Abstract
The transport of glucose across the bacterial cell membrane of Thermoanaerobacter thermosulfuricus (Clostridium thermohydrosulfuricum) Rt8.B1 was governed by a permease which did not catalyze concomitant substrate transport and phosphorylation and thus was not a phosphoenolpyruvate-dependent phosphotransferase. Glucose uptake was carrier mediated, could not be driven by an artificial membrane potential (Δψ) in the presence or absence of sodium, and was not sensitive to inhibitors which dissipate the proton motive force (Δp; tetrachlorosalicylanilide, N,N-dicyclohexylcarboiimide, and 2,4-dinitrophenol), and no uptake of the nonmetabolizable analog 2-deoxyglucose could be demonstrated. The glucokinase apparent Km for glucose (0.21 mM) was similar to the Kt (affinity constant) for glucose uptake (0.15 mM), suggesting that glucokinase controls the rate of glucose uptake. Inhibitors of ATP synthesis (iodoacetate and sodium fluoride) also inhibited glucose uptake, and this effect was due to a reduction in the level of ATP available to glucokinase for glucose phosphorylation. These results indicated that T. thermosulfuricus Rt8.B1 lacks a concentrative uptake system for glucose and that uptake is via facilitated diffusion, followed by ATP-dependent phosphorylation by glucokinase. In T. thermosulfuricus Rt8.B1, glucose is metabolized by the