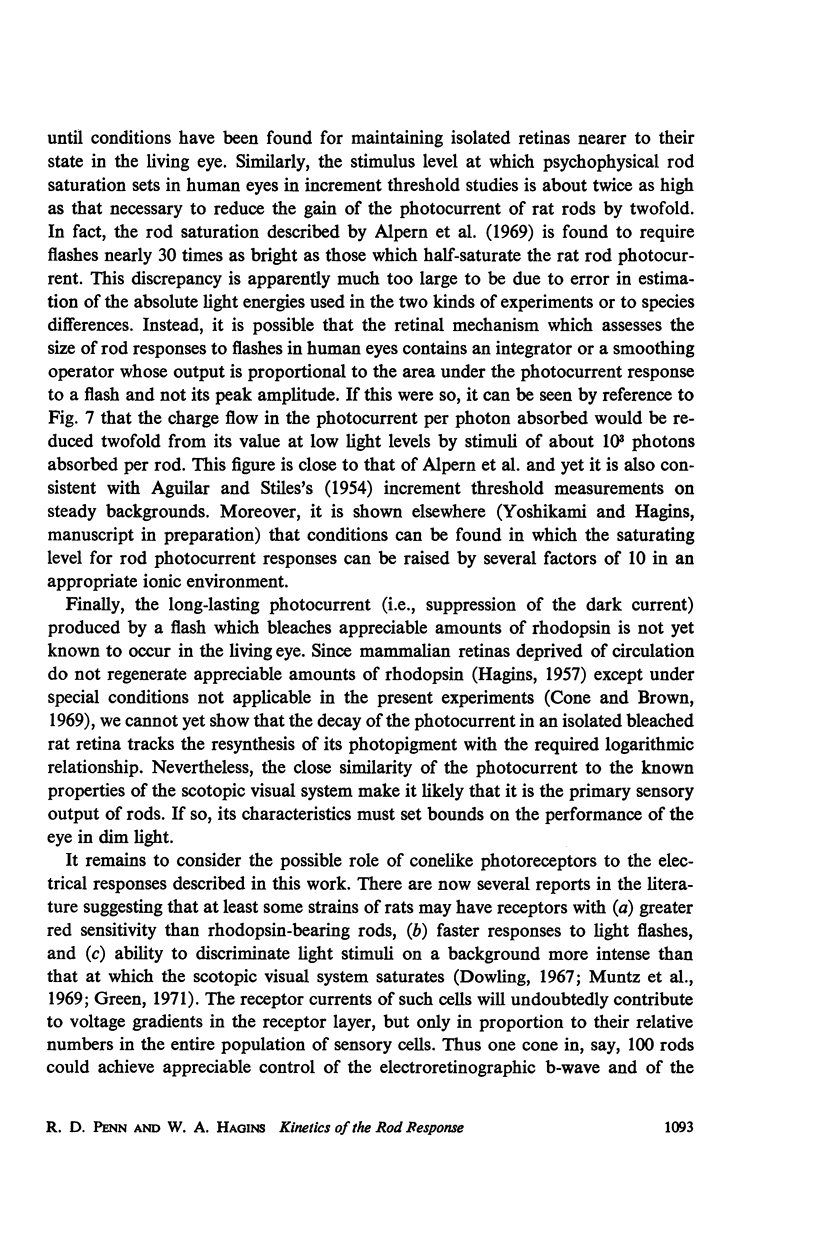
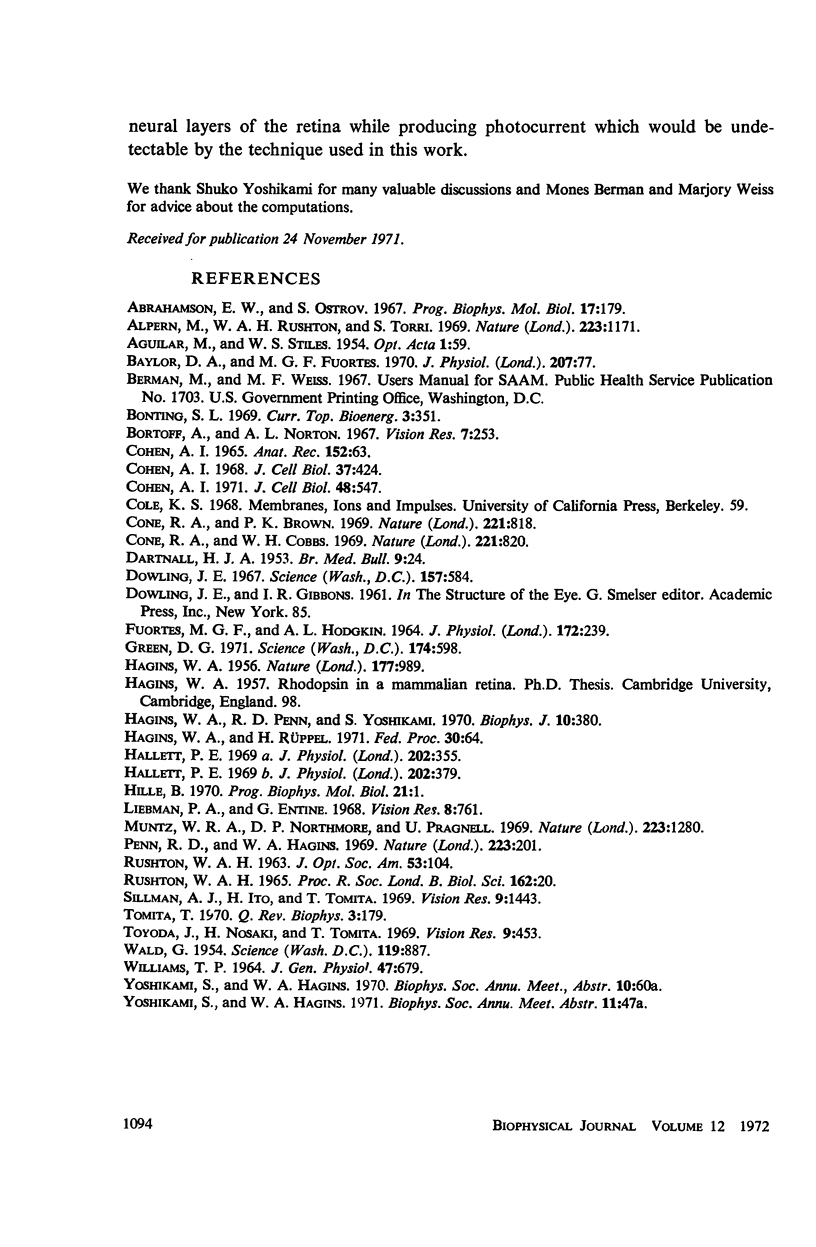
Selected References
These references are in PubMed. This may not be the complete list of references from this article.
- Abrahamson E. W., Ostroy S. E. The photochemical and macromolecular aspects of vision. Prog Biophys Mol Biol. 1967;17:179–215. doi: 10.1016/0079-6107(67)90007-7. [DOI] [PubMed] [Google Scholar]
- Alpern M., Rushton W. A., Torri S. Encoding of nerve signals from retinal rods. Nature. 1969 Sep 13;223(5211):1171–1172. doi: 10.1038/2231171a0. [DOI] [PubMed] [Google Scholar]
- Bortoff A., Norton A. L. An electrical model of the vertebrate photoreceptor cell. Vision Res. 1967 Mar;7(3):253–263. doi: 10.1016/0042-6989(67)90089-2. [DOI] [PubMed] [Google Scholar]
- COHEN A. I. NEW DETAILS OF THE ULTRASTRUCTURE OF THE OUTER SEGMENTS AND CILIARY CONNECTIVES OF THE RODS OF HUMAN AND MACAQUE RETINAS. Anat Rec. 1965 May;152:63–79. doi: 10.1002/ar.1091520108. [DOI] [PubMed] [Google Scholar]
- Cone R. A., Brown P. K. Spontaneous regeneration of rhodopsin in the isolated rat retina. Nature. 1969 Mar 1;221(5183):818–820. doi: 10.1038/221818a0. [DOI] [PubMed] [Google Scholar]
- Cone R. A., Cobbs W. H., 3rd Rhodopsin cycle in the living eye of the rat. Nature. 1969 Mar 1;221(5183):820–822. doi: 10.1038/221820a0. [DOI] [PubMed] [Google Scholar]
- DARTNALL H. J. A. The interpretation of spectral sensitivity curves. Br Med Bull. 1953;9(1):24–30. doi: 10.1093/oxfordjournals.bmb.a074302. [DOI] [PubMed] [Google Scholar]
