Abstract
The influence of D-ribose as a cosubstrate on the uptake and metabolism of the non-growth substrate D-xylose by Saccharomyces cerevisiae ATCC 26602 was investigated. Xylose was taken up by means of low- and high-affinity glucose transport systems. In cells exposed for 2 days to a mixture of xylose and ribose, only the high-affinity system could be detected. Glucose strongly inhibited the transport of xylose by both systems. Starvation or exposure to either xylose or ribose resulted in inactivation of xylose transport, which did not occur in the presence of a mixture of ribose and xylose. A constitutive non-glucose-repressible NADPH2-dependent xylose reductase with a specific activity of ca. 5 mU/mg of protein that converted xylose to xylitol was present in a glucose-grown culture. No activity converting xylitol to xylulose or vice versa was found in crude extracts. Both xylose and ribose were converted to their corresponding polyols, xylitol and ribitol, as indicated by 13C nuclear magnetic resonance spectroscopy. Furthermore, ethanol was detected, and this implied that pathways for the complete catabolism of xylose and ribose exist. However, the NADPH2 required for the conversion of xylose to xylitol is apparently not supplied by the pentose phosphate pathway since the ethanol produced from D-[1-13C]xylose was labelled only in the C-2 position. Acetic acid was produced from ribose and may assist in the conversion of xylose to xylitol by cycling NADPH2.
Full text
PDF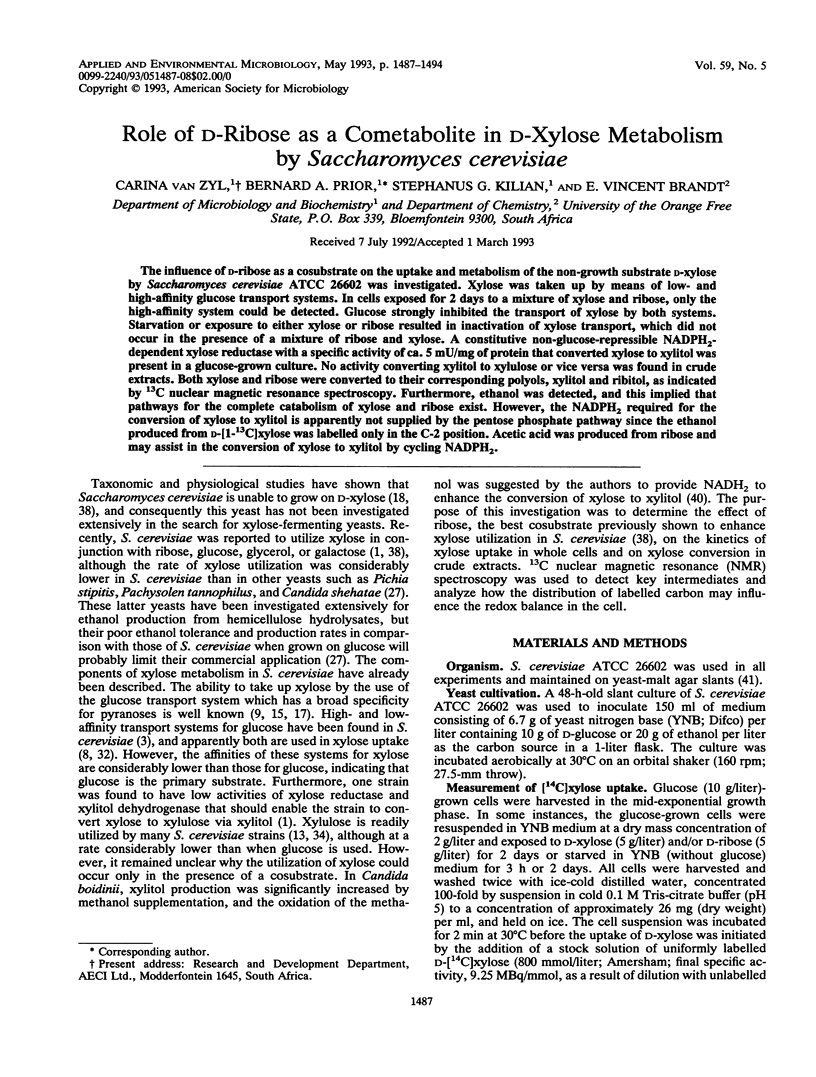
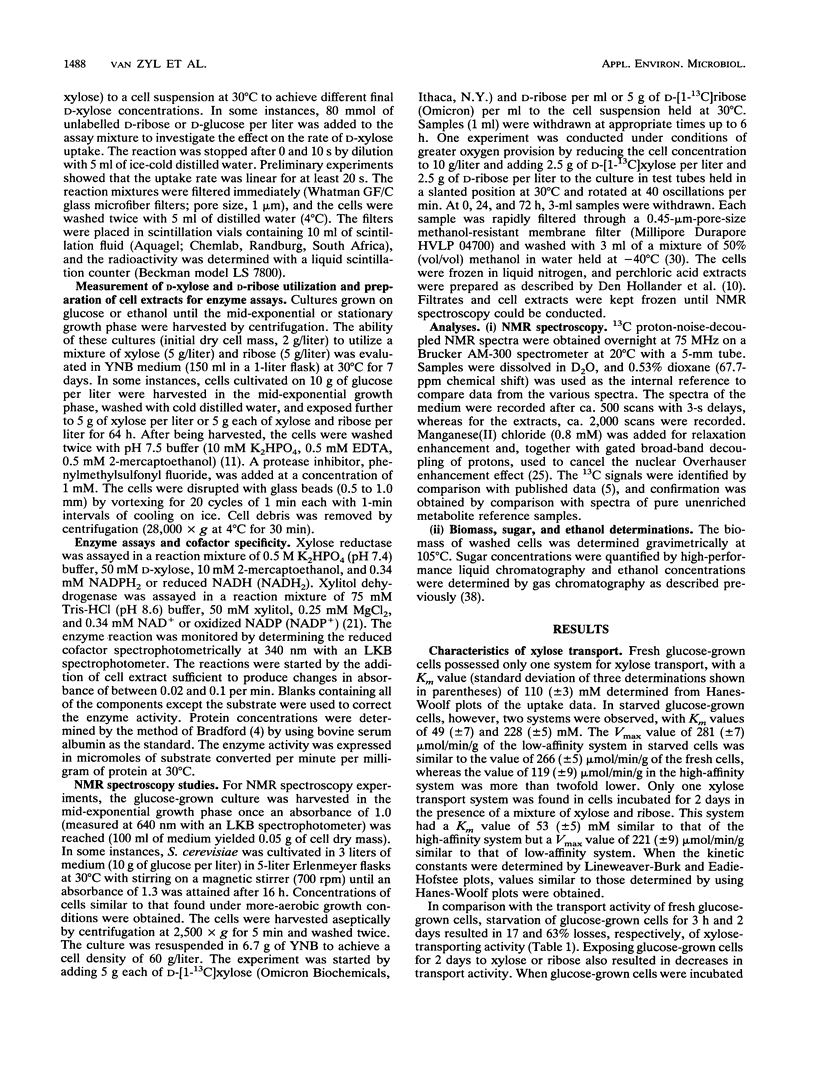
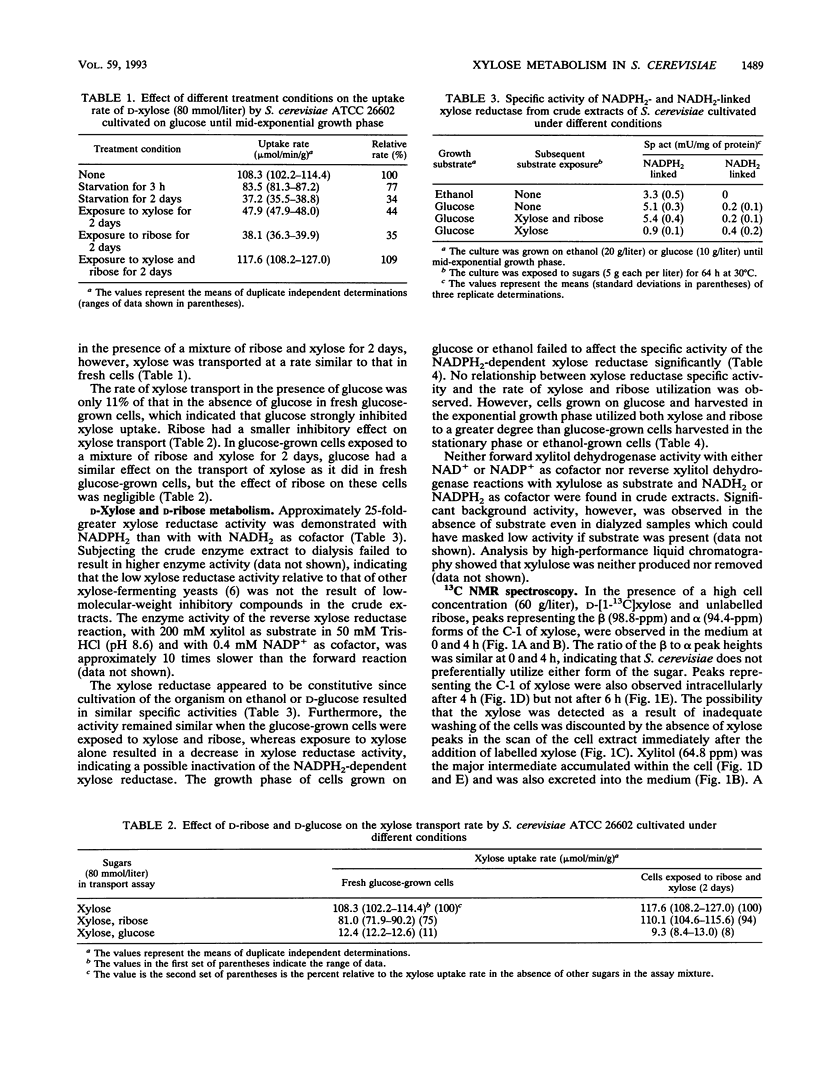
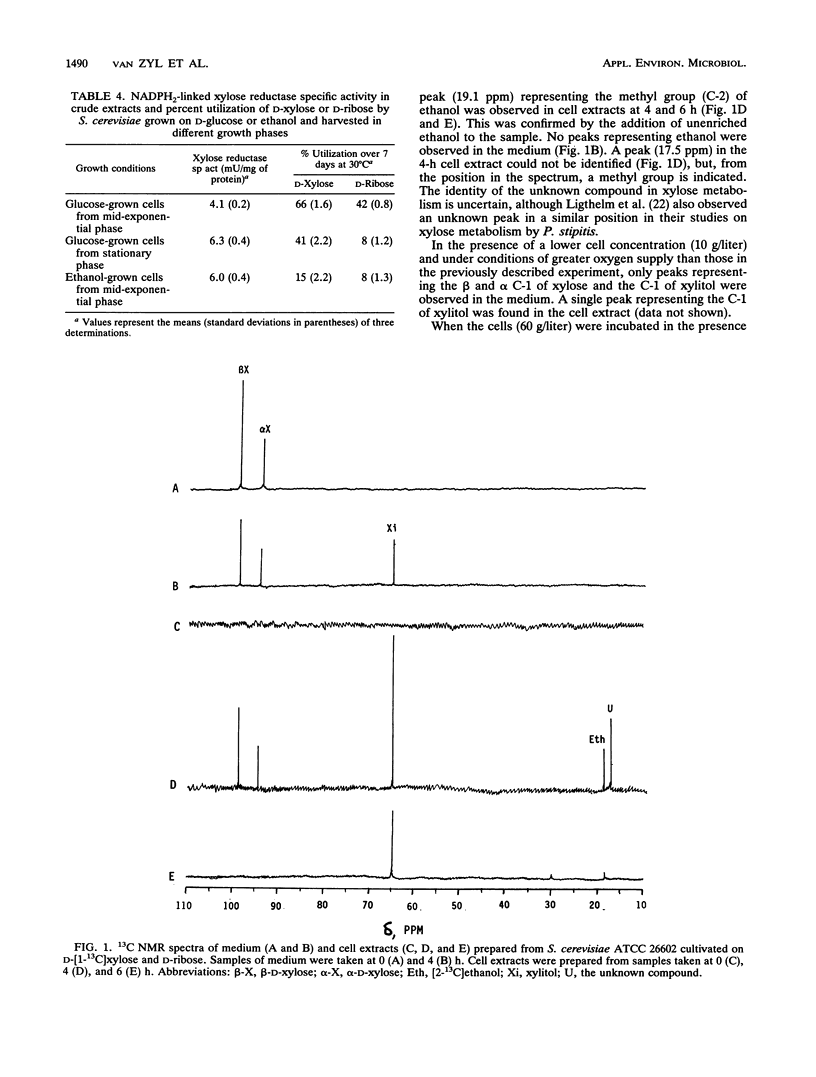
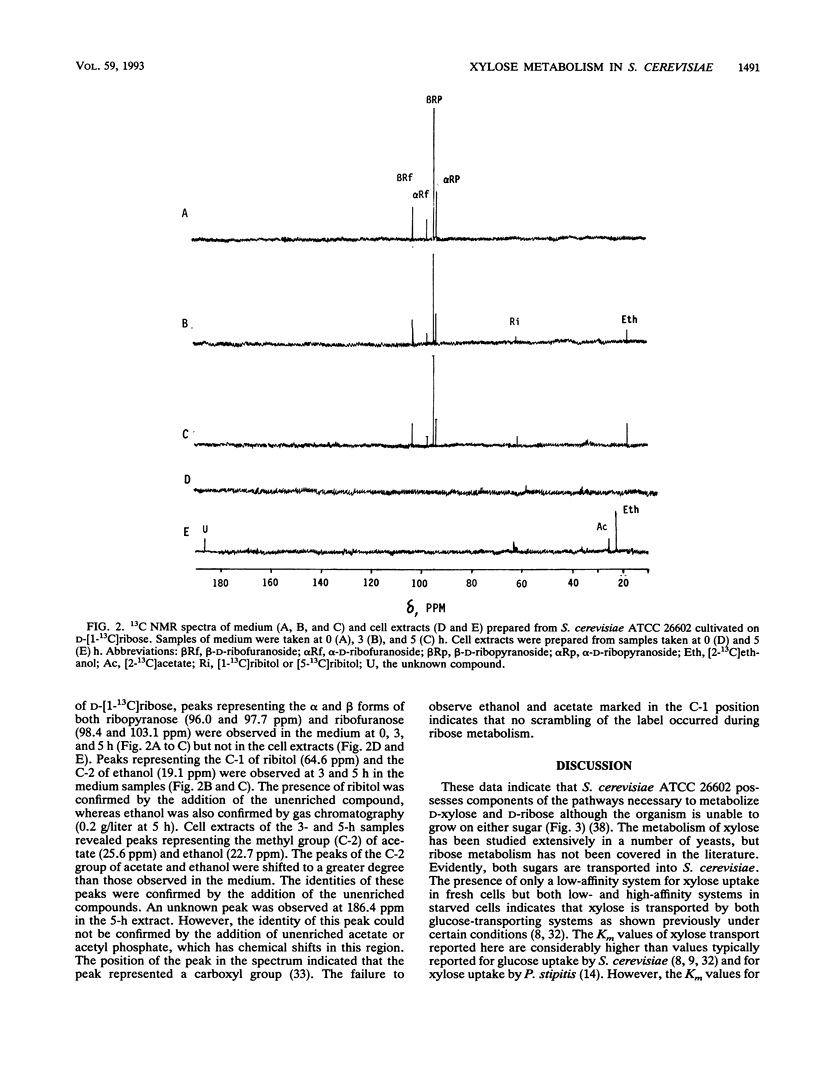
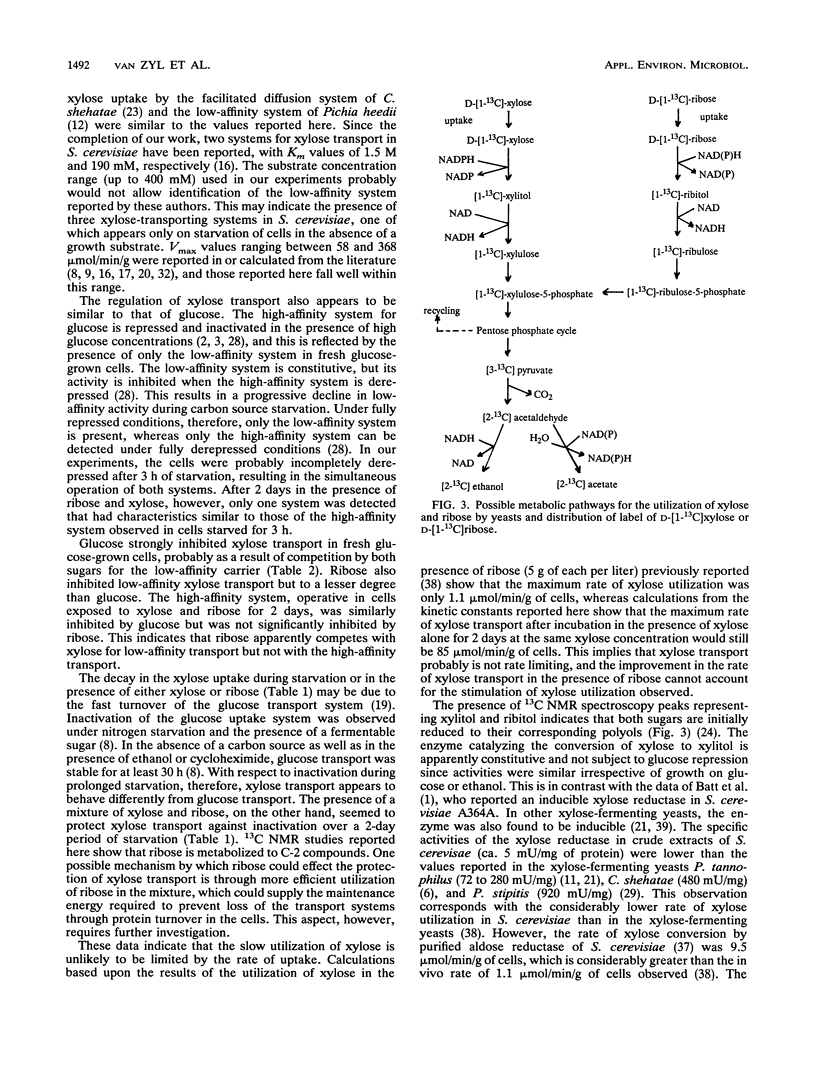
