Abstract
Marine bacteria in Resurrection Bay near Seward, Alaska, and in the central North Sea off the Dutch coast were cultured in filtered autoclaved seawater following dilution to extinction. The populations present before dilution varied from 0.11 × 109 to 1.07 × 109 cells per liter. The mean cell volume varied between 0.042 and 0.074 μm3, and the mean apparent DNA content of the cells ranged from 2.5 to 4.7 fg of DNA per cell. All three parameters were determined by high-resolution flow cytometry. All 37 strains that were obtained from very high dilutions of Resurrection Bay and North Sea samples represented facultatively oligotrophic bacteria. However, 15 of these isolates were eventually obtained from dilution cultures that could initially be cultured only on very low-nutrient media and that could initially not form visible colonies on any of the agar media tested, indicating that these cultures contained obligately oligotrophic bacteria. It was concluded that the cells in these 15 dilution cultures had adapted to growth under laboratory conditions after several months of nutrient deprivation prior to isolation. From the North Sea experiment, it was concluded that the contribution of facultative oligotrophs and eutrophs to the total population was less than 1% and that while more than half of the population behaved as obligately oligotrophic bacteria upon first cultivation in the dilution culture media, around 50% could not be cultured at all. During one of the Resurrection Bay experiments, 53% of the dilution cultures obtained from