Abstract
Organophosphate (OP) exposure can be lethal at high doses while lower doses may impair performance of critical tasks. The ability to predict such effects for realistic exposure scenarios would greatly improve OP risk assessment. To this end, a physiologically based model for diisopropylfluorophosphate (DFP) pharmacokinetics and acetylcholinesterase (AChE) inhibition was developed. DFP tissue/blood partition coefficients, rates of DFP hydrolysis by esterases, and DFP-esterase bimolecular inhibition rate constants were determined in rat tissue homogenates. Other model parameters were scaled for rats and mice using standard allometric relationships. These DFP-specific parameter values were used with the model to simulate pharmacokinetic data from mice and rats. Literature data were used for model validation. DFP concentrations in mouse plasma and brain, as well as AChE inhibition and AChE resynthesis data, were successfully simulated for a single iv injection. Effects of repeated, subcutaneous DFP dosing on AChE activity in rat plasma and brain were also well simulated except for an apparent decrease in basal AChE activity in the brain which persisted 35 days after the last dose. The psychologically based pharmacokinetic (PBPK) model parameter values specific for DFP in humans, for example, tissue/blood partition coefficients, enzymatic and nonenzymatic DFP hydrolysis rates, and bimolecular inhibition rate constants for target enzymes were scaled from rodent data or obtained from the literature. Good agreement was obtained between model predictions and human exposure data on