Embden-Meyerhof-Parnas pathway, which yields 2 mol of ATP (G. M. Cook, unpublished data). Since only 1 mol of ATP is used to transport 1 mol of glucose, the energetics of this system are therefore similar to those found in bacteria which possess a phosphotransferase.
Full text
PDF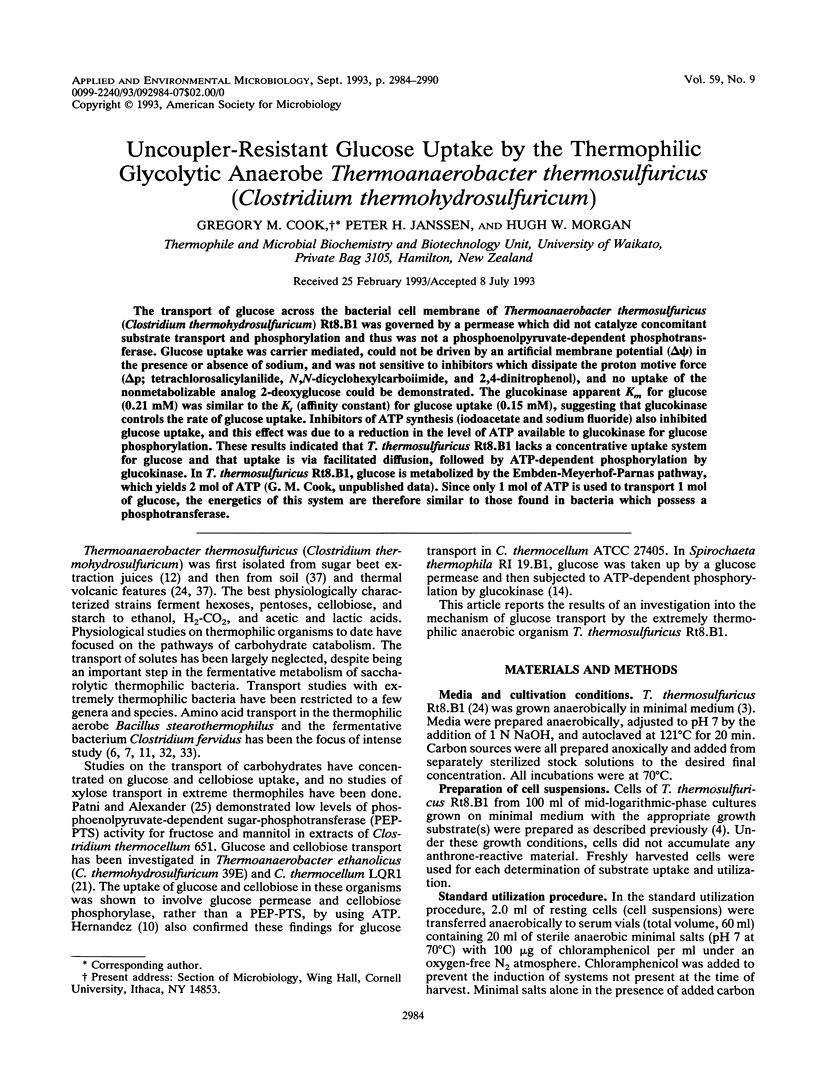
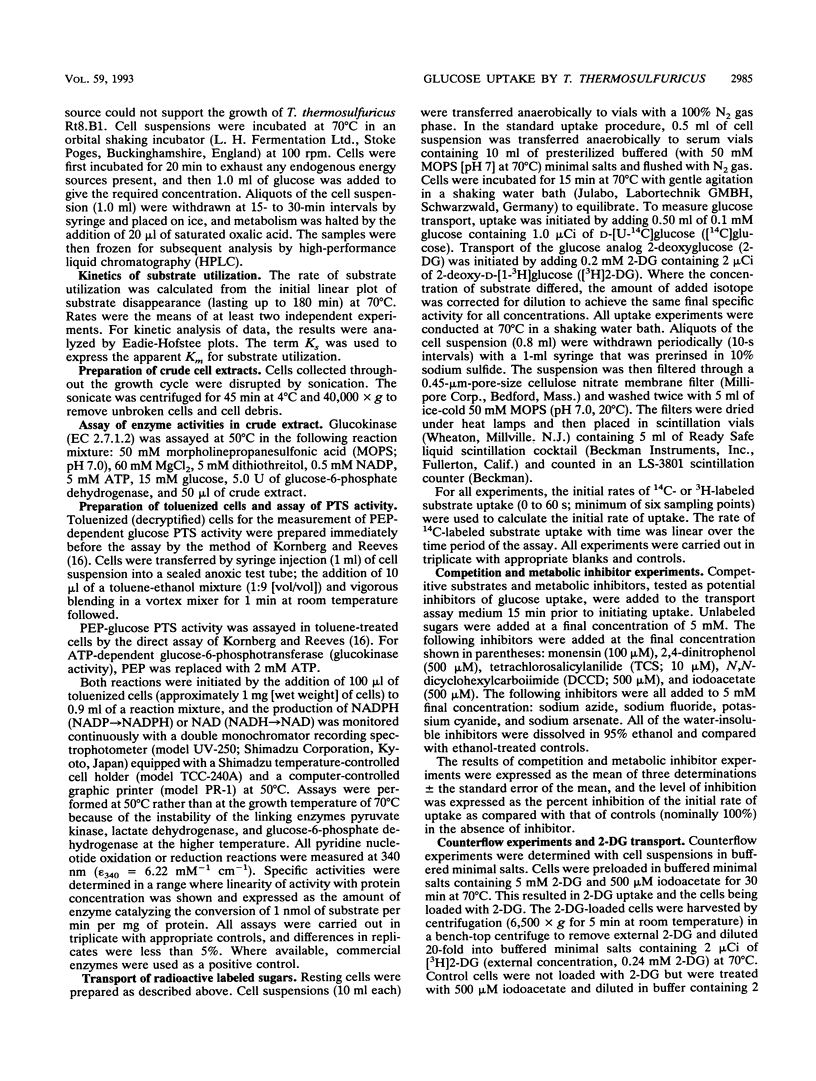
