Abstract
Enhanced transport of trace metal in porous media can occur in the presence of a ligand or "carrier" that has a high affinity for binding the pollutant, is dispersed and mobile in the soil environment, is recalcitrant with respect to microbial degradation, and is acceptable to the public. These aspects of the facilitated transport to trace metals are discussed with respect to a naturally occurring carrier: extracellular polymers of bacterial origin. The literature is reviewed regarding the production and composition of bacterial extracellular polymers, the processes relevant to the facilitated transport of trace metals in soil by bacterial polymers, and potential for transformation of polymers in soils by microbial degradation. Model calculations of contaminant retardation are presented for the case of polymer-mediated transport of cadmium in a sandy aquifer material. The available information suggests that extracellular polymers can bind metal ions and are mobile in the soil environment. Extracellular polymers also appear to be relatively slowly degraded by soil microorganisms. These properties and the supporting model calculations indicate that extracellular polymers of bacterial origin merit consideration as agents that may be applied to contaminated soils to enhance trace metal mobility.
Full text
PDF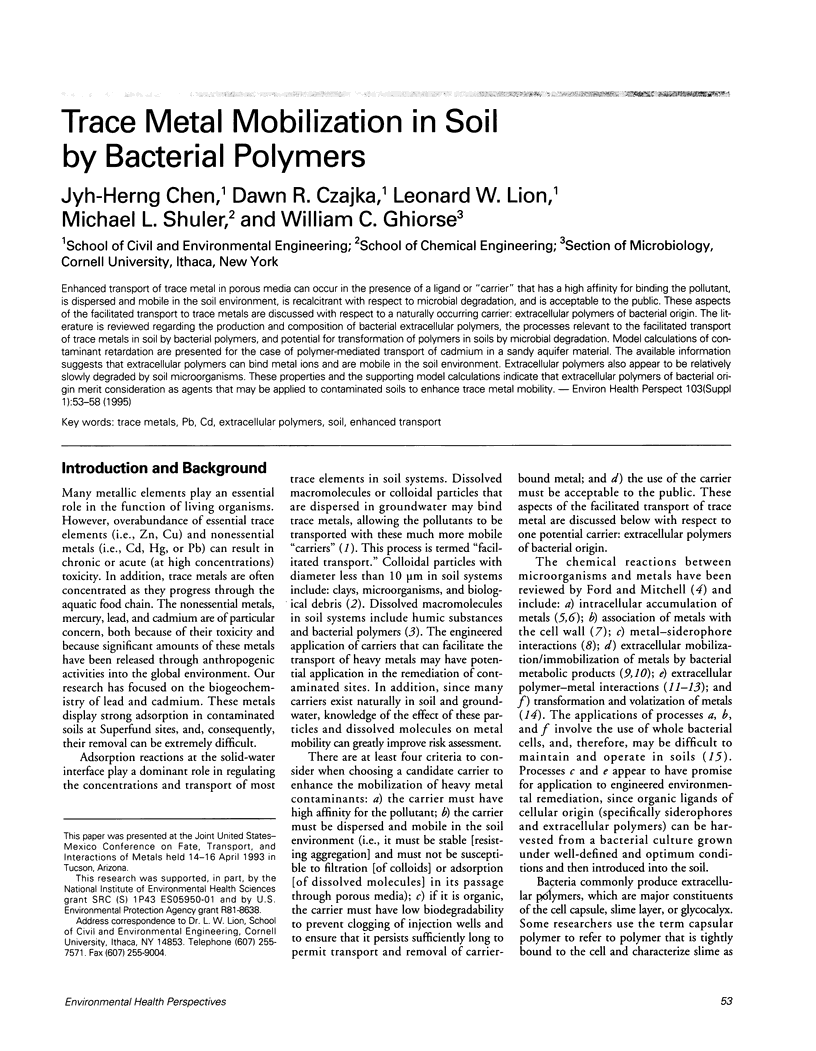
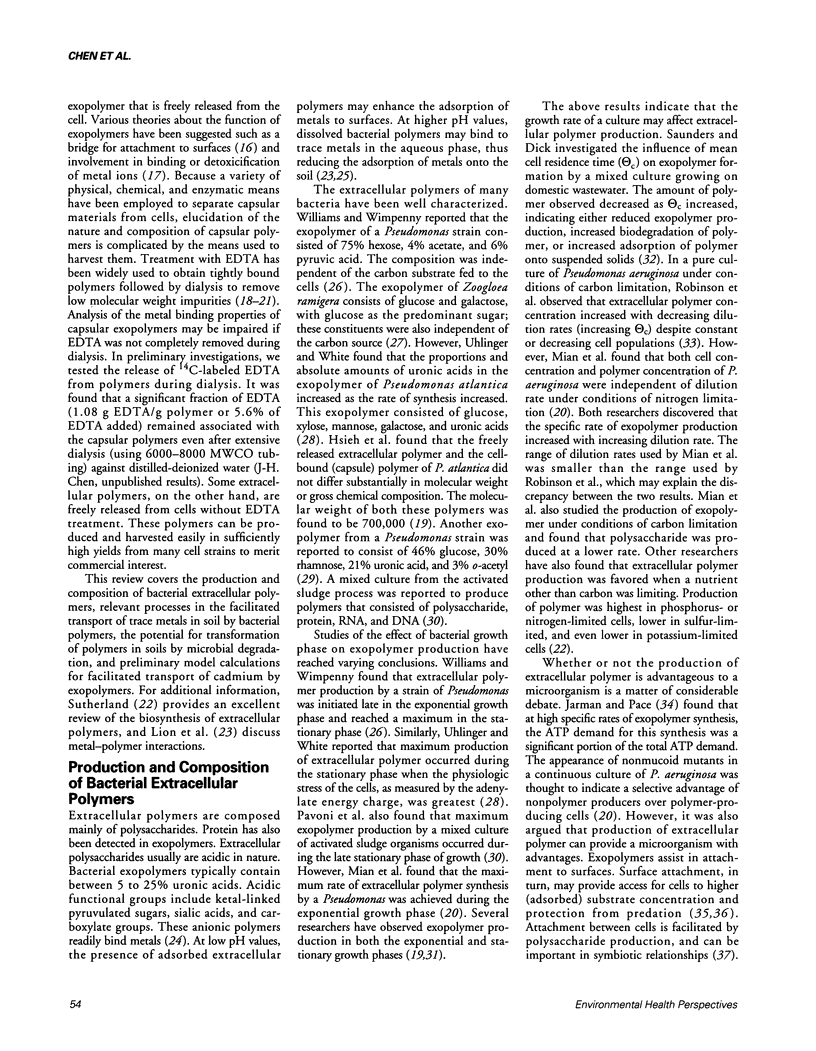
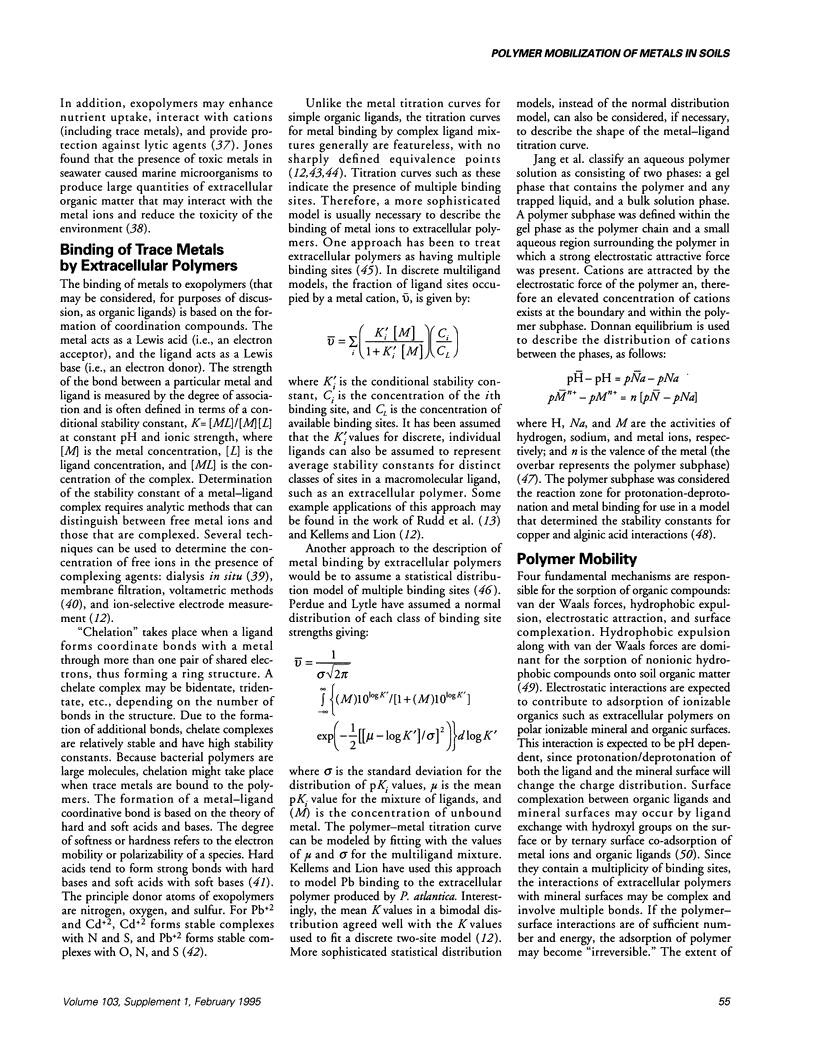
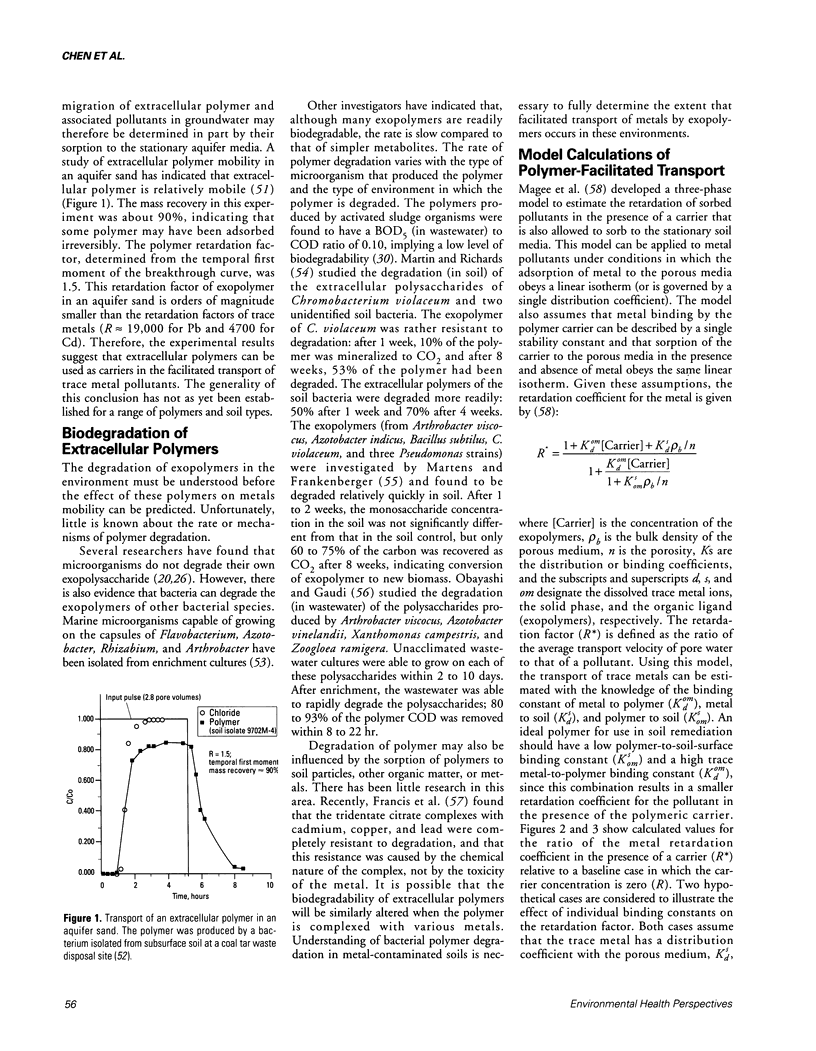
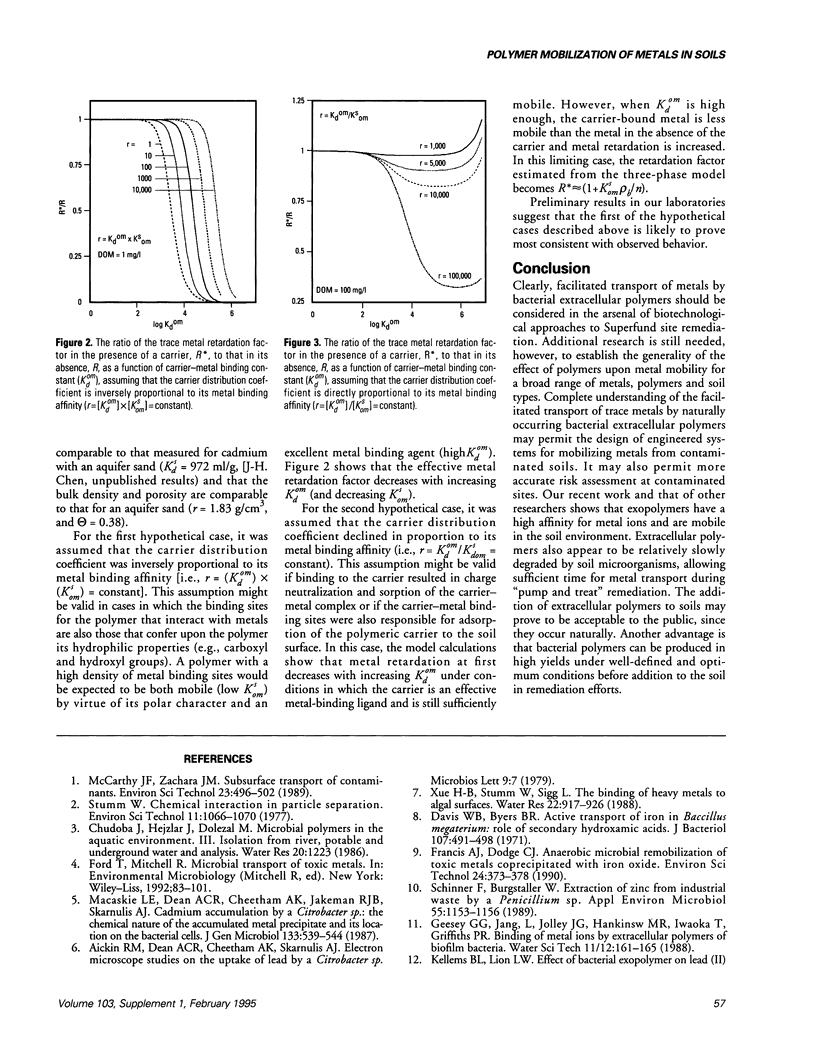
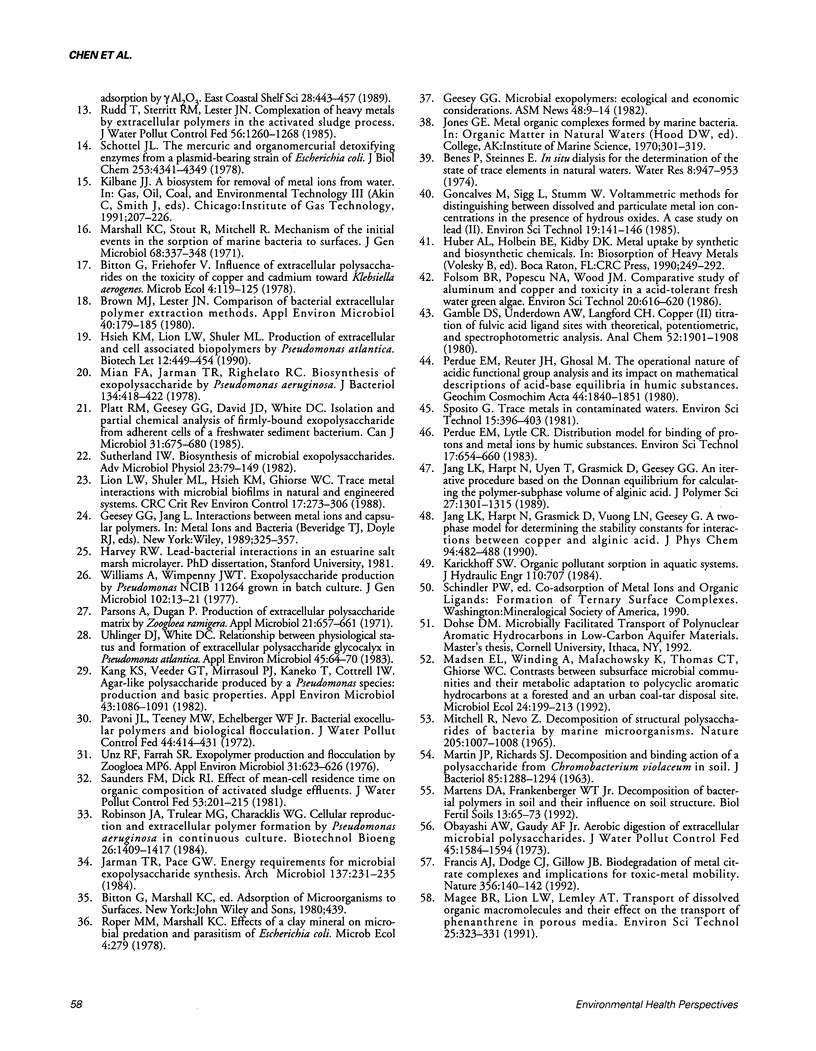
Selected References
These references are in PubMed. This may not be the complete list of references from this article.