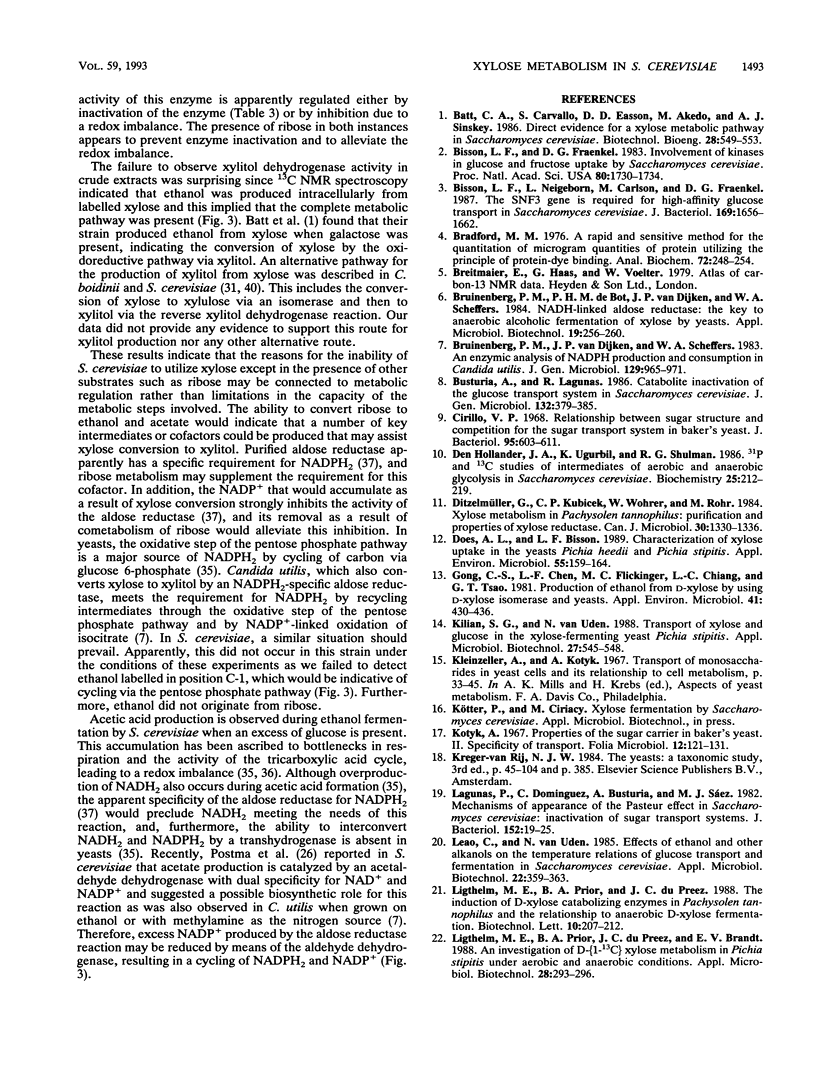
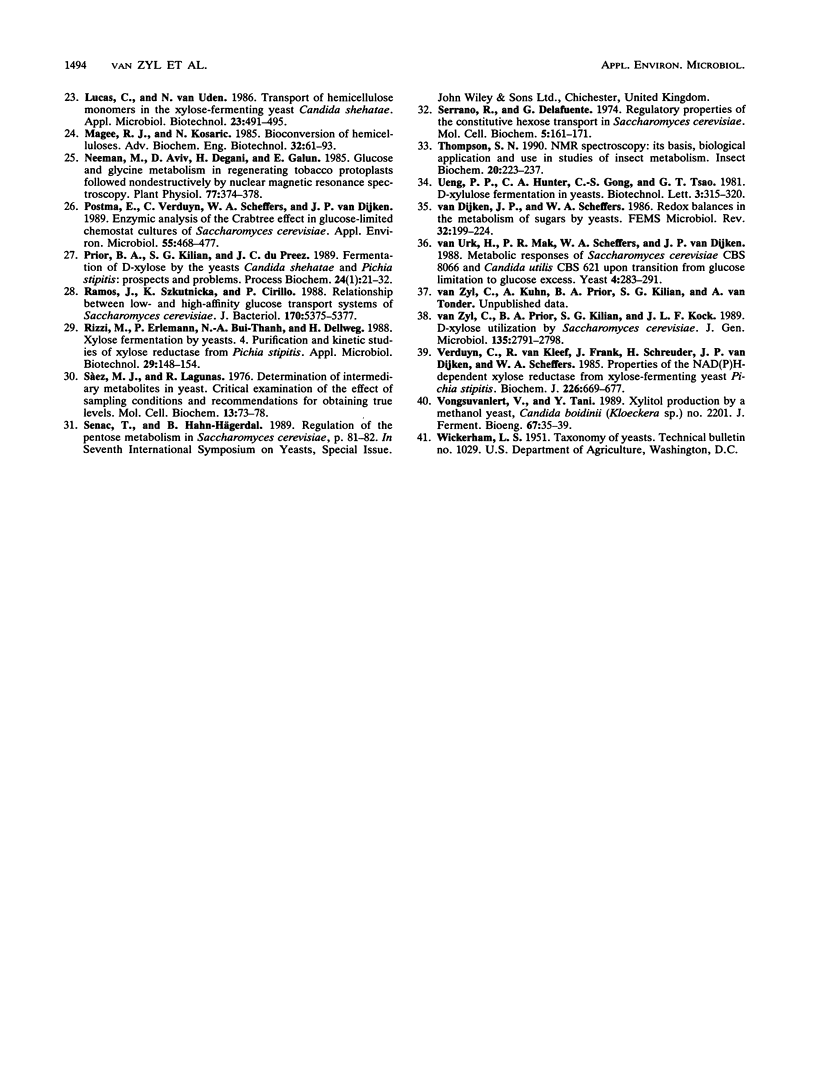
Selected References
These references are in PubMed. This may not be the complete list of references from this article.
- Bisson L. F., Fraenkel D. G. Involvement of kinases in glucose and fructose uptake by Saccharomyces cerevisiae. Proc Natl Acad Sci U S A. 1983 Mar;80(6):1730–1734. doi: 10.1073/pnas.80.6.1730. [DOI] [PMC free article] [PubMed] [Google Scholar]
- Bisson L. F., Neigeborn L., Carlson M., Fraenkel D. G. The SNF3 gene is required for high-affinity glucose transport in Saccharomyces cerevisiae. J Bacteriol. 1987 Apr;169(4):1656–1662. doi: 10.1128/jb.169.4.1656-1662.1987. [DOI] [PMC free article] [PubMed] [Google Scholar]
- Bradford M. M. A rapid and sensitive method for the quantitation of microgram quantities of protein utilizing the principle of protein-dye binding. Anal Biochem. 1976 May 7;72:248–254. doi: 10.1016/0003-2697(76)90527-3. [DOI] [PubMed] [Google Scholar]