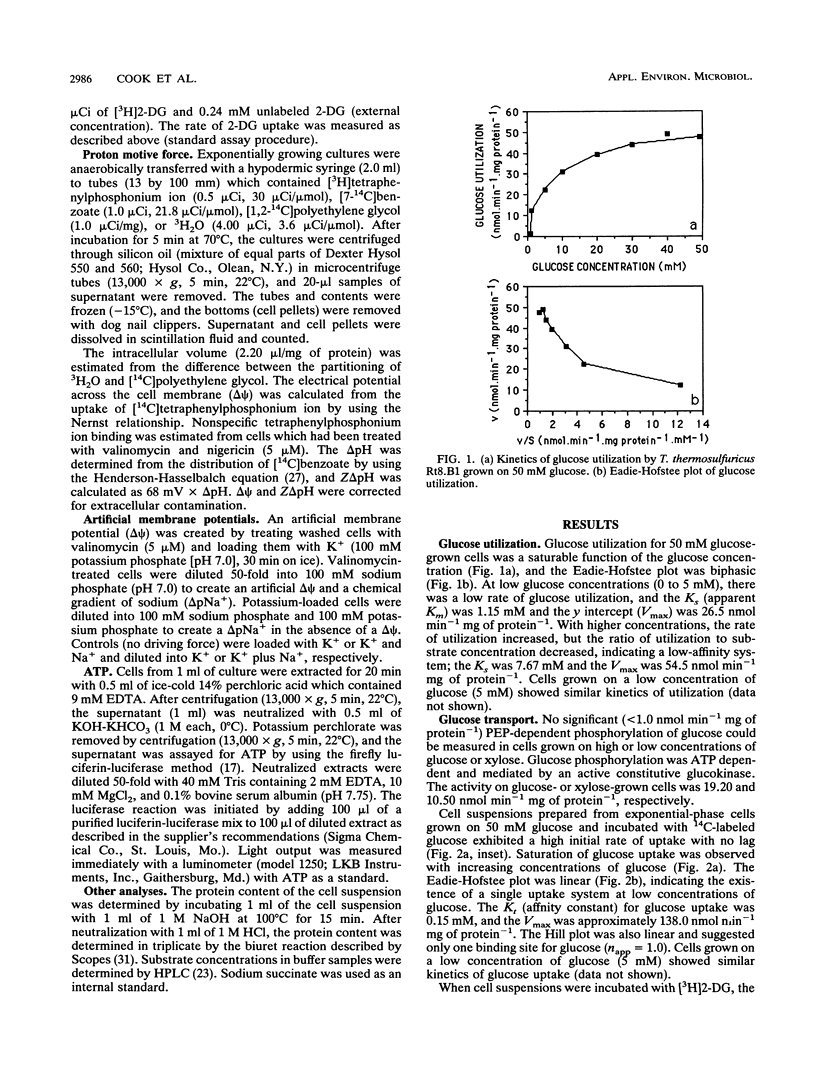
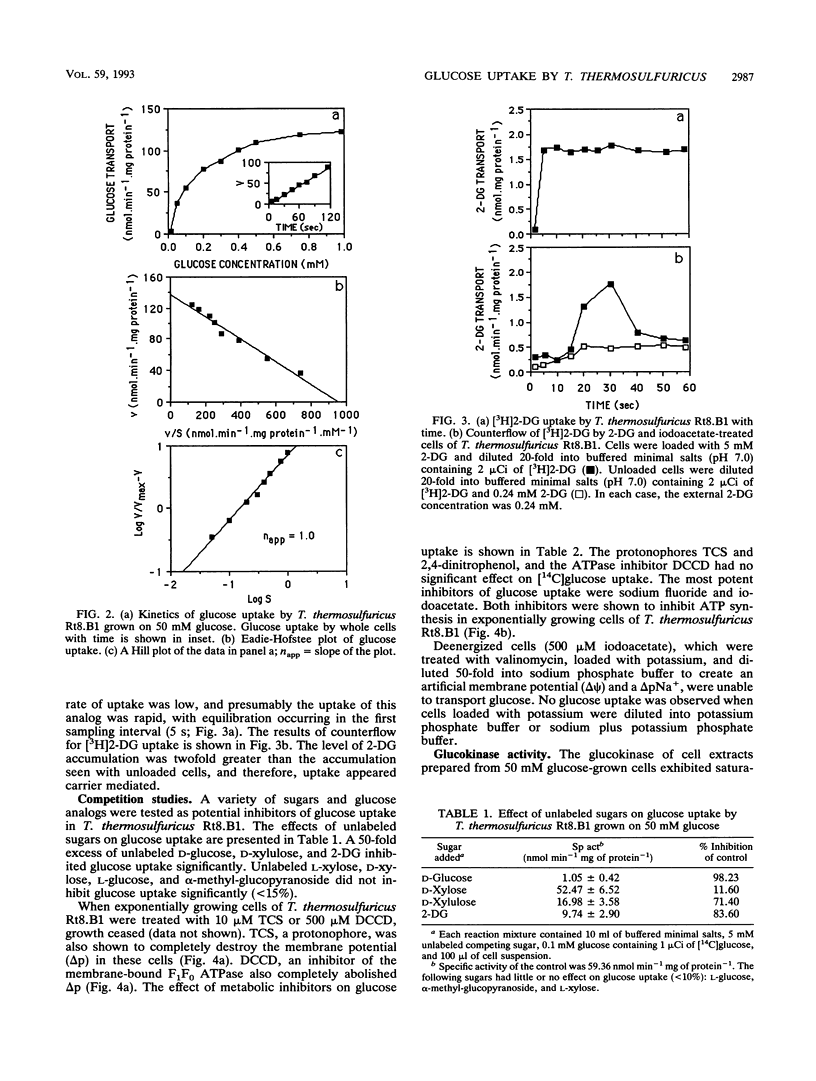
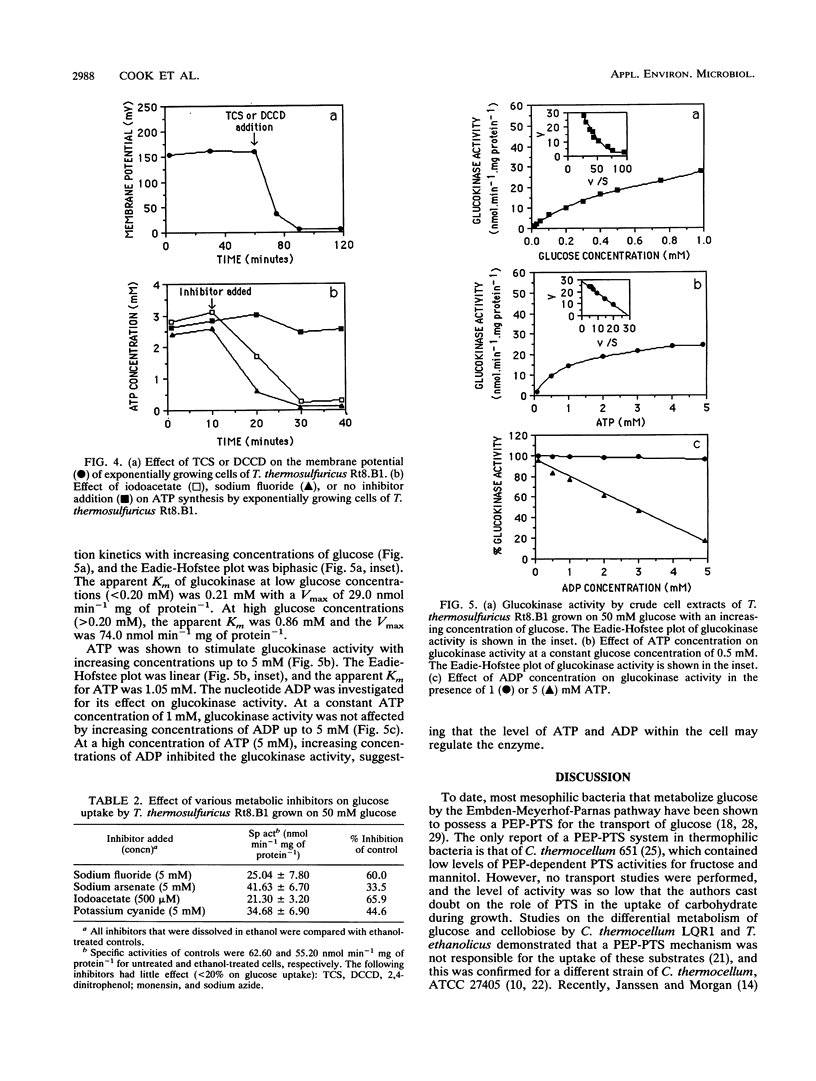
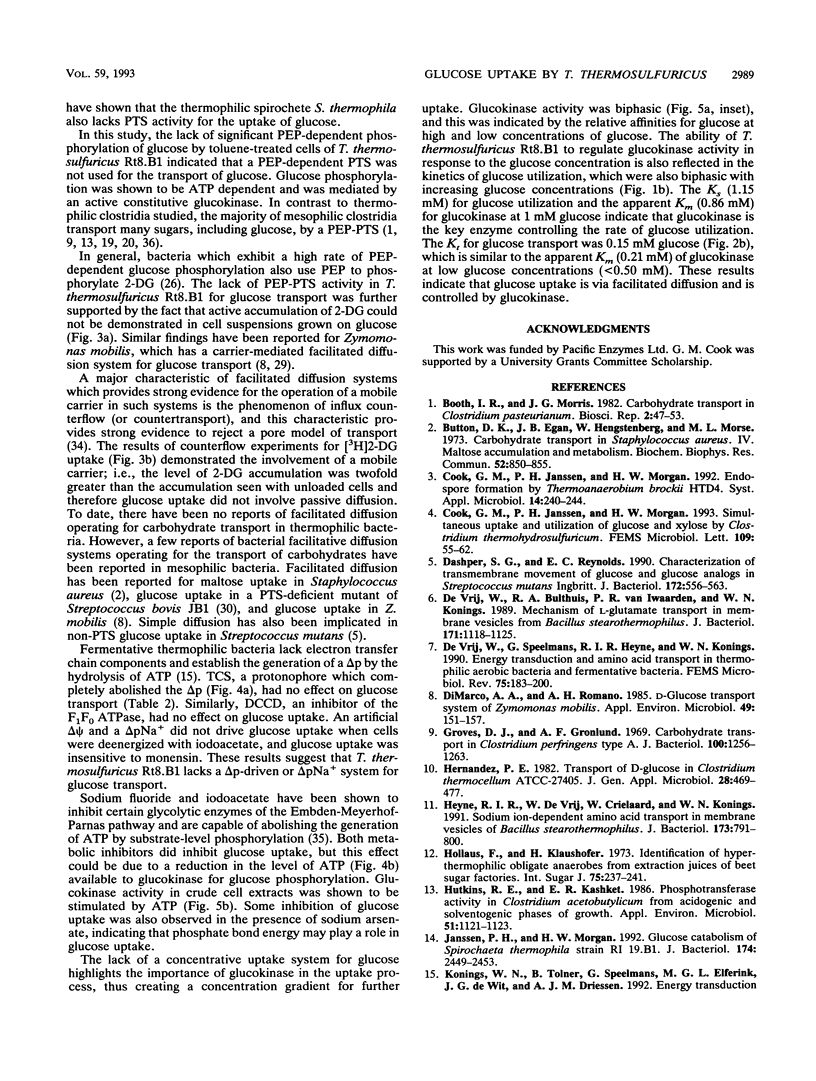
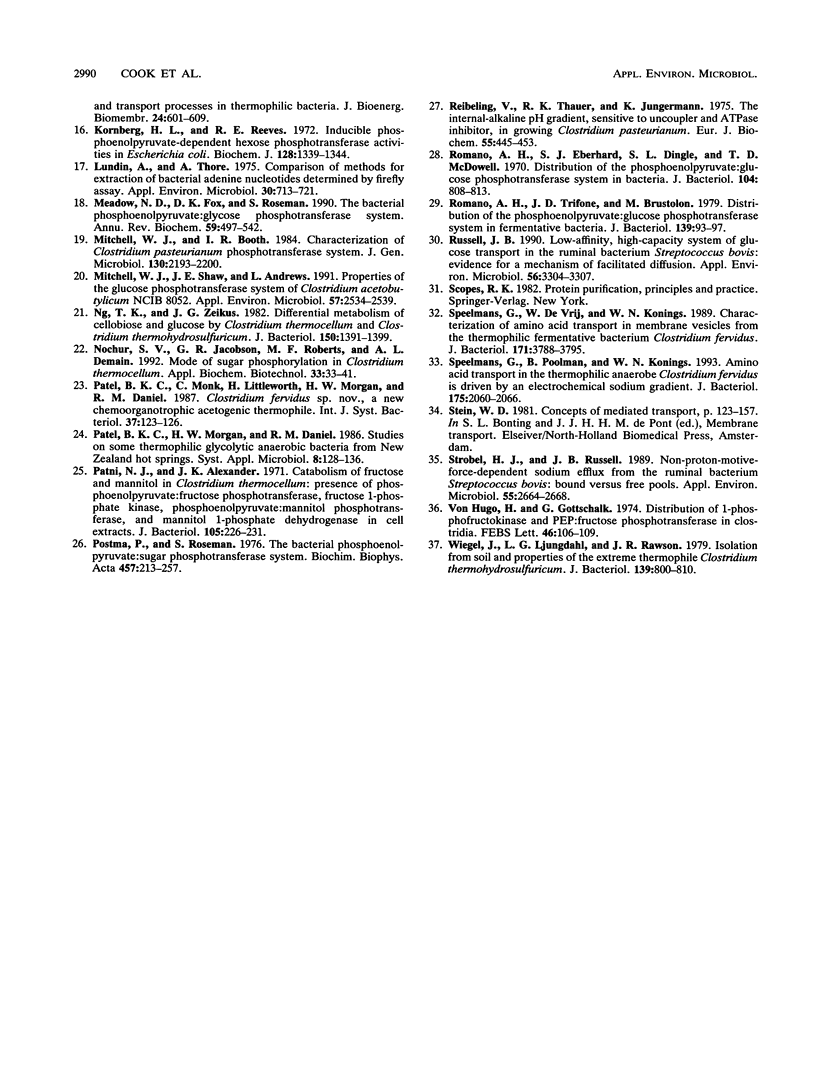
Selected References
These references are in PubMed. This may not be the complete list of references from this article.
- Booth I. R., Morris J. G. Carbohydrate transport in Clostridium pasteurianum. Biosci Rep. 1982 Jan;2(1):47–53. doi: 10.1007/BF01142198. [DOI] [PubMed] [Google Scholar]
- Button D. K., Egan J. B., Hengstenberg W., Morse M. L. Carbohydrate transport in Staphylococcus aureus. IV. Maltose accumulation and metabolism. Biochem Biophys Res Commun. 1973 Jun 8;52(3):850–855. doi: 10.1016/0006-291x(73)91015-2. [DOI] [PubMed] [Google Scholar]
- Dashper S. G., Reynolds E. C. Characterization of transmembrane movement of glucose and glucose analogs in Streptococcus mutants Ingbritt. J Bacteriol. 1990 Feb;172(2):556–563. doi: 10.1128/jb.172.2.556-563.1990. [DOI] [PMC free article] [PubMed] [Google Scholar]
- Dimarco A. A., Romano A. H. d-Glucose Transport System of Zymomonas mobilis. Appl Environ Microbiol. 1985 Jan;49(1):151–157. doi: 10.1128/aem.49.1.151-157.1985. [DOI] [PMC free article] [PubMed] [Google Scholar]
- Groves D. J., Gronlund A. F. Carbohydrate transport in Clostridium perfringens type A. J Bacteriol. 1969 Dec;100(3):1256–1263. doi: 10.1128/jb.100.3.1256-1263.1969. [DOI] [PMC free article] [PubMed] [Google Scholar]
- Heyne R. I., de Vrij W., Crielaard W., Konings W. N. Sodium ion-dependent amino acid transport in membrane vesicles of Bacillus stearothermophilus. J Bacteriol. 1991 Jan;173(2):791–800. doi: 10.1128/jb.173.2.791-800.1991. [DOI] [PMC free article] [PubMed] [Google Scholar]
- Hutkins R. W., Kashket E. R. Phosphotransferase Activity in Clostridium acetobutylicum from Acidogenic and Solventogenic Phases of Growth. Appl Environ Microbiol. 1986 May;51(5):1121–1123. doi: 10.1128/aem.51.5.1121-1123.1986. [DOI] [PMC free article] [PubMed] [Google Scholar]
- Janssen P. H., Morgan H. W. Glucose catabolism by Spirochaeta thermophila RI 19.B1. J Bacteriol. 1992 Apr;174(8):2449–2453. doi: 10.1128/jb.174.8.2449-2453.1992. [DOI] [PMC free article] [PubMed] [Google Scholar]
- Kornberg H. L., Reeves R. E. Inducible phosphoenolpyruvate-dependent hexose phosphotransferase activities in Escherichia coli. Biochem J. 1972 Aug;128(5):1339–1344. doi: 10.1042/bj1281339. [DOI] [PMC free article] [PubMed] [Google Scholar]
- Lundin A., Thore A. Comparison of methods for extraction of bacterial adenine nucleotides determined by firefly assay. Appl Microbiol. 1975 Nov;30(5):713–721. doi: 10.1128/am.30.5.713-721.1975. [DOI] [PMC free article] [PubMed] [Google Scholar]
- Meadow N. D., Fox D. K., Roseman S. The bacterial phosphoenolpyruvate: glycose phosphotransferase system. Annu Rev Biochem. 1990;59:497–542. doi: 10.1146/annurev.bi.59.070190.002433. [DOI] [PubMed] [Google Scholar]
- Mitchell W. J., Shaw J. E., Andrews L. Properties of the glucose phosphotransferase system of Clostridium acetobutylicum NCIB 8052. Appl Environ Microbiol. 1991 Sep;57(9):2534–2539. doi: 10.1128/aem.57.9.2534-2539.1991. [DOI] [PMC free article] [PubMed] [Google Scholar]
- Ng T. K., Zeikus J. G. Differential metabolism of cellobiose and glucose by Clostridium thermocellum and Clostridium thermohydrosulfuricum. J Bacteriol. 1982 Jun;150(3):1391–1399. doi: 10.1128/jb.150.3.1391-1399.1982. [DOI] [PMC free article] [PubMed] [Google Scholar]
