Abstract
A detailed analysis is made of the model system of two parallel cylindrical polyelectrolytes which contain ionizable groups on their surfaces and are immersed in an ionic bathing medium. The interaction between the cylinders is examined by considering the interplay between repulsive electrostatic forces and attractive forces of electrodynamic origin. The repulsive force arises from the screened coulomb interaction between the surface charge distributions on the cylinders and has been treated by developing a solution to the linearized Poisson-Boltzmann equation. The boundary condition at the cylinder surfaces is determined as a self-consistent functional of the potential, with the input consisting of the density of ionizable groups and their dissociation constants. It is suggested that a reasonably accurate representation for the form of the attractive force can be obtained by performing a pairwise summation of the individual interatomic forces. A quantitative estimate is obtained using a Hamaker constant chosen on the basis of rigorous calculations on simpler systems. It is found that a balance exists between these repulsive and attractive forces at separations in good agreement with those observed in arrays of tobacco mosaic virus and in the A band myosin lattice in striated muscle. The behavior of the balance point as a function of the pH and ionic strength of the bathing medium closely parallels that seen experimentally.
Full text
PDF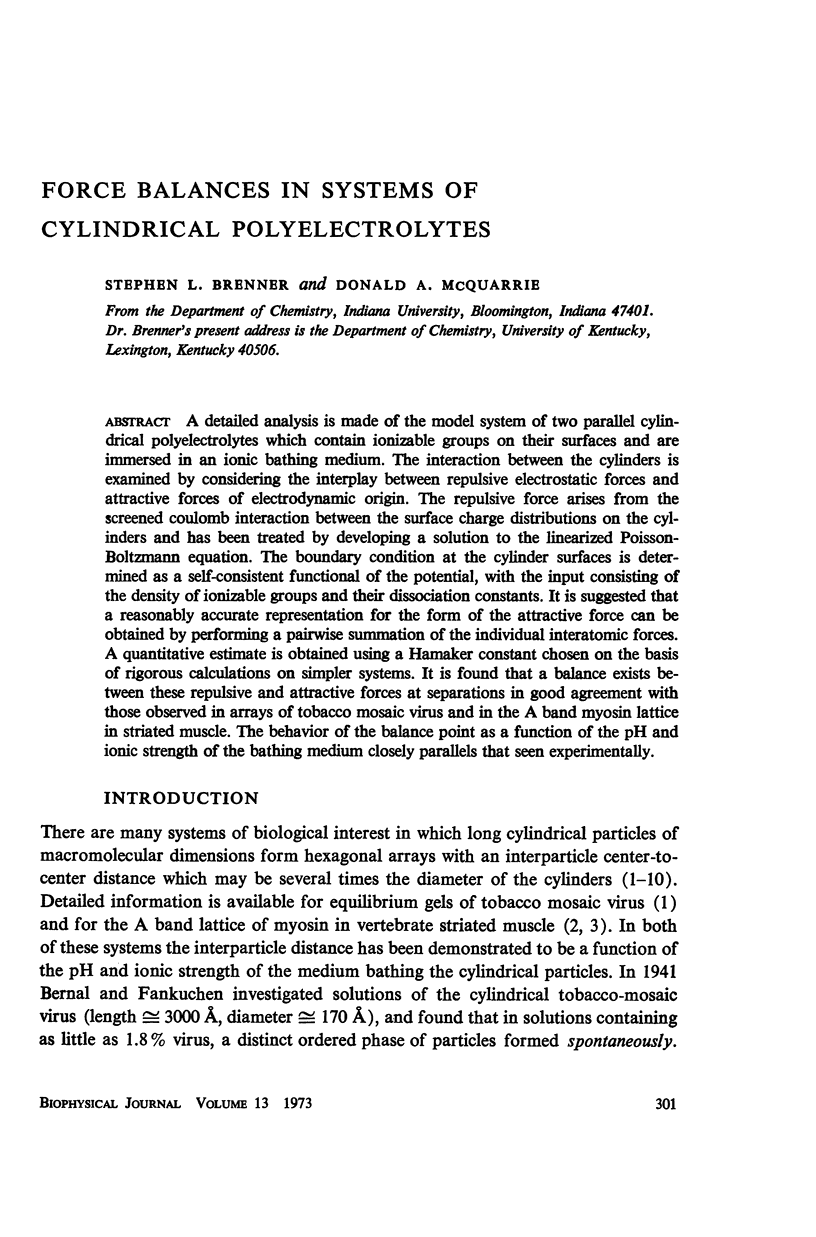
