Abstract
Mucor racemosus cells adapted to either cycloheximide or trichodermin were approximately 40-fold more resistant to cycloheximide than nonadapted cells. Ribosomes isolated from adapted and nonadapted cells were equally sensitive to cycloheximide in an in vitro poly(U) translation assay. There was no detectable modification of cycloheximide by adapted cells. Uptake of drug by nonadapted and adapted cells was characterized by a rapid initial accumulation during the first 2 min of incubation with [3H]cycloheximide, followed by a steady-state intracellular drug concentration well below that of the medium. The steady-state drug concentration was approximately 10-fold lower in adapted cells than in nonadapted cells. Treatment of cells with sodium azide or dinitrophenol abolished the difference between uptake of drug by nonadapted and adapted cells and resulted in intracellular drug levels equal to that of the medium. Direct efflux measurements showed that adapted cells loaded with cycloheximide were able to excrete the drug far more rapidly than nonadapted cells. These results suggest that both nonadapted and adapted cells possess an energy-dependent efflux mechanism for transporting cycloheximide and that resistance in adapted cells is due to increased efficiency of transport.
Full text
PDF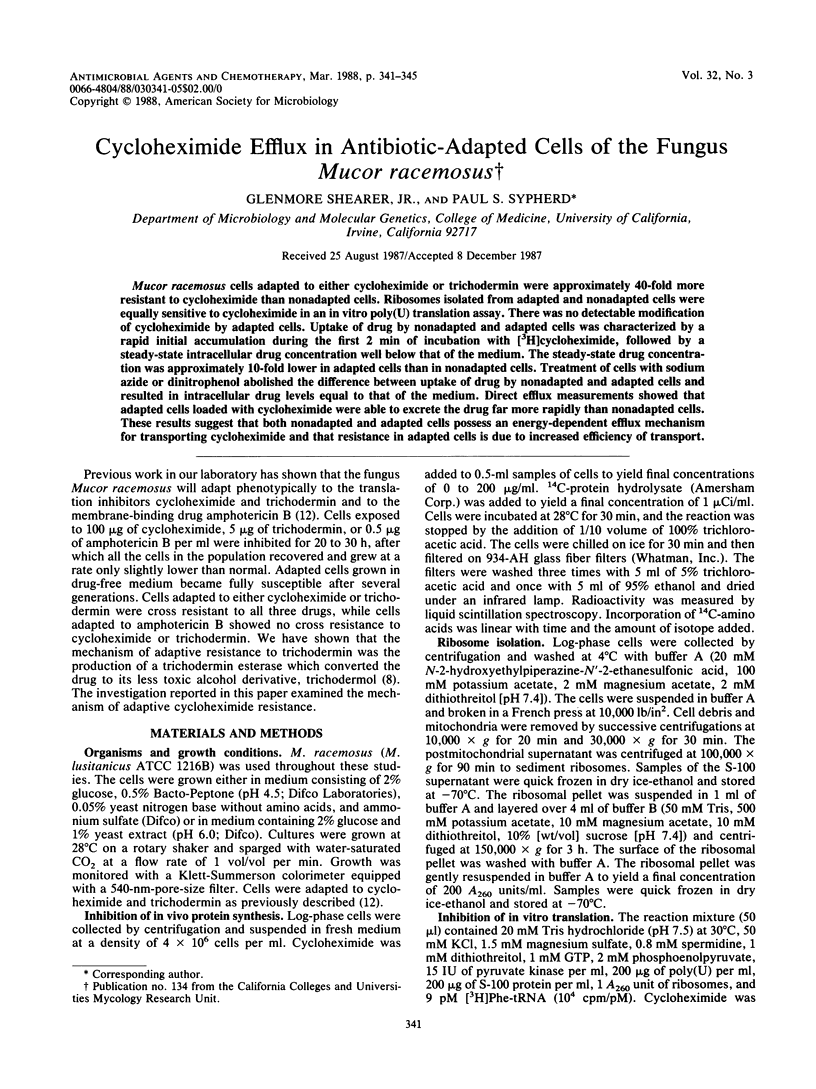
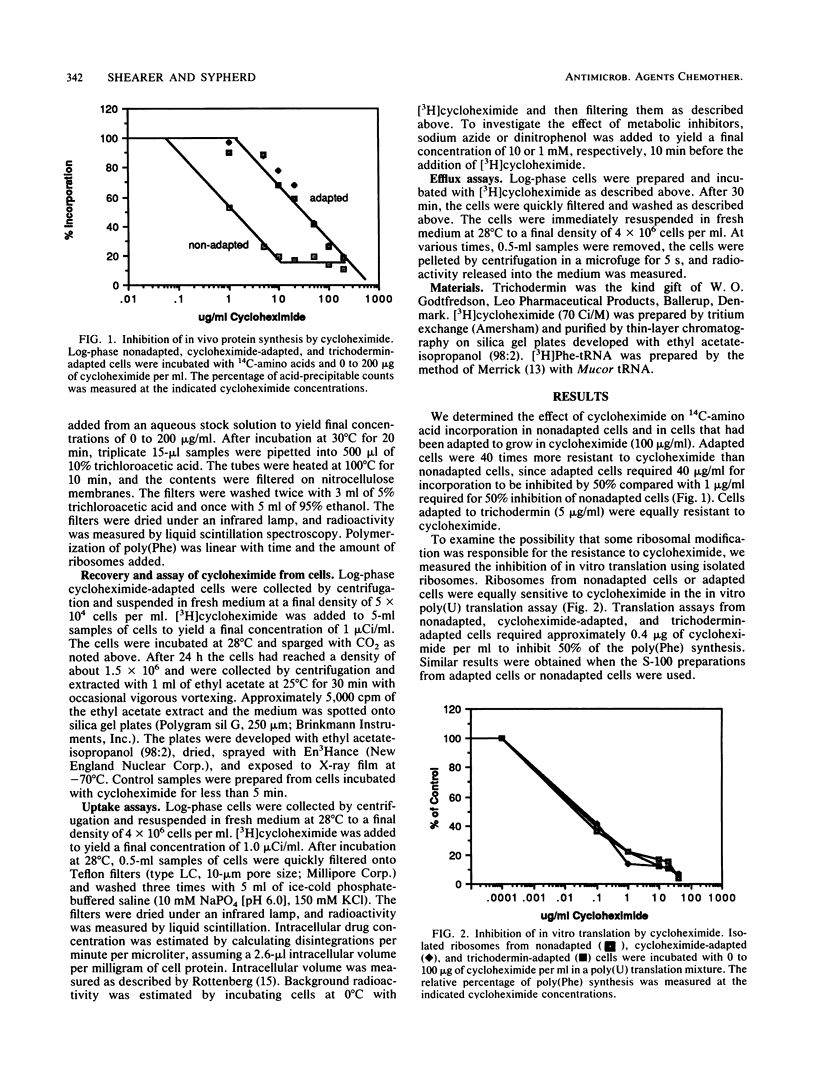
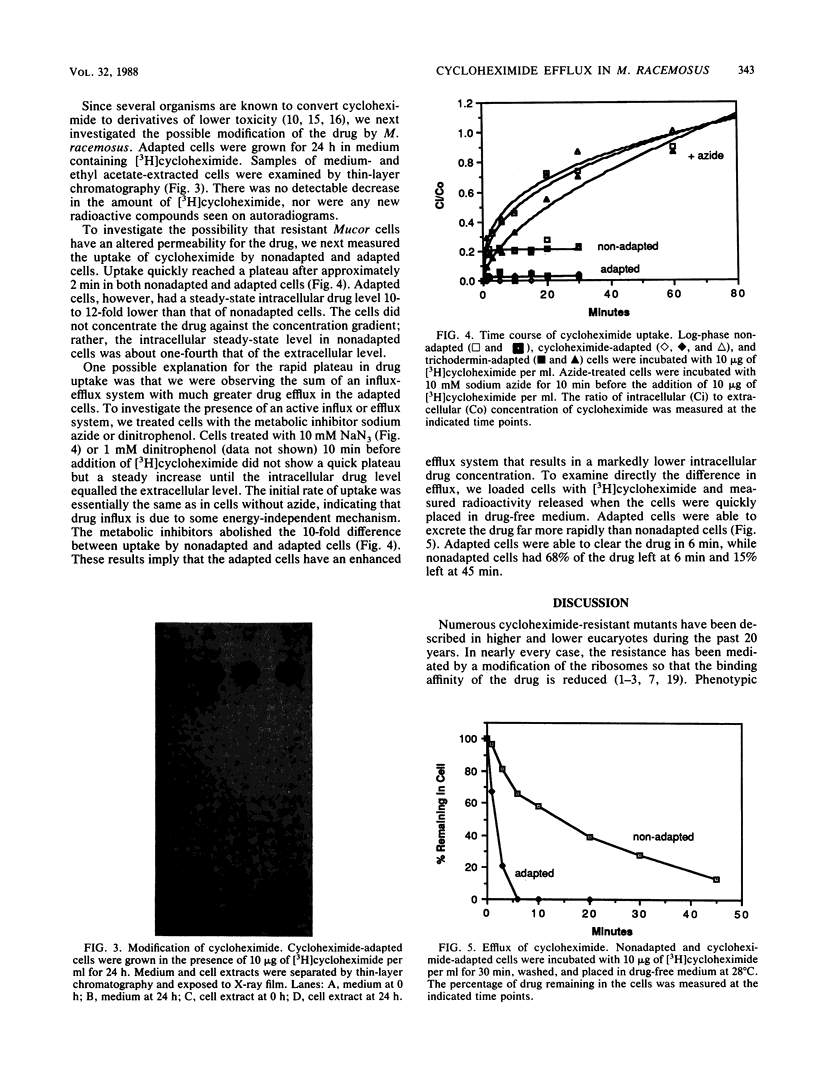
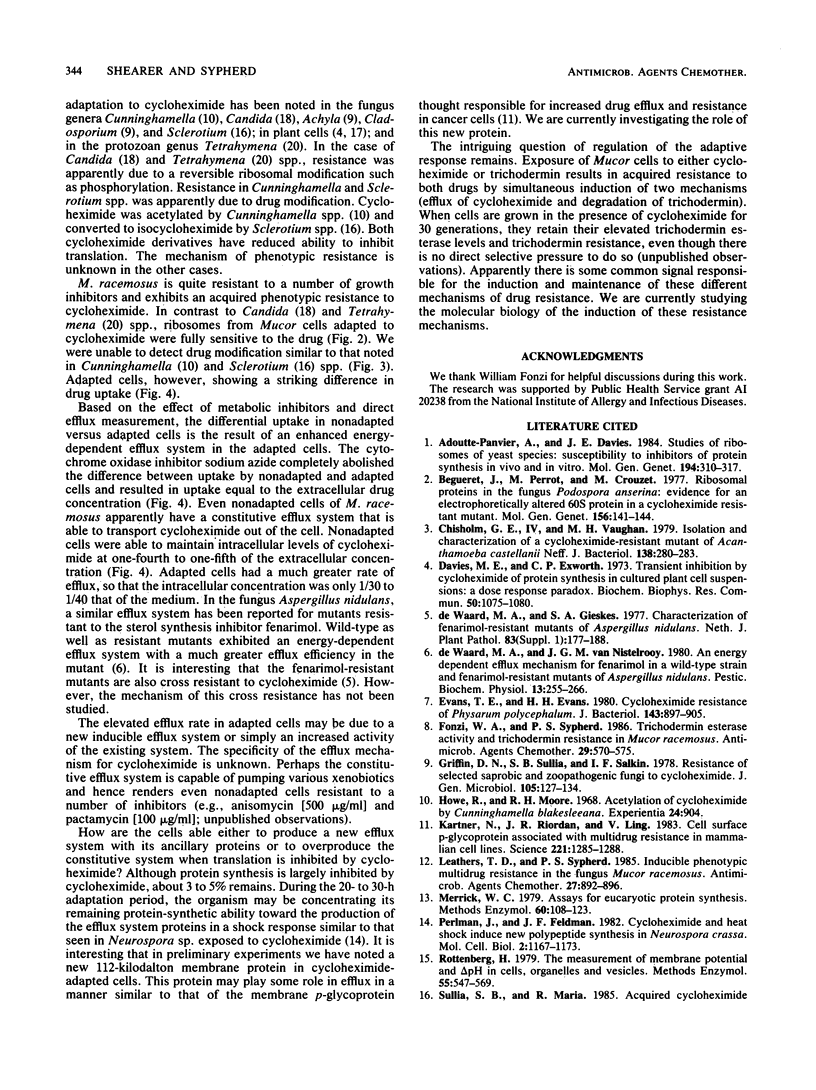
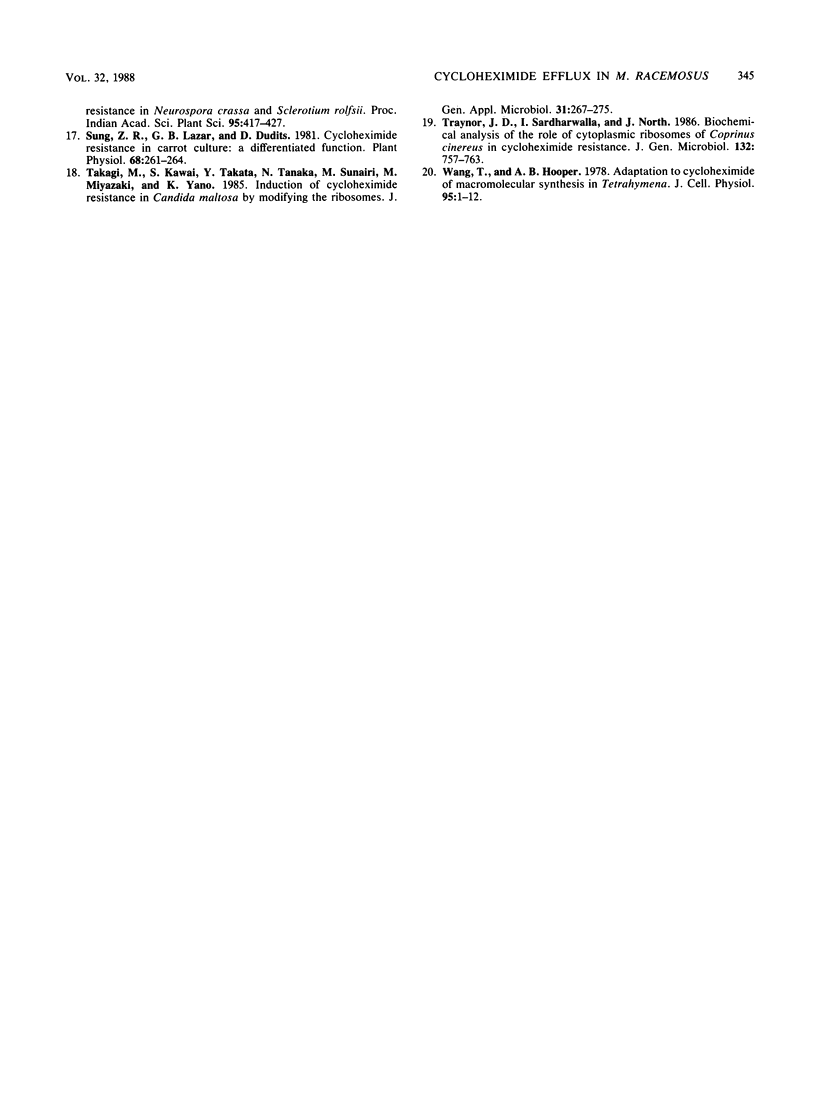
Images in this article
Selected References
These references are in PubMed. This may not be the complete list of references from this article.