- Dowling J. E. Visual adaptation: its mechanism. Science. 1967 Aug 4;157(3788):584–585. [PubMed] [Google Scholar]
- FUORTES M. G., HODGKIN A. L. CHANGES IN TIME SCALE AND SENSITIVITY IN THE OMMATIDIA OF LIMULUS. J Physiol. 1964 Aug;172:239–263. doi: 10.1113/jphysiol.1964.sp007415. [DOI] [PMC free article] [PubMed] [Google Scholar]
- Green D. G. Light adaptation in the rat retina: evidence for two receptor mechanisms. Science. 1971 Nov 5;174(4009):598–600. doi: 10.1126/science.174.4009.598. [DOI] [PubMed] [Google Scholar]
- HAGINS W. A. Flash photolysis of rhodopsin in the retina. Nature. 1956 May 26;177(4517):989–990. doi: 10.1038/177989b0. [DOI] [PubMed] [Google Scholar]
- Hagins W. A., Penn R. D., Yoshikami S. Dark current and photocurrent in retinal rods. Biophys J. 1970 May;10(5):380–412. doi: 10.1016/S0006-3495(70)86308-1. [DOI] [PMC free article] [PubMed] [Google Scholar]
- Hagins W. A., Rüppel H. Fast photoelectric effects and the properties of vertebrate photoreceptors as electric cables. Fed Proc. 1971 Jan-Feb;30(1):64–68. [PubMed] [Google Scholar]
- Hallett P. E. Impulse functions for human rod vision. J Physiol. 1969 Jun;202(2):379–402. doi: 10.1113/jphysiol.1969.sp008817. [DOI] [PMC free article] [PubMed] [Google Scholar]
- Liebman P. A., Entine G. Visual pigments of frog and tadpole (Rana pipiens). Vision Res. 1968 Jul;8(7):761–775. doi: 10.1016/0042-6989(68)90128-4. [DOI] [PubMed] [Google Scholar]
- Muntz W. R., Northmore D. P., Pragnell V. Increment thresholds in photopic conditions in the hooded rat. Nature. 1969 Sep 20;223(5212):1280–1281. doi: 10.1038/2231280a0. [DOI] [PubMed] [Google Scholar]
