Abstract
The acetogenic bacterium Clostridium thermoaceticum ATCC 39073 grew at the expense of the two-carbon substrates oxalate and glyoxylate. Other two-carbon substrates (acetaldehyde, acetate, ethanol, ethylene glycol, glycolaldehyde, glycolate, and glyoxal) were not growth supportive. Growth increased linearly with increasing substrate concentrations up to 45 mM oxalate and glyoxylate, and supplemental CO2 was not required for growth. Oxalate and glyoxylate yielded 4.9 and 9.4 g, respectively, of cell biomass (dry weight) per mol of substrate utilized. Acetate was the major reduced end product recovered from oxalate and glyoxylate cultures. 14C labeling studies showed that oxalate was subject to decarboxylation, and product analysis indicated that oxalate was utilized by the following reaction: 4-OOC-COO- + 5H2O → CH3COO- + 6HCO3- + OH-. Oxalate- and glyoxylate-dependent growth produced lower acetate concentrations per unit of cell biomass synthesized than did H2-, CO-, methanol-, formate-, O-methyl-, or glucose-dependent growth. Protein profiles of oxalate-grown cells were dissimilar from protein profiles of glyoxylate-, CO-, or formate-grown cells, suggesting induction of new proteins for the utilization of oxalate. C. thermoaceticum DSM 2955 and Clostridium thermoautotrophicum JW 701/3 also grew at the expense