- Brown M. J., Lester J. N. Comparison of bacterial extracellular polymer extraction methods. Appl Environ Microbiol. 1980 Aug;40(2):179–185. doi: 10.1128/aem.40.2.179-185.1980. [DOI] [PMC free article] [PubMed] [Google Scholar]
- Davis W. B., Byers B. R. Active transport of iron in Bacillus megaterium: role of secondary hydroxamic acids. J Bacteriol. 1971 Aug;107(2):491–498. doi: 10.1128/jb.107.2.491-498.1971. [DOI] [PMC free article] [PubMed] [Google Scholar]
- Kang K. S., Veeder G. T., Mirrasoul P. J., Kaneko T., Cottrell I. W. Agar-like polysaccharide produced by a pseudomonas species: production and basic properties. Appl Environ Microbiol. 1982 May;43(5):1086–1091. doi: 10.1128/aem.43.5.1086-1091.1982. [DOI] [PMC free article] [PubMed] [Google Scholar]
- MARTIN J. P., RICHARDS S. J. DECOMPOSITION AND BINDING ACTION OF A POLYSACCHARIDE FROM CHROMOBACTERIUM VIOLACIUM IN SOIL. J Bacteriol. 1963 Jun;85:1288–1294. doi: 10.1128/jb.85.6.1288-1294.1963. [DOI] [PMC free article] [PubMed] [Google Scholar]
- Mian F. A., Jarman T. R., Righelato R. C. Biosynthesis of exopolysaccharide by Pseudomonas aeruginosa. J Bacteriol. 1978 May;134(2):418–422. doi: 10.1128/jb.134.2.418-422.1978. [DOI] [PMC free article] [PubMed] [Google Scholar]
- Parsons A. B., Dugan P. R. Production of extracellular polysaccharide matrix by Zoogloea ramigera. Appl Microbiol. 1971 Apr;21(4):657–661. doi: 10.1128/am.21.4.657-661.1971. [DOI] [PMC free article] [PubMed] [Google Scholar]
- Pavoni J. L., Tenney M. W., Echelberger W. F., Jr Bacterial exocellular polymers and biological flocculation. J Water Pollut Control Fed. 1972 Mar;44(3):414–429. [PubMed] [Google Scholar]
- Platt R. M., Geesey G. G., Davis J. D., White D. C. Isolation and partial chemical analysis of firmly bound exopolysaccharide from adherent cells of a freshwater sediment bacterium. Can J Microbiol. 1985;31:675–680. doi: 10.1139/m85-128. [DOI] [PubMed] [Google Scholar]
- Schinner F., Burgstaller W. Extraction of Zinc from Industrial Waste by a Penicillium sp. Appl Environ Microbiol. 1989 May;55(5):1153–1156. doi: 10.1128/aem.55.5.1153-1156.1989. [DOI] [PMC free article] [PubMed] [Google Scholar]
- Schottel J. L. The mercuric and organomercurial detoxifying enzymes from a plasmid-bearing strain of Escherichia coli. J Biol Chem. 1978 Jun 25;253(12):4341–4349. [PubMed] [Google Scholar]
- Sutherland I. W. Biosynthesis of microbial exopolysaccharides. Adv Microb Physiol. 1982;23:79–150. doi: 10.1016/s0065-2911(08)60336-7. [DOI] [PubMed] [Google Scholar]
- Uhlinger D. J., White D. C. Relationship Between Physiological Status and Formation of Extracellular Polysaccharide Glycocalyx in Pseudomonas atlantica. Appl Environ Microbiol. 1983 Jan;45(1):64–70. doi: 10.1128/aem.45.1.64-70.1983. [DOI] [PMC free article] [PubMed] [Google Scholar]
- Unz R. F., Farrah S. R. Exopolymer production and flocculation by zoogloea mp6. Appl Environ Microbiol. 1976 Apr;31(4):623–626. doi: 10.1128/aem.31.4.623-626.1976. [DOI] [PMC free article] [PubMed] [Google Scholar]
- Williams A. G., Wimpenny J. W. Exopolysaccharide production by Pseudomonas NCIB11264 grown in batch culture. J Gen Microbiol. 1977 Sep;102(1):13–21. doi: 10.1099/00221287-102-1-13. [DOI] [PubMed] [Google Scholar]