the inhibition of red blood cell AChE and plasma butyrylcholinesterase after an intramuscular injection of 33 micrograms/kg DFP and at 24 hr after acute doses of DFP (10-54 micrograms/kg), as well as for repeated DFP exposures.(ABSTRACT TRUNCATED AT 250 WORDS)
Full text
PDF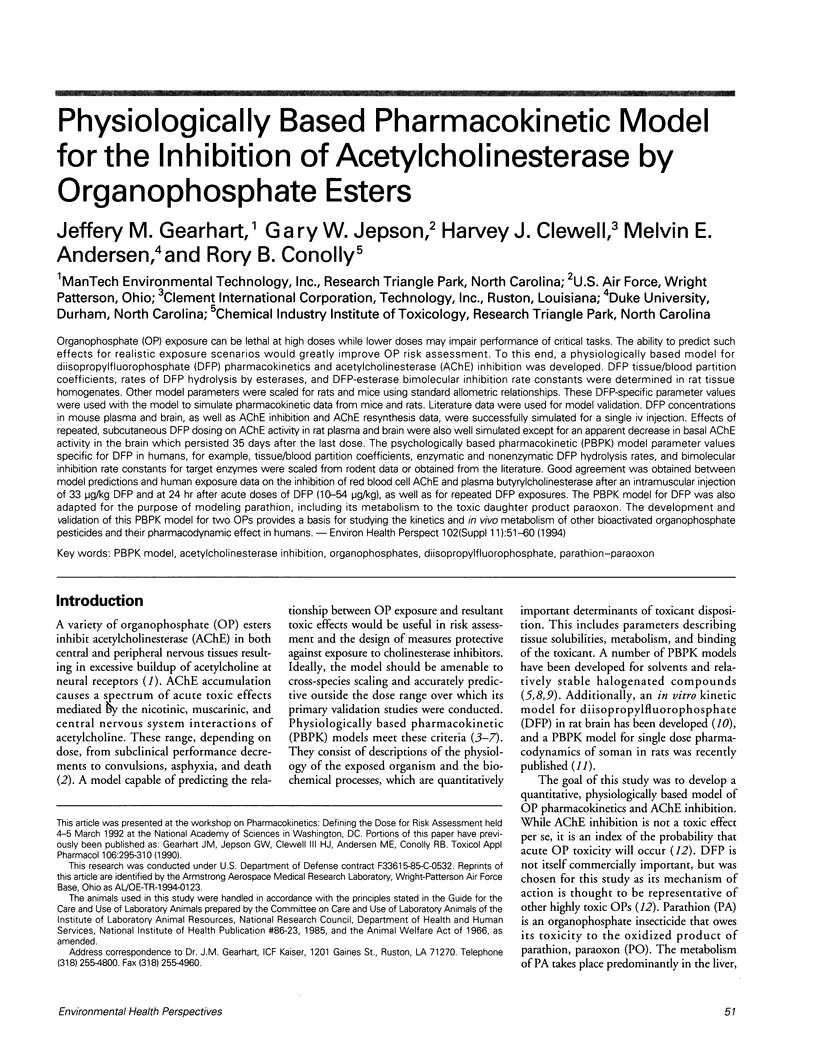
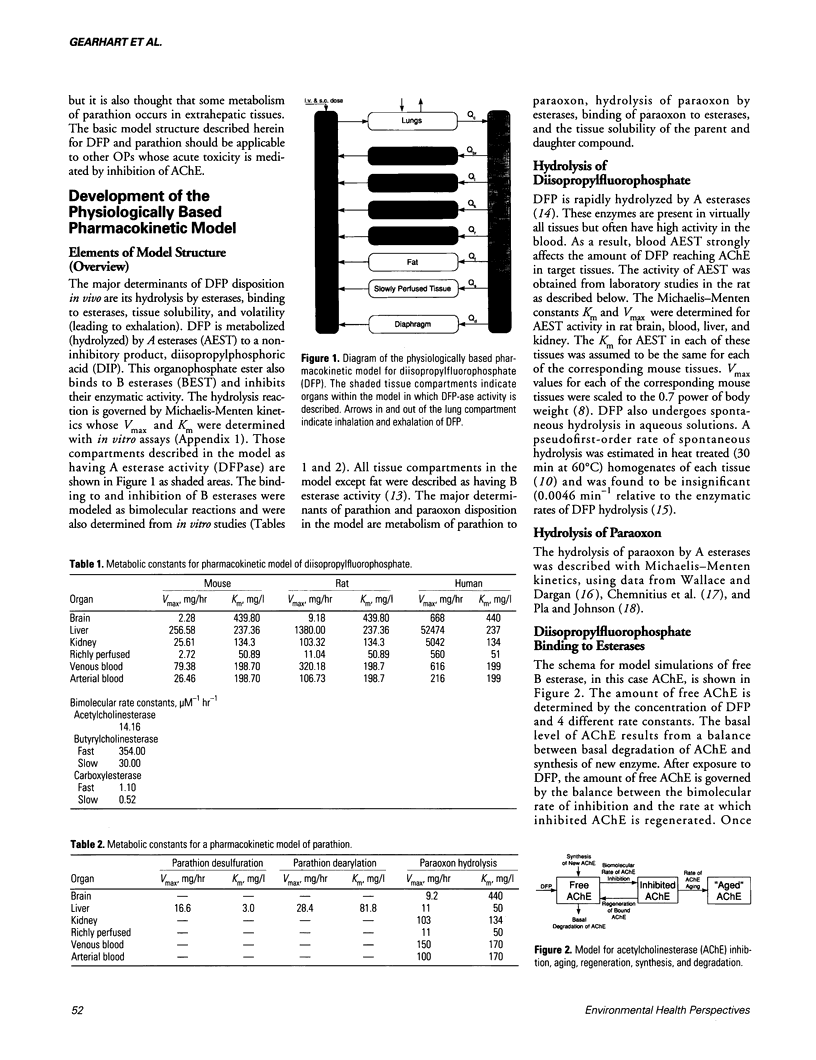
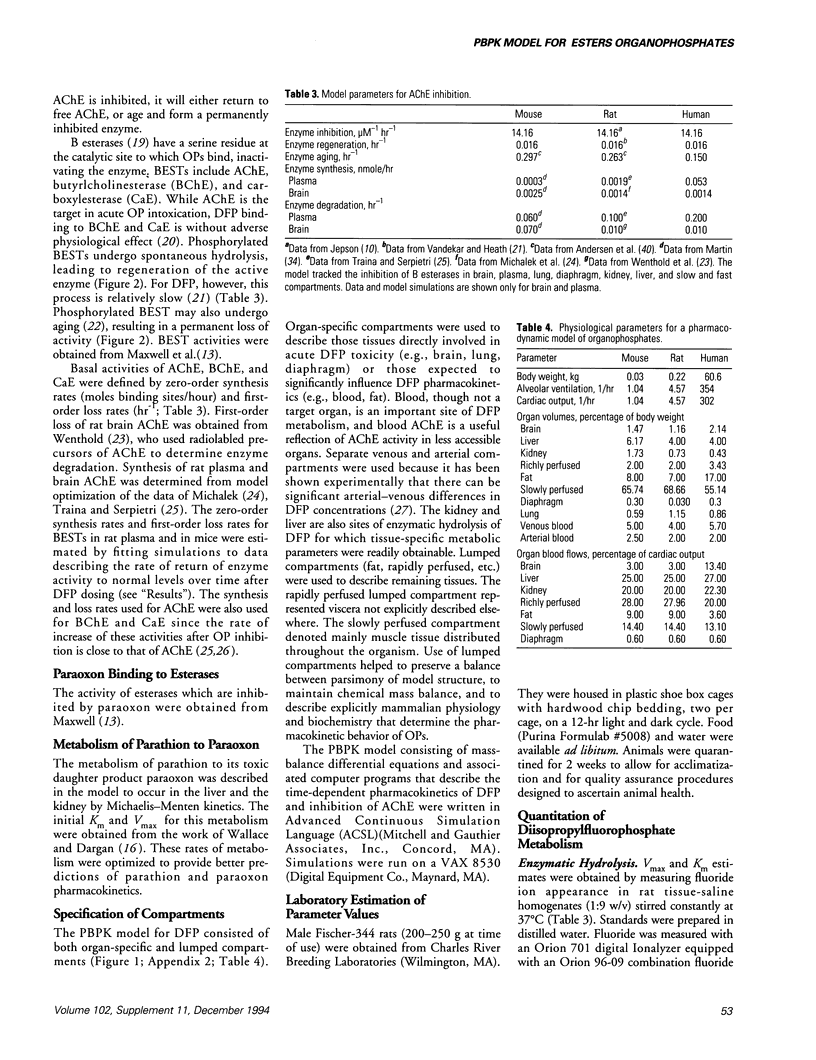