of oxalate and glyoxylate. However, oxalate and glyoxylate did not support the growth of C. thermoaceticum OMD (a nonautotrophic strain) or six other species of acetogenic bacteria tested.
Full text
PDF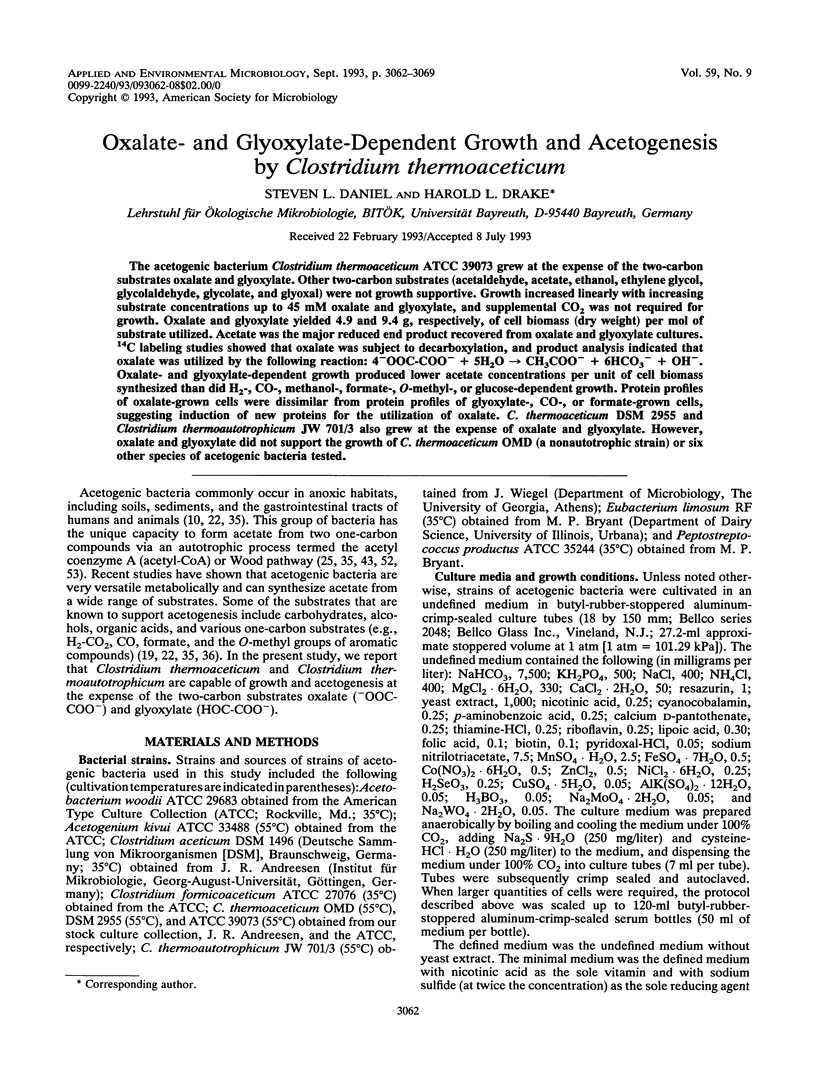
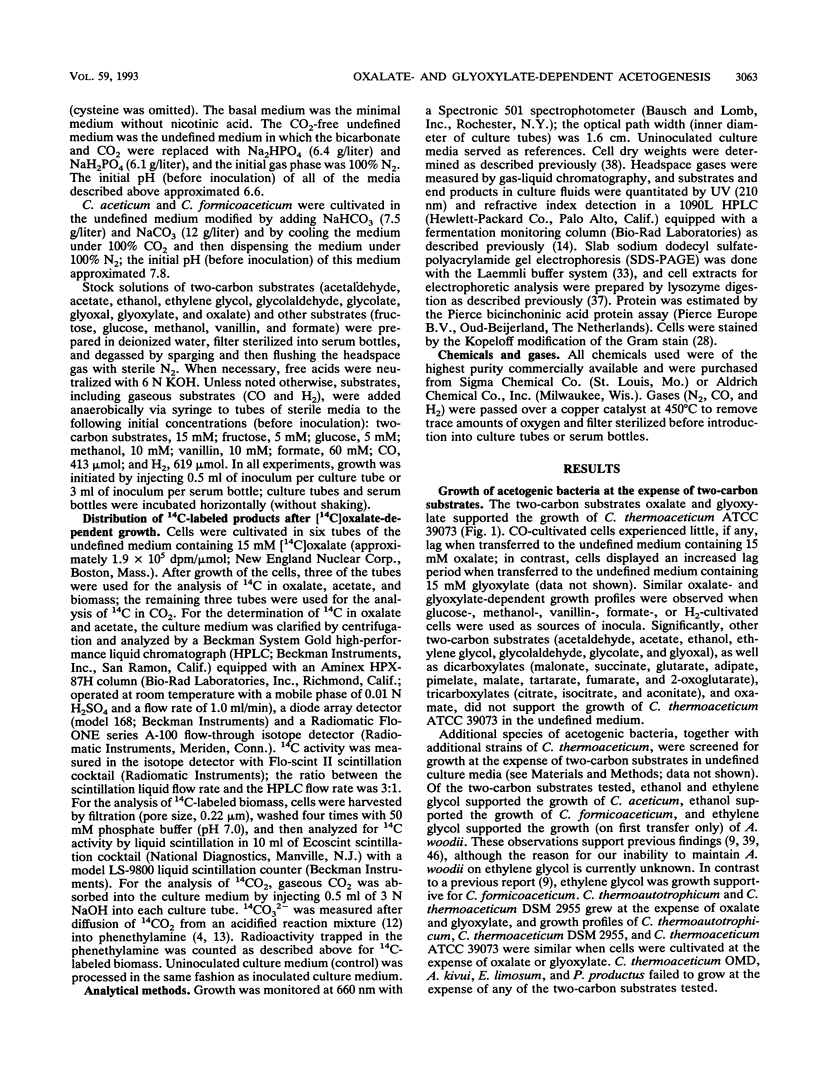
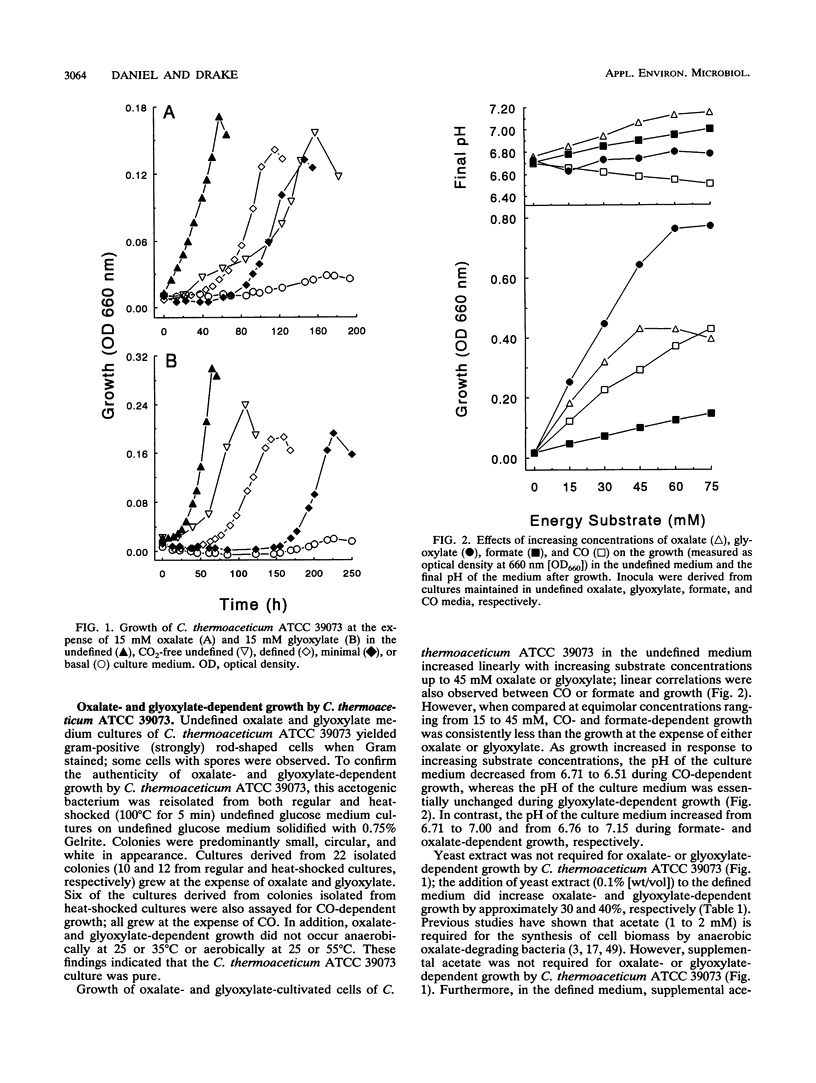