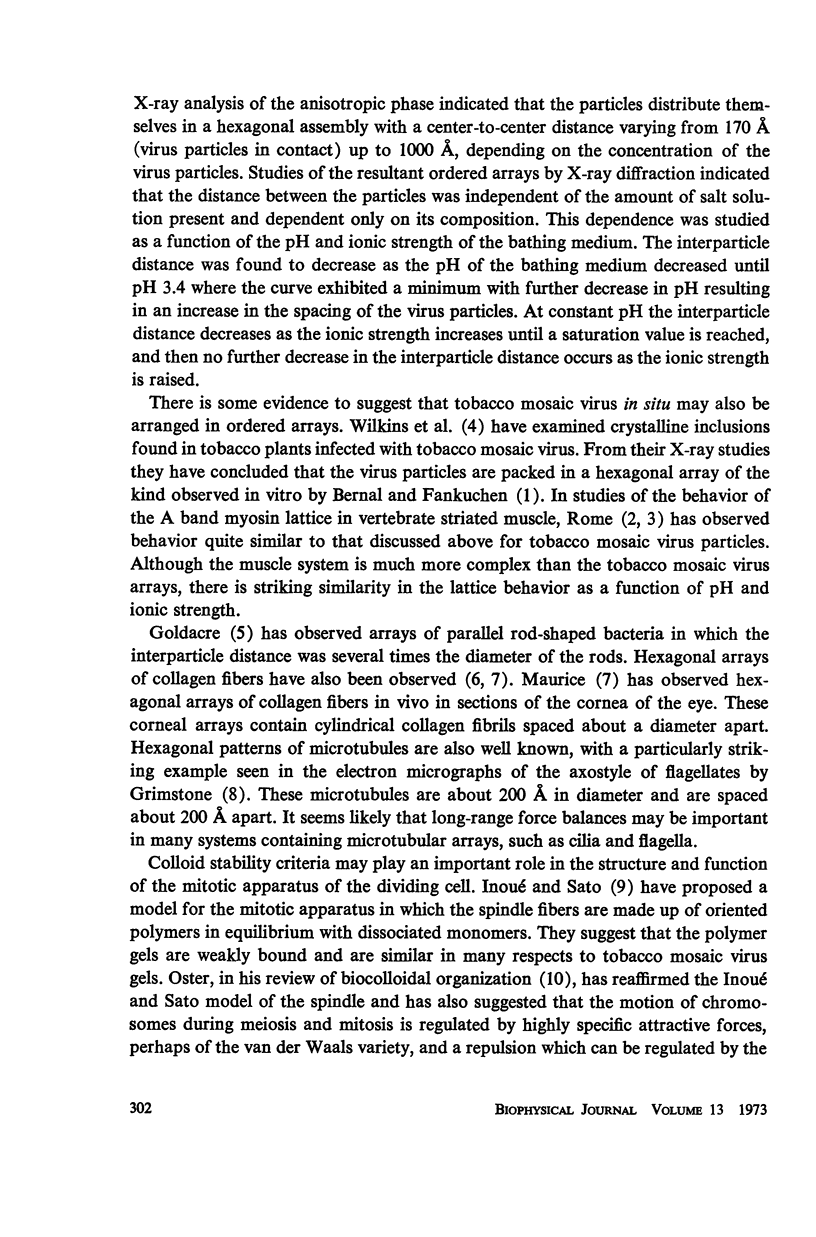
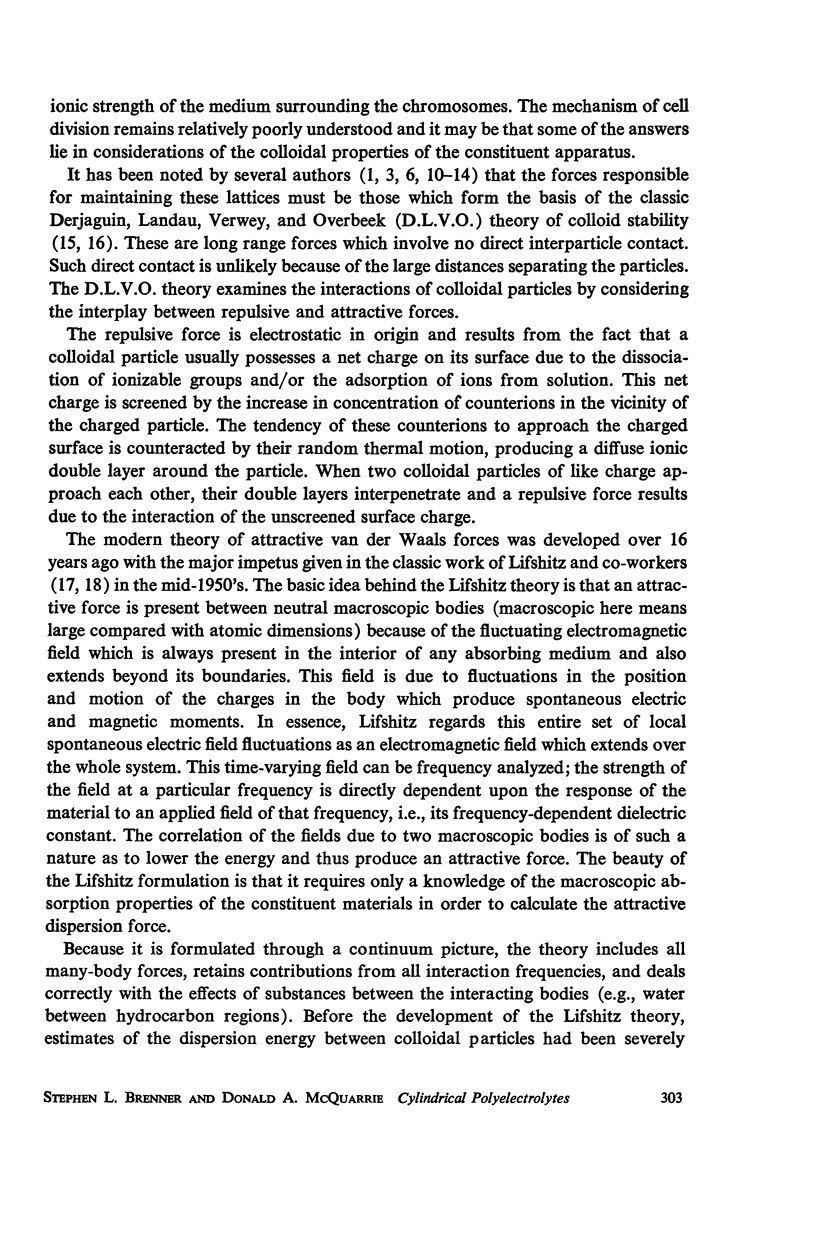
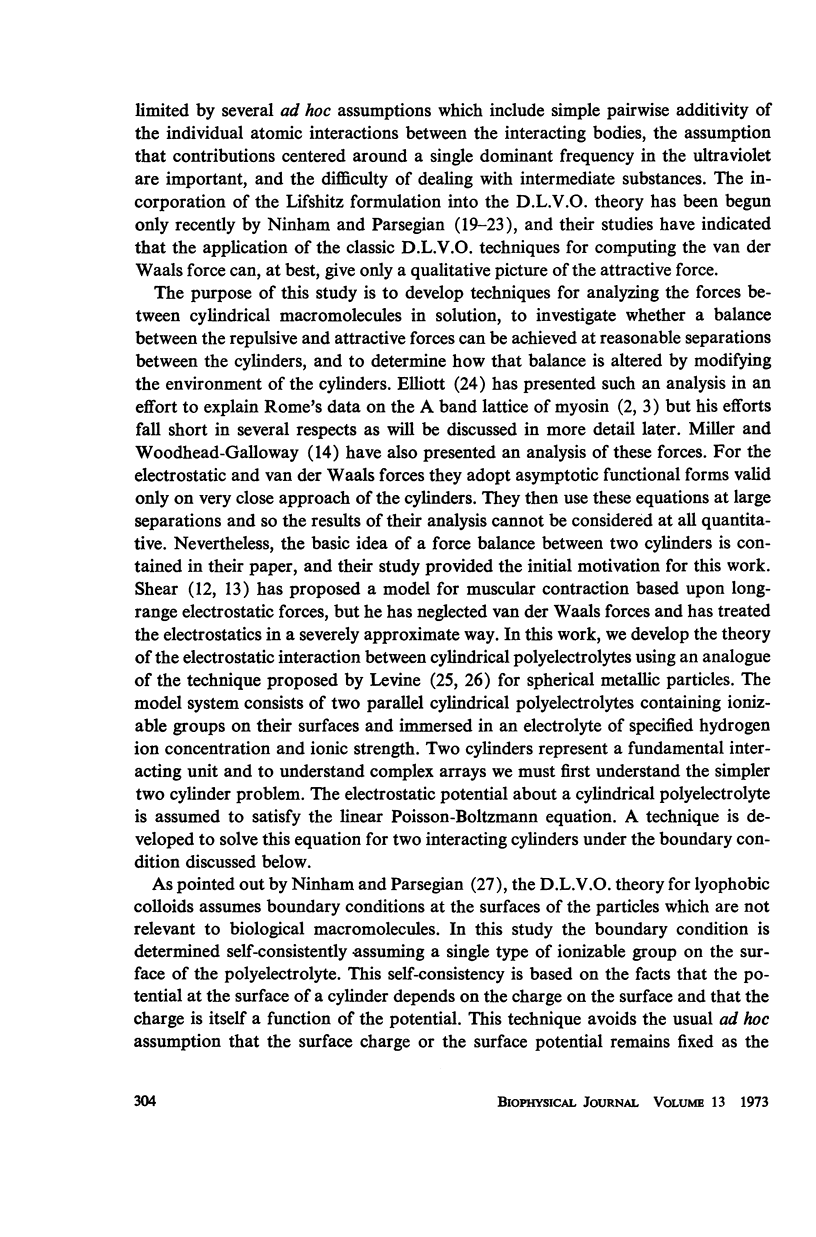
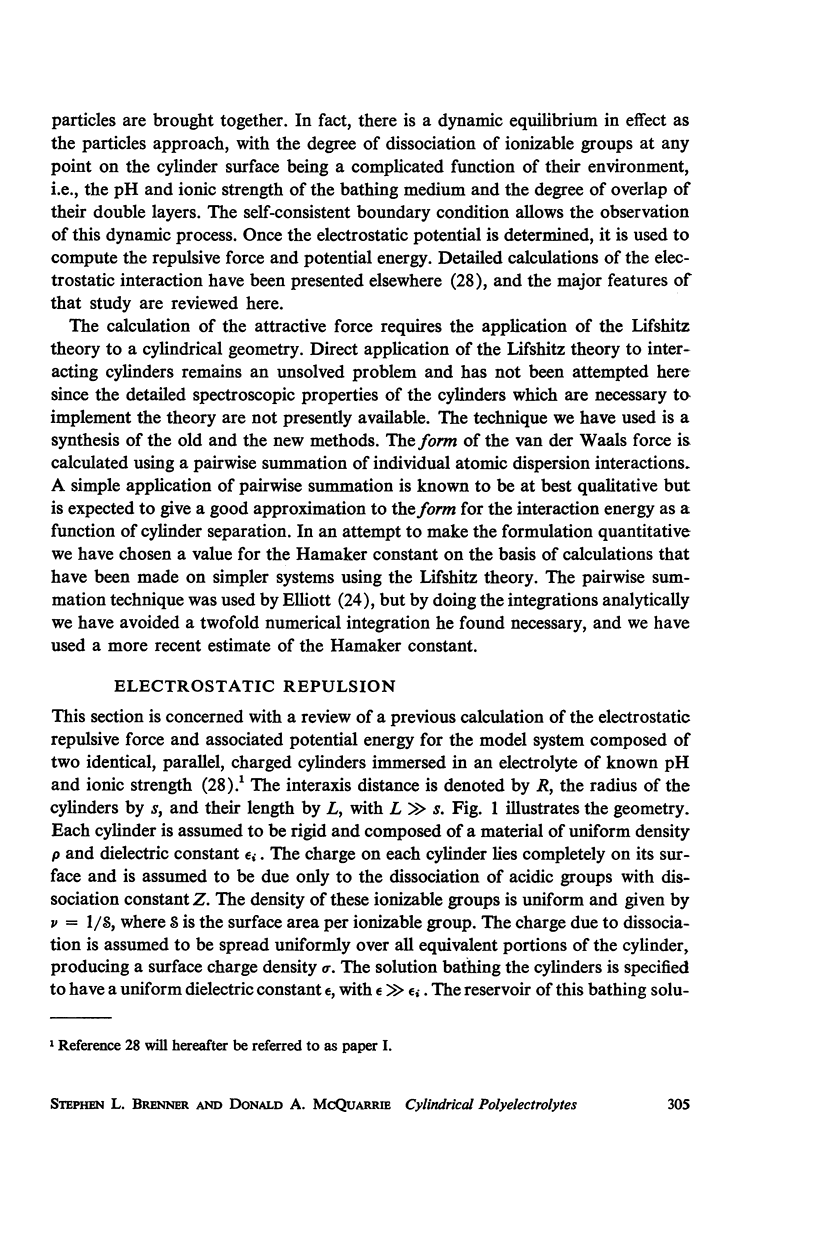
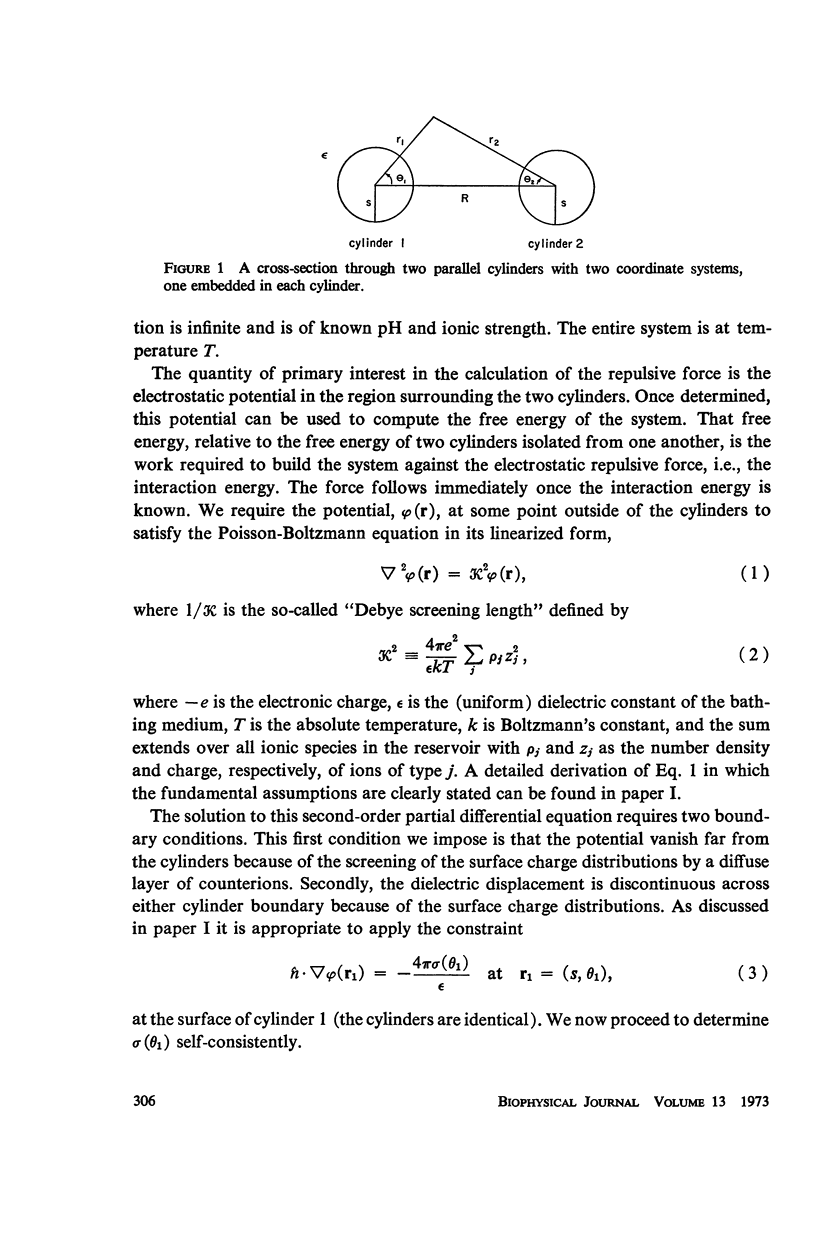
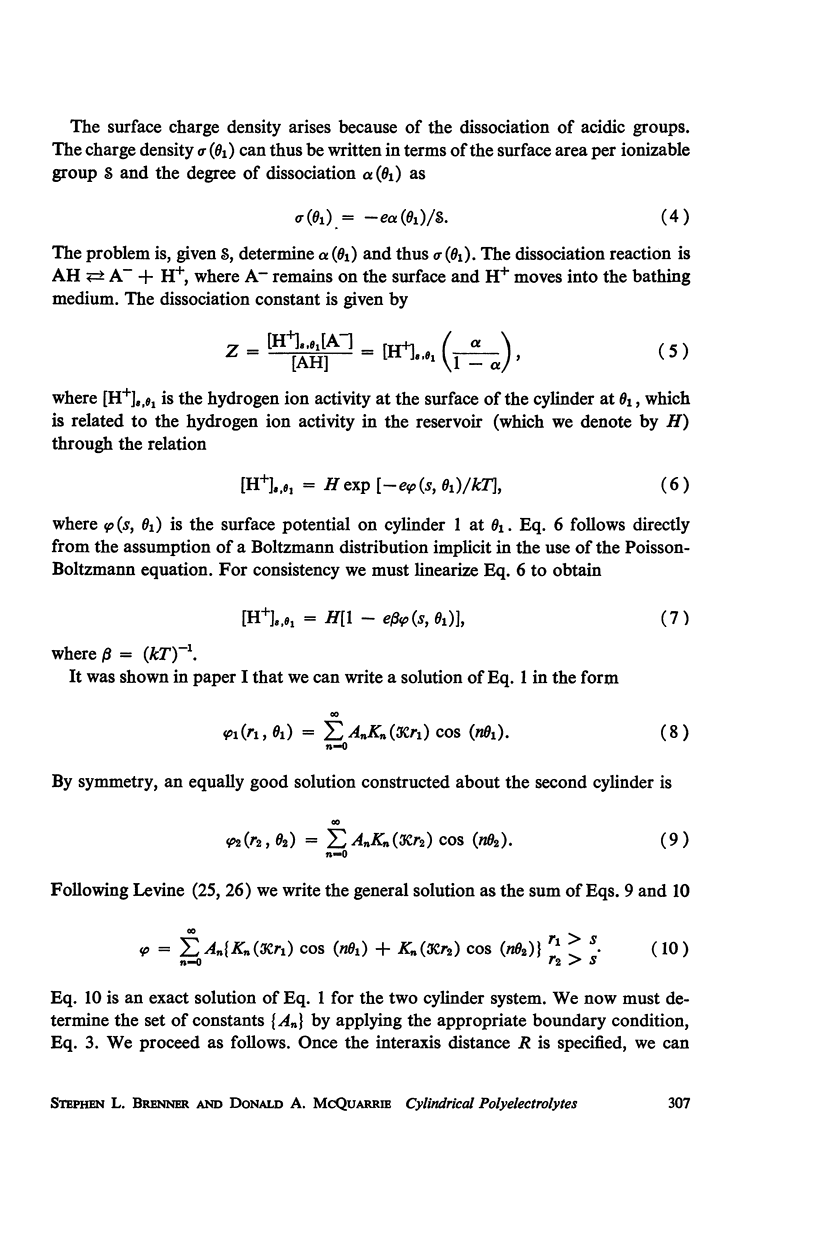
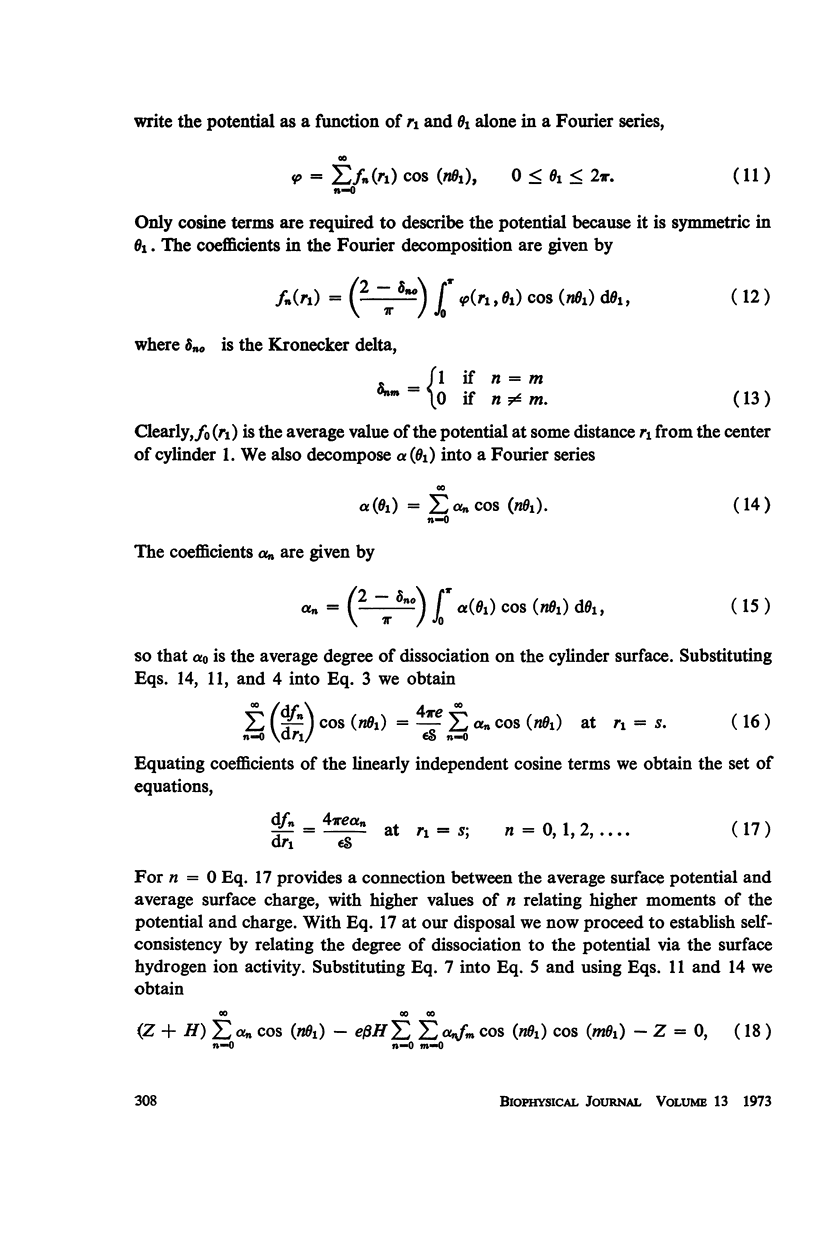
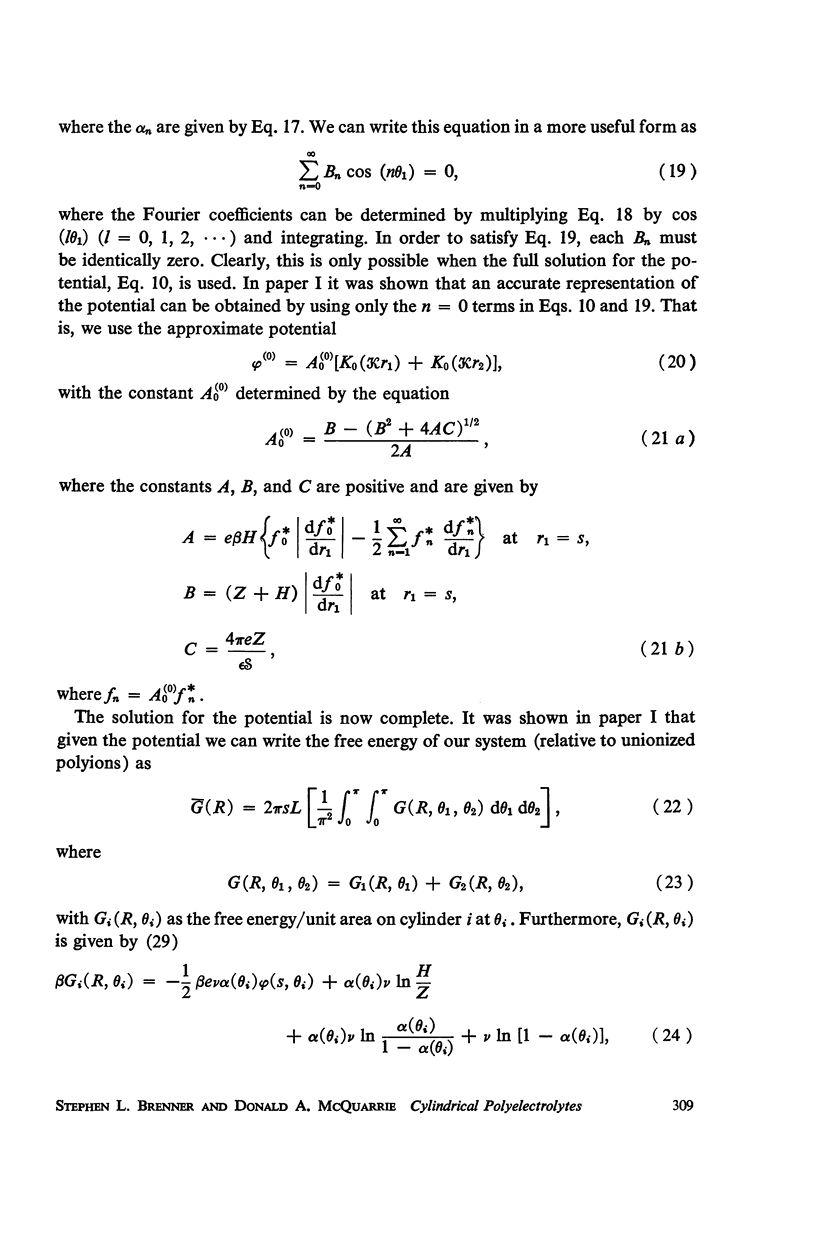
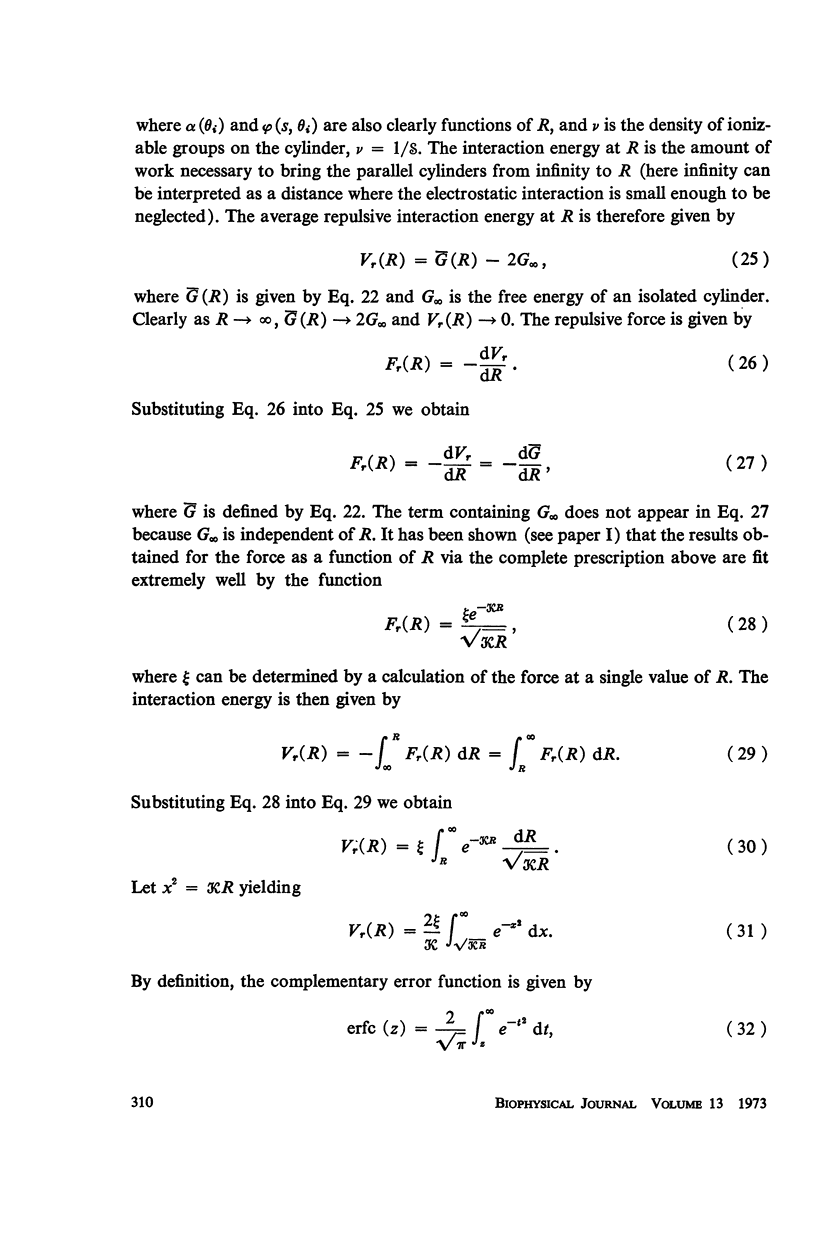
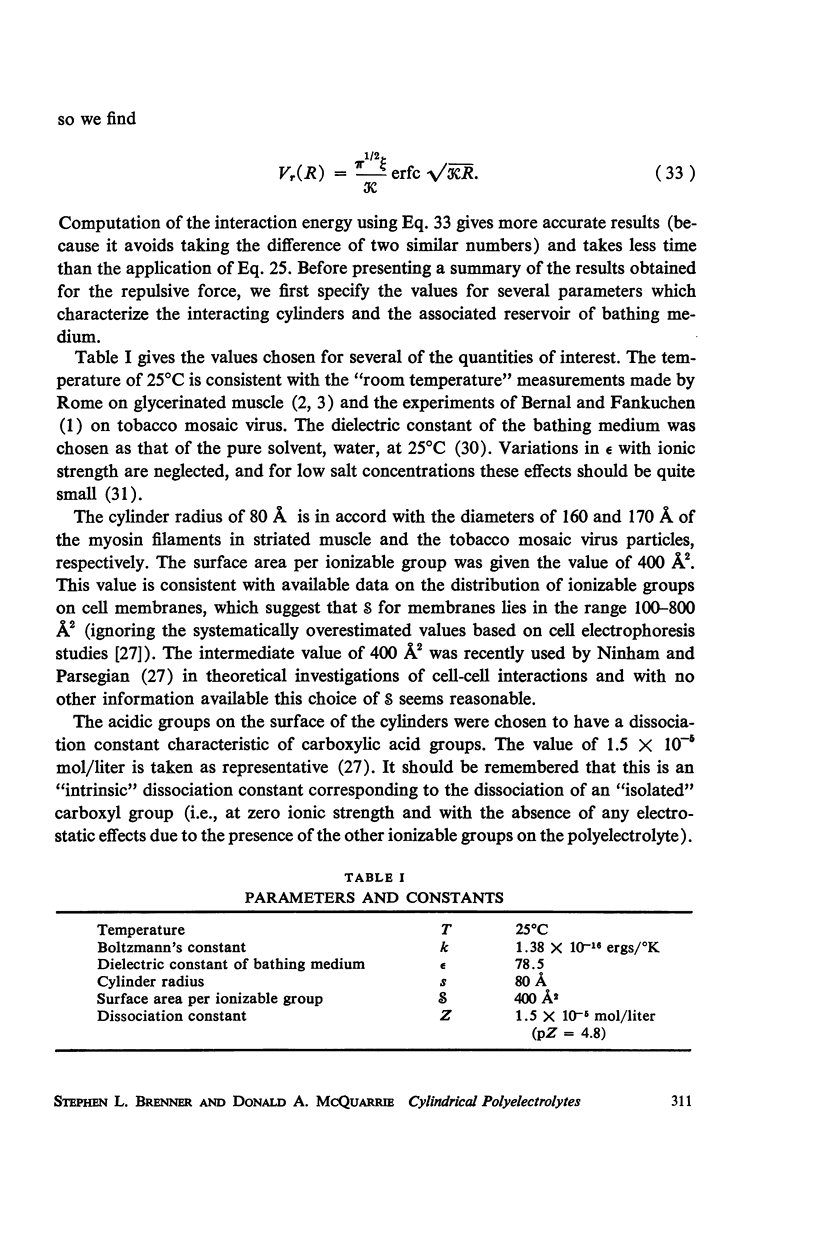
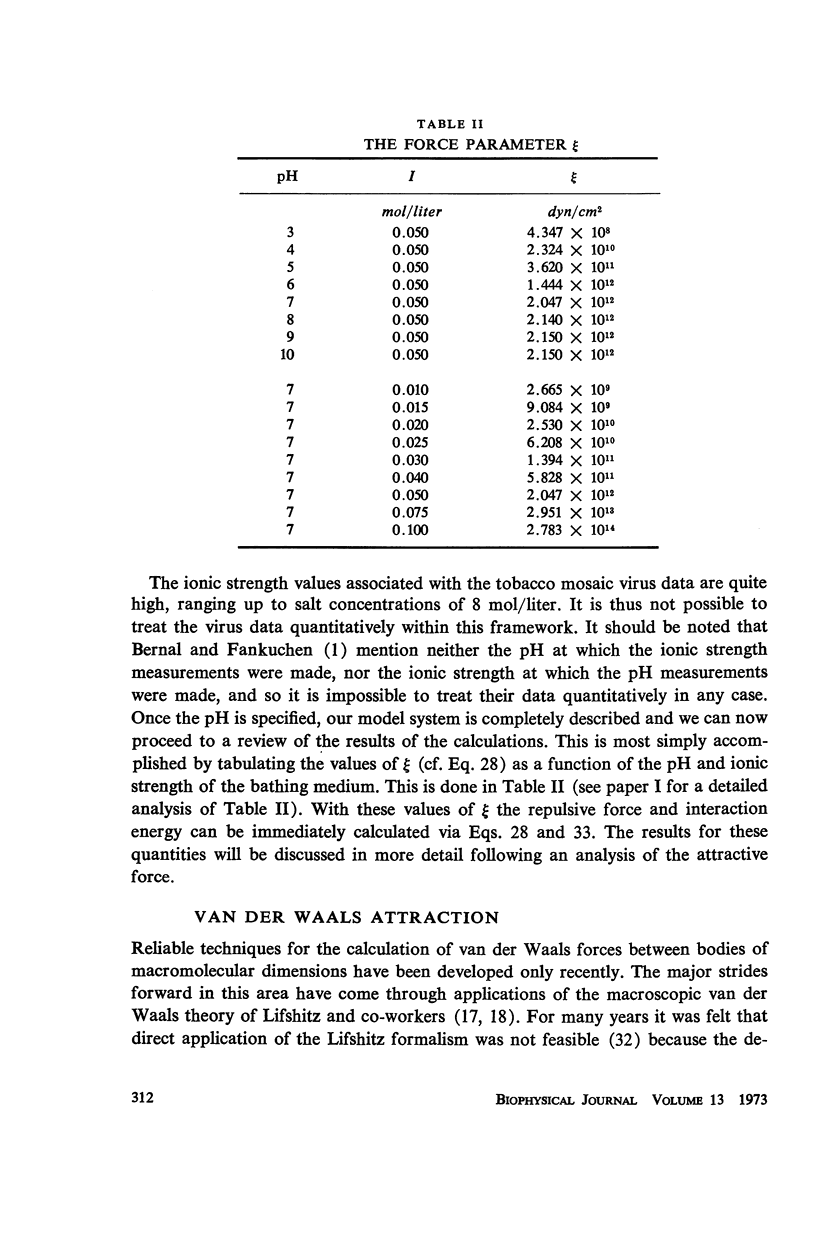
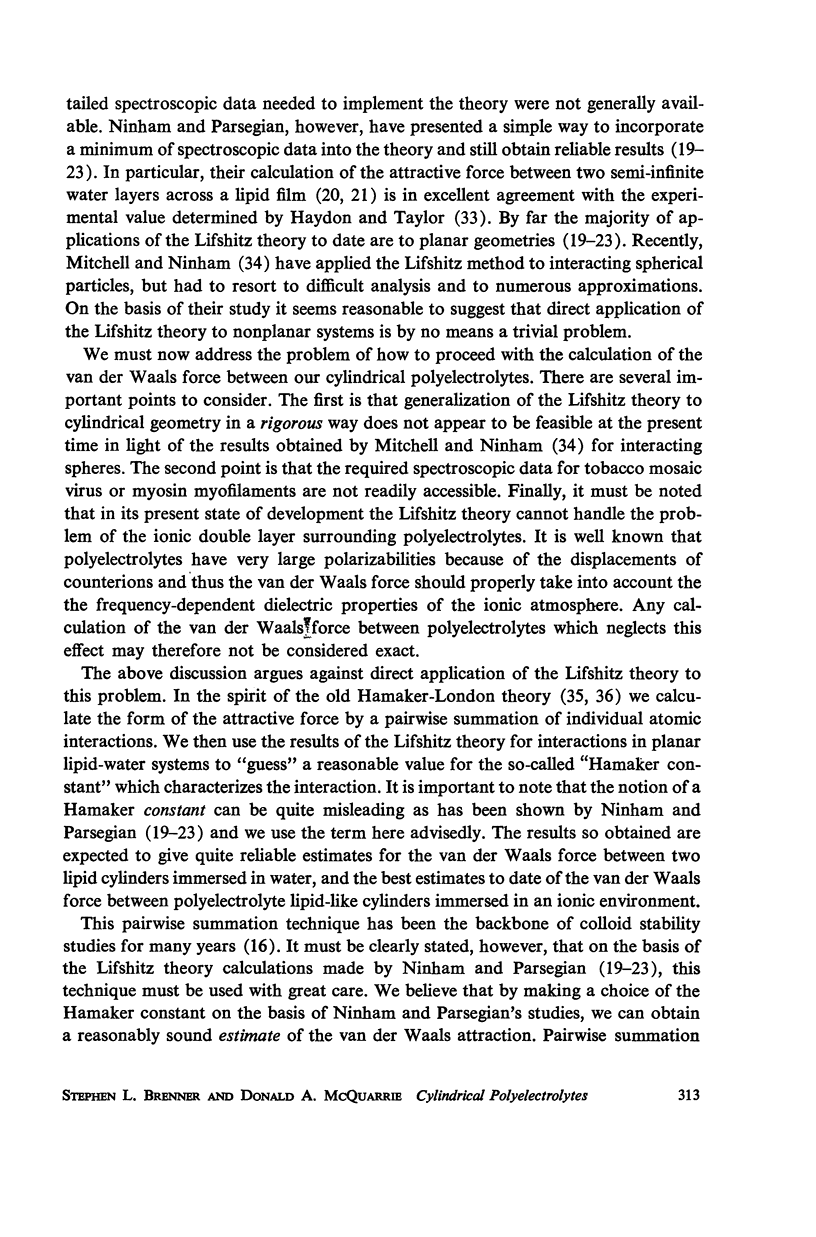
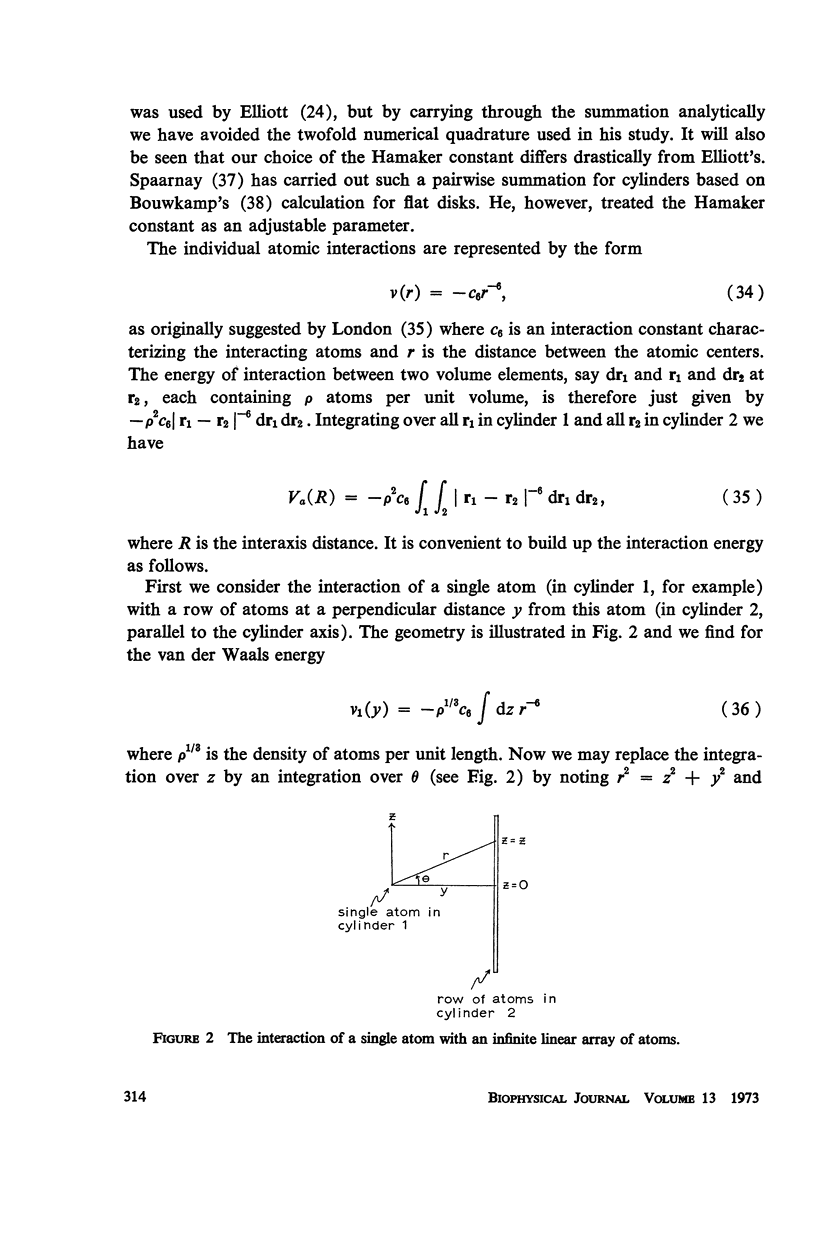
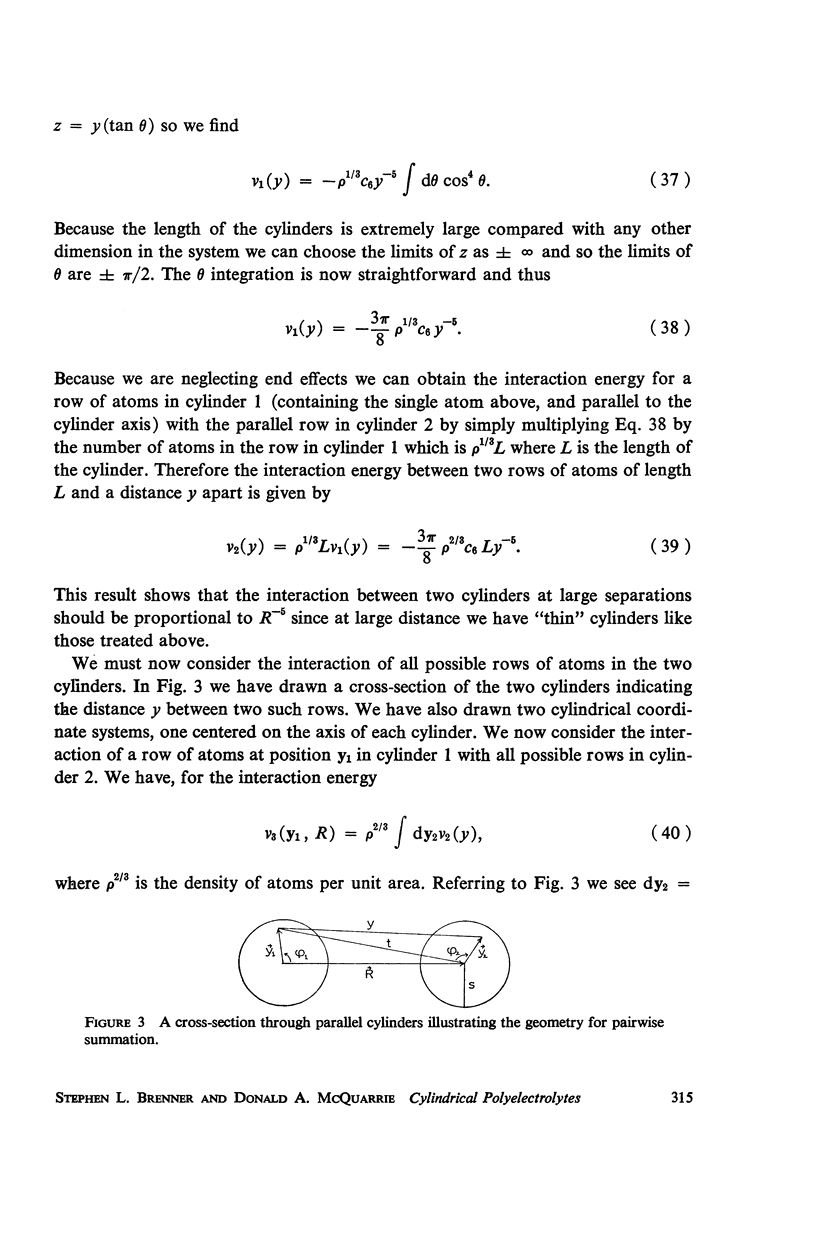
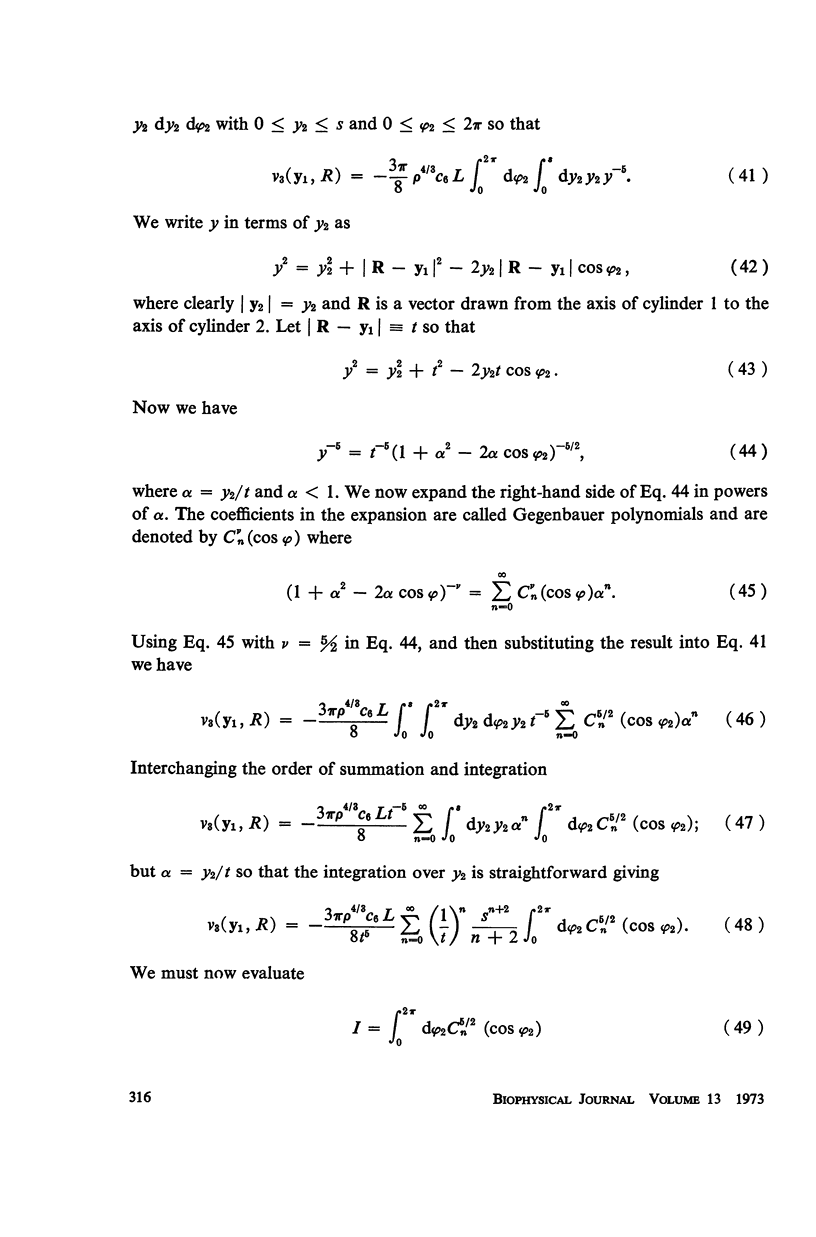
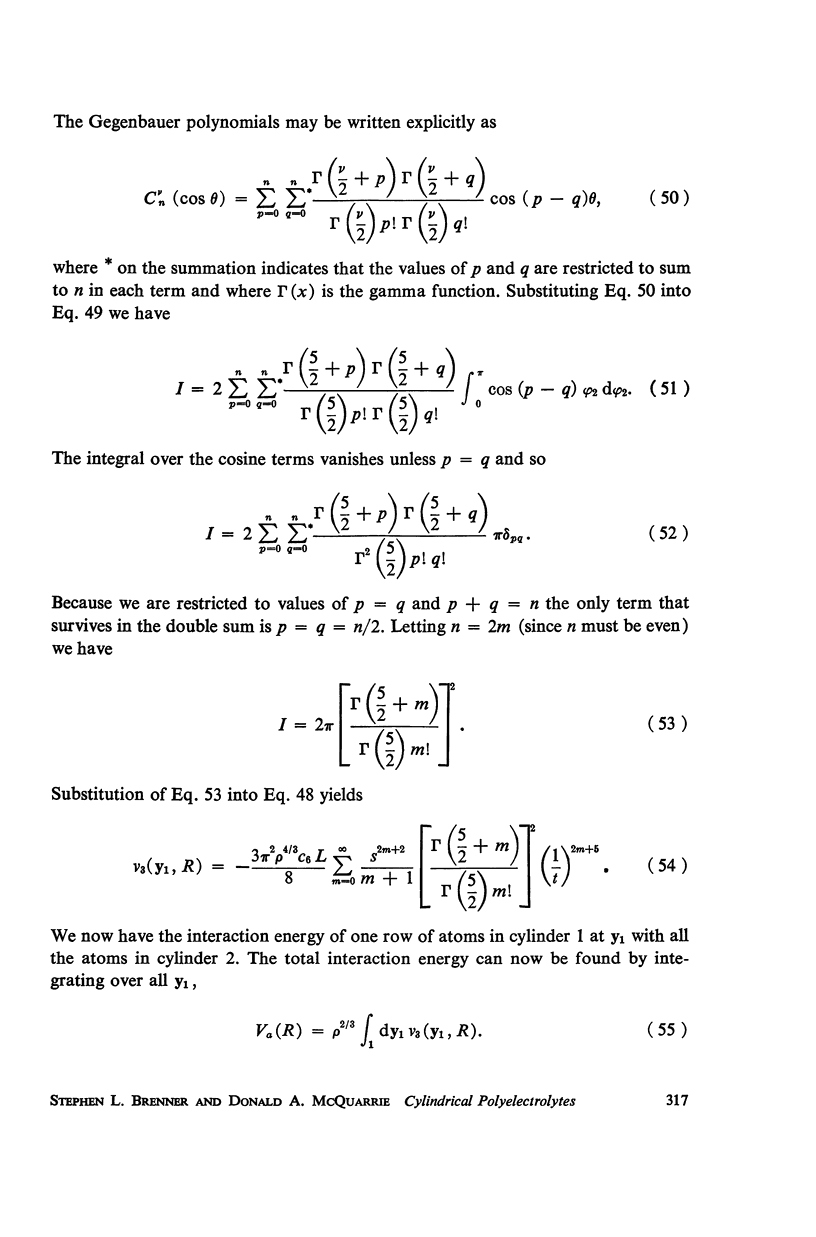
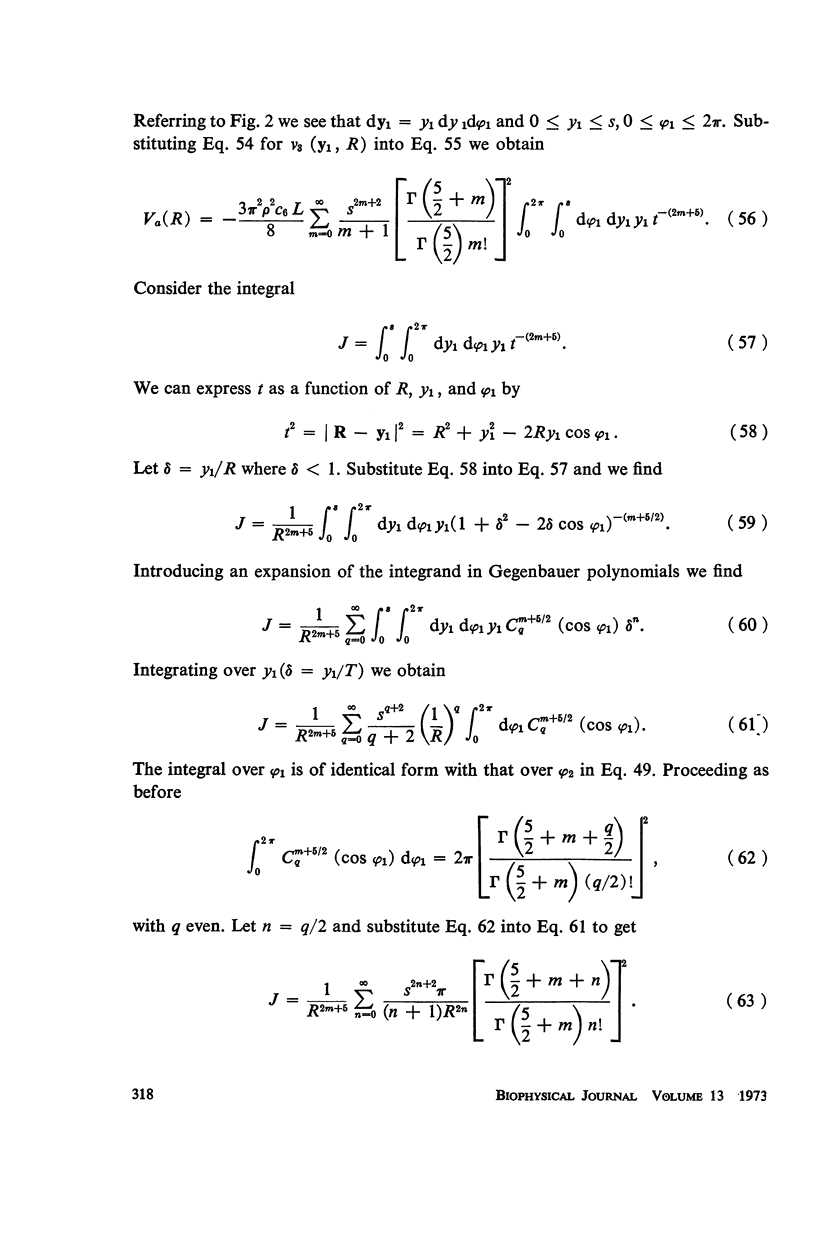
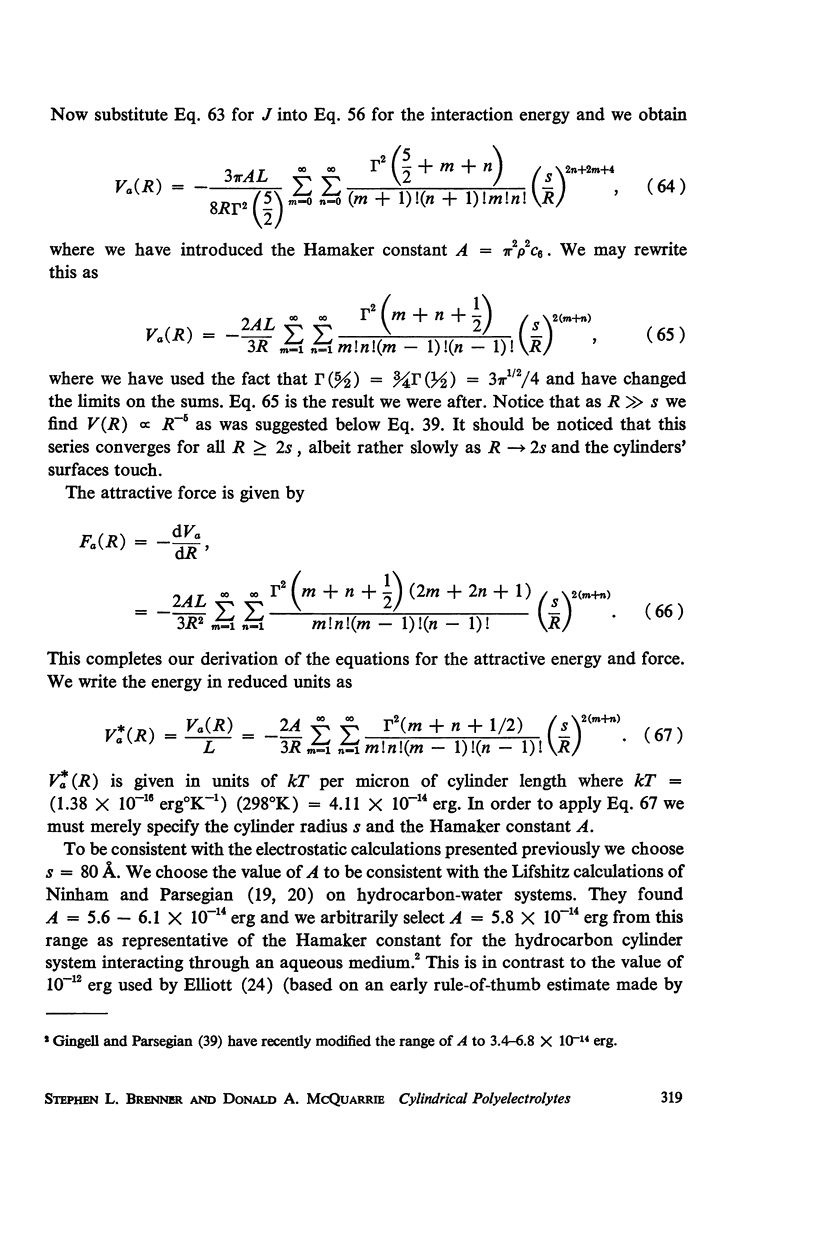
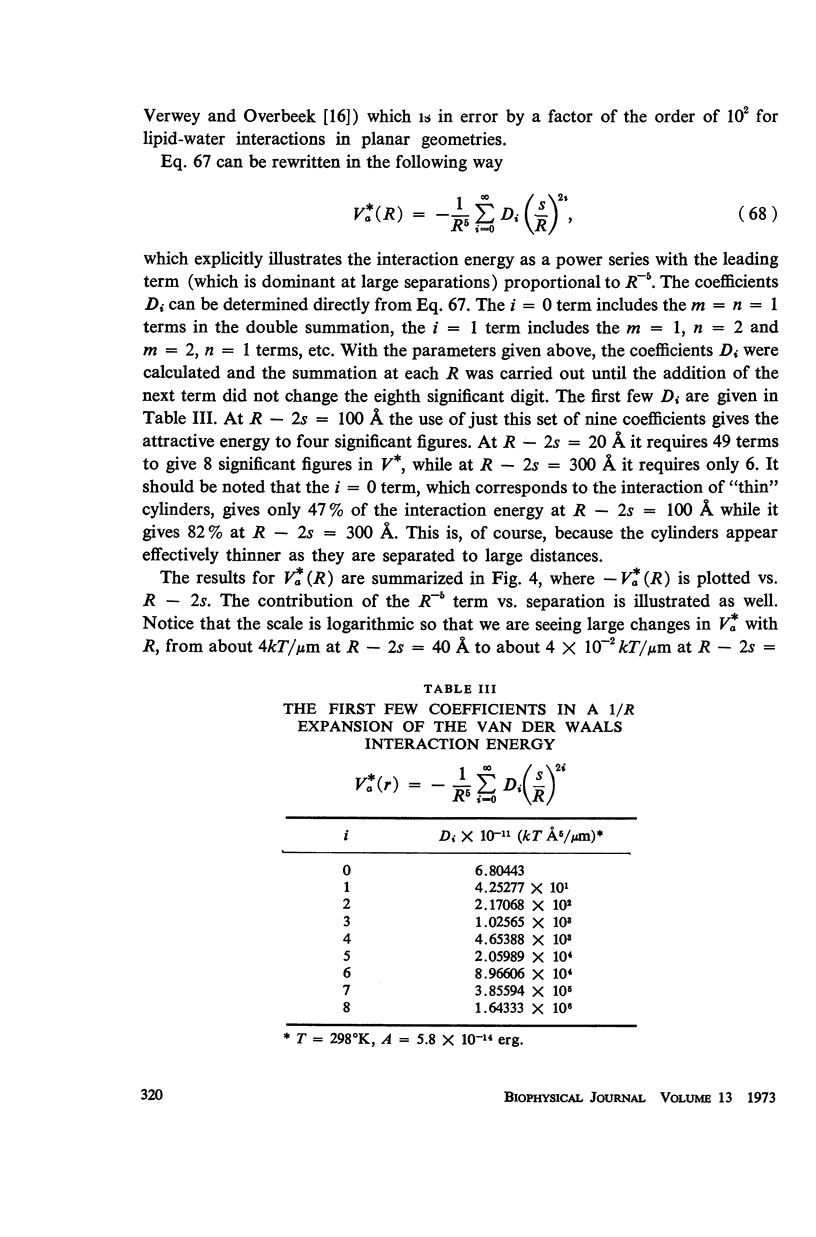
