Abstract
Several methods were used to determine enoxacin uptake in Escherichia coli strains because washing of cells removed all or most cell-associated enoxacin whereas no washing was associated with large amounts of cell-bound enoxacin. Washing after up to 40 to 45 min of exposure to enoxacin followed by suspension in drug-free medium prevented a significant effect of enoxacin on cell growth. Cell uptakes obtained with different methods showed no difference in the shape of the timed uptake curves but did show significant quantitative differences. These results are consistent with cell-associated enoxacin comprising a freely exchangeable pool of drug. Lineweaver-Burk plots of uptake were consistent with uptake of enoxacin by simple diffusion. No saturability and no competition with ciprofloxacin were observed. Low temperature (4 degrees C) was associated with decreased uptake. Arsenate, carbonyl cyanide m-chlorophenylhydrazone, sodium fluoride, sodium azide, and 2,4-dinitrophenol had no effect on uptake. We conclude that the mechanism of transport of enoxacin into cells is by simple diffusion. Mutants of E. coli with deficiency of outer membrane proteins F and C and an enoxacin-resistant mutant selected by serial passage with increasing enoxacin concentrations demonstrated that F porins play a significant role in enoxacin uptake and influence susceptibility to enoxacin. Uptake was shown to be similar in a strain of Bacillus subtilis.
Full text
PDF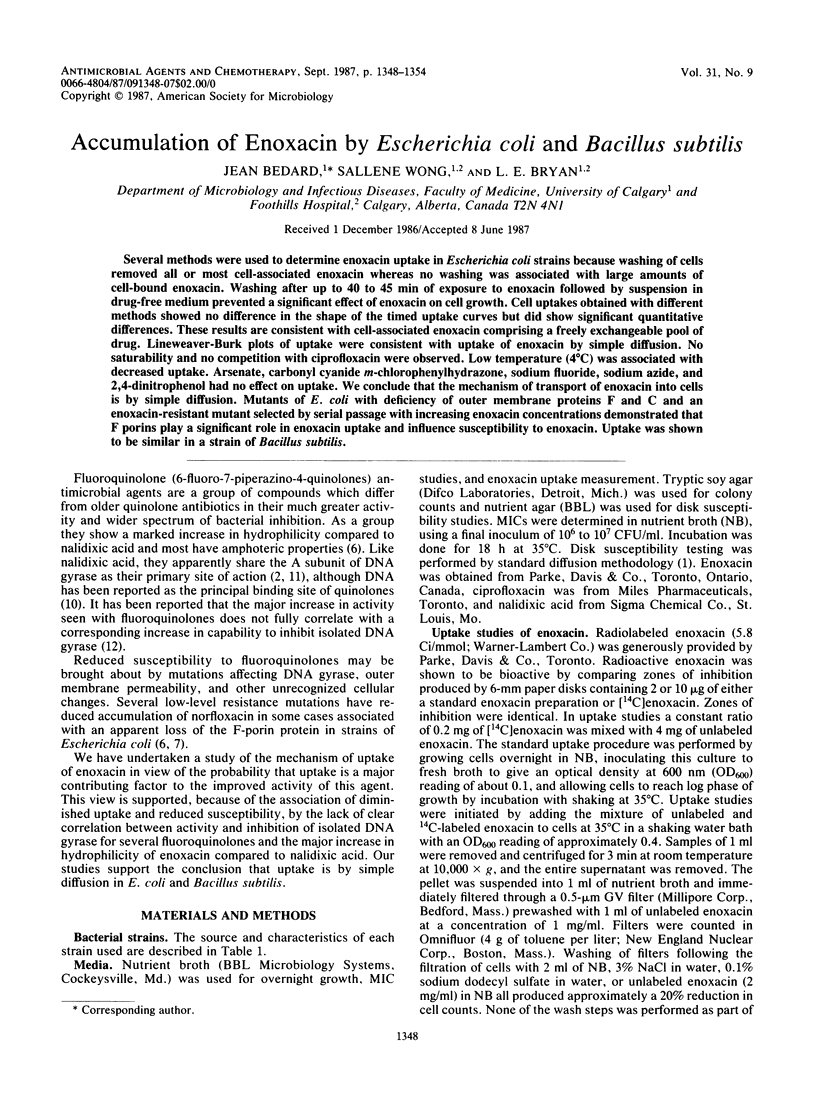
