Abstract
The biosynthesis of monobactams by strains of Chromobacterium violaceum, Acetobacter sp., and Agrobacterium radiobacter was studied. Monobactams were produced during logarithmic growth by C. violaceum and Acetobacter sp. and during late log growth on glycerol and in stationary phase by A. radiobacter. The addition of various amino acids failed to significantly stimulate monobactam production in any of the producing organisms. Several 14C-amino acids and pyruvate were incorporated in vivo into monobactams. Serine, glycine, and cysteine were better incorporated than alanine or aspartate, whereas an excess of nonradioactive serine depressed the incorporation of labelled cysteine, glycine, and pyruvate. A comparison of [1-14C] glycine and [2-14C] glycine incorporation data suggests that glycine was first converted to serine. With a mixture of [U-14C[serine and [3-3H]serine, C. violaceum synthesized a monobactam with a complete retention of tritium, whereas with a [U-14C] cystine and [3-3H] cystine mixture, there was an extensive loss of C-3 tritium. Acetobacter sp. and A. radiobacter also utilized the double-labeled serine without the loss of tritium in their respective monobactams. It appears, therefore that in the three organisms, the carbon atoms of the beta-lactam ring of the monobactam are derived directly from serine without the loss of the C-3 hydrogen atoms, probably by an SN2 ring closure mechanism. With [methyl-14C] methionine, most of the radioactivity in the monobactam from Acetobacter sp. was in the methyl moiety of the beta-lactam ring methoxyl group.
Full text
PDF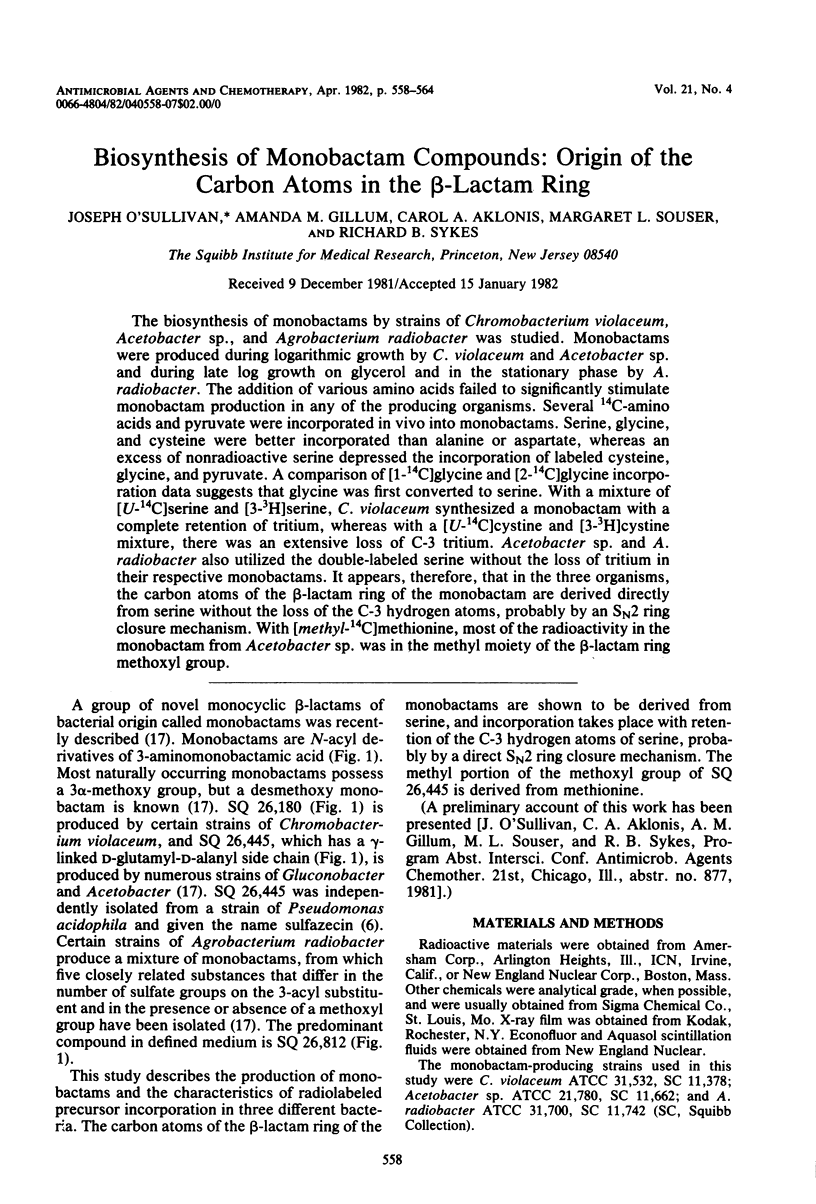
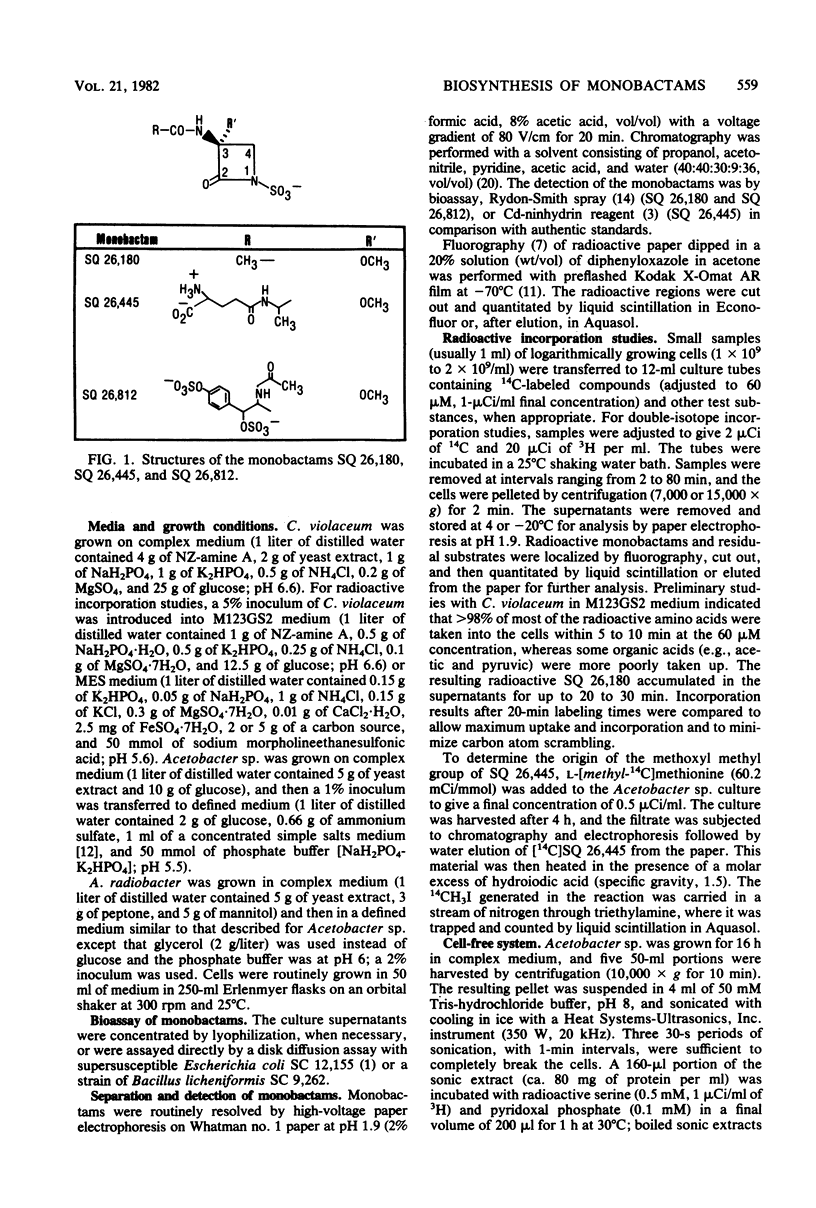