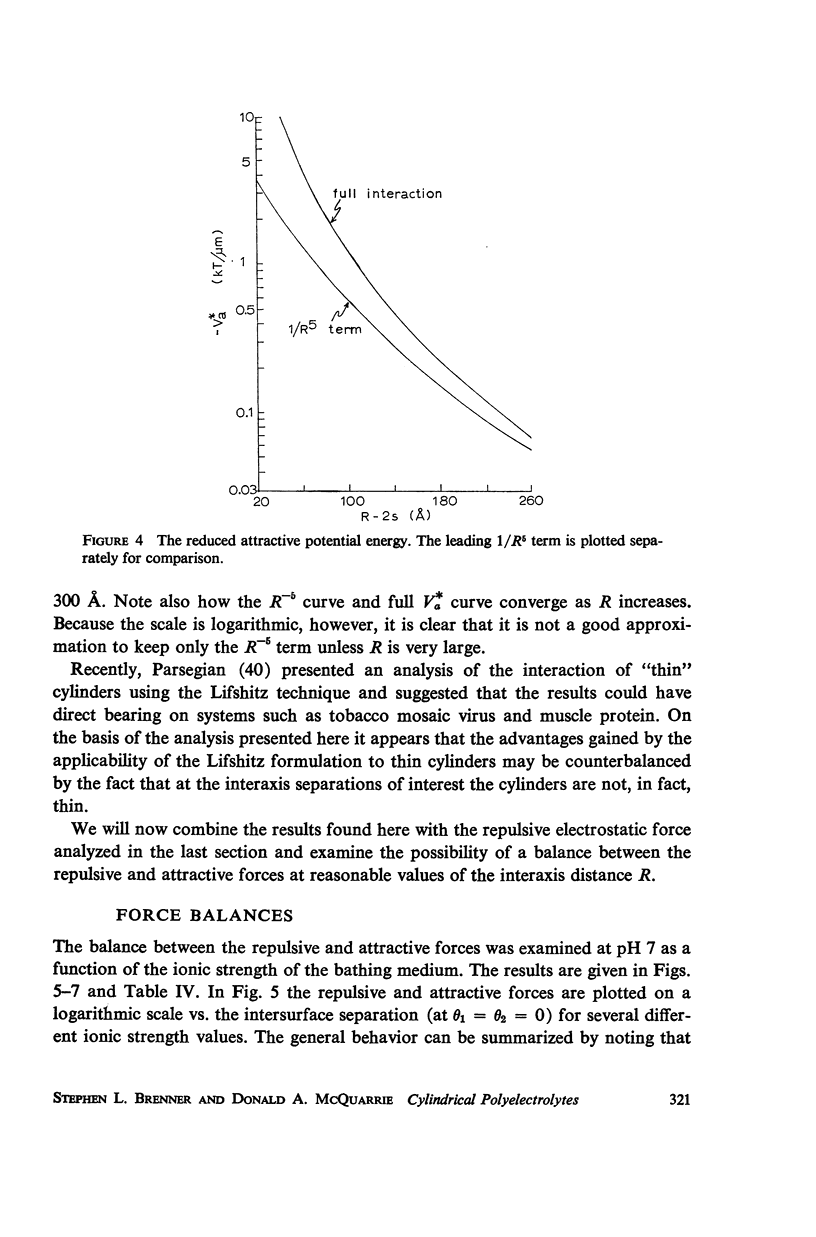
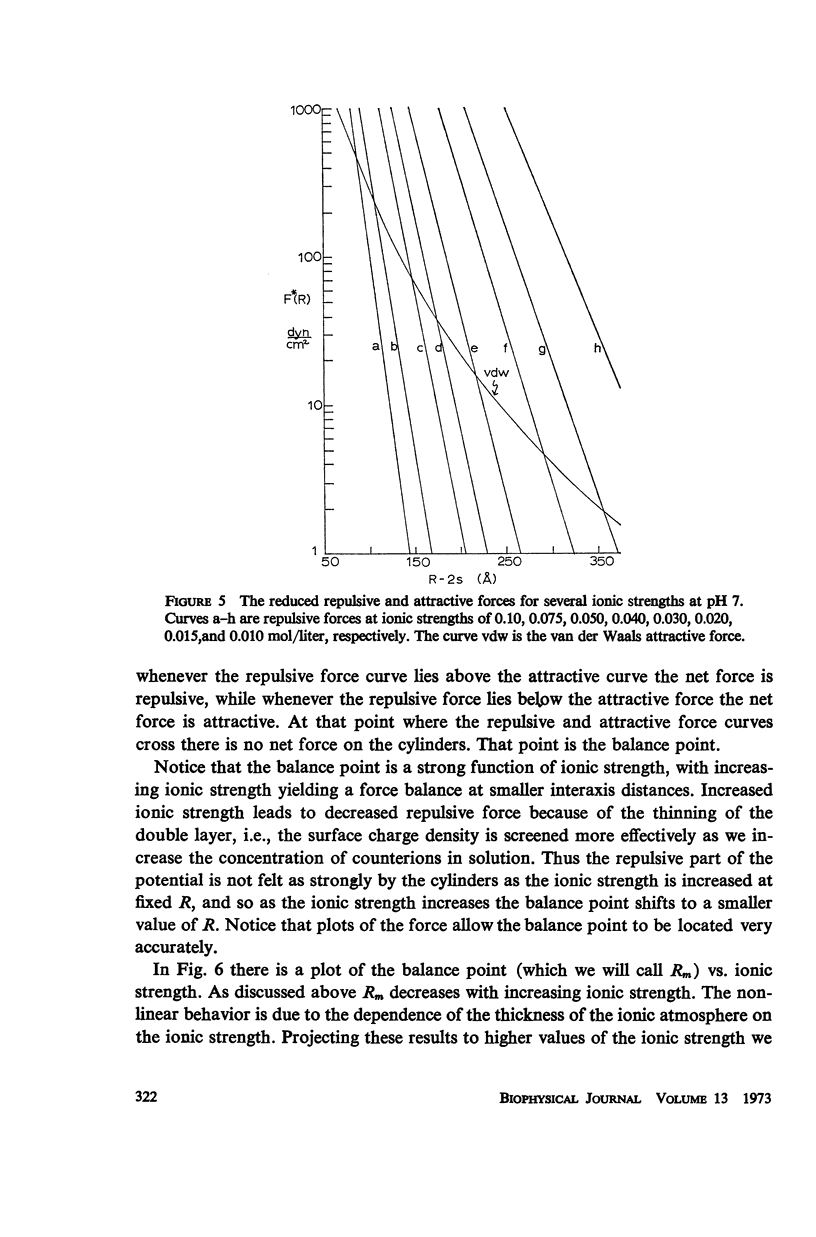
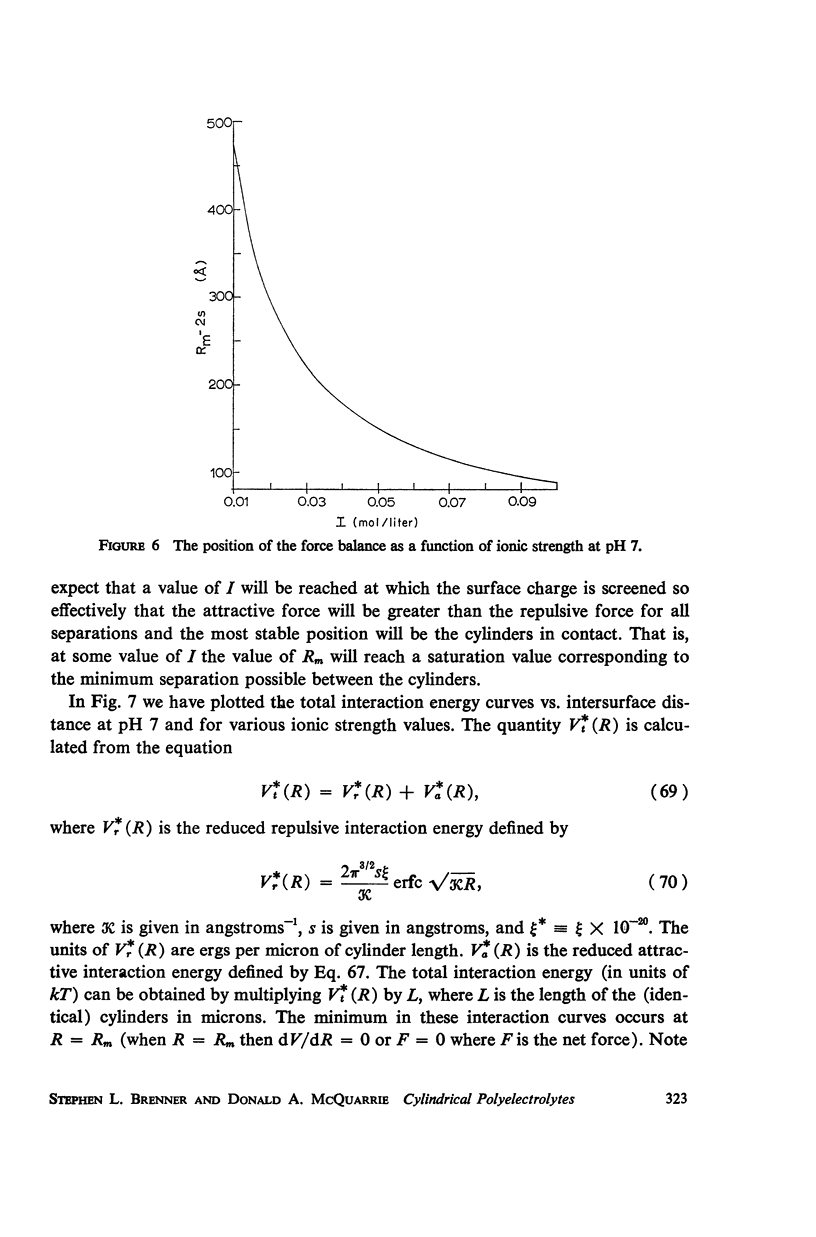
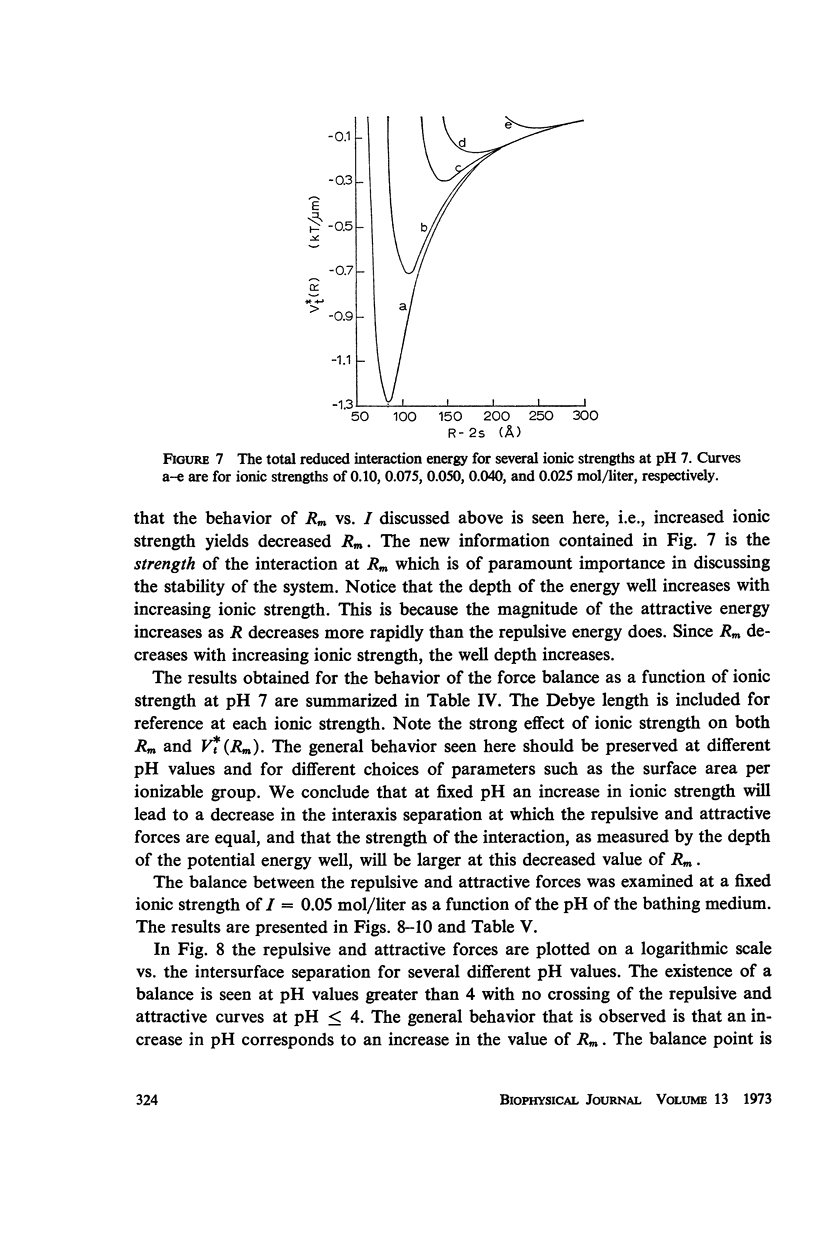
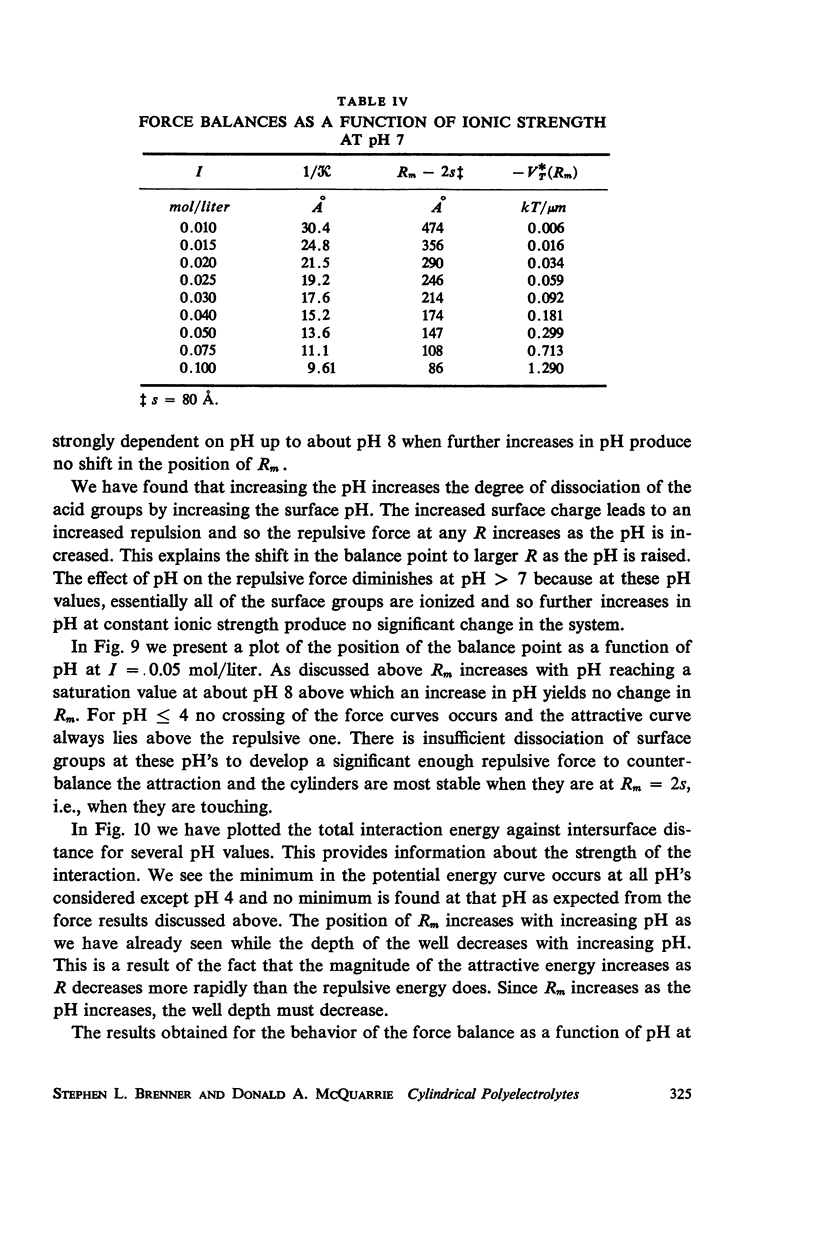
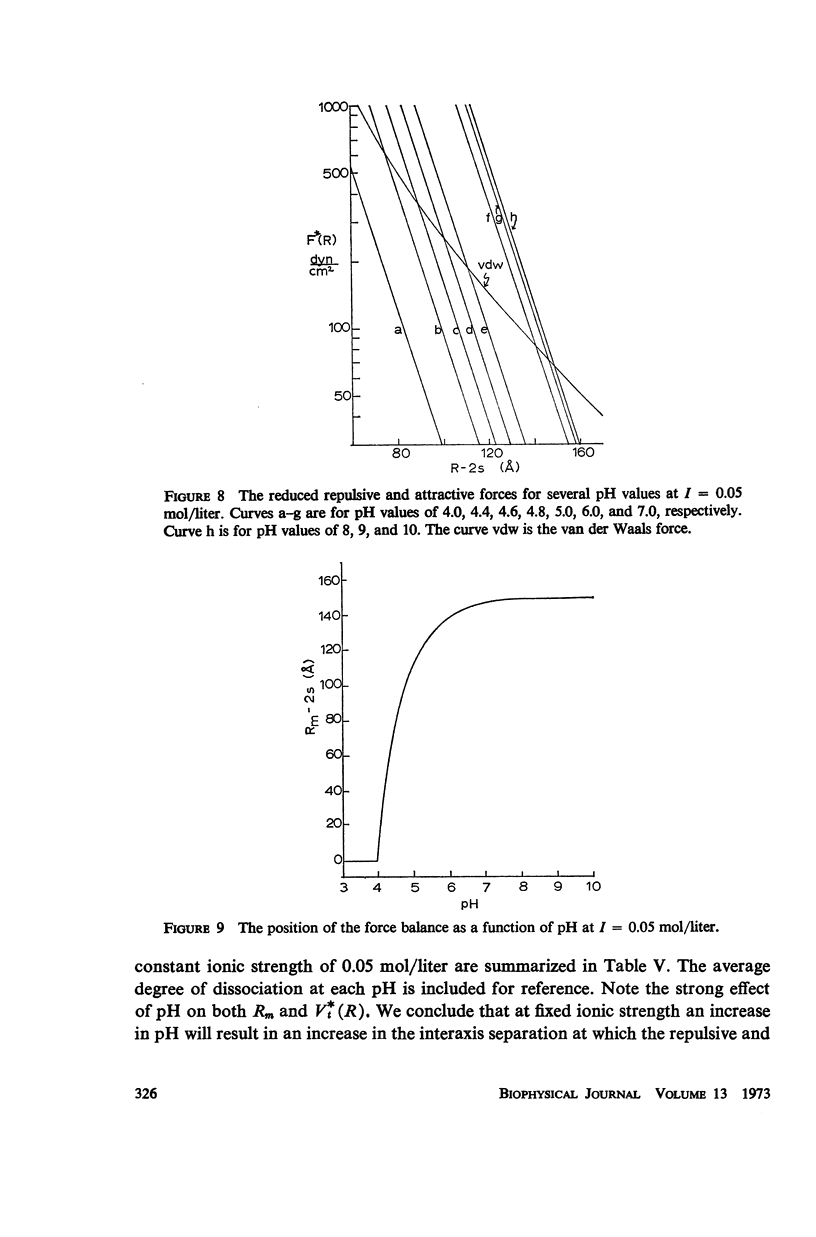
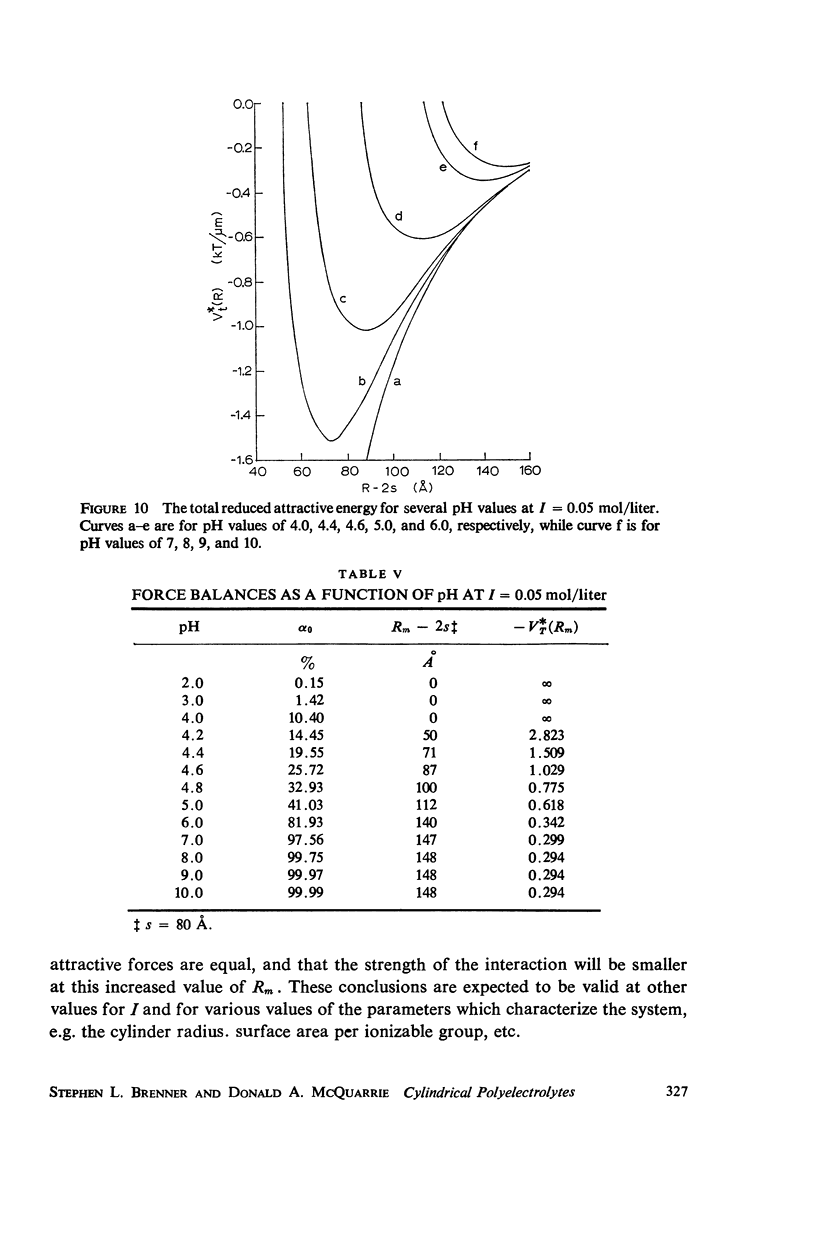
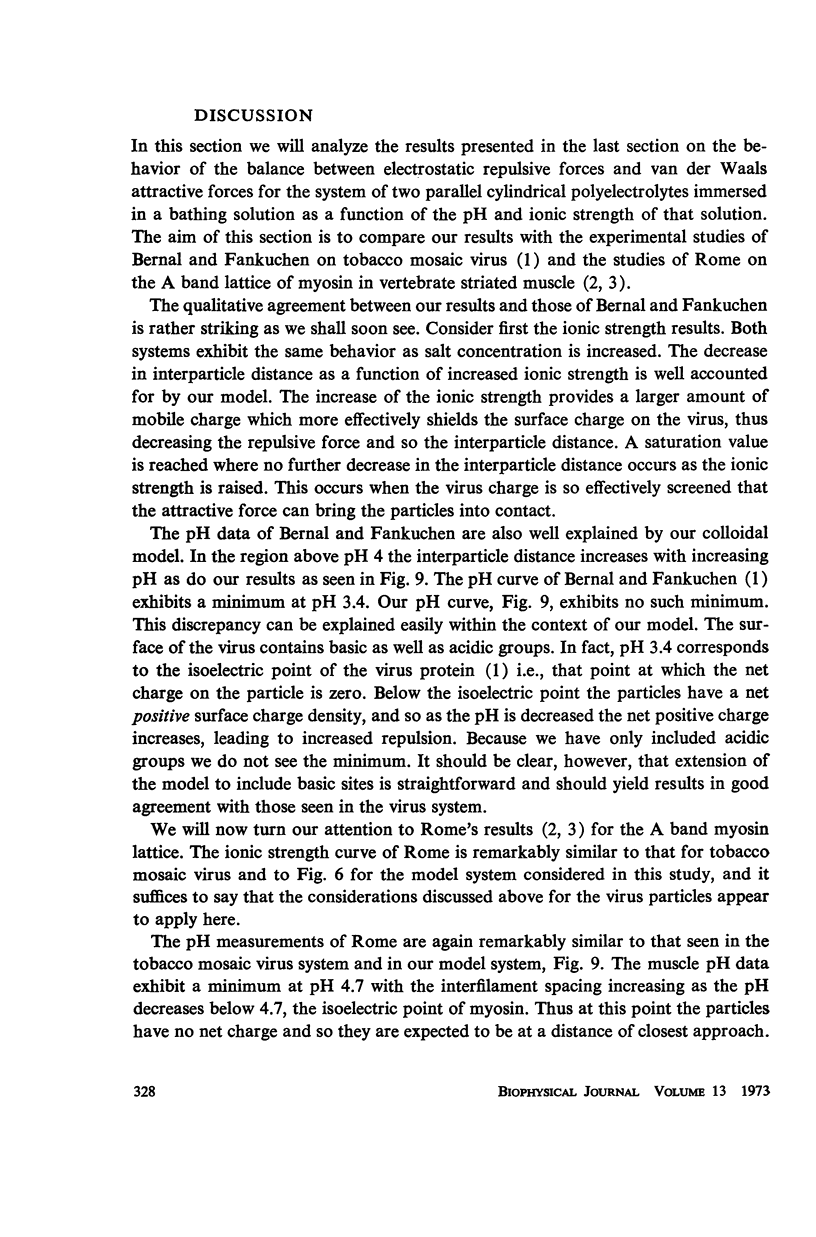
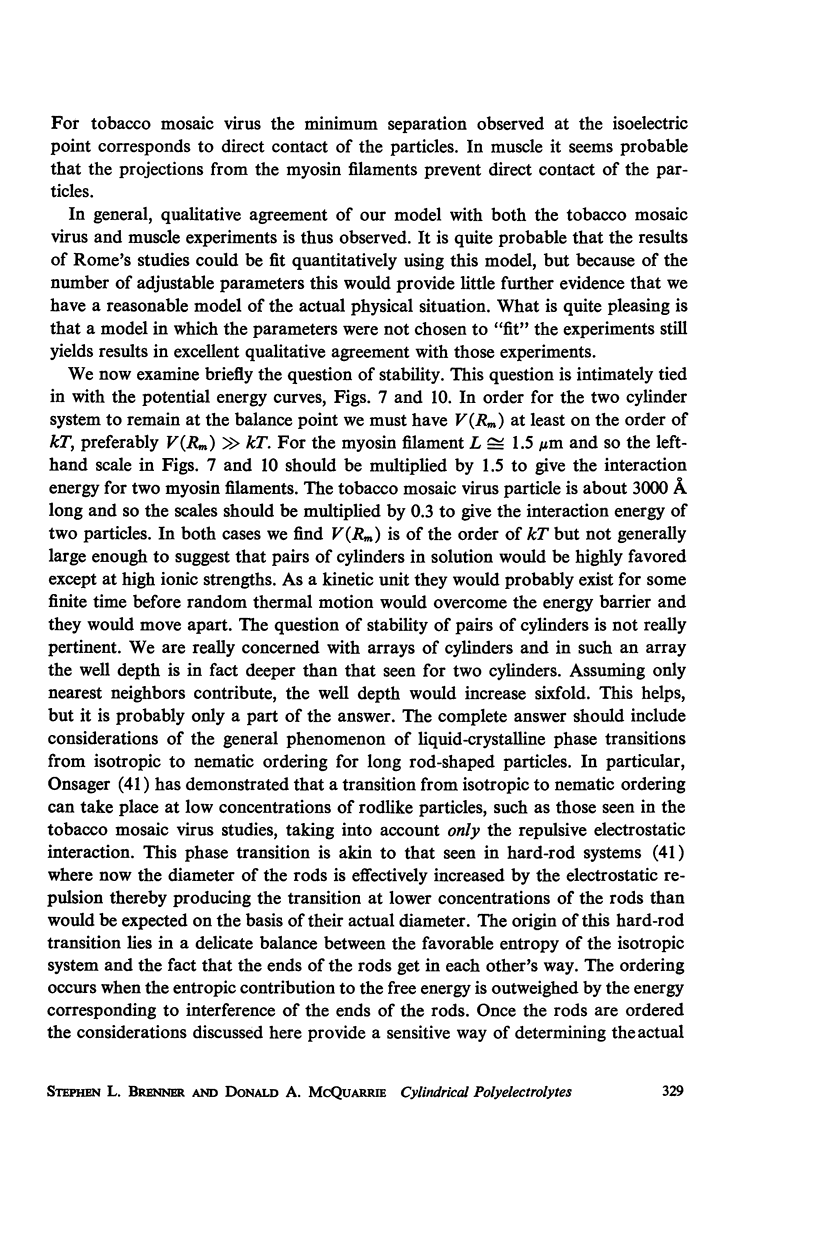
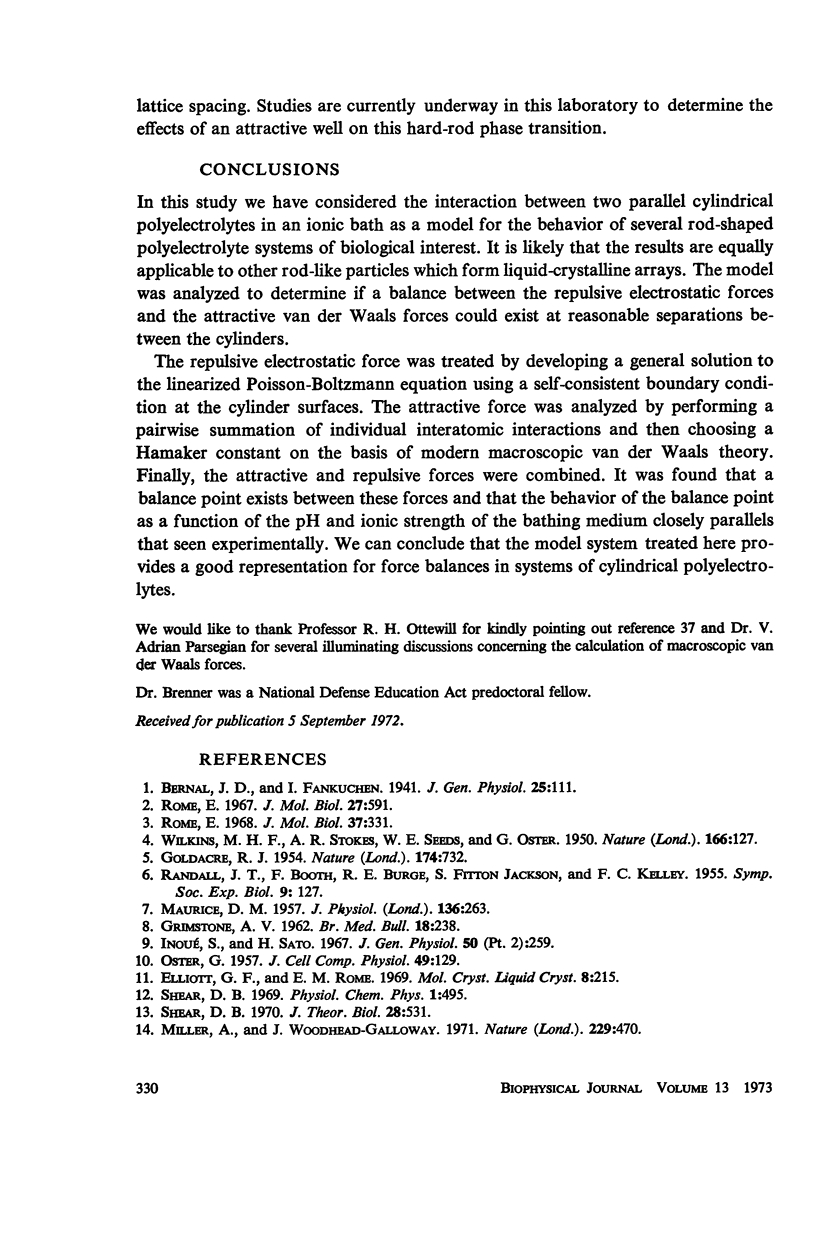
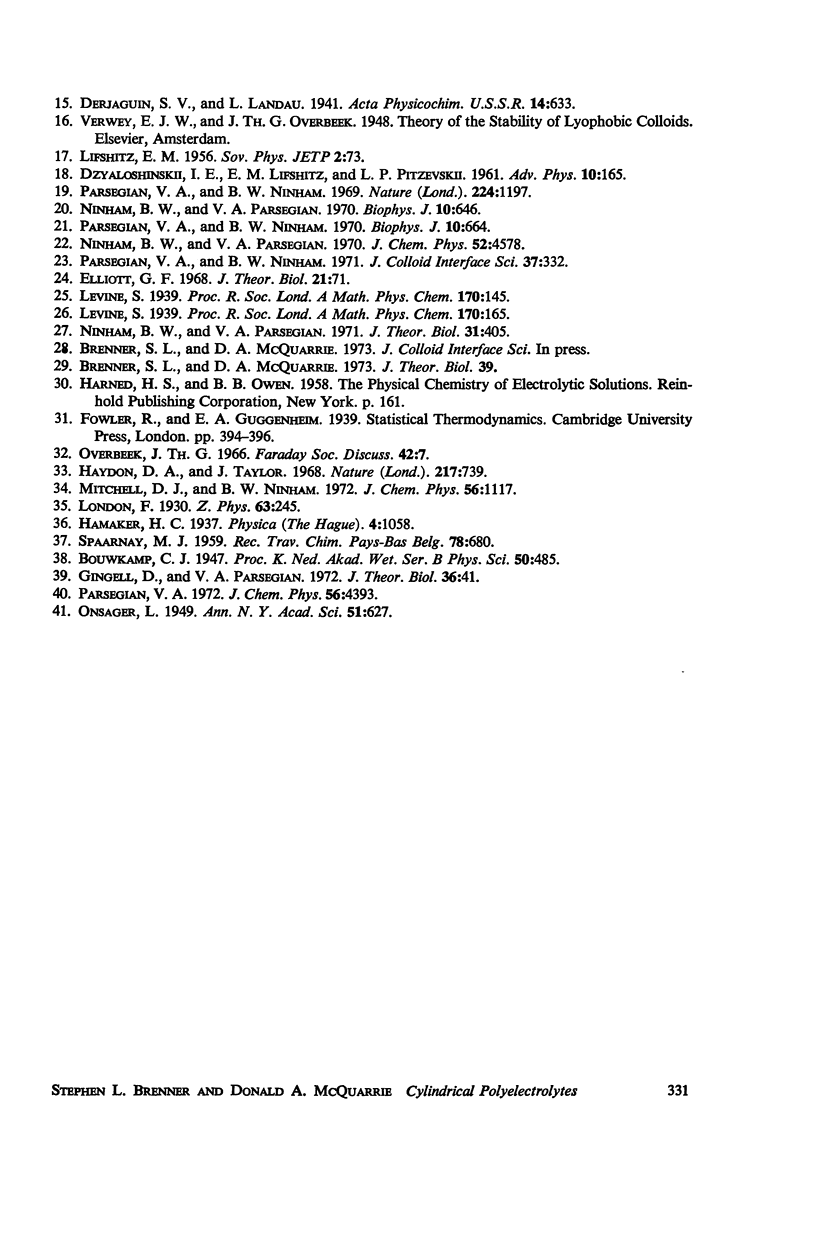
Selected References
These references are in PubMed. This may not be the complete list of references from this article.
- Bernal J. D., Fankuchen I. X-RAY AND CRYSTALLOGRAPHIC STUDIES OF PLANT VIRUS PREPARATIONS : I. INTRODUCTION AND PREPARATION OF SPECIMENS II. MODES OF AGGREGATION OF THE VIRUS PARTICLES. J Gen Physiol. 