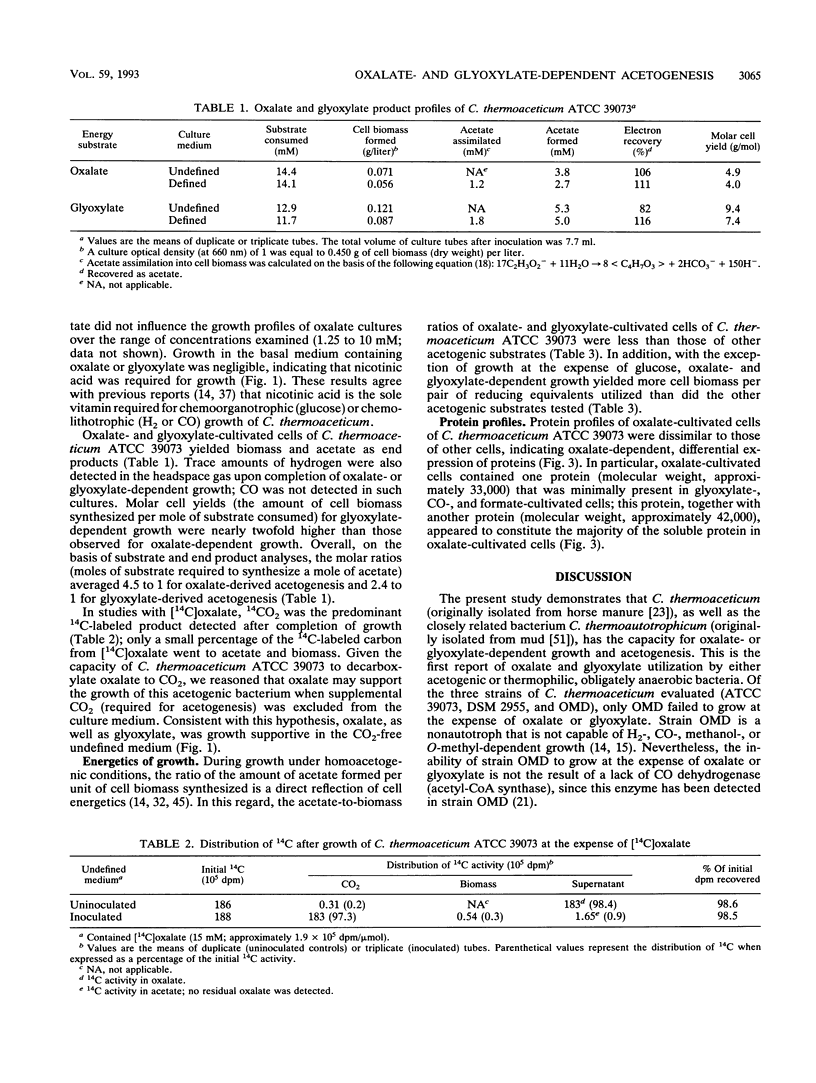
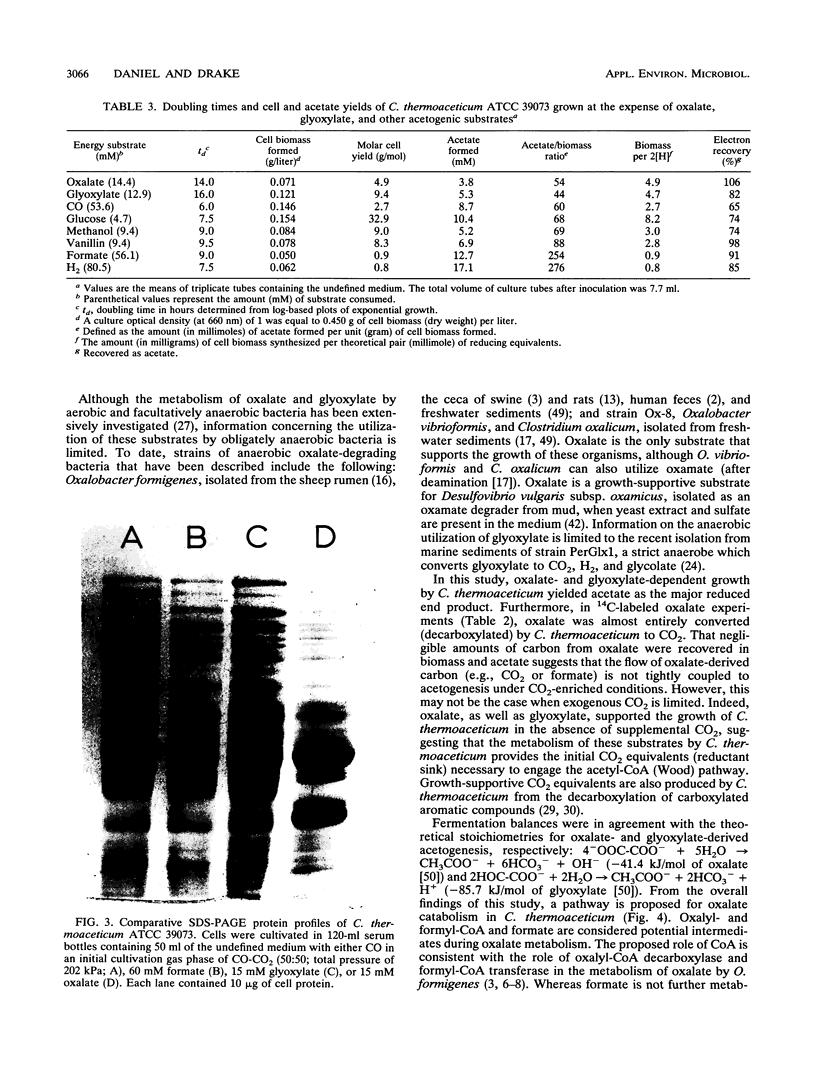
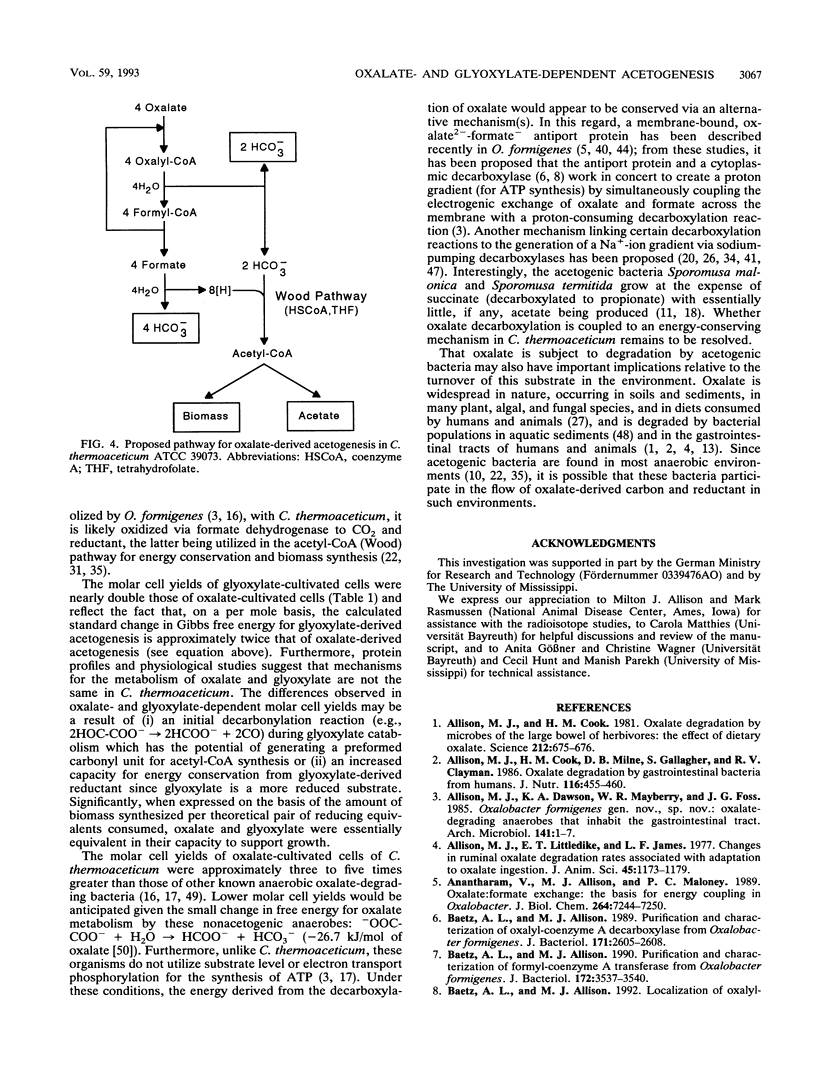
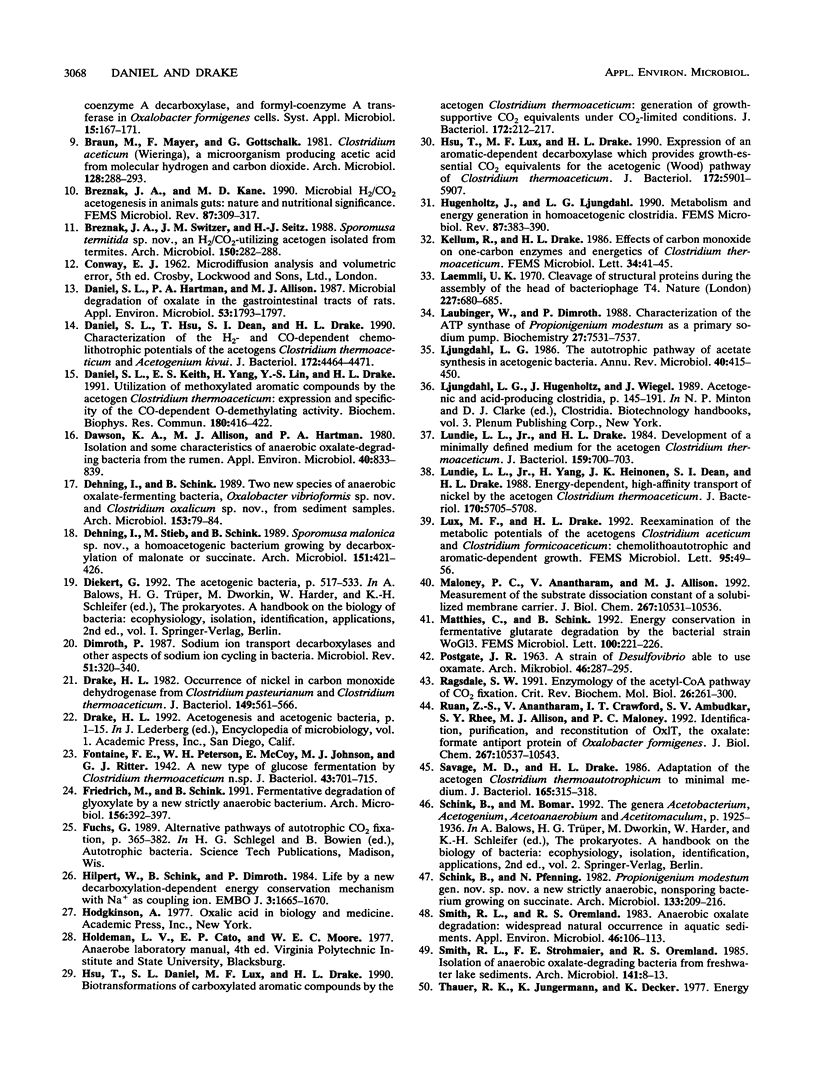
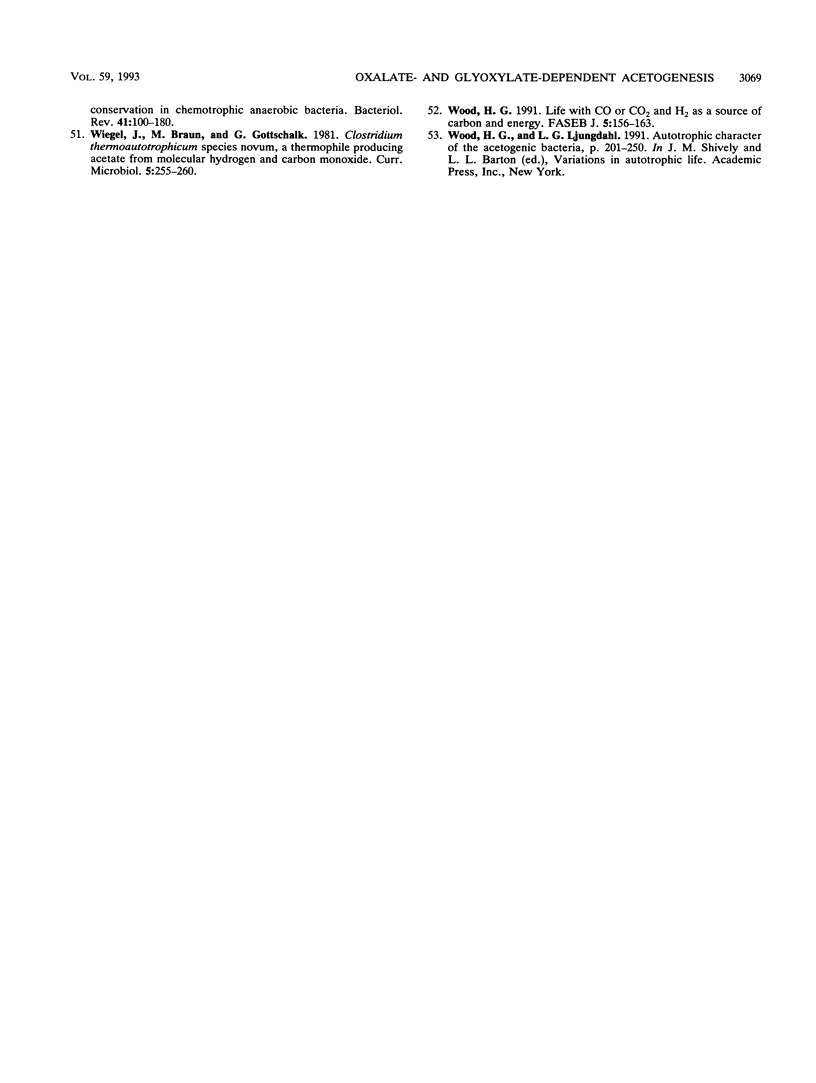
Images in this article
Selected References
These references are in PubMed. This may not be the complete list of references from this article.