- Bruinenberg P. M., van Dijken J. P., Scheffers W. A. An enzymic analysis of NADPH production and consumption in Candida utilis. J Gen Microbiol. 1983 Apr;129(4):965–971. doi: 10.1099/00221287-129-4-965. [DOI] [PubMed] [Google Scholar]
- Busturia A., Lagunas R. Catabolite inactivation of the glucose transport system in Saccharomyces cerevisiae. J Gen Microbiol. 1986 Feb;132(2):379–385. doi: 10.1099/00221287-132-2-379. [DOI] [PubMed] [Google Scholar]
- Cirillo V. P. Relationship between sugar structure and competition for the sugar transport system in Bakers' yeast. J Bacteriol. 1968 Feb;95(2):603–611. doi: 10.1128/jb.95.2.603-611.1968. [DOI] [PMC free article] [PubMed] [Google Scholar]
- Does A. L., Bisson L. F. Characterization of Xylose Uptake in the Yeasts Pichia heedii and Pichia stipitis. Appl Environ Microbiol. 1989 Jan;55(1):159–164. doi: 10.1128/aem.55.1.159-164.1989. [DOI] [PMC free article] [PubMed] [Google Scholar]
- Gong C. S., Chen L. F., Flickinger M. C., Chiang L. C., Tsao G. T. Production of Ethanol from d-Xylose by Using d-Xylose Isomerase and Yeasts. Appl Environ Microbiol. 1981 Feb;41(2):430–436. doi: 10.1128/aem.41.2.430-436.1981. [DOI] [PMC free article] [PubMed] [Google Scholar]
- Kotyk A. Properties of the sugar carrier in baker's yeast. II. Specificity of transport. Folia Microbiol (Praha) 1967;12(2):121–131. doi: 10.1007/BF02896872. [DOI] [PubMed] [Google Scholar]
- Lagunas R., Dominguez C., Busturia A., Sáez M. J. Mechanisms of appearance of the Pasteur effect in Saccharomyces cerevisiae: inactivation of sugar transport systems. J Bacteriol. 1982 Oct;152(1):19–25. doi: 10.1128/jb.152.1.19-25.1982. [DOI] [PMC free article] [PubMed] [Google Scholar]