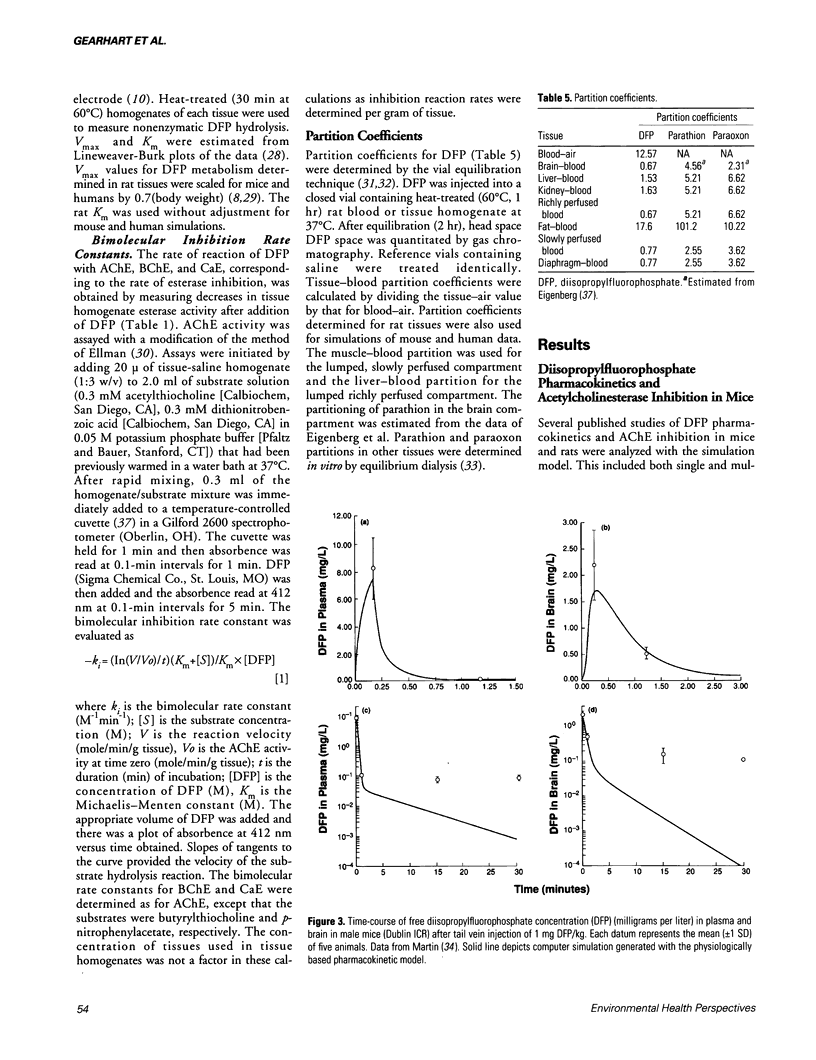
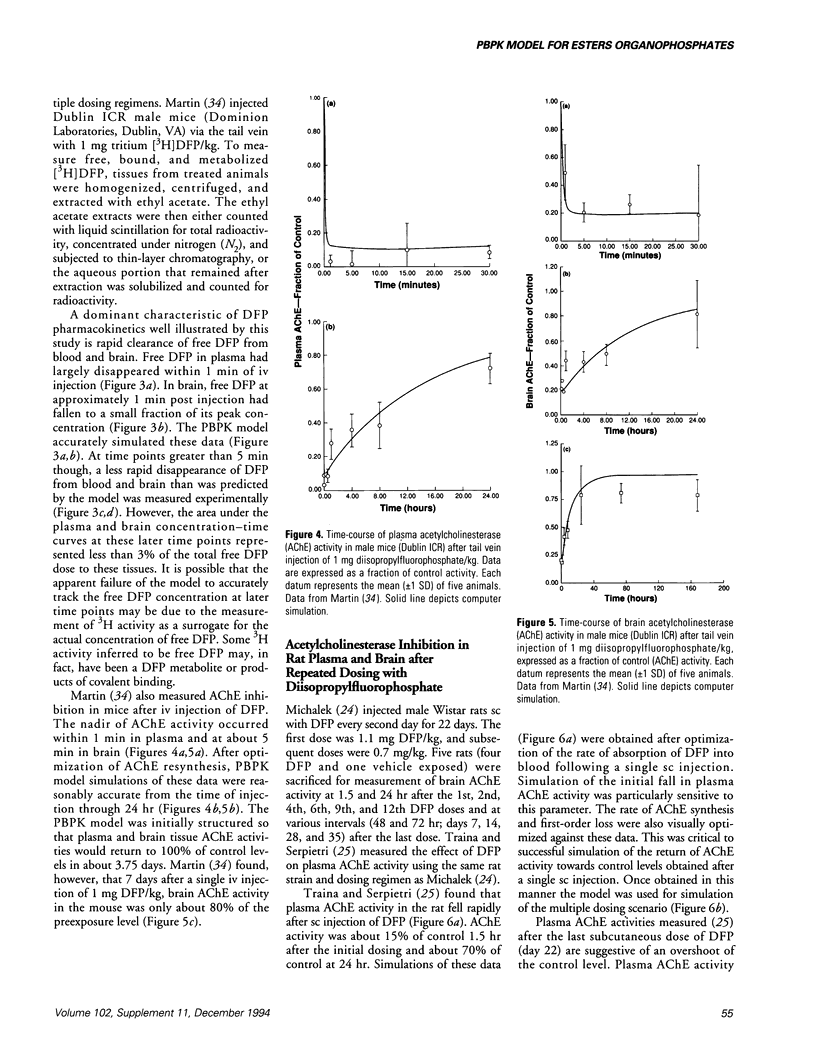
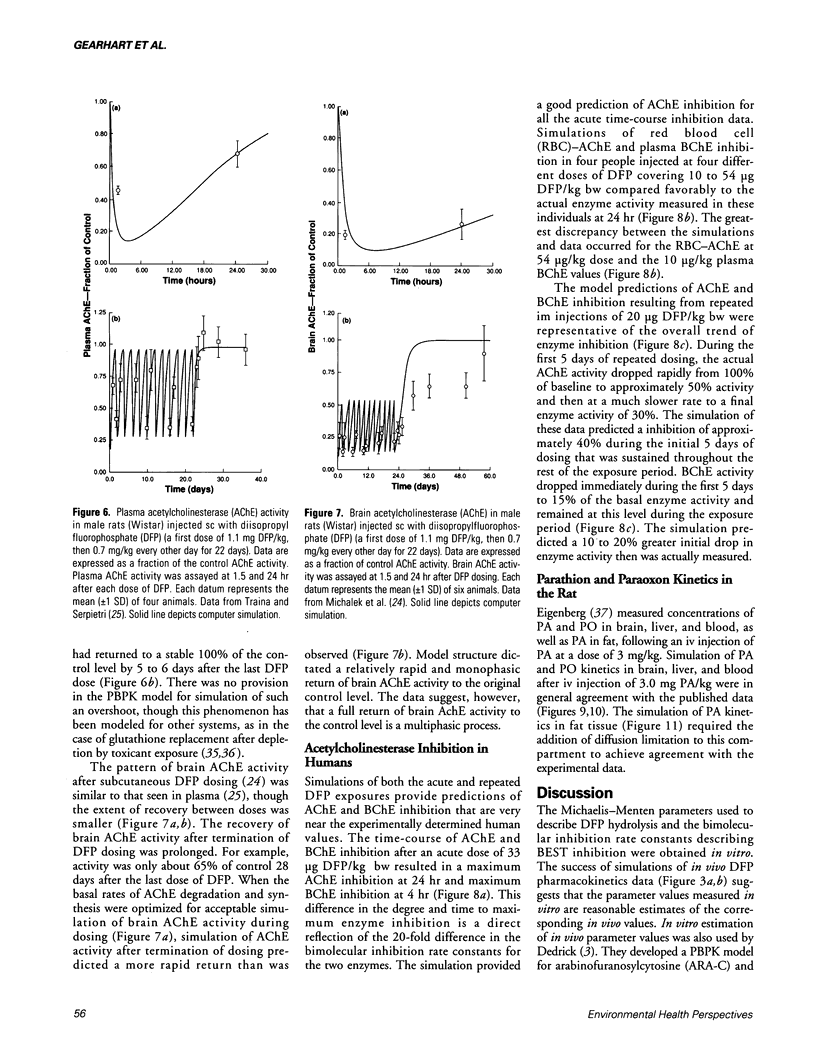
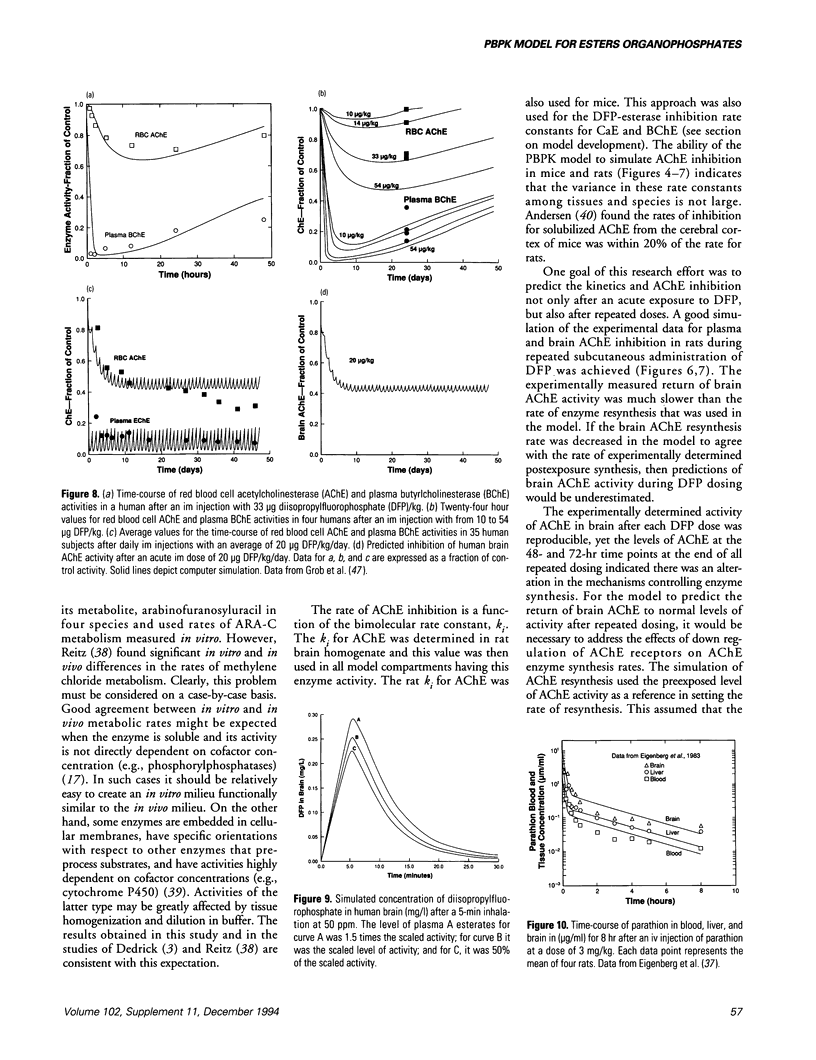
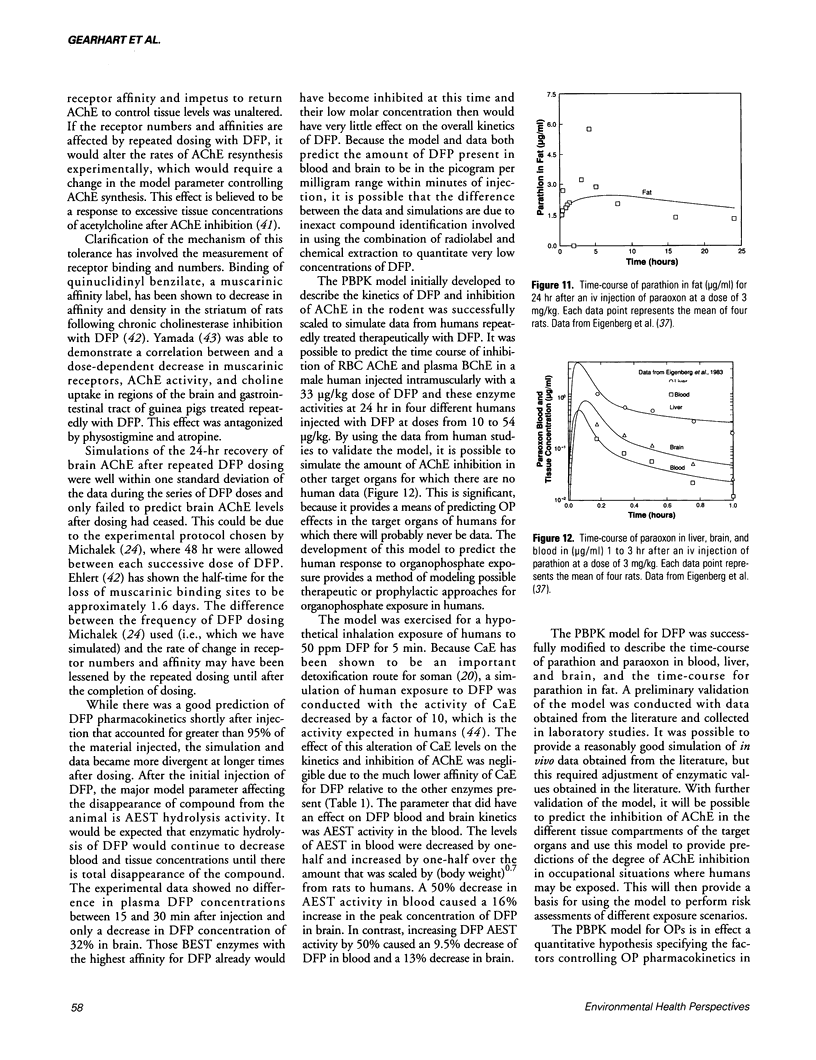
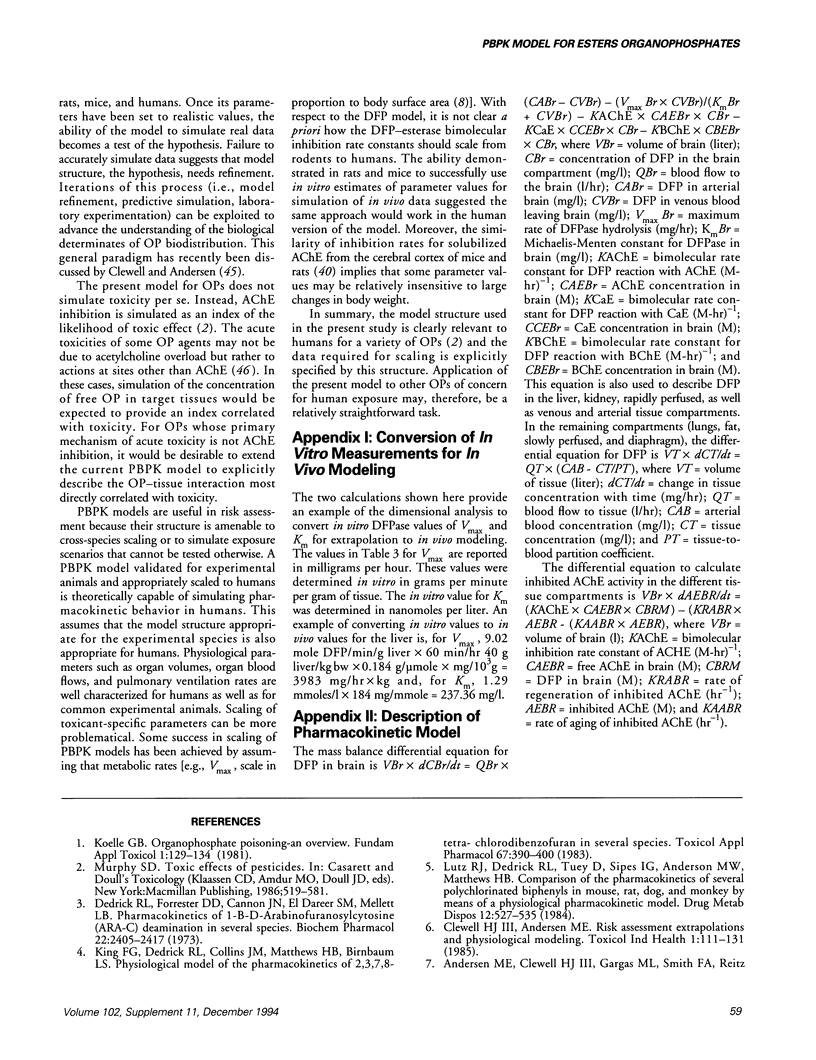
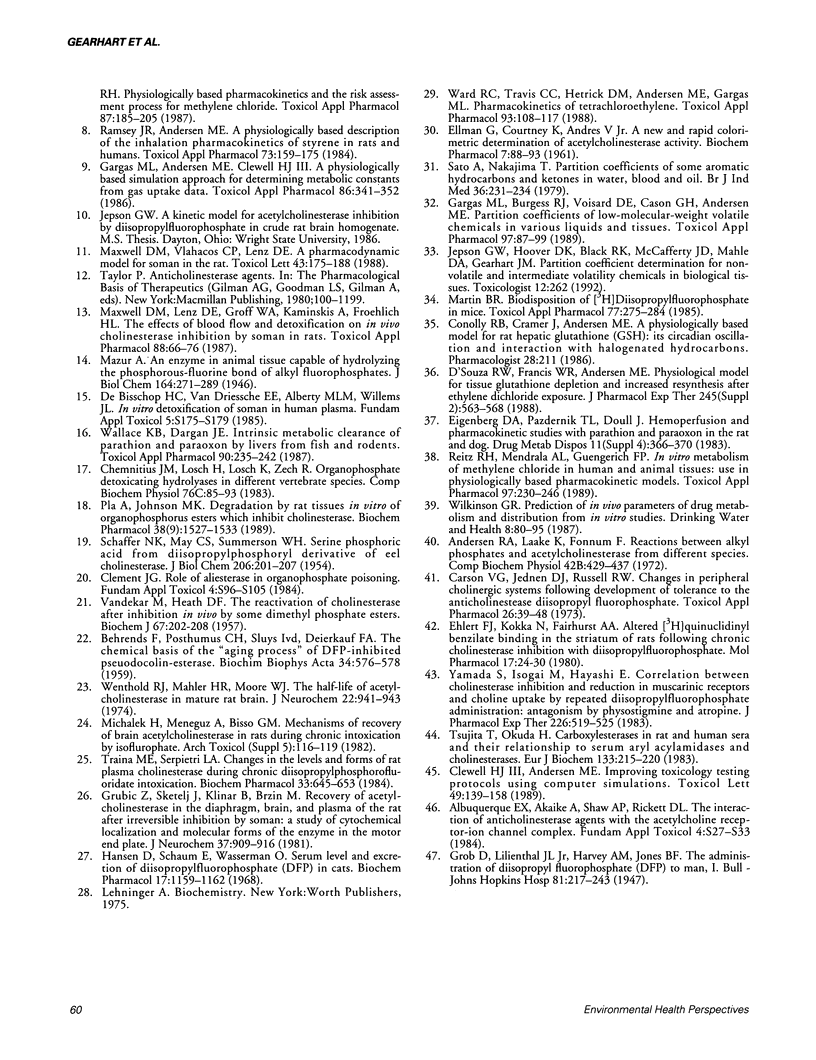
Selected References
These references are in PubMed. This may not be the complete list of references from this article.