1941 Sep 20;25(1):111–146. doi: 10.1085/jgp.25.1.111. [DOI] [PMC free article] [PubMed] [Google Scholar]
- Elliott G. F. Force-balances and stability in hexagonally-packed polyelectrolyte systems. J Theor Biol. 1968 Oct;21(1):71–87. doi: 10.1016/0022-5193(68)90060-x. [DOI] [PubMed] [Google Scholar]
- GOLDACRE R. J. Crystalline bacteria arrays and specific long-range forces. Nature. 1954 Oct 16;174(4433):732–734. doi: 10.1038/174732a0. [DOI] [PubMed] [Google Scholar]
- Gingell D., Parsegian V. A. Computation of van der Waals interactions in aqueous systems using reflectivity data. J Theor Biol. 1972 Jul;36(1):41–52. doi: 10.1016/0022-5193(72)90175-0. [DOI] [PubMed] [Google Scholar]
- Inoué S., Sato H. Cell motility by labile association of molecules. The nature of mitotic spindle fibers and their role in chromosome movement. J Gen Physiol. 1967 Jul;50(6 Suppl):259–292. [PMC free article] [PubMed] [Google Scholar]
- MAURICE D. M. The structure and transparency of the cornea. J Physiol. 1957 Apr 30;136(2):263–286. doi: 10.1113/jphysiol.1957.sp005758. [DOI] [PMC free article] [PubMed] [Google Scholar]
- Miller A., Woodhead-Galloway J. Long range forces in muscle. Nature. 1971 Feb 12;229(5285):470–473. doi: 10.1038/229470a0. [DOI] [PubMed] [Google Scholar]
- Ninham B. W., Parsegian V. A. Electrostatic potential between surfaces bearing ionizable groups in ionic equilibrium with physiologic saline solution. J Theor Biol. 1971 Jun;31(3):405–428. doi: 10.1016/0022-5193(71)90019-1. [DOI] [PubMed] [Google Scholar]