- Allison M. J., Cook H. M., Milne D. B., Gallagher S., Clayman R. V. Oxalate degradation by gastrointestinal bacteria from humans. J Nutr. 1986 Mar;116(3):455–460. doi: 10.1093/jn/116.3.455. [DOI] [PubMed] [Google Scholar]
- Allison M. J., Cook H. M. Oxalate degradation by microbes of the large bowel of herbivores: the effect of dietary oxalate. Science. 1981 May 8;212(4495):675–676. doi: 10.1126/science.7221555. [DOI] [PubMed] [Google Scholar]
- Allison M. J., Dawson K. A., Mayberry W. R., Foss J. G. Oxalobacter formigenes gen. nov., sp. nov.: oxalate-degrading anaerobes that inhabit the gastrointestinal tract. Arch Microbiol. 1985 Feb;141(1):1–7. doi: 10.1007/BF00446731. [DOI] [PubMed] [Google Scholar]
- Allison M. J., Littledike E. T., James L. F. Changes in ruminal oxalate degradation rates associated with adaptation to oxalate ingestion. J Anim Sci. 1977 Nov;45(5):1173–1179. doi: 10.2527/jas1977.4551173x. [DOI] [PubMed] [Google Scholar]
- Anantharam V., Allison M. J., Maloney P. C. Oxalate:formate exchange. The basis for energy coupling in Oxalobacter. J Biol Chem. 1989 May 5;264(13):7244–7250. [PubMed] [Google Scholar]
- Baetz A. L., Allison M. J. Purification and characterization of formyl-coenzyme A transferase from Oxalobacter formigenes. J Bacteriol. 1990 Jul;172(7):3537–3540. doi: 10.1128/jb.172.7.3537-3540.1990. [DOI] [PMC free article] [PubMed] [Google Scholar]
- Baetz A. L., Allison M. J. Purification and characterization of oxalyl-coenzyme A decarboxylase from Oxalobacter formigenes. J Bacteriol. 1989 May;171(5):2605–2608. doi: 10.1128/jb.171.5.2605-2608.1989. [DOI] [PMC free article] [PubMed] [Google Scholar]
- Braun M., Mayer F., Gottschalk G. Clostridium aceticum (Wieringa), a microorganism producing acetic acid from molecular hydrogen and carbon dioxide. Arch Microbiol. 1981 Jan;128(3):288–293. doi: 10.1007/BF00422532. [DOI] [PubMed] [Google Scholar]
- Breznak J. A., Kane M. D. Microbial H2/CO2 acetogenesis in animal guts: nature and nutritional significance. FEMS Microbiol Rev. 1990 Dec;7(3-4):309–313. doi: 10.1111/j.1574-6968.1990.tb04929.x. [DOI] [PubMed] [Google Scholar]
- Daniel S. L., Hartman P. A., Allison M. J. Microbial degradation of oxalate in the gastrointestinal tracts of rats. Appl Environ Microbiol. 1987 Aug;53(8):1793–1797. doi: 10.1128/aem.53.8.1793-1797.1987. [DOI] [PMC free article] [PubMed] [Google Scholar]
- Daniel S. L., Hsu T., Dean S. I., Drake H. L. Characterization of the H2- and CO-dependent chemolithotrophic potentials of the acetogens Clostridium thermoaceticum and Acetogenium kivui. J Bacteriol. 1990 Aug;172(8):4464–4471. