Abstract
Ramoplanin, a new lipoglycopeptide antibiotic, inhibits cell wall peptidoglycan biosynthesis in gram-positive bacteria. In both Staphylococcus aureus and Bacillus megaterium, UDP-N-acetylmuramyl-pentapeptides (UDP-MurNAc-pentapeptides) accumulated at concentrations of ramoplanin close to the MIC, indicating that inhibition of peptidoglycan biosynthesis occurred after formation of cytoplasmic precursors. Susceptible bacteria bound or accumulated approximately 5 x 10(4) molecules of ramoplanin per cell, only 1/100th of the amount of vancomycin which binds to groups within peptidoglycan conforming to the pattern L-alpha alpha (amino acid)-D-alpha alpha-D-alpha alpha, suggesting that ramoplanin has a different target site. This was confirmed by in vitro studies involving a wall-membrane particulate fraction from Gaffkya homari in which peptidoglycan synthesis from UDP-MurNAc-tetrapeptide was inhibited by ramoplanin but not by vancomycin. The incorporation of peptidoglycan precursors into nascent peptidoglycan of a toluenized cell preparation of B. megaterium was inhibited by ramoplanin, indicating that the antibiotic acts at a step before transpeptidation. In vitro studies of a wall-membrane particulate fraction of B. megaterium indicated that ramoplanin did not prevent the formation of lipid intermediate I (undecaprenyl-P-P-MurNAc-pentapeptide) but inhibited the next reaction in which N-acetylglucosamine is transferred to that lipid intermediate. The high concentrations required to inhibit in vitro peptidoglycan-synthesizing systems probably reflect the high concentrations of target sites present. High concentrations of ramoplanin also damage certain properties of the cell membrane, but low concentrations only affected wall synthesis in intact bacteria without perturbing membrane function. These studies indicate that the primary target of ramoplanin is peptidoglycan biosynthesis and that the probable reaction inhibited is the N-acetylglucosaminyltransferase-catalyzed conversion of lipid intermediate I to lipid intermediate II.
Full text
PDF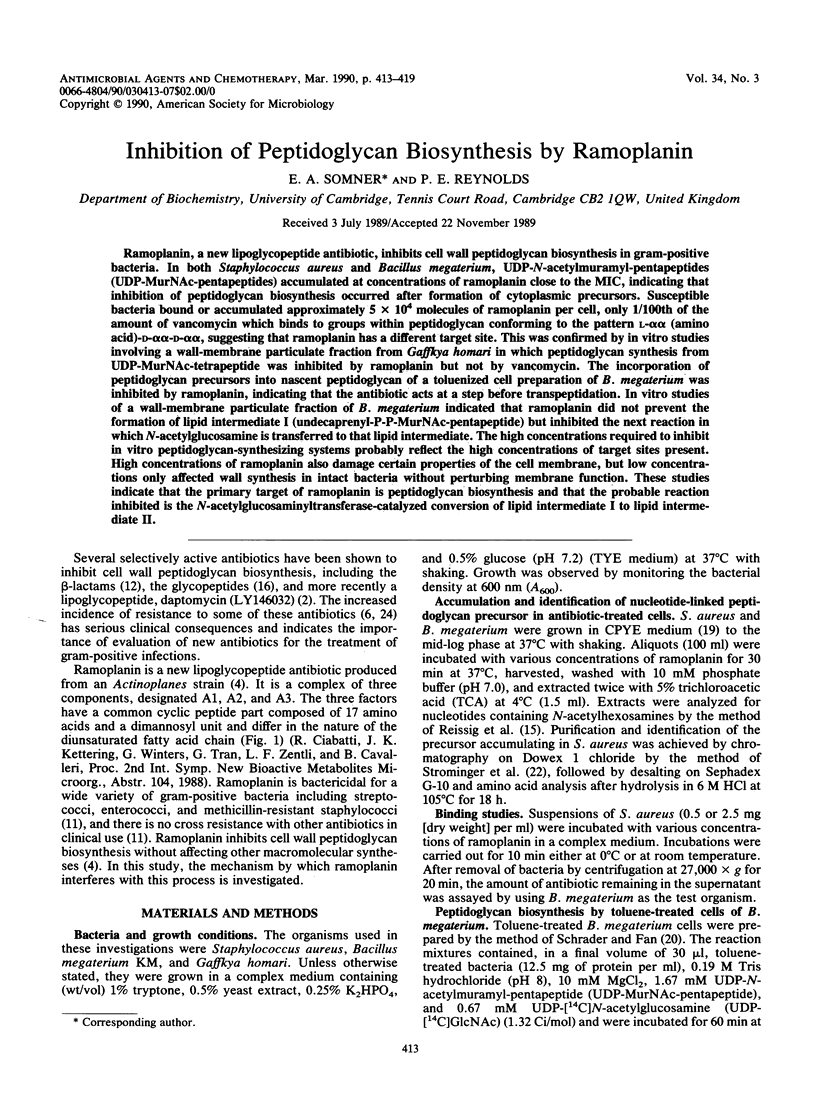
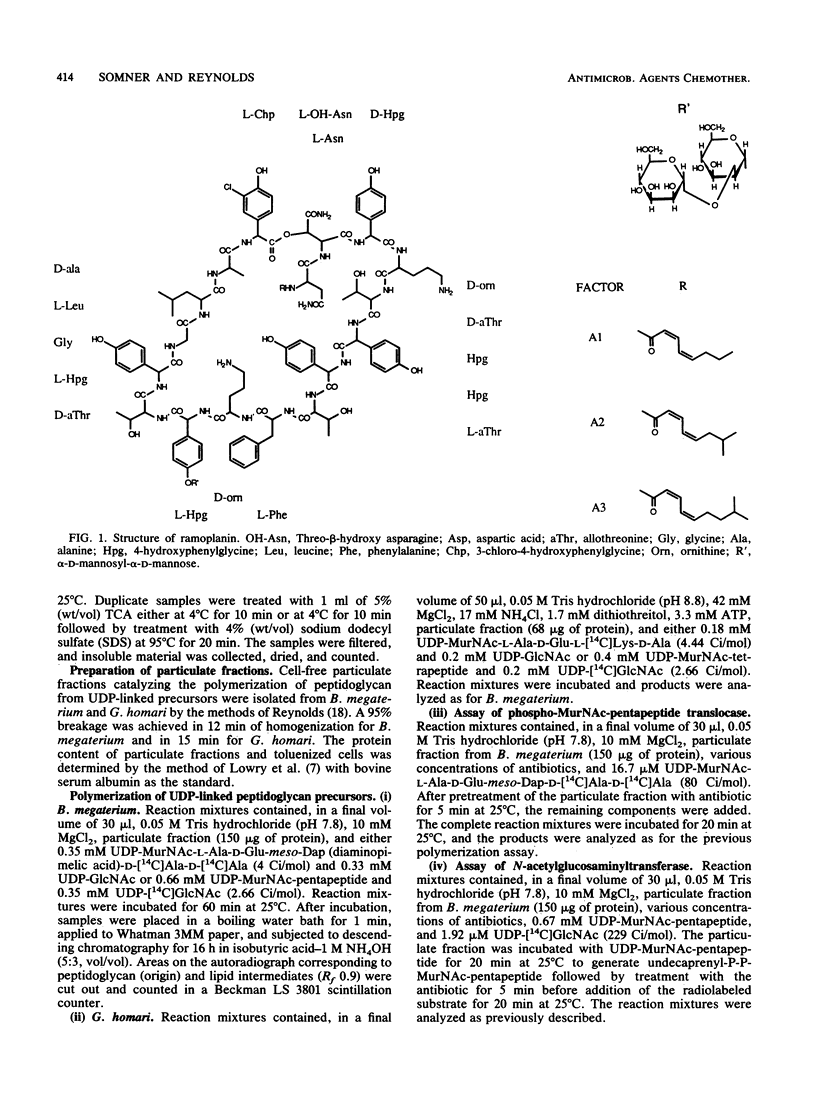
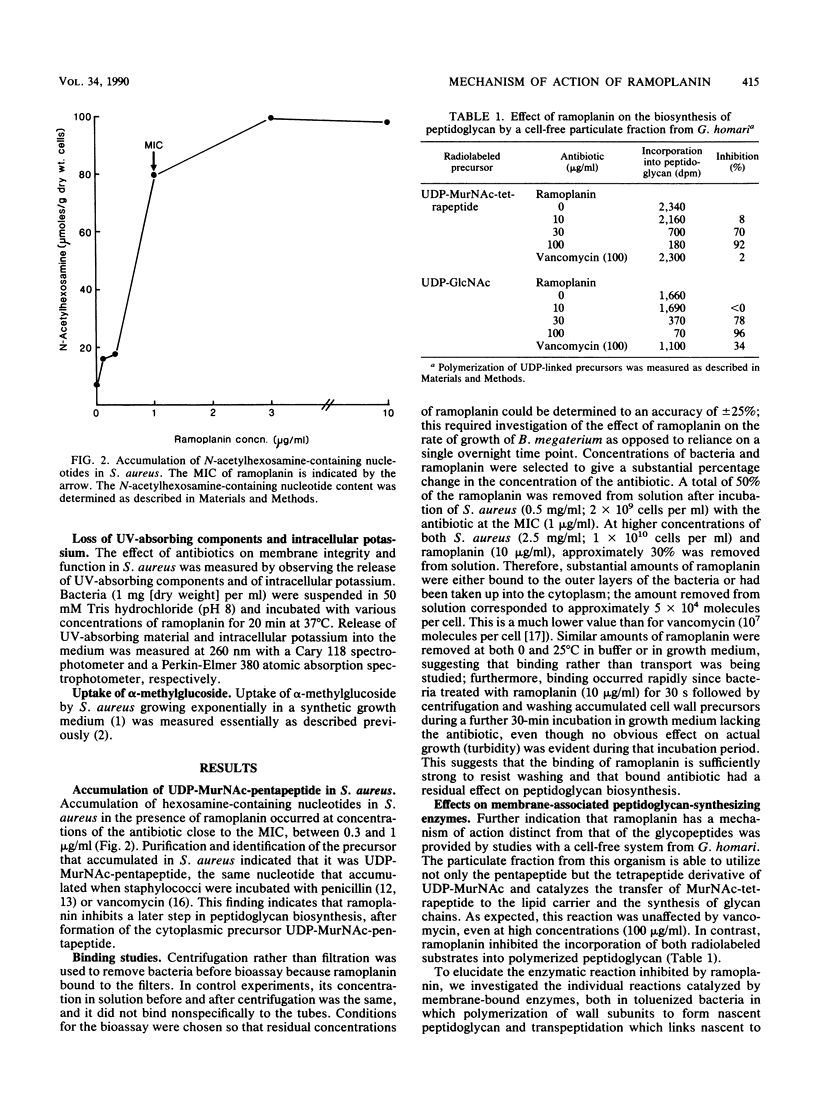
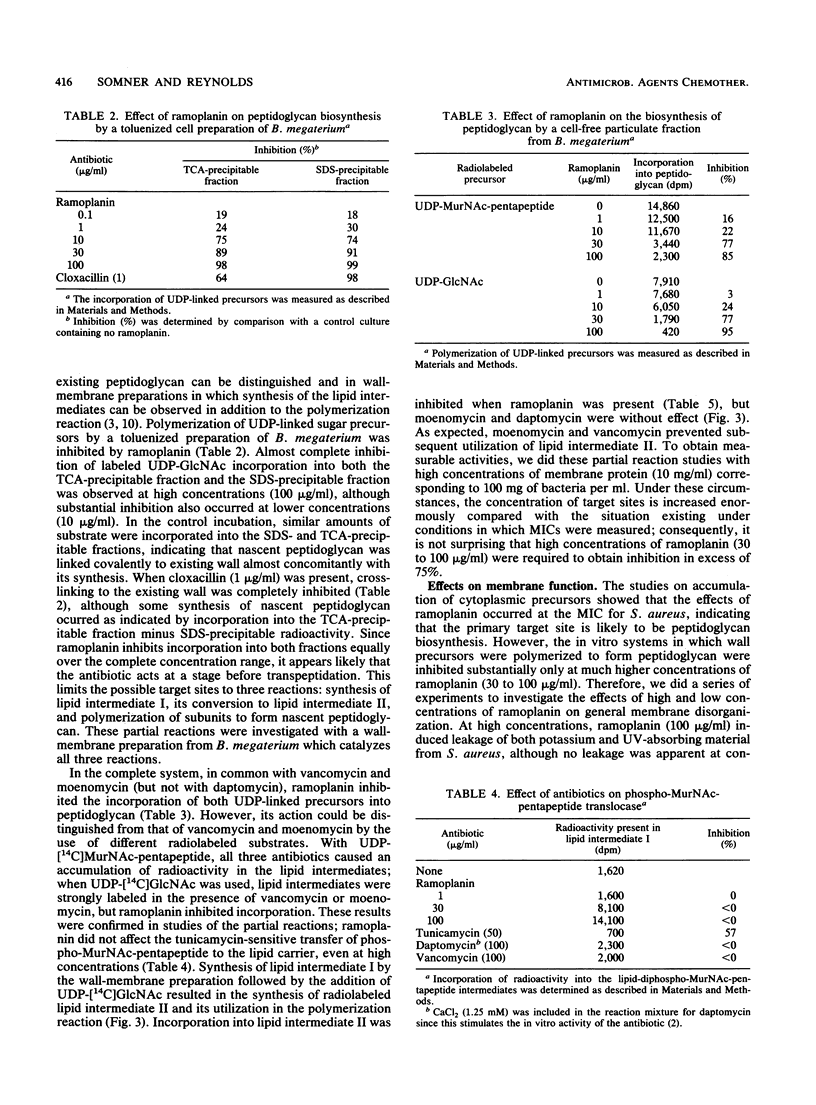
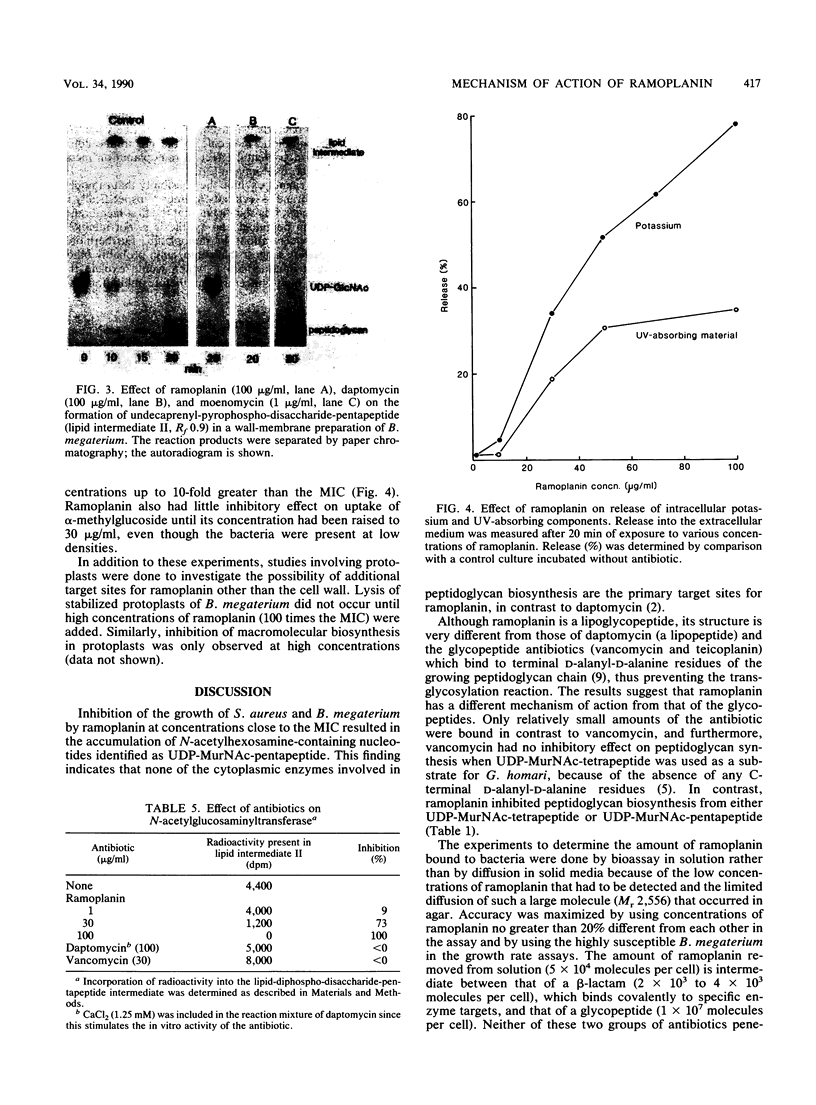
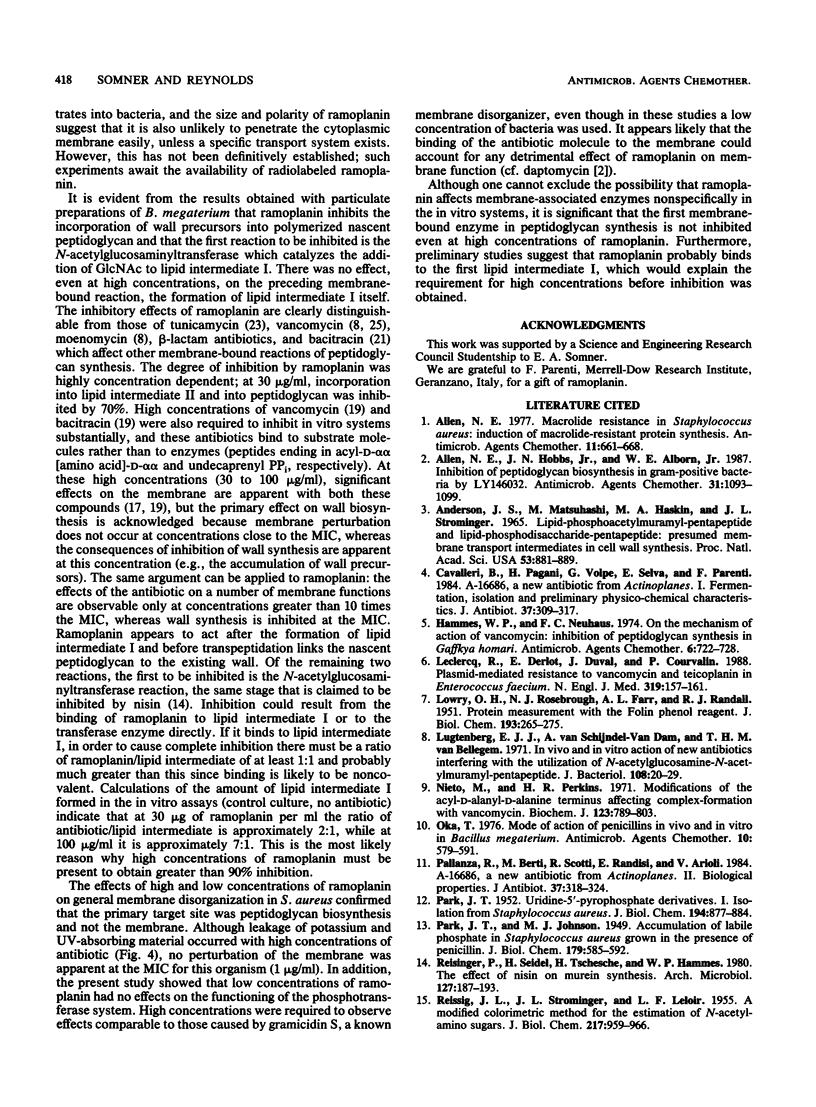
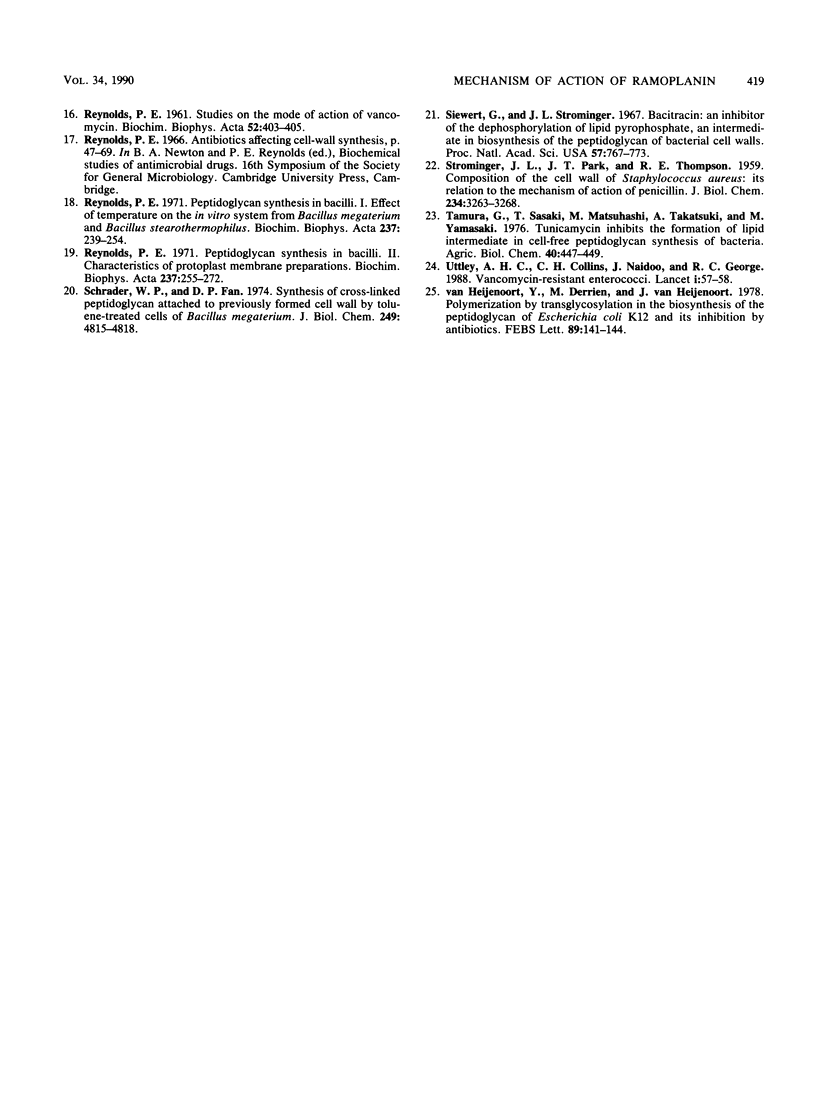
Images in this article
Selected References
These references are in PubMed. This may not be the complete list of references from this article.
- ANDERSON J. S., MATSUHASHI M., HASKIN M. A., STROMINGER J. L. LIPID-PHOSPHOACETYLMURAMYL-PENTAPEPTIDE AND LIPID-PHOSPHODISACCHARIDE-PENTAPEPTIDE: PRESUMED MEMBRANE TRANSPORT INTERMEDIATES IN CELL WALL SYNTHESIS. Proc Natl Acad Sci U S A. 1965 Apr;53:881–889. doi: 10.1073/pnas.53.4.881. [DOI] [PMC free article] [PubMed] [Google Scholar]
- Allen N. E., Hobbs J. N., Alborn W. E., Jr Inhibition of peptidoglycan biosynthesis in gram-positive bacteria by LY146032. Antimicrob Agents Chemother. 1987 Jul;31(7):1093–1099. doi: 10.1128/aac.31.7.1093. [DOI] [PMC free article] [PubMed] [Google Scholar]
- Allen N. E. Macrolide resistance in Staphylococcus aureus: induction of macrolide-resistant protein synthesis. Antimicrob Agents Chemother. 1977 Apr;11(4):661–668. doi: 10.1128/aac.11.4.661. [DOI] [PMC free article] [PubMed] [Google Scholar]