- Ninham B. W., Parsegian V. A. Van der Waals forces. Special characteristics in lipid-water systems and a general method of calculation based on the Lifshitz theory. Biophys J. 1970 Jul;10(7):646–663. doi: 10.1016/S0006-3495(70)86326-3. [DOI] [PMC free article] [PubMed] [Google Scholar]
- OSTER G. Organization in biocolloidal systems. J Cell Physiol Suppl. 1957 May;49(Suppl 1):129–140. doi: 10.1002/jcp.1030490413. [DOI] [PubMed] [Google Scholar]
- Parsegian V. A., Ninham B. W. Temperature-dependent van der Waals forces. Biophys J. 1970 Jul;10(7):664–674. doi: 10.1016/S0006-3495(70)86327-5. [DOI] [PMC free article] [PubMed] [Google Scholar]
- Rome E. Light and X-ray diffraction studies of the filament lattice of glycerol-extracted rabbit psoas muscle. J Mol Biol. 1967 Aug 14;27(3):591–602. doi: 10.1016/0022-2836(67)90061-7. [DOI] [PubMed] [Google Scholar]
- Rome E. X-ray diffraction studies of the filament lattice of striated muscle in various bathing media. J Mol Biol. 1968 Oct 28;37(2):331–344. doi: 10.1016/0022-2836(68)90272-6. [DOI] [PubMed] [Google Scholar]
- Shear D. B. Electrostatic forces in muscle contraction. J Theor Biol. 1970 Sep;28(3):531–546. doi: 10.1016/0022-5193(70)90087-1. [DOI] [PubMed] [Google Scholar]
- WILKINS M. H. F., STOKES A. R., SEEDS W. E., OSTER G. Tobacco mosaic virus crystals and three-dimensional microscopic vision. Nature. 1950 Jul 22;166(4212):127–129. doi: 10.1038/166127a0. [DOI] [PubMed] [Google Scholar]