- Penn R. D., Hagins W. A. Signal transmission along retinal rods and the origin of the electroretinographic a-wave. Nature. 1969 Jul 12;223(5202):201–204. doi: 10.1038/223201a0. [DOI] [PubMed] [Google Scholar]
- RUSHTON W. A. Increment threshold and dark adaptation. J Opt Soc Am. 1963 Jan;53:104–109. doi: 10.1364/josa.53.000104. [DOI] [PubMed] [Google Scholar]
- RUSHTON W. A. VISUAL ADAPTATION. Proc R Soc Lond B Biol Sci. 1965 Mar 16;162:20–46. doi: 10.1098/rspb.1965.0024. [DOI] [PubMed] [Google Scholar]
- Sillman A. J., Ito H., Tomita T. Studies on the mass receptor potential of the isolated frog retina. II. On the basis of the ionic mechanism. Vision Res. 1969 Dec;9(12):1443–1451. doi: 10.1016/0042-6989(69)90060-1. [DOI] [PubMed] [Google Scholar]
- Tomita T. Electrical activity of vertebrate photoreceptors. Q Rev Biophys. 1970 May;3(2):179–222. doi: 10.1017/s0033583500004571. [DOI] [PubMed] [Google Scholar]
- Toyoda J., Nosaki H., Tomita T. Light-induced resistance changes in single photoreceptors of Necturus and Gekko. Vision Res. 1969 Apr;9(4):453–463. doi: 10.1016/0042-6989(69)90134-5. [DOI] [PubMed] [Google Scholar]
- WALD G. On the mechanism of the visual threshold and visual adaptation. Science. 1954 Jun 25;119(3104):887–892. doi: 10.1126/science.119.3104.887. [DOI] [PubMed] [Google Scholar]
- WILLIAMS T. P. PHOTOREVERSAL OF RHODOPSIN BLEACHING. J Gen Physiol. 1964 Mar;47:679–689. doi: 10.1085/jgp.47.4.679. [DOI] [PMC free article] [PubMed] [Google Scholar]