- Cavalleri B., Pagani H., Volpe G., Selva E., Parenti F. A-16686, a new antibiotic from Actinoplanes. I. Fermentation, isolation and preliminary physico-chemical characteristics. J Antibiot (Tokyo) 1984 Apr;37(4):309–317. doi: 10.7164/antibiotics.37.309. [DOI] [PubMed] [Google Scholar]
- Hammes W. P., Neuhaus F. C. On the mechanism of action of vancomycin: inhibition of peptidoglycan synthesis in Gaffkya homari. Antimicrob Agents Chemother. 1974 Dec;6(6):722–728. doi: 10.1128/aac.6.6.722. [DOI] [PMC free article] [PubMed] [Google Scholar]
- LOWRY O. H., ROSEBROUGH N. J., FARR A. L., RANDALL R. J. Protein measurement with the Folin phenol reagent. J Biol Chem. 1951 Nov;193(1):265–275. [PubMed] [Google Scholar]
- Leclercq R., Derlot E., Duval J., Courvalin P. Plasmid-mediated resistance to vancomycin and teicoplanin in Enterococcus faecium. N Engl J Med. 1988 Jul 21;319(3):157–161. doi: 10.1056/NEJM198807213190307. [DOI] [PubMed] [Google Scholar]
- Lugtenberg E. J., v Schijndel-van Dam A., van Bellegem T. H. In vivo and in vitro action of new antibiotics interfering with the utilization of N-acetyl-glucosamine-N-acetyl-muramyl-pentapeptide. J Bacteriol. 1971 Oct;108(1):20–29. doi: 10.1128/jb.108.1.20-29.1971. [DOI] [PMC free article] [PubMed] [Google Scholar]
- Nieto M., Perkins H. R. Modifications of the acyl-D-alanyl-D-alanine terminus affecting complex-formation with vancomycin. Biochem J. 1971 Aug;123(5):789–803. doi: 10.1042/bj1230789. [DOI] [PMC free article] [PubMed] [Google Scholar]
- Oka T. Mode of action of penicillins in vivo and in vitro in Bacillus megaterium. Antimicrob Agents Chemother. 1976 Oct;10(4):579–591. doi: 10.1128/aac.10.4.579. [DOI] [PMC free article] [PubMed] [Google Scholar]
- PARK J. T. Uridine-5'-pyrophosphate derivatives. II. Isolation from Staphylococcus aureus. J Biol Chem. 1952 Feb;194(2):877–884. [PubMed] [Google Scholar]
- Pallanza R., Berti M., Scotti R., Randisi E., Arioli V. A-16686, a new antibiotic from Actinoplanes. II. Biological properties. J Antibiot (Tokyo) 1984 Apr;37(4):318–324. doi: 10.7164/antibiotics.37.318. [DOI] [PubMed] [Google Scholar]
- REISSIG J. L., STORMINGER J. L., LELOIR L. F. A modified colorimetric method for the estimation of N-acetylamino sugars. J Biol Chem. 1955 Dec;217(2):959–966. [PubMed] [Google Scholar]
- REYNOLDS P. E. Studies on the mode of action of vancomycin. Biochim Biophys Acta. 1961 Sep 16;52:403–405. doi: 10.1016/0006-3002(61)90698-9. [DOI] [PubMed] [Google Scholar]
- Reisinger P., Seidel H., Tschesche H., Hammes W. P. The effect of nisin on murein synthesis. Arch Microbiol. 1980 Oct;127(3):187–193. doi: 10.1007/BF00427192. [DOI] [PubMed] [Google Scholar]
- Reynolds P. E. Peptidoglycan synthesis in bacilli. I. Effect of temperature on the in vitro system from Bacillus megaterium and Bacillus stearothermophilus. Biochim Biophys Acta. 1971 May 18;237(2):239–254. [PubMed] [Google Scholar]
- Reynolds P. E. Peptidoglycan synthesis in bacilli. II. Characteristics of protoplast membrane preparations. Biochim Biophys Acta. 1971 May 18;237(2):255–272. doi: 10.1016/0304-4165(71)90316-3. [DOI] [PubMed] [Google Scholar]
- STROMINGER J. L., PARK J. T., THOMPSON R. E. Composition of the cell wall of Staphylococcus aureus: its relation to the mechanism of action of penicillin. J Biol Chem. 1959 Dec;234:3263–3268. [PubMed] [Google Scholar]