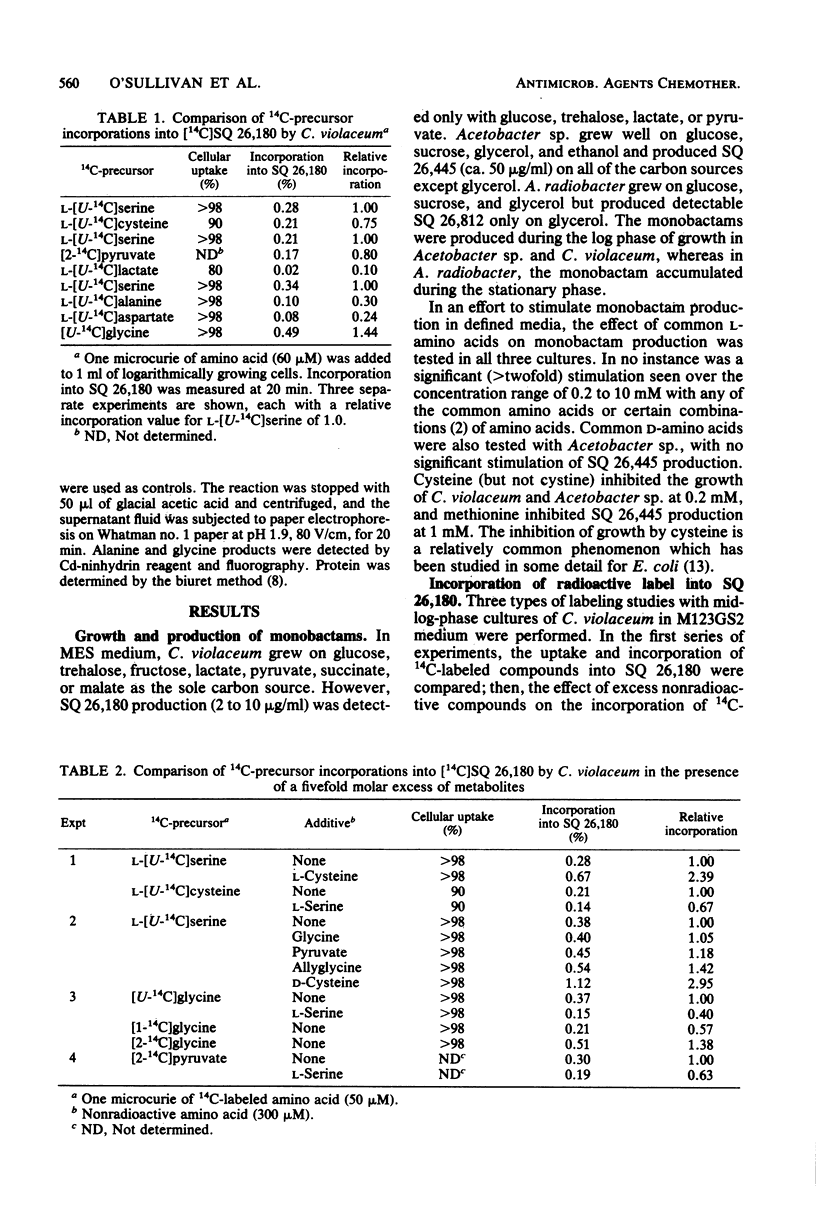
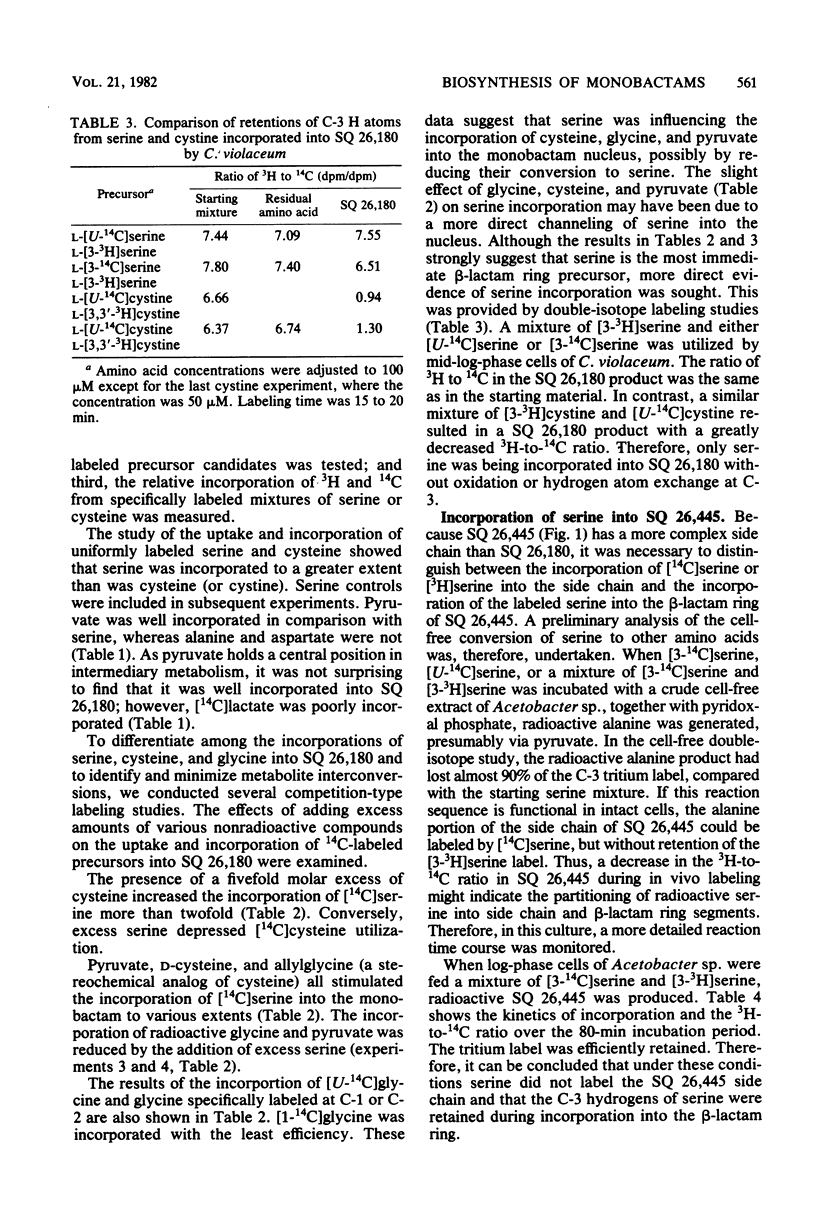
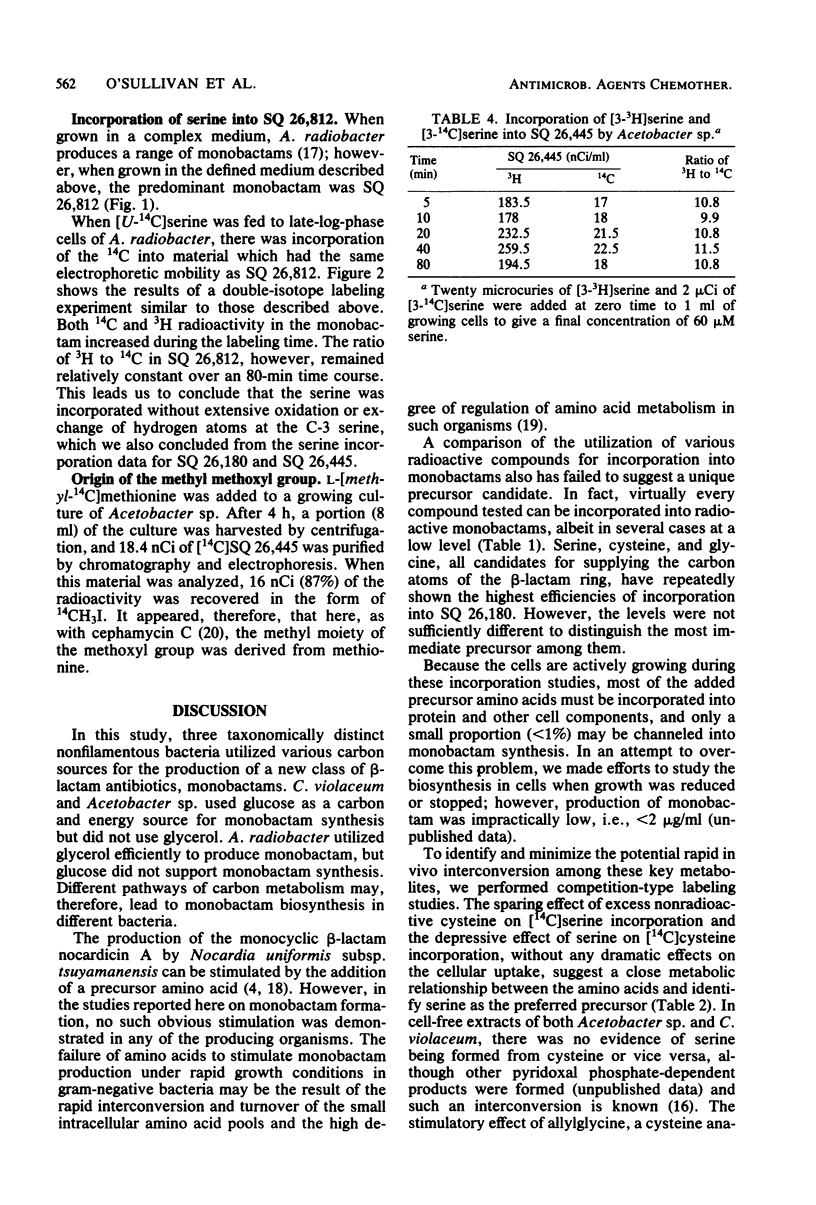
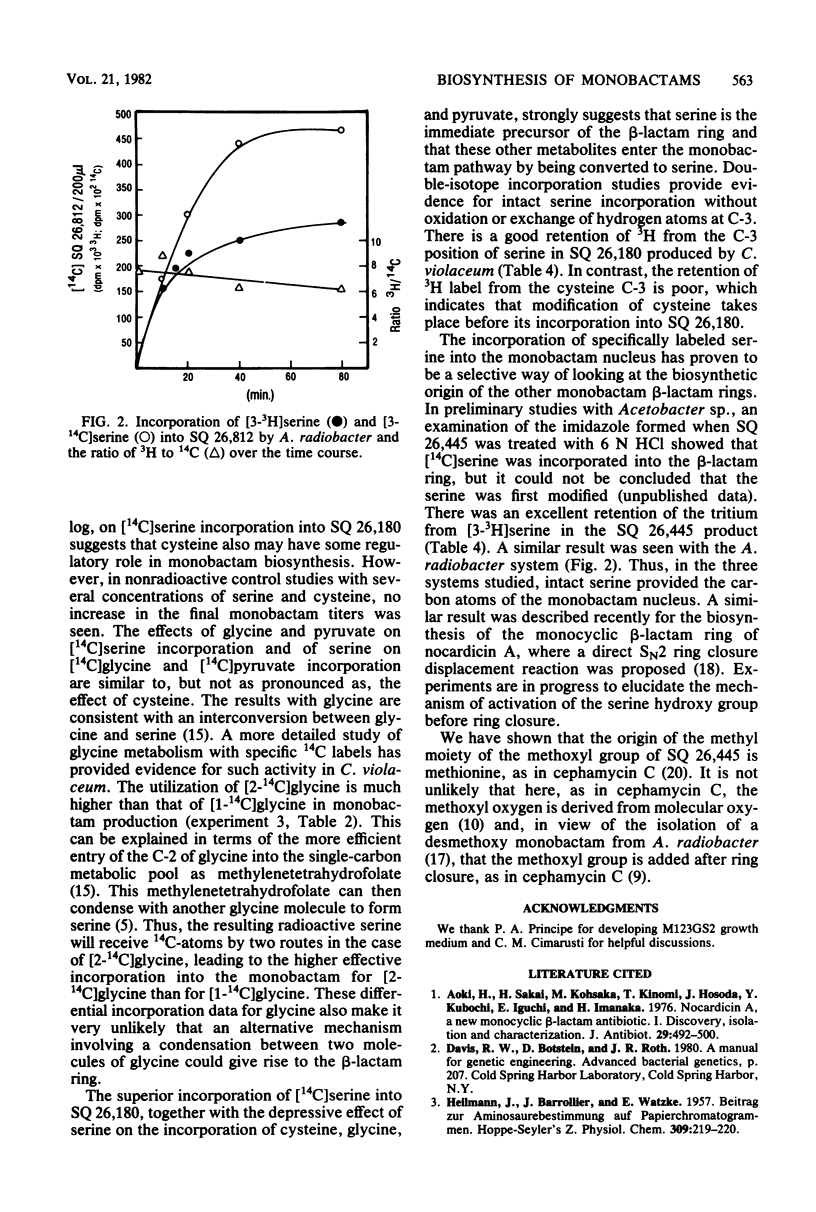
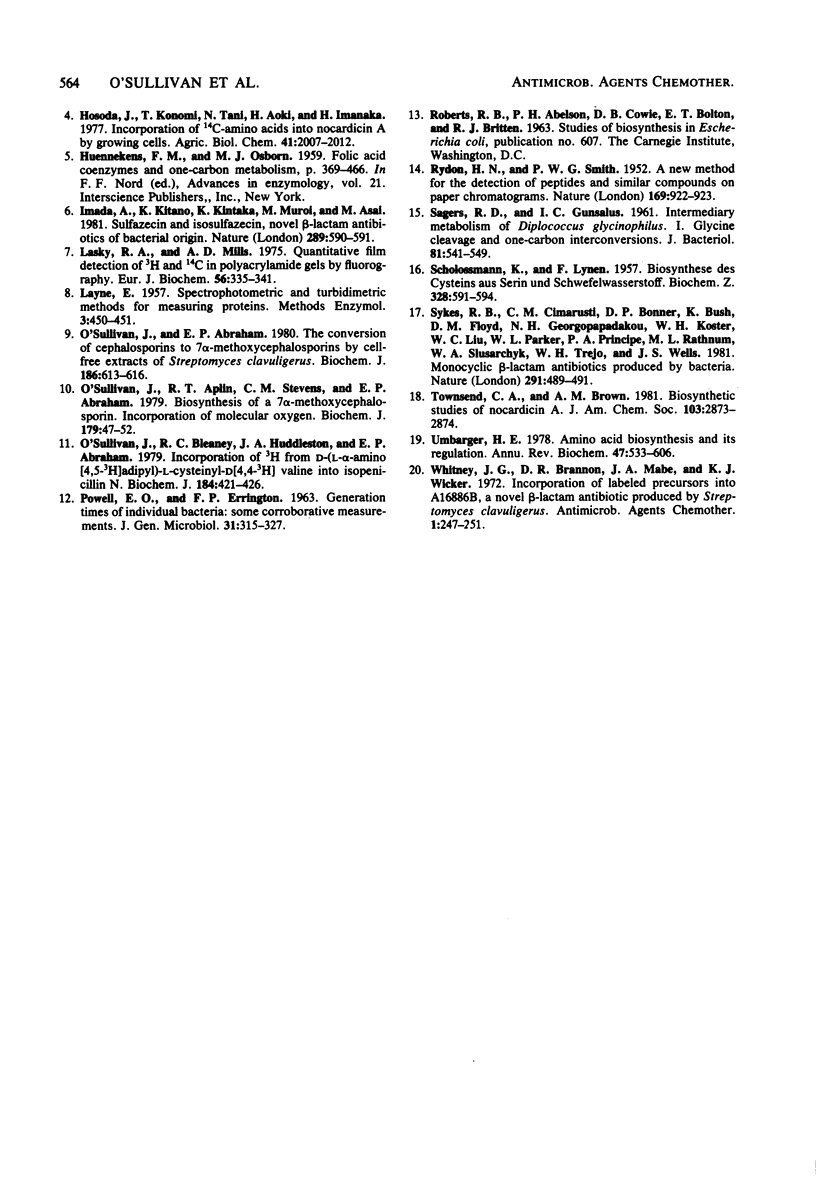
Selected References
These references are in PubMed. This may not be the complete list of references from this article.