- Magee R. J., Kosaric N. Bioconversion of hemicellulosics. Adv Biochem Eng Biotechnol. 1985;32:61–93. doi: 10.1007/BFb0009525. [DOI] [PubMed] [Google Scholar]
- Neeman M., Aviv D., Degani H., Galun E. Glucose and glycine metabolism in regenerating tobacco protoplasts: followed nondestructively by nuclear magnetic resonance spectroscopy. Plant Physiol. 1985 Feb;77(2):374–378. doi: 10.1104/pp.77.2.374. [DOI] [PMC free article] [PubMed] [Google Scholar]
- Postma E., Verduyn C., Scheffers W. A., Van Dijken J. P. Enzymic analysis of the crabtree effect in glucose-limited chemostat cultures of Saccharomyces cerevisiae. Appl Environ Microbiol. 1989 Feb;55(2):468–477. doi: 10.1128/aem.55.2.468-477.1989. [DOI] [PMC free article] [PubMed] [Google Scholar]
- Ramos J., Szkutnicka K., Cirillo V. P. Relationship between low- and high-affinity glucose transport systems of Saccharomyces cerevisiae. J Bacteriol. 1988 Nov;170(11):5375–5377. doi: 10.1128/jb.170.11.5375-5377.1988. [DOI] [PMC free article] [PubMed] [Google Scholar]
- Serrano R., Delafuente G. Regulatory properties of the constitutive hexose transport in Saccharomyces cerevisiae. Mol Cell Biochem. 1974 Dec 20;5(3):161–171. doi: 10.1007/BF01731379. [DOI] [PubMed] [Google Scholar]
- Sáez M. J., Lagunas R. Determination of intermediary metabolites in yeast. Critical examination of the effect of sampling conditions and recommendations for obtaining true levels. Mol Cell Biochem. 1976 Nov 30;13(2):73–78. doi: 10.1007/BF01837056. [DOI] [PubMed] [Google Scholar]
- Van Urk H., Mak P. R., Scheffers W. A., van Dijken J. P. Metabolic responses of Saccharomyces cerevisiae CBS 8066 and Candida utilis CBS 621 upon transition from glucose limitation to glucose excess. Yeast. 1988 Dec;4(4):283–291. doi: 10.1002/yea.320040406. [DOI] [PubMed] [Google Scholar]
- Verduyn C., Van Kleef R., Frank J., Schreuder H., Van Dijken J. P., Scheffers W. A. Properties of the NAD(P)H-dependent xylose reductase from the xylose-fermenting yeast Pichia stipitis. Biochem J. 1985 Mar 15;226(3):669–677. doi: 10.1042/bj2260669. [DOI] [PMC free article] [PubMed] [Google Scholar]
- den Hollander J. A., Ugurbil K., Shulman R. G. 31P and 13C NMR studies of intermediates of aerobic and anaerobic glycolysis in Saccharomyces cerevisiae. Biochemistry. 1986 Jan 14;25(1):212–219. doi: 10.1021/bi00349a030. [DOI] [PubMed] [Google Scholar]
- van Zyl C., Prior B. A., Kilian S. G., Kock J. L. D-xylose utilization by Saccharomyces cerevisiae. J Gen Microbiol. 1989 Nov;135(11):2791–2798. doi: 10.1099/00221287-135-11-2791. [DOI] [PubMed] [Google Scholar]