- Schrader W. P., Fan D. P. Synthesis of cross-linked peptidoglycan attached to previously formed cell wall by toluene-treated cells of Bacillus megaterium. J Biol Chem. 1974 Aug 10;249(15):4815–4818. [PubMed] [Google Scholar]
- Siewert G., Strominger J. L. Bacitracin: an inhibitor of the dephosphorylation of lipid pyrophosphate, an intermediate in the biosynthesis of the peptidoglycan of bacterial cell walls. Proc Natl Acad Sci U S A. 1967 Mar;57(3):767–773. doi: 10.1073/pnas.57.3.767. [DOI] [PMC free article] [PubMed] [Google Scholar]
- Uttley A. H., Collins C. H., Naidoo J., George R. C. Vancomycin-resistant enterococci. Lancet. 1988 Jan 2;1(8575-6):57–58. doi: 10.1016/s0140-6736(88)91037-9. [DOI] [PubMed] [Google Scholar]
- Van Heijenoort Y., Derrien M., Van Heijenoort J. Polymerization by transglycosylation in the biosynthesis of the peptidoglycan of Escherichia coli K 12 and its inhibition by antibiotics. FEBS Lett. 1978 May 1;89(1):141–144. doi: 10.1016/0014-5793(78)80540-7. [DOI] [PubMed] [Google Scholar]