samples diluted more than 2.5 × 105 times consisted of such obligate oligotrophs. These cultures invariably harbored a small rod-shaped bacterium with a mean cell volume of 0.05 to 0.06 μm3 and an apparent DNA content of 1 to 1.5 fg per cell. This cell type had the dimensions of ultramicrobacteria. Isolates of these ultramicrobacterial cultures that were eventually obtained on relatively high-nutrient agar plates were, with respect to cell volume and apparent DNA content, identical to the cells in the initially obligately oligotrophic bacterial dilution culture. Determination of kinetic parameters from one of these small rod-shaped strains revealed a high specific affinity for the uptake of mixed amino acids (a°A, 1,860 liters/g of cells per h), but not for glucose or alanine as the sole source of carbon and energy (a°A, ± 200 liters/g of cells per h). The ultramicrobial strains obtained are potentially a very important part of picoplankton biomass in the areas investigated.
Full text
PDF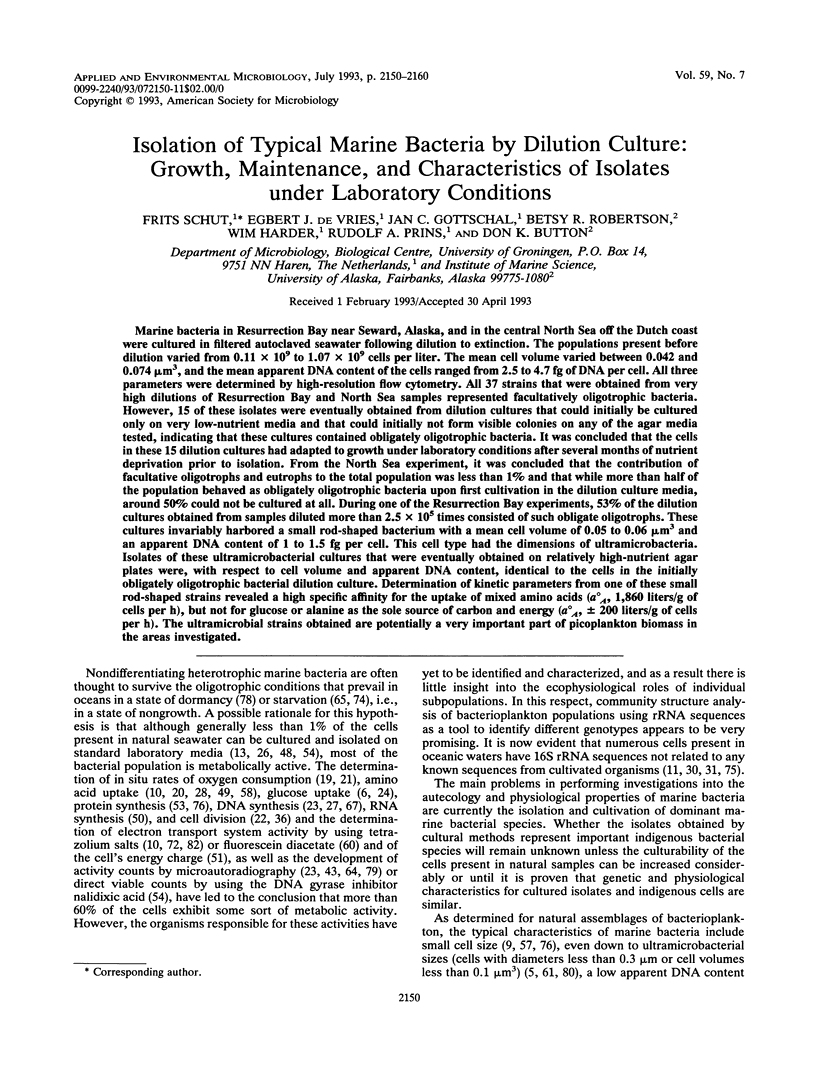