- Patni N. J., Alexander J. K. Catabolism of fructose and mannitol in Clostridium thermocellum: presence of phosphoenolpyruvate: fructose phosphotransferase, fructose 1-phosphate kinase, phosphoenolpyruvate: mannitol phosphotransferase, and mannitol 1-phosphate dehydrogenase in cell extracts. J Bacteriol. 1971 Jan;105(1):226–231. doi: 10.1128/jb.105.1.226-231.1971. [DOI] [PMC free article] [PubMed] [Google Scholar]
- Postma P. W., Roseman S. The bacterial phosphoenolpyruvate: sugar phosphotransferase system. Biochim Biophys Acta. 1976 Dec 14;457(3-4):213–257. doi: 10.1016/0304-4157(76)90001-0. [DOI] [PubMed] [Google Scholar]
- Riebeling V., Thauer R. K., Jungermann K. The internal-alkaline pH gradient, sensitive to uncoupler and ATPase inhibitor, in growing Clostridium pasteurianum. Eur J Biochem. 1975 Jul 1;55(2):445–453. doi: 10.1111/j.1432-1033.1975.tb02181.x. [DOI] [PubMed] [Google Scholar]
- Romano A. H., Eberhard S. J., Dingle S. L., McDowell T. D. Distribution of the phosphoenolpyruvate: glucose phosphotransferase system in bacteria. J Bacteriol. 1970 Nov;104(2):808–813. doi: 10.1128/jb.104.2.808-813.1970. [DOI] [PMC free article] [PubMed] [Google Scholar]
- Romano A. H., Trifone J. D., Brustolon M. Distribution of the phosphoenolpyruvate:glucose phosphotransferase system in fermentative bacteria. J Bacteriol. 1979 Jul;139(1):93–97. doi: 10.1128/jb.139.1.93-97.1979. [DOI] [PMC free article] [PubMed] [Google Scholar]
- Russell J. B. Low-affinity, high-capacity system of glucose transport in the ruminal bacterium Streptococcus bovis: evidence for a mechanism of facilitated diffusion. Appl Environ Microbiol. 1990 Nov;56(11):3304–3307. doi: 10.1128/aem.56.11.3304-3307.1990. [DOI] [PMC free article] [PubMed] [Google Scholar]
- Speelmans G., Poolman B., Konings W. N. Amino acid transport in the thermophilic anaerobe Clostridium fervidus is driven by an electrochemical sodium gradient. J Bacteriol. 1993 Apr;175(7):2060–2066. doi: 10.1128/jb.175.7.2060-2066.1993. [DOI] [PMC free article] [PubMed] [Google Scholar]
- Speelmans G., de Vrij W., Konings W. N. Characterization of amino acid transport in membrane vesicles from the thermophilic fermentative bacterium Clostridium fervidus. J Bacteriol. 1989 Jul;171(7):3788–3795. doi: 10.1128/jb.171.7.3788-3795.1989. [DOI] [PMC free article] [PubMed] [Google Scholar]
- Strobel H. J., Russell J. B. Non-proton-motive-force-dependent sodium efflux from the ruminal bacterium Streptococcus bovis: bound versus free pools. Appl Environ Microbiol. 1989 Oct;55(10):2664–2668. doi: 10.1128/aem.55.10.2664-2668.1989. [DOI] [PMC free article] [PubMed] [Google Scholar]
- Wiegel J., Ljungdahl L. G., Rawson J. R. Isolation from soil and properties of the extreme thermophile Clostridium thermohydrosulfuricum. J Bacteriol. 1979 Sep;139(3):800–810. doi: 10.1128/jb.139.3.800-810.1979. [DOI] [PMC free article] [PubMed] [Google Scholar]
- de Vrij W., Bulthuis R. A., van Iwaarden P. R., Konings W. N. Mechanism of L-glutamate transport in membrane vesicles from Bacillus stearothermophilus. J Bacteriol. 1989 Feb;171(2):1118–1125. doi: 10.1128/jb.171.2.1118-1125.1989. [DOI] [PMC free article] [PubMed] [Google Scholar]
- von Hugo H., Gottschalk G. Distribution of 1-phosphofructokinase and PEP:fructose phosphotransferase activity in Clostridia. FEBS Lett. 1974 Sep 15;46(1):106–108. doi: 10.1016/0014-5793(74)80345-5. [DOI] [PubMed] [Google Scholar]