- Albuquerque E. X., Akaike A., Shaw K. P., Rickett D. L. The interaction of anticholinesterase agents with the acetylcholine receptor-ionic channel complex. Fundam Appl Toxicol. 1984 Apr;4(2 Pt 2):S27–S33. doi: 10.1016/0272-0590(84)90135-0. [DOI] [PubMed] [Google Scholar]
- Andersen M. E., Clewell H. J., 3rd, Gargas M. L., Smith F. A., Reitz R. H. Physiologically based pharmacokinetics and the risk assessment process for methylene chloride. Toxicol Appl Pharmacol. 1987 Feb;87(2):185–205. doi: 10.1016/0041-008x(87)90281-x. [DOI] [PubMed] [Google Scholar]
- Andersen R. A., Laake K., Fonnum F. Reactions between alkyl phosphates and acetylcholinesterase from different species. Comp Biochem Physiol B. 1972 Jul 15;42(3):429–437. doi: 10.1016/0305-0491(72)90259-3. [DOI] [PubMed] [Google Scholar]
- Carson V. G., Jenden D. J., Russell R. W. Changes in peripheral cholinergic systems following development of tolerance to the anticholinesterase diisopropylfluorophosphate. Toxicol Appl Pharmacol. 1973 Sep;26(1):39–48. doi: 10.1016/0041-008x(73)90083-5. [DOI] [PubMed] [Google Scholar]
- Chemnitius J. M., Losch H., Losch K., Zech R. Organophosphate detoxicating hydrolases in different vertebrate species. Comp Biochem Physiol C. 1983;76(1):85–93. doi: 10.1016/0742-8413(83)90048-8. [DOI] [PubMed] [Google Scholar]
- Clement J. G. Role of aliesterase in organophosphate poisoning. Fundam Appl Toxicol. 1984 Apr;4(2 Pt 2):S96–105. doi: 10.1016/0272-0590(84)90141-6. [DOI] [PubMed] [Google Scholar]
- Clewell H. J., 3rd, Andersen M. E. Improving toxicology testing protocols using computer simulations. Toxicol Lett. 1989 Dec;49(2-3):139–158. doi: 10.1016/0378-4274(89)90029-5. [DOI] [PubMed] [Google Scholar]
- Clewell H. J., 3rd, Andersen M. E. Risk assessment extrapolations and physiological modeling. Toxicol Ind Health. 1985 Dec;1(4):111–131. doi: 10.1177/074823378500100408. [DOI] [PubMed] [Google Scholar]
- D'Souza R. W., Francis W. R., Andersen M. E. Physiological model for tissue glutathione depletion and increased resynthesis after ethylene dichloride exposure. J Pharmacol Exp Ther. 1988 May;245(2):563–568. [PubMed] [Google Scholar]
- De Bisschop H. C., Van Driessche E. E., Alberty M. L., Willems J. L. In vitro detoxification of soman in human plasma. Fundam Appl Toxicol. 1985 Dec;5(6 Pt 2):S175–S179. [PubMed] [Google Scholar]
- Dedrick R. L., Forrester D. D., Cannon J. N., el-Dareer S. M., Mellett L. B. Pharmacokinetics of 1-beta-D-arabinofuranosylcytosine (ARA-C) deamination in several species. Biochem Pharmacol. 1973 Oct 1;22(19):2405–2417. doi: 10.1016/0006-2952(73)90342-0. [DOI] [PubMed] [Google Scholar]
- ELLMAN G. L., COURTNEY K. D., ANDRES V., Jr, FEATHER-STONE R. M. A new and rapid colorimetric determination of acetylcholinesterase activity. Biochem Pharmacol. 1961 Jul;7:88–95. doi: 10.1016/0006-2952(61)90145-9. [DOI] [PubMed] [Google Scholar]
- Ehlert F. J., Kokka N., Fairhurst A. S. Altered [3H]quinuclidinyl benzilate binding in the striatum of rats following chronic cholinesterase inhibition with diisopropylfluorophosphate. Mol Pharmacol. 1980 Jan;17(1):24–30. [PubMed] [Google Scholar]
- Eigenberg D. A., Pazdernik T. L., Doull J. Hemoperfusion and pharmacokinetic studies with parathion and paraoxon in the rat and dog. Drug Metab Dispos. 1983 Jul-Aug;11(4):366–370. [PubMed] [Google Scholar]
- Gargas M. L., Andersen M. E., Clewell H. J., 3rd A physiologically based simulation approach for determining metabolic constants from gas uptake data. Toxicol Appl Pharmacol. 1986 Dec;86(3):341–352. doi: 10.1016/0041-008x(86)90361-3. [DOI] [PubMed] [Google Scholar]
- Gargas M. L., Burgess R. J., Voisard D. E., Cason G. H., Andersen M. E. Partition coefficients of low-molecular-weight volatile chemicals in various liquids and tissues. Toxicol Appl Pharmacol. 1989 Mar 15;98(1):87–99. doi: 10.1016/0041-008x(89)90137-3. [DOI] [PubMed] [Google Scholar]
- Grubić Z., Sketelj J., Klinar B., Brzin M. Recovery of acetylcholinesterase in the diaphragm, brain, and plasma of the rat after irreversible inhibition by soman: a study of cytochemical localization and molecular forms of the enzyme in the motor end plate. J Neurochem. 1981 Oct;37(4):909–916. doi: 10.1111/j.1471-4159.1981.tb04478.x. [DOI] [PubMed] [Google Scholar]
- Hansen D., Schaum E., Wassermann O. Serum level and excretion of diisopropylfluorophosphate (DFP) in cats. Biochem Pharmacol. 1968 Jul;17(7):1159–1162. doi: 10.1016/0006-2952(68)90051-8. [DOI] [PubMed] [Google Scholar]