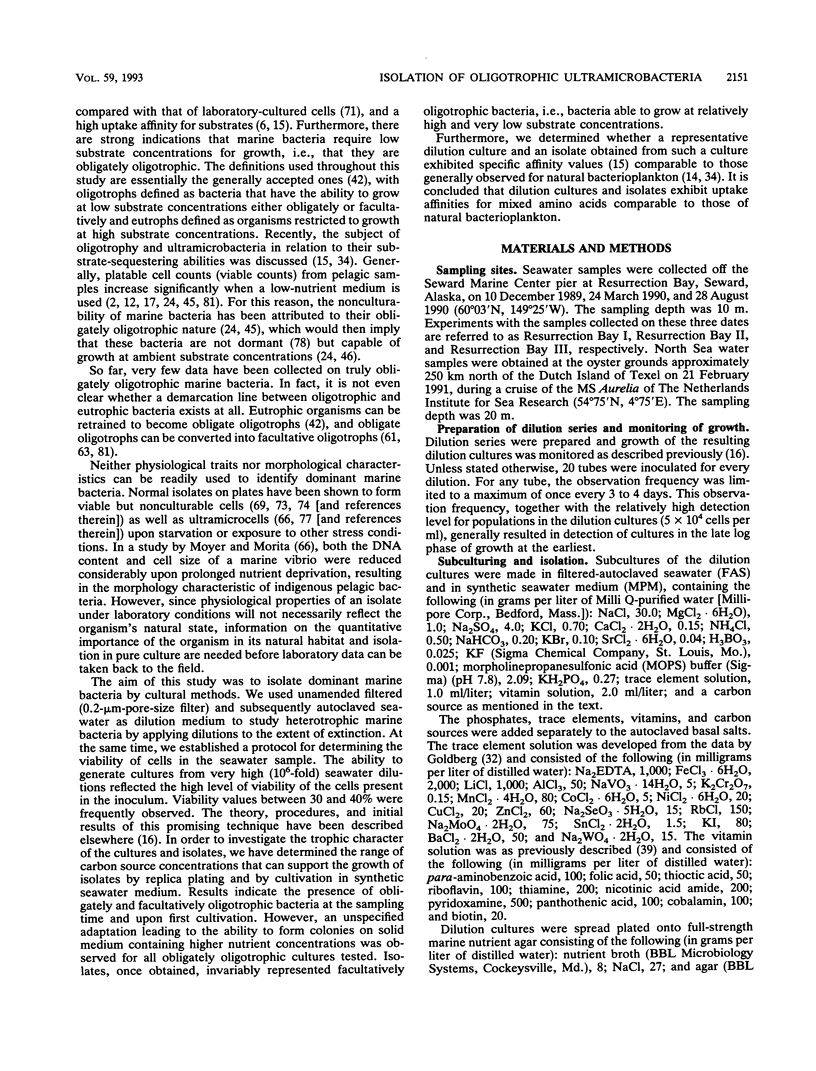
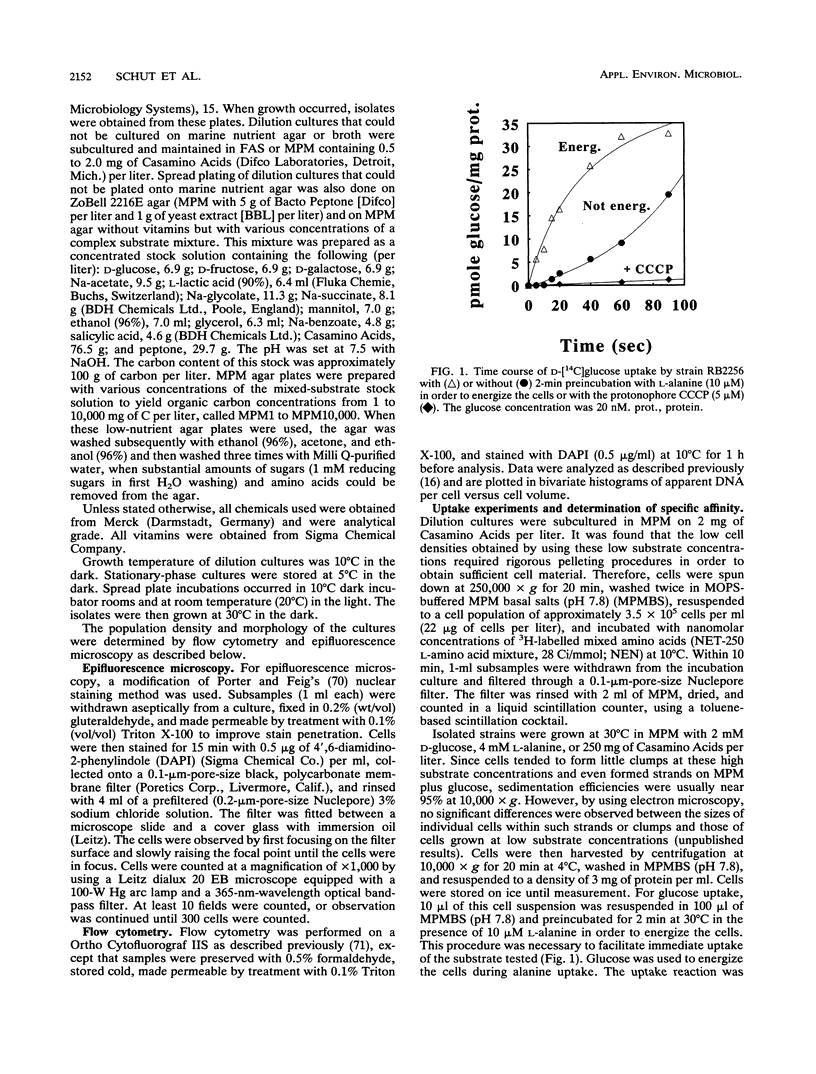
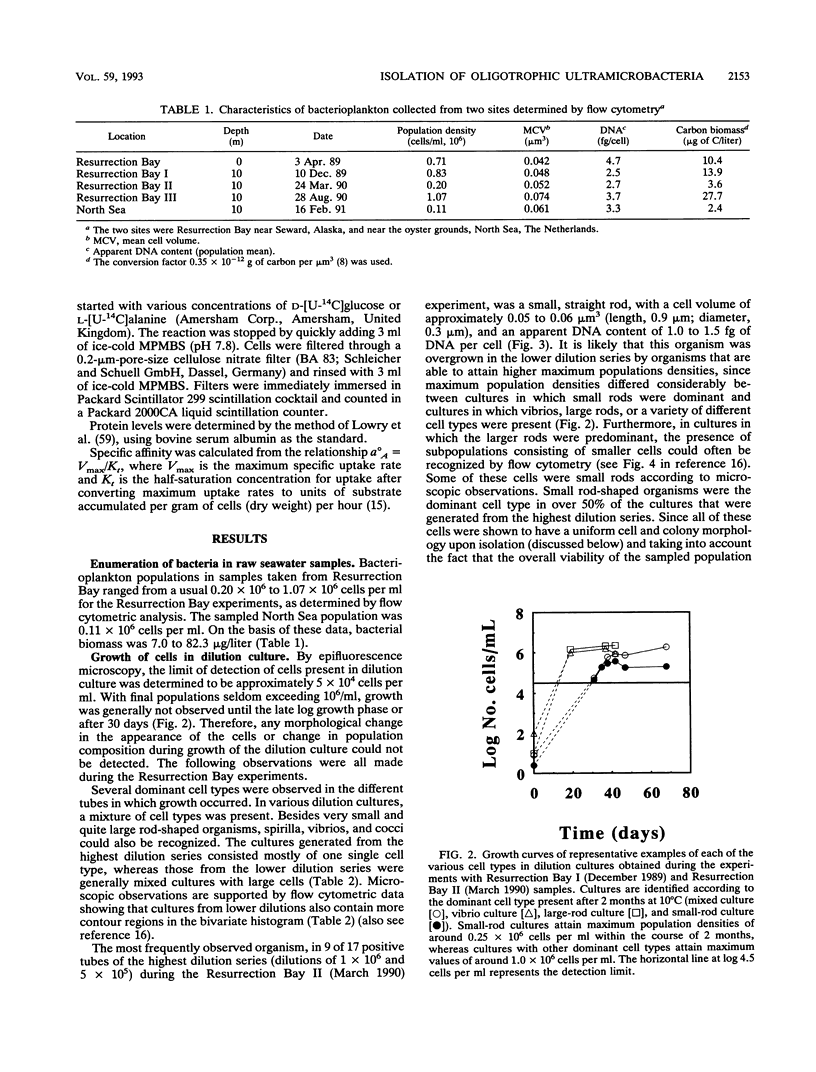
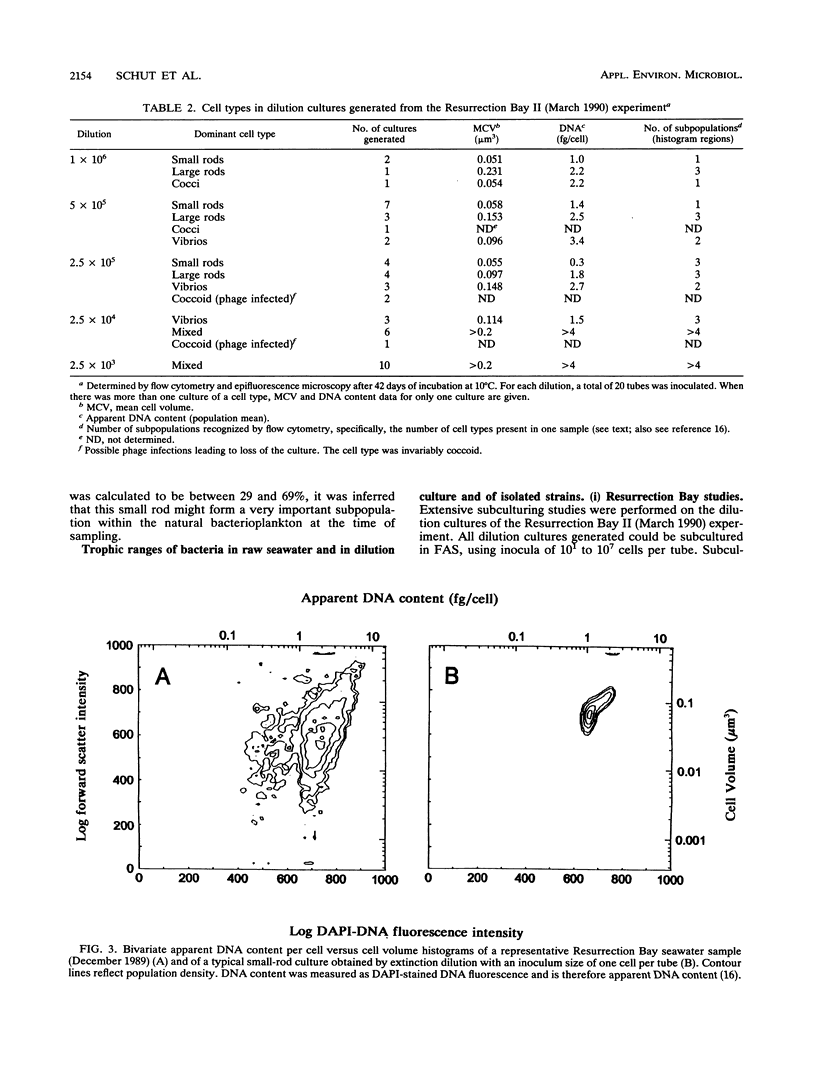
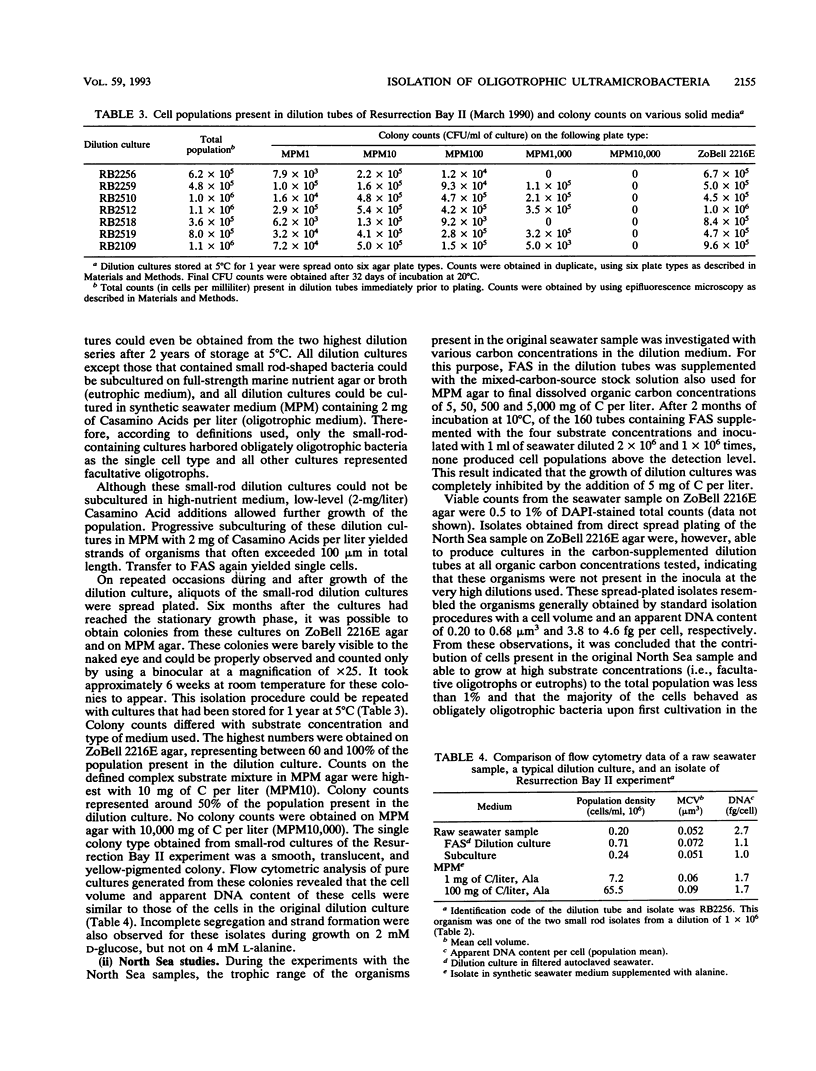
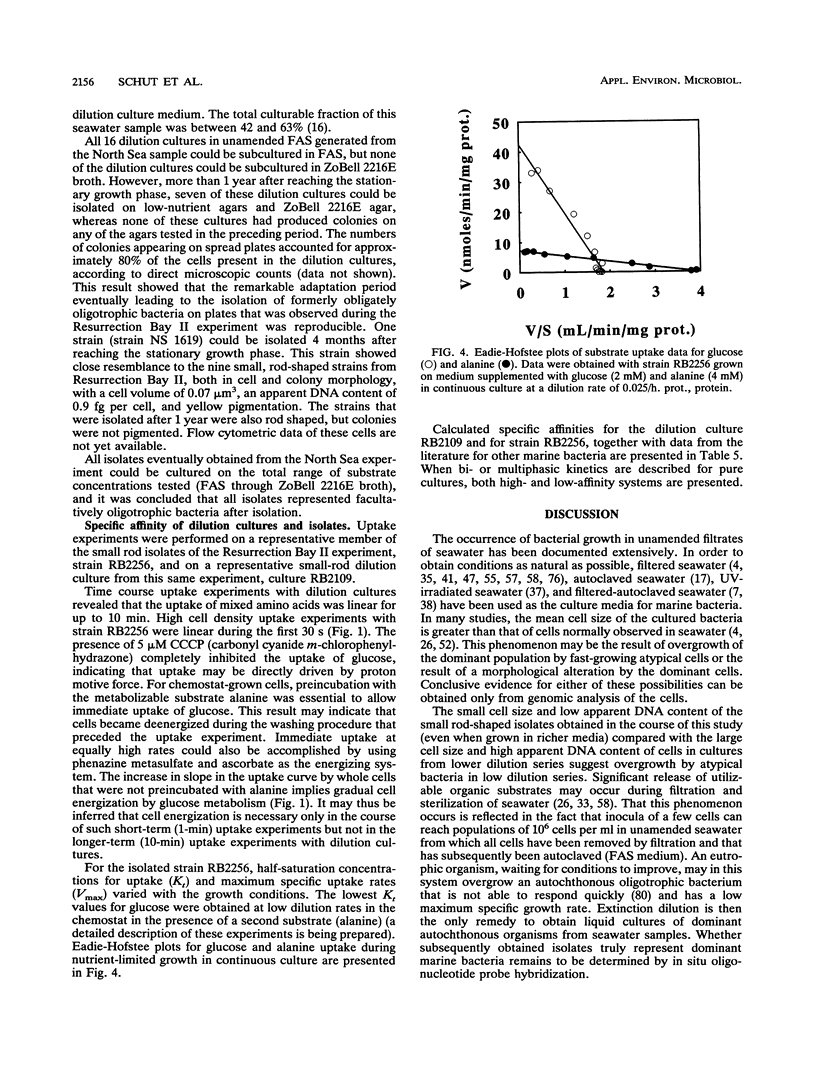
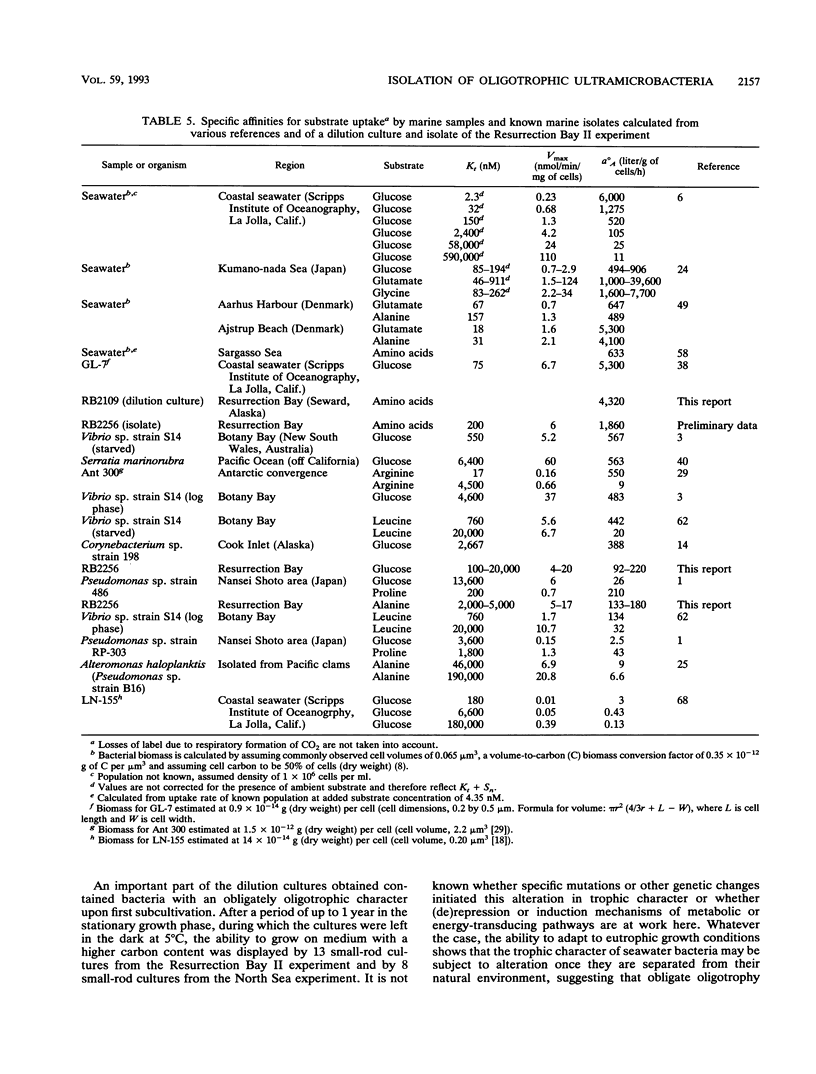
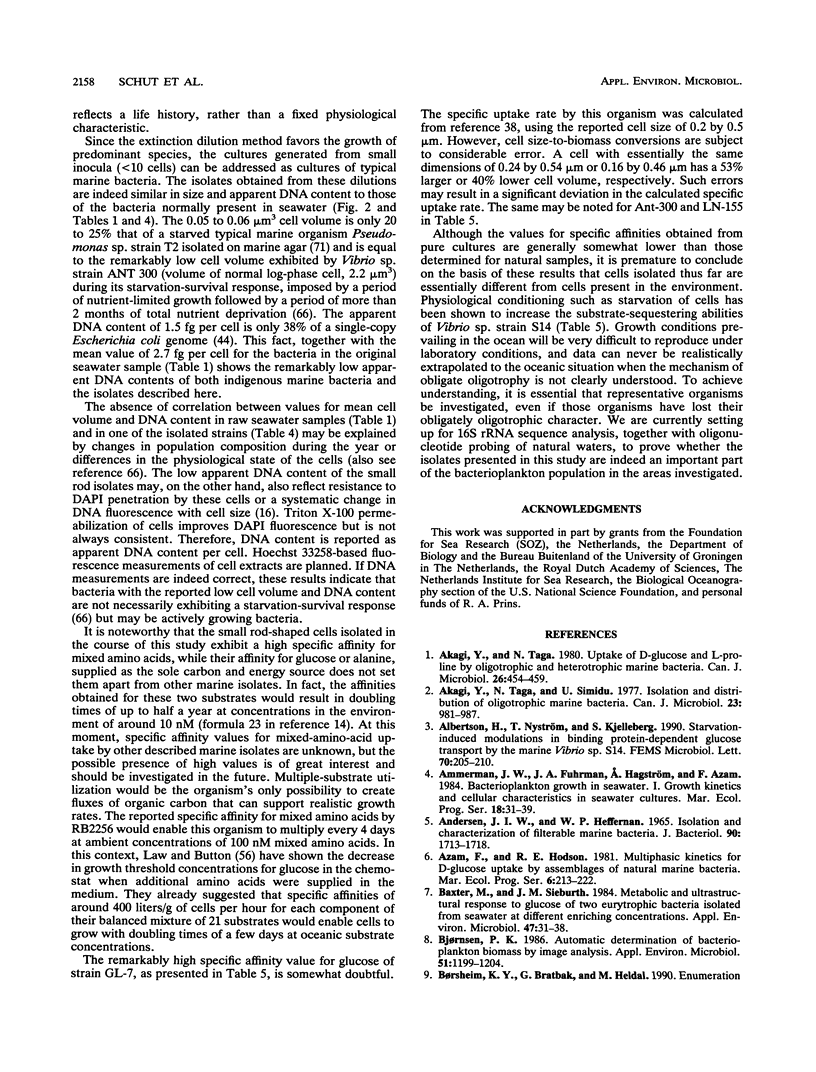
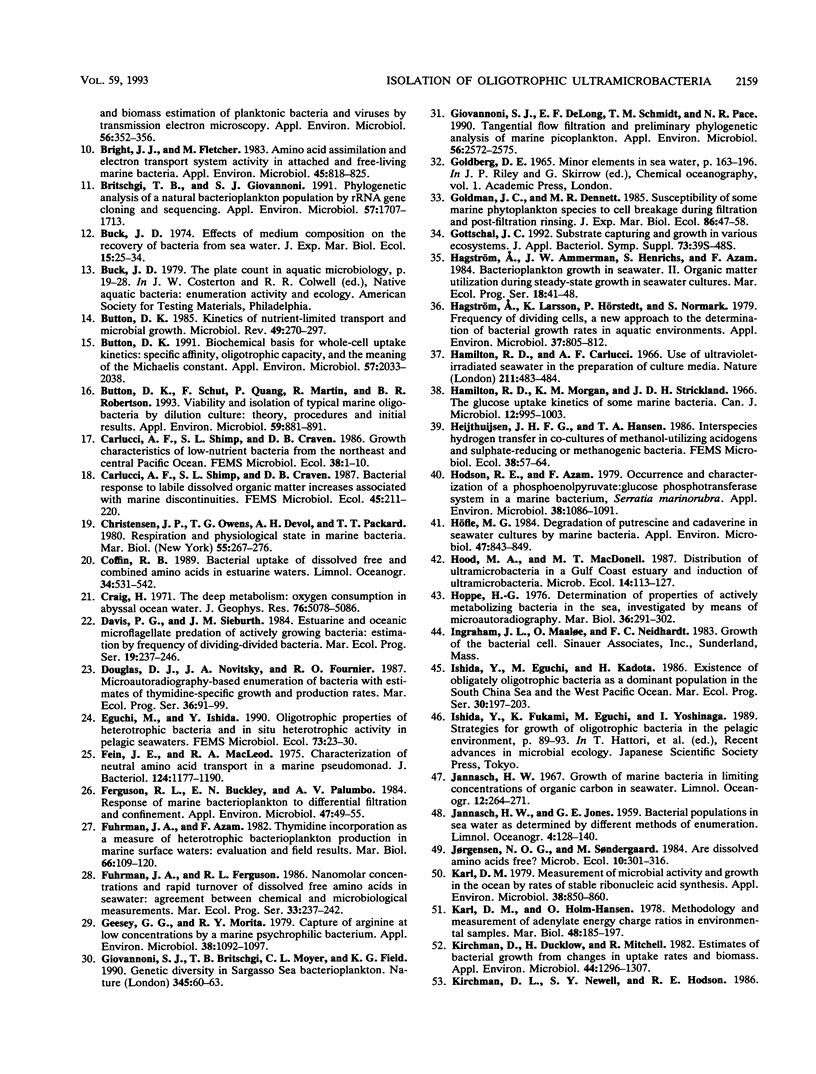
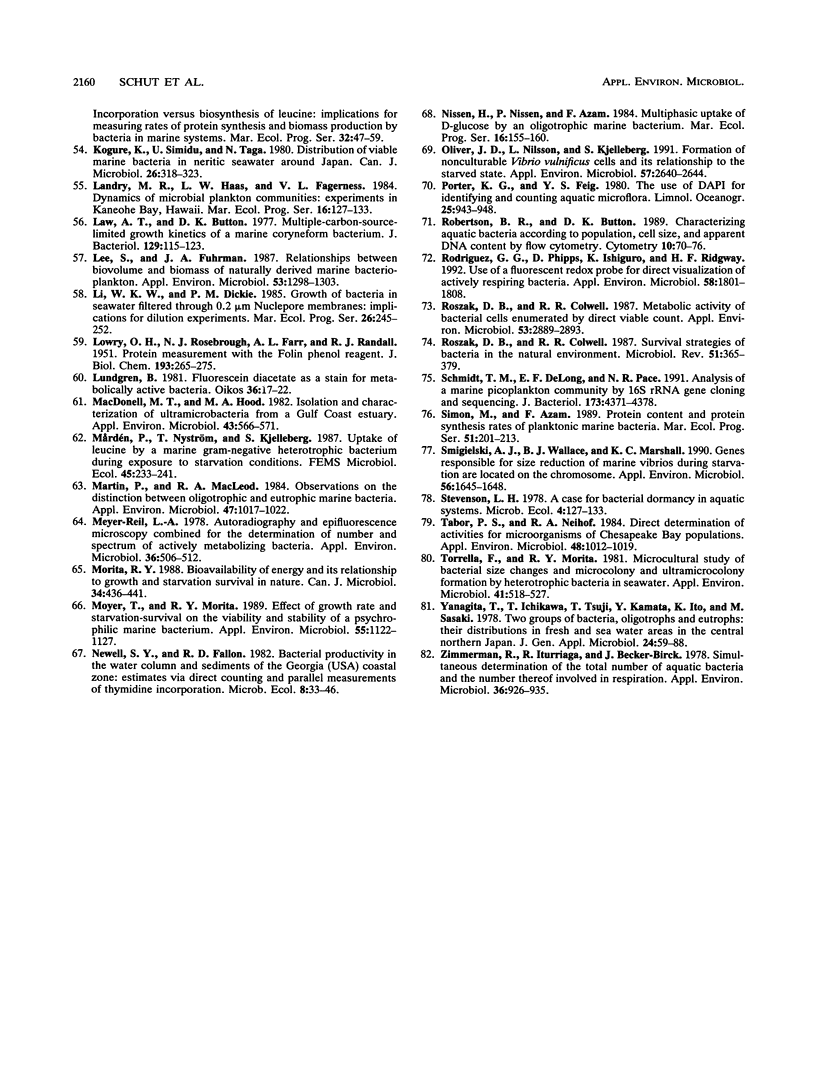
Selected References
These references are in PubMed. This may not be the complete list of references from this article.
- Akagi Y., Taga N. Uptake of D-glucose and L-proline by oligotrophic and heterotrophic marine bacteria. Can J Microbiol. 1980 Apr;26(4):454–459. doi: 10.1139/m80-075. [DOI] [PubMed] [Google Scholar]
- Albertson N. H., Nyström T., Kjelleberg S. Starvation-induced modulations in binding protein-dependent glucose transport by the marine Vibrio sp. S14. FEMS Microbiol Lett. 1990 Jul;58(2):205–209. doi: 10.1111/j.1574-6968.1990.tb13979.x. [DOI] [PubMed] [Google Scholar]
- Anderson J. I., Heffernan W. P. Isolation and characterization of filterable marine bacteria. J Bacteriol. 1965 Dec;90(6):1713–1718. doi: 10.1128/jb.90.6.1713-1718.1965. [DOI] [PMC free article] [PubMed] [Google Scholar]
- Aragi Y., Taga N., Simidu U. Isolation and distribution of oligotrophic marine bacteria. Can J Microbiol. 1977 Aug;23(8):981–987. doi: 10.1139/m77-146. [DOI] [PubMed] [Google Scholar]
- Baxter M., Sieburth J. M. Metabolic and ultrastructural response to glucose of two eurytrophic bacteria isolated from seawater at different enriching concentrations. Appl Environ Microbiol. 1984 Jan;47(1):31–38. doi: 10.1128/aem.47.1.31-38.1984. [DOI] [PMC free article] [PubMed] [Google Scholar]
- Bjørnsen P. K. Automatic determination of bacterioplankton biomass by image analysis. Appl Environ Microbiol. 1986 Jun;51(6):1199–1204. doi: 10.1128/aem.51.6.1199-1204.1986. [DOI] [PMC free article] [PubMed] [Google Scholar]
- Bright J. J., Fletcher M. Amino Acid assimilation and electron transport system activity in attached and free-living marine bacteria. Appl Environ Microbiol. 1983 Mar;45(3):818–825. doi: 10.1128/aem.45.3.818-825.1983. [DOI] [PMC free article] [PubMed] [Google Scholar]
- Britschgi T. B., Giovannoni S. J. Phylogenetic analysis of a natural marine bacterioplankton population by rRNA gene cloning and sequencing. Appl Environ Microbiol. 1991 Jun;57(6):1707–1713. doi: 10.1128/aem.57.6.1707-1713.1991. [DOI] [PMC free article] [PubMed] [Google Scholar]
- Button D. K. Biochemical basis for whole-cell uptake kinetics: specific affinity, oligotrophic capacity, and the meaning of the michaelis constant. Appl Environ Microbiol. 1991 Jul;57(7):2033–2038. doi: 10.1128/aem.57.7.2033-2038.1991. [DOI] [PMC free article] [PubMed] [Google Scholar]
- Button D. K. Kinetics of nutrient-limited transport and microbial growth. Microbiol Rev. 1985 Sep;49(3):270–297. doi: 10.1128/mr.49.3.270-297.1985. [DOI] [PMC free article] [PubMed] [Google Scholar]
- Button D. K., Schut F., Quang P., Martin R., Robertson B. R. Viability and isolation of marine bacteria by dilution culture: theory, procedures, and initial results. Appl Environ Microbiol. 1993 Mar;59(3):881–891. doi: 10.1128/aem.59.3.881-891.1993. [DOI] [PMC free article] [PubMed] [Google Scholar]
- Børsheim K. Y., Bratbak G., Heldal M. Enumeration and biomass estimation of planktonic bacteria and viruses by transmission electron microscopy. Appl Environ Microbiol. 1990 Feb;56(2):352–356. doi: 10.1128/aem.56.2.352-356.1990. [DOI] [PMC free article] [PubMed] [Google Scholar]
- Fein J. E., MacLeod R. A. Characterization of neutral amino acid transport in a marine pseudomonad. J Bacteriol. 1975 Dec;124(3):1177–1190. doi: 10.1128/jb.124.3.1177-1190.1975. [DOI] [PMC free article] [PubMed] [Google Scholar]
- Ferguson R. L., Buckley E. N., Palumbo A. V. Response of marine bacterioplankton to differential filtration and confinement. Appl Environ Microbiol. 1984 Jan;47(1):49–55. doi: 10.1128/aem.47.1.49-55.1984. [DOI] [PMC free article] [PubMed] [Google Scholar]
- Geesey G. G., Morita R. Y. Capture of arginine at low concentrations by a marine psychrophilic bacterium. Appl Environ Microbiol. 1979 Dec;38(6):1092–1097. doi: 10.1128/aem.38.6.1092-1097.1979. [DOI] [PMC free article] [PubMed] [Google Scholar]
- Giovannoni S. J., Britschgi T. B., Moyer C. L., Field K. G. Genetic diversity in Sargasso Sea bacterioplankton. Nature. 1990 May 3;345(6270):60–63. doi: 10.1038/345060a0. [DOI] [PubMed] [Google Scholar]
- Giovannoni S. J., DeLong E. F., Schmidt T. M., Pace N. R. Tangential flow filtration and preliminary phylogenetic analysis of marine picoplankton. Appl Environ Microbiol. 1990 Aug;56(8):2572–2575. doi: 10.1128/aem.56.8.2572-2575.1990. [DOI] [PMC free article] [PubMed] [Google Scholar]
- Gottschal J. C. Substrate capturing and growth in various ecosystems. Soc Appl Bacteriol Symp Ser. 1992;21:39S–48S. doi: 10.1111/j.1365-2672.1992.tb03623.x. [DOI] [PubMed] [Google Scholar]
- Hagström A., Larsson U., Hörstedt P., Normark S. Frequency of dividing cells, a new approach to the determination of bacterial growth rates in aquatic environments. Appl Environ Microbiol. 1979 May;37(5):805–812. doi: 10.1128/aem.37.5.805-812.1979. [DOI] [PMC free article] [PubMed] [Google Scholar]
- Hamilton R. D., Carlucci A. F. Use of ultra-violet-irradiated sea water in the preparation of culture media. Nature. 1966 Jul 30;211(5048):483–484. doi: 10.1038/211483a0. [DOI] [PubMed] [Google Scholar]
- Hamilton R. D., Morgan K. M., Strickland J. D. The glucose uptake kinetics of some marine bacteria. Can J Microbiol. 1966 Oct;12(5):995–1003. doi: 10.1139/m66-134. [DOI] [PubMed] [Google Scholar]
- Hodson R. E., Azam F. Occurrence and Characterization of a Phosphoenolpyruvate: Glucose Phosphotransferase System in a Marine Bacterium, Serratia marinorubra. Appl Environ Microbiol. 1979 Dec;38(6):1086–1091. doi: 10.1128/aem.38.6.1086-1091.1979. [DOI] [PMC free article] [PubMed] [Google Scholar]