- Begueret J., Perrot M., Crouzet M. Ribosomal proteins in the fungus Podospora anserina: evidence for an electrophoretically altered 60S protein in a cycloheximide resistant mutant. Mol Gen Genet. 1977 Nov 14;156(2):141–144. doi: 10.1007/BF00283486. [DOI] [PubMed] [Google Scholar]
- Chisholm G. E., 4th, Vaughan M. H. Isolation and characterization of a cycloheximide-resistant mutant of Acanthamoeba castellanii Neff. J Bacteriol. 1979 Apr;138(1):280–283. doi: 10.1128/jb.138.1.280-283.1979. [DOI] [PMC free article] [PubMed] [Google Scholar]
- Davies M. E., Exworth C. P. Transient inhibition by cycloheximide of protein synthesis in cultured plant cell suspensions: a dose response paradox. Biochem Biophys Res Commun. 1973 Feb 20;50(4):1075–1080. doi: 10.1016/0006-291x(73)91516-7. [DOI] [PubMed] [Google Scholar]
- Evans T. E., Evans H. H. Cycloheximide resistance of Physarum polycephalum. J Bacteriol. 1980 Aug;143(2):897–905. doi: 10.1128/jb.143.2.897-905.1980. [DOI] [PMC free article] [PubMed] [Google Scholar]
- Fonzi W. A., Sypherd P. S. Trichodermin esterase activity and trichodermin resistance in Mucor racemosus. Antimicrob Agents Chemother. 1986 Apr;29(4):570–575. doi: 10.1128/aac.29.4.570. [DOI] [PMC free article] [PubMed] [Google Scholar]
- Griffin D. H., Sullia S. B., Salkin I. F. Resistance of selected saprobic and zoopathogenic fungi to cycloheximide. J Gen Microbiol. 1978 Mar;105(1):127–134. doi: 10.1099/00221287-105-1-127. [DOI] [PubMed] [Google Scholar]
- Howe R., Moore R. H. Acetylation of cycloheximide by Cunninghamella blakesleeana. Experientia. 1968 Sep 15;24(9):904–904. doi: 10.1007/BF02138641. [DOI] [PubMed] [Google Scholar]
- Kartner N., Riordan J. R., Ling V. Cell surface P-glycoprotein associated with multidrug resistance in mammalian cell lines. Science. 1983 Sep 23;221(4617):1285–1288. doi: 10.1126/science.6137059. [DOI] [PubMed] [Google Scholar]
- Leathers T. D., Sypherd P. S. Inducible phenotypic multidrug resistance in the fungus Mucor racemosus. Antimicrob Agents Chemother. 1985 Jun;27(6):892–896. doi: 10.1128/aac.27.6.892. [DOI] [PMC free article] [PubMed] [Google Scholar]
- Merrick W. C. Assays for eukaryotic protein synthesis. Methods Enzymol. 1979;60:108–123. doi: 10.1016/s0076-6879(79)60011-3. [DOI] [PubMed] [Google Scholar]
- Perlman J., Feldman J. F. Cycloheximide and heat shock induce new polypeptide synthesis in Neurospora crassa. Mol Cell Biol. 1982 Oct;2(10):1167–1173. doi: 10.1128/mcb.2.10.1167. [DOI] [PMC free article] [PubMed] [Google Scholar]
- Rottenberg H. The measurement of membrane potential and deltapH in cells, organelles, and vesicles. Methods Enzymol. 1979;55:547–569. doi: 10.1016/0076-6879(79)55066-6. [DOI] [PubMed] [Google Scholar]
- Sung Z. R. Cycloheximide resistance in carrot culture: a differentiated function. Plant Physiol. 1981 Jul;68(1):261–264. doi: 10.1104/pp.68.1.261. [DOI] [PMC free article] [PubMed] [Google Scholar]
- Traynor J. D., Sardharwalla I., North J. Biochemical analysis of the role of cytoplasmic ribosomes of Coprinus cinereus in cycloheximide resistance. J Gen Microbiol. 1986 Mar;132(3):757–763. doi: 10.1099/00221287-132-3-757. [DOI] [PubMed] [Google Scholar]
- Wang T. C., Hooper A. B. Adaptation to cycloheximide of macromolecular synthesis in Tetrahymena. J Cell Physiol. 1978 Apr;95(1):1–11. doi: 10.1002/jcp.1040950102. [DOI] [PubMed] [Google Scholar]