- Aoki H., Sakai H., Kohsaka M., Konomi T., Hosoda J. Nocardicin A, a new monocyclic beta-lactam antibiotic. I. Discovery, isolation and characterization. J Antibiot (Tokyo) 1976 May;29(5):492–500. doi: 10.7164/antibiotics.29.492. [DOI] [PubMed] [Google Scholar]
- BREITENBACH J. W., DERKOSCH J., WESSELY F. Energetics of peptide formation. Nature. 1952 May 31;169(4309):922–922. doi: 10.1038/169922a0. [DOI] [PubMed] [Google Scholar]
- HEILMANN J., BARROLLIER J., WATZKE E. Beitrag zur Aminosäurebestimmung auf Papierchromatogrammen. Hoppe Seylers Z Physiol Chem. 1957;309(4-6):219–220. [PubMed] [Google Scholar]
- Imada A., Kitano K., Kintaka K., Muroi M., Asai M. Sulfazecin and isosulfazecin, novel beta-lactam antibiotics of bacterial origin. Nature. 1981 Feb 12;289(5798):590–591. doi: 10.1038/289590a0. [DOI] [PubMed] [Google Scholar]
- Laskey R. A., Mills A. D. Quantitative film detection of 3H and 14C in polyacrylamide gels by fluorography. Eur J Biochem. 1975 Aug 15;56(2):335–341. doi: 10.1111/j.1432-1033.1975.tb02238.x. [DOI] [PubMed] [Google Scholar]
- O'Sullivan J., Abraham E. P. The conversion of cephalosporins to 7 alpha-methoxycephalosporins by cell-free extracts of Streptomyces clavuligerus. Biochem J. 1980 Feb 15;186(2):613–616. doi: 10.1042/bj1860613. [DOI] [PMC free article] [PubMed] [Google Scholar]
- O'Sullivan J., Aplin R. T., Stevens C. M., Abraham E. P. Biosynthesis of a 7-alpha-methoxycephalosporin. Incorporation of molecular oxygen. Biochem J. 1979 Apr 1;179(1):47–52. doi: 10.1042/bj1790047. [DOI] [PMC free article] [PubMed] [Google Scholar]
- O'Sullivan J., Bleaney R. C., Huddleston J. A., Abraham E. P. Incorporation of 3H from delta-(L-alpha-amino (4,5-3H)adipyl)-L-cysteinyl-D-(4,4-3H)valine into isopenicillin N. Biochem J. 1979 Nov 15;184(2):421–426. doi: 10.1042/bj1840421. [DOI] [PMC free article] [PubMed] [Google Scholar]
- POWELL E. O., ERRINGTON F. P. Generation times of individual bacteria: some corroborative measurements. J Gen Microbiol. 1963 May;31:315–327. doi: 10.1099/00221287-31-2-315. [DOI] [PubMed] [Google Scholar]
- SAGERS R. D., GUNSALUS I. C. Intermediatry metabolism of Diplococcus glycinophilus. I. Glycine cleavage and one-carbon interconversions. J Bacteriol. 1961 Apr;81:541–549. doi: 10.1128/jb.81.4.541-549.1961. [DOI] [PMC free article] [PubMed] [Google Scholar]
- SCHLOSSMANN K., LYNEN F. Biosynthese des Cysteins aus Serin und Schwefelwasserstoff. Biochem Z. 1957;328(7):591–594. [PubMed] [Google Scholar]
- Sykes R. B., Cimarusti C. M., Bonner D. P., Bush K., Floyd D. M., Georgopapadakou N. H., Koster W. M., Liu W. C., Parker W. L., Principe P. A. Monocyclic beta-lactam antibiotics produced by bacteria. Nature. 1981 Jun 11;291(5815):489–491. doi: 10.1038/291489a0. [DOI] [PubMed] [Google Scholar]
- Umbarger H. E. Amino acid biosynthesis and its regulation. Annu Rev Biochem. 1978;47:532–606. doi: 10.1146/annurev.bi.47.070178.002533. [DOI] [PubMed] [Google Scholar]
- Whitney J. G., Brannon D. R., Mabe J. A., Wicker K. J. Incorporation of labeled precursors into A16886B, a novel -lactam antibiotic produced by Streptomyces clavuligerus. Antimicrob Agents Chemother. 1972 Mar;1(3):247–251. doi: 10.1128/aac.1.3.247. [DOI] [PMC free article] [PubMed] [Google Scholar]