- Höfle M. G. Degradation of putrescine and cadaverine in seawater cultures by marine bacteria. Appl Environ Microbiol. 1984 Apr;47(4):843–849. doi: 10.1128/aem.47.4.843-849.1984. [DOI] [PMC free article] [PubMed] [Google Scholar]
- Karl D. M. Measurement of microbial activity and growth in the ocean by rates of stable ribonucleic Acid synthesis. Appl Environ Microbiol. 1979 Nov;38(5):850–860. doi: 10.1128/aem.38.5.850-860.1979. [DOI] [PMC free article] [PubMed] [Google Scholar]
- Kirchman D., Ducklow H., Mitchell R. Estimates of bacterial growth from changes in uptake rates and biomass. Appl Environ Microbiol. 1982 Dec;44(6):1296–1307. doi: 10.1128/aem.44.6.1296-1307.1982. [DOI] [PMC free article] [PubMed] [Google Scholar]
- Kogure K., Simidu U., Taga N. Distribution of viable marine bacteria in neritic seawater around Japan. Can J Microbiol. 1980 Mar;26(3):318–323. doi: 10.1139/m80-052. [DOI] [PubMed] [Google Scholar]
- LOWRY O. H., ROSEBROUGH N. J., FARR A. L., RANDALL R. J. Protein measurement with the Folin phenol reagent. J Biol Chem. 1951 Nov;193(1):265–275. [PubMed] [Google Scholar]
- Law A. T., Button D. K. Multiple-carbon-source-limited growth kinetics of a marine coryneform bacterium. J Bacteriol. 1977 Jan;129(1):115–123. doi: 10.1128/jb.129.1.115-123.1977. [DOI] [PMC free article] [PubMed] [Google Scholar]
- Lee S., Fuhrman J. A. Relationships between Biovolume and Biomass of Naturally Derived Marine Bacterioplankton. Appl Environ Microbiol. 1987 Jun;53(6):1298–1303. doi: 10.1128/aem.53.6.1298-1303.1987. [DOI] [PMC free article] [PubMed] [Google Scholar]
- Macdonell M. T., Hood M. A. Isolation and characterization of ultramicrobacteria from a gulf coast estuary. Appl Environ Microbiol. 1982 Mar;43(3):566–571. doi: 10.1128/aem.43.3.566-571.1982. [DOI] [PMC free article] [PubMed] [Google Scholar]
- Martin P., Macleod R. A. Observations on the distinction between oligotrophic and eutrophic marine bacteria. Appl Environ Microbiol. 1984 May;47(5):1017–1022. doi: 10.1128/aem.47.5.1017-1022.1984. [DOI] [PMC free article] [PubMed] [Google Scholar]
- Meyer-Reil L. A. Autoradiography and epifluorescence microscopy combined for the determination of number and spectrum of actively metabolizing bacteria in natural water. Appl Environ Microbiol. 1978 Sep;36(3):506–512. doi: 10.1128/aem.36.3.506-512.1978. [DOI] [PMC free article] [PubMed] [Google Scholar]
- Moyer C. L., Morita R. Y. Effect of growth rate and starvation-survival on the viability and stability of a psychrophilic marine bacterium. Appl Environ Microbiol. 1989 May;55(5):1122–1127. doi: 10.1128/aem.55.5.1122-1127.1989. [DOI] [PMC free article] [PubMed] [Google Scholar]
- Oliver J. D., Nilsson L., Kjelleberg S. Formation of nonculturable Vibrio vulnificus cells and its relationship to the starvation state. Appl Environ Microbiol. 1991 Sep;57(9):2640–2644. doi: 10.1128/aem.57.9.2640-2644.1991. [DOI] [PMC free article] [PubMed] [Google Scholar]
- Robertson B. R., Button D. K. Characterizing aquatic bacteria according to population, cell size, and apparent DNA content by flow cytometry. Cytometry. 1989 Jan;10(1):70–76. doi: 10.1002/cyto.990100112. [DOI] [PubMed] [Google Scholar]
- Rodriguez G. G., Phipps D., Ishiguro K., Ridgway H. F. Use of a fluorescent redox probe for direct visualization of actively respiring bacteria. Appl Environ Microbiol. 1992 Jun;58(6):1801–1808. doi: 10.1128/aem.58.6.1801-1808.1992. [DOI] [PMC free article] [PubMed] [Google Scholar]
- Roszak D. B., Colwell R. R. Metabolic activity of bacterial cells enumerated by direct viable count. Appl Environ Microbiol. 1987 Dec;53(12):2889–2893. doi: 10.1128/aem.53.12.2889-2893.1987. [DOI] [PMC free article] [PubMed] [Google Scholar]
- Roszak D. B., Colwell R. R. Survival strategies of bacteria in the natural environment. Microbiol Rev. 1987 Sep;51(3):365–379. doi: 10.1128/mr.51.3.365-379.1987. [DOI] [PMC free article] [PubMed] [Google Scholar]
- Schmidt T. M., DeLong E. F., Pace N. R. Analysis of a marine picoplankton community by 16S rRNA gene cloning and sequencing. J Bacteriol. 1991 Jul;173(14):4371–4378. doi: 10.1128/jb.173.14.4371-4378.1991. [DOI] [PMC free article] [PubMed] [Google Scholar]
- Smigielski A. J., Wallace B., Marshall K. C. Genes Responsible for Size Reduction of Marine Vibrios during Starvation Are Located on the Chromosome. Appl Environ Microbiol. 1990 Jun;56(6):1645–1648. doi: 10.1128/aem.56.6.1645-1648.1990. [DOI] [PMC free article] [PubMed] [Google Scholar]
- Tabor P. S., Neihof R. A. Direct determination of activities for microorganisms of chesapeake bay populations. Appl Environ Microbiol. 1984 Nov;48(5):1012–1019. doi: 10.1128/aem.48.5.1012-1019.1984. [DOI] [PMC free article] [PubMed] [Google Scholar]
- Torrella F., Morita R. Y. Microcultural study of bacterial size changes and microcolony and ultramicrocolony formation by heterotrophic bacteria in seawater. Appl Environ Microbiol. 1981 Feb;41(2):518–527. doi: 10.1128/aem.41.2.518-527.1981. [DOI] [PMC free article] [PubMed] [Google Scholar]
- Zimmermann R., Iturriaga R., Becker-Birck J. Simultaneous determination of the total number of aquatic bacteria and the number thereof involved in respiration. Appl Environ Microbiol. 1978 Dec;36(6):926–935. doi: 10.1128/aem.36.6.926-935.1978. [DOI] [PMC free article] [PubMed] [Google Scholar]