- King F. G., Dedrick R. L., Collins J. M., Matthews H. B., Birnbaum L. S. Physiological model for the pharmacokinetics of 2,3,7,8-tetrachlorodibenzofuran in several species. Toxicol Appl Pharmacol. 1983 Mar 15;67(3):390–400. doi: 10.1016/0041-008x(83)90323-x. [DOI] [PubMed] [Google Scholar]
- Koelle G. B. Organophosphate poisoning--an overview. Fundam Appl Toxicol. 1981 Mar-Apr;1(2):129–134. [PubMed] [Google Scholar]
- Lutz R. J., Dedrick R. L., Tuey D., Sipes I. G., Anderson M. W., Matthews H. B. Comparison of the pharmacokinetics of several polychlorinated biphenyls in mouse, rat, dog, and monkey by means of a physiological pharmacokinetic model. Drug Metab Dispos. 1984 Sep-Oct;12(5):527–535. [PubMed] [Google Scholar]
- Martin B. R. Biodisposition of [3H]diisopropylfluorophosphate in mice. Toxicol Appl Pharmacol. 1985 Feb;77(2):275–284. doi: 10.1016/0041-008x(85)90327-8. [DOI] [PubMed] [Google Scholar]
- Maxwell D. M., Lenz D. E., Groff W. A., Kaminskis A., Froehlich H. L. The effects of blood flow and detoxification on in vivo cholinesterase inhibition by soman in rats. Toxicol Appl Pharmacol. 1987 Mar 30;88(1):66–76. doi: 10.1016/0041-008x(87)90270-5. [DOI] [PubMed] [Google Scholar]
- Maxwell D. M., Vlahacos C. P., Lenz D. E. A pharmacodynamic model for soman in the rat. Toxicol Lett. 1988 Oct;43(1-3):175–188. doi: 10.1016/0378-4274(88)90027-6. [DOI] [PubMed] [Google Scholar]
- Michalek H., Meneguz A., Bisso G. M. Mechanisms of recovery of brain acetylcholinesterase in rats during chronic intoxication by isoflurophate. Arch Toxicol Suppl. 1982;5:116–119. doi: 10.1007/978-3-642-68511-8_21. [DOI] [PubMed] [Google Scholar]
- Pla A., Johnson M. K. Degradation by rat tissues in vitro of organophosphorus esters which inhibit cholinesterase. Biochem Pharmacol. 1989 May 1;38(9):1527–1533. doi: 10.1016/0006-2952(89)90193-7. [DOI] [PubMed] [Google Scholar]
- Ramsey J. C., Andersen M. E. A physiologically based description of the inhalation pharmacokinetics of styrene in rats and humans. Toxicol Appl Pharmacol. 1984 Mar 30;73(1):159–175. doi: 10.1016/0041-008x(84)90064-4. [DOI] [PubMed] [Google Scholar]
- Reitz R. H., Mendrala A. L., Guengerich F. P. In vitro metabolism of methylene chloride in human and animal tissues: use in physiologically based pharmacokinetic models. Toxicol Appl Pharmacol. 1989 Feb;97(2):230–246. doi: 10.1016/0041-008x(89)90328-1. [DOI] [PubMed] [Google Scholar]
- SCHAFFER N. K., MAY S. C., Jr, SUMMERSON W. H. Serine phosphoric acid from diisopropylphosphoryl derivative of eel cholinesterase. J Biol Chem. 1954 Jan;206(1):201–207. [PubMed] [Google Scholar]
- Sato A., Nakajima T. Partition coefficients of some aromatic hydrocarbons and ketones in water, blood and oil. Br J Ind Med. 1979 Aug;36(3):231–234. doi: 10.1136/oem.36.3.231. [DOI] [PMC free article] [PubMed] [Google Scholar]
- Traina M. E., Serpietri L. A. Changes in the levels and forms of rat plasma cholinesterases during chronic diisopropylphosphorofluoridate intoxication. Biochem Pharmacol. 1984 Feb 15;33(4):645–653. doi: 10.1016/0006-2952(84)90321-6. [DOI] [PubMed] [Google Scholar]
- Tsujita T., Okuda H. Carboxylesterases in rat and human sera and their relationship of serum aryl acylamidases and cholinesterases. Eur J Biochem. 1983 Jun 1;133(1):215–220. doi: 10.1111/j.1432-1033.1983.tb07450.x. [DOI] [PubMed] [Google Scholar]
- VANDEKAR M., HEATH D. F. The reactivation of cholinesterase after Inhibition in vivo by some dimethyl phosphate esters. Biochem J. 1957 Oct;67(2):202–208. doi: 10.1042/bj0670202. [DOI] [PMC free article] [PubMed] [Google Scholar]
- Wallace K. B., Dargan J. E. Intrinsic metabolic clearance of parathion and paraoxon by livers from fish and rodents. Toxicol Appl Pharmacol. 1987 Sep 15;90(2):235–242. doi: 10.1016/0041-008x(87)90331-0. [DOI] [PubMed] [Google Scholar]
- Ward R. C., Travis C. C., Hetrick D. M., Andersen M. E., Gargas M. L. Pharmacokinetics of tetrachloroethylene. Toxicol Appl Pharmacol. 1988 Mar 30;93(1):108–117. doi: 10.1016/0041-008x(88)90030-0. [DOI] [PubMed] [Google Scholar]
- Wenthold R. J., Mahler H. R., Moore W. J. The half-life of acetylcholinesterase in mature rat brain. J Neurochem. 1974 Jun;22(6):941–943. doi: 10.1111/j.1471-4159.1974.tb04319.x. [DOI] [PubMed] [Google Scholar]
- Yamada S., Isogai M., Okudaira H., Hayashi E. Correlation between cholinesterase inhibition and reduction in muscarinic receptors and choline uptake by repeated diisopropylfluorophosphate administration: antagonism by physostigmine and atropine. J Pharmacol Exp Ther. 1983 Aug;226(2):519–525. [PubMed] [Google Scholar]