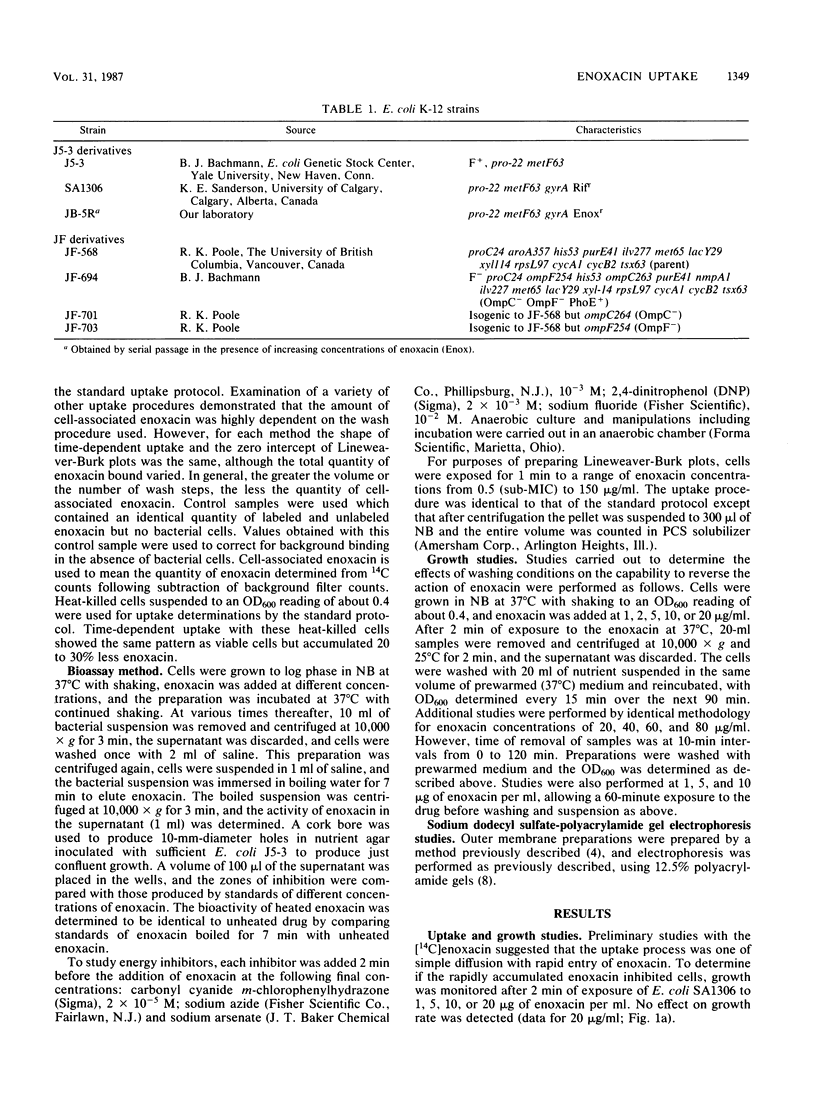
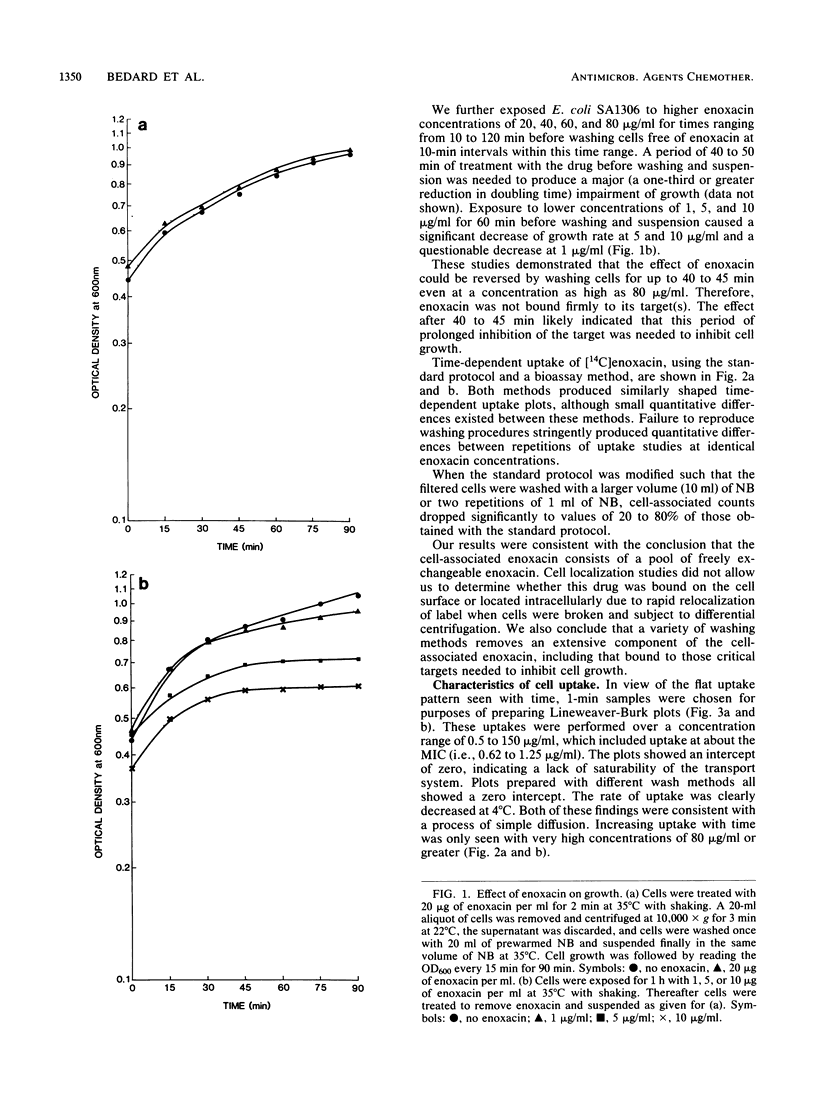
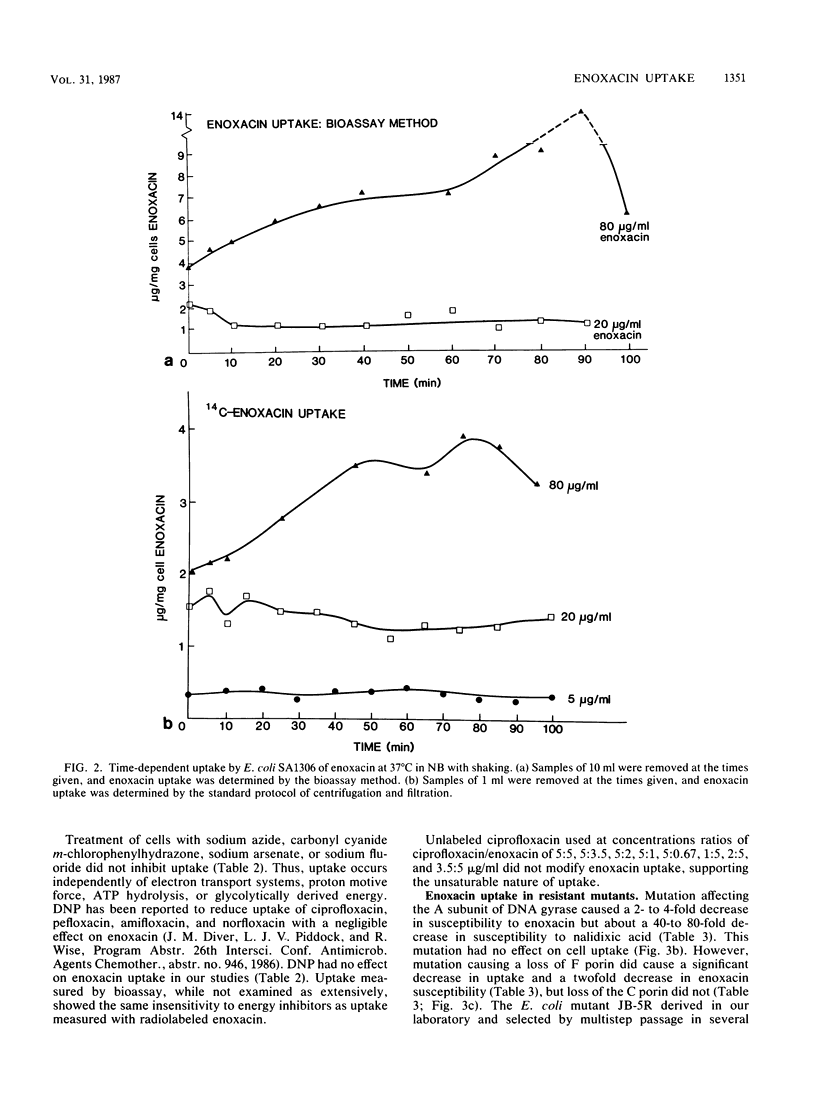
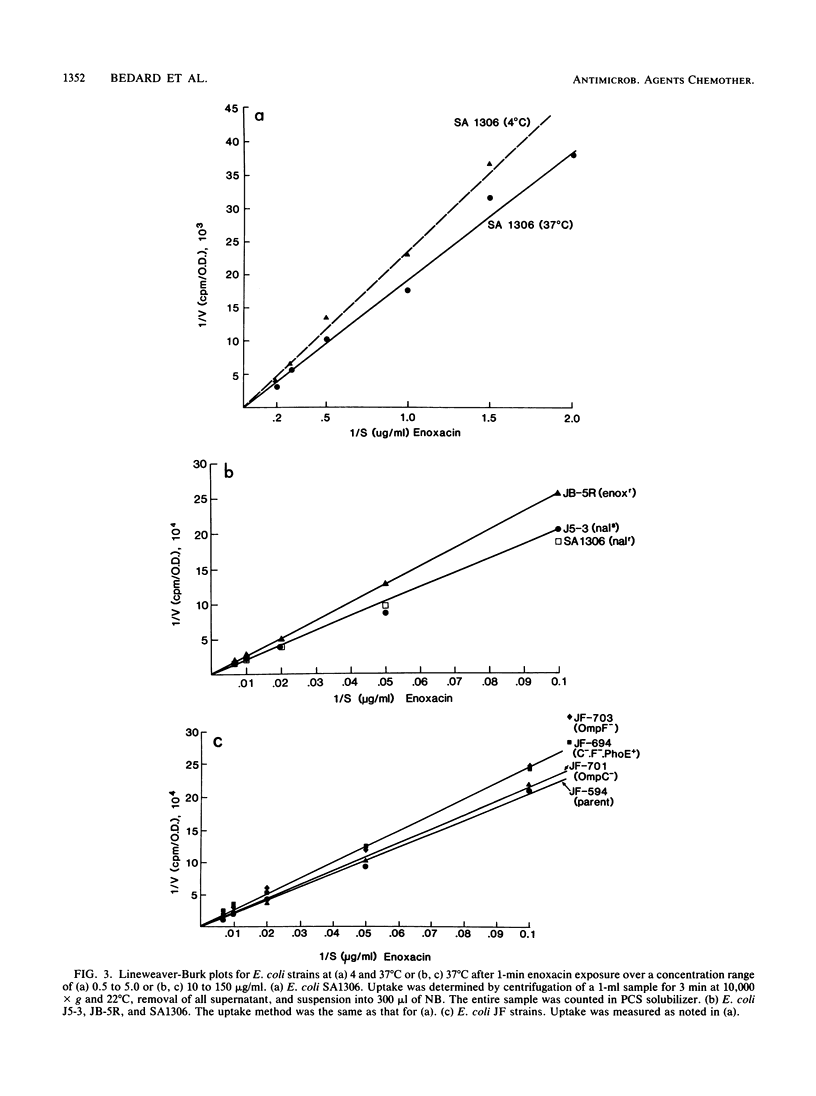
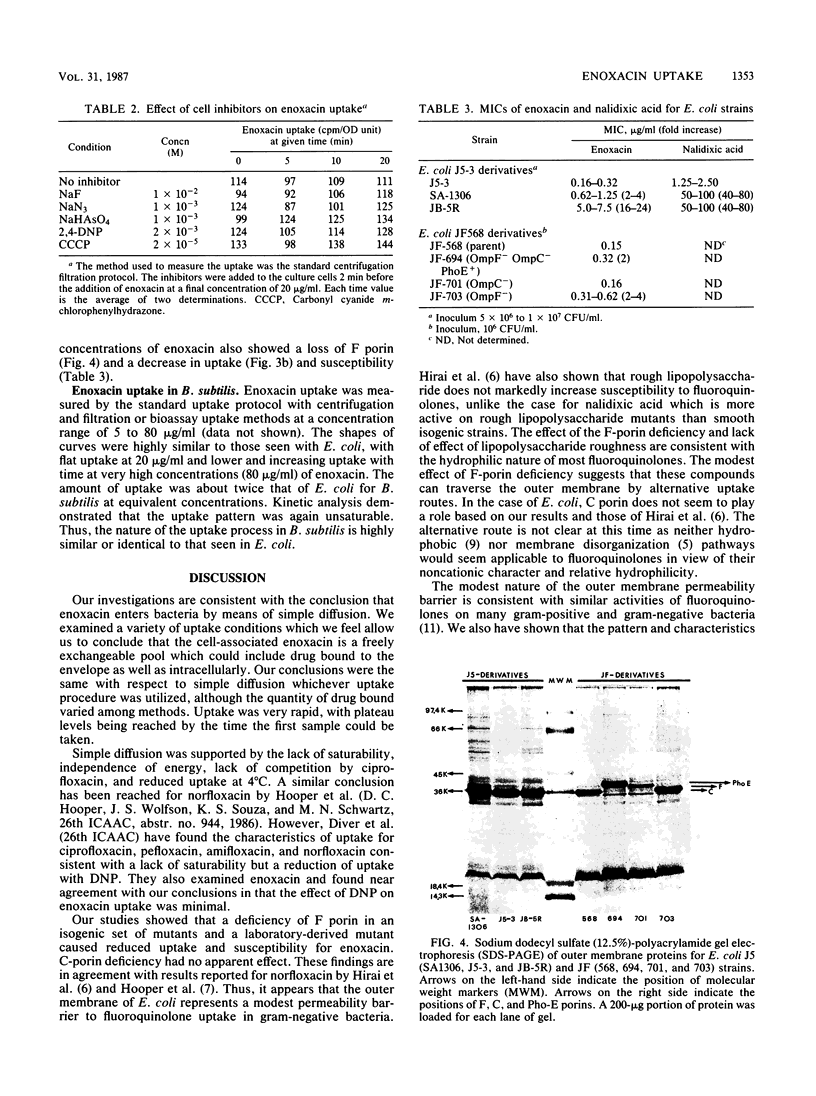
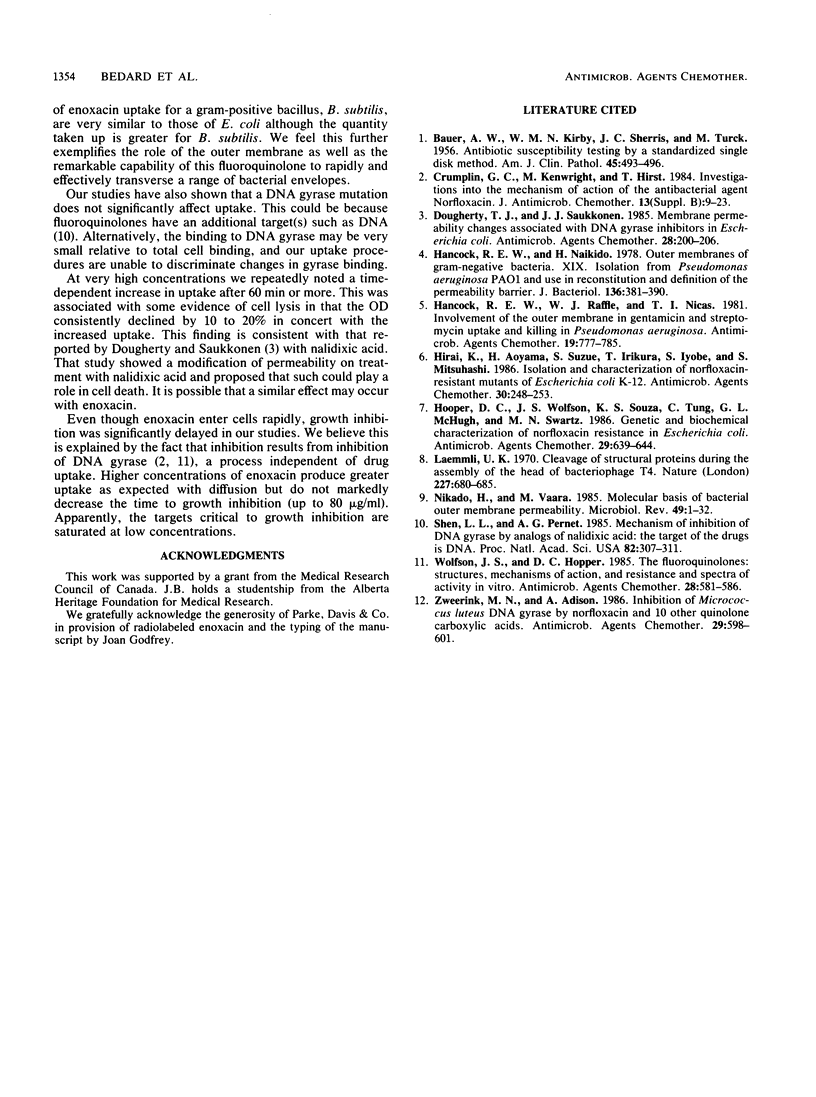
Images in this article
Selected References
These references are in PubMed. This may not be the complete list of references from this article.
- Bauer A. W., Kirby W. M., Sherris J. C., Turck M. Antibiotic susceptibility testing by a standardized single disk method. Am J Clin Pathol. 1966 Apr;45(4):493–496. [PubMed] [Google Scholar]
- Crumplin G. C., Kenwright M., Hirst T. Investigations into the mechanism of action of the antibacterial agent norfloxacin. J Antimicrob Chemother. 1984 May;13 (Suppl B):9–23. doi: 10.1093/jac/13.suppl_b.9. [DOI] [PubMed] [Google Scholar]
- Dougherty T. J., Saukkonen J. J. Membrane permeability changes associated with DNA gyrase inhibitors in Escherichia coli. Antimicrob Agents Chemother. 1985 Aug;28(2):200–206. doi: 10.1128/aac.28.2.200. [DOI] [PMC free article] [PubMed] [Google Scholar]
- Hancock R. E., Nikaido H. Outer membranes of gram-negative bacteria. XIX. Isolation from Pseudomonas aeruginosa PAO1 and use in reconstitution and definition of the permeability barrier. J Bacteriol. 1978 Oct;136(1):381–390. doi: 10.1128/jb.136.1.381-390.1978. [DOI] [PMC free article] [PubMed] [Google Scholar]
- Hancock R. E., Raffle V. J., Nicas T. I. Involvement of the outer membrane in gentamicin and streptomycin uptake and killing in Pseudomonas aeruginosa. Antimicrob Agents Chemother. 1981 May;19(5):777–785. doi: 10.1128/aac.19.5.777. [DOI] [PMC free article] [PubMed] [Google Scholar]
- Hirai K., Aoyama H., Suzue S., Irikura T., Iyobe S., Mitsuhashi S. Isolation and characterization of norfloxacin-resistant mutants of Escherichia coli K-12. Antimicrob Agents Chemother. 1986 Aug;30(2):248–253. doi: 10.1128/aac.30.2.248. [DOI] [PMC free article] [PubMed] [Google Scholar]
- Hooper D. C., Wolfson J. S., Souza K. S., Tung C., McHugh G. L., Swartz M. N. Genetic and biochemical characterization of norfloxacin resistance in Escherichia coli. Antimicrob Agents Chemother. 1986 Apr;29(4):639–644. doi: 10.1128/aac.29.4.639. [DOI] [PMC free article] [PubMed] [Google Scholar]
- Laemmli U. K. Cleavage of structural proteins during the assembly of the head of bacteriophage T4. Nature. 1970 Aug 15;227(5259):680–685. doi: 10.1038/227680a0. [DOI] [PubMed] [Google Scholar]
- Nikaido H., Vaara M. Molecular basis of bacterial outer membrane permeability. Microbiol Rev. 1985 Mar;49(1):1–32. doi: 10.1128/mr.49.1.1-32.1985. [DOI] [PMC free article] [PubMed] [Google Scholar]
- Shen L. L., Pernet A. G. Mechanism of inhibition of DNA gyrase by analogues of nalidixic acid: the target of the drugs is DNA. Proc Natl Acad Sci U S A. 1985 Jan;82(2):307–311. doi: 10.1073/pnas.82.2.307. [DOI] [PMC free article] [PubMed] [Google Scholar]
- Wolfson J. S., Hooper D. C. The fluoroquinolones: structures, mechanisms of action and resistance, and spectra of activity in vitro. Antimicrob Agents Chemother. 1985 Oct;28(4):581–586. doi: 10.1128/aac.28.4.581. [DOI] [PMC free article] [PubMed] [Google Scholar]
- Zweerink M. M., Edison A. Inhibition of Micrococcus luteus DNA gyrase by norfloxacin and 10 other quinolone carboxylic acids. Antimicrob Agents Chemother. 1986 Apr;29(4):598–601. doi: 10.1128/aac.29.4.598. [DOI] [PMC free article] [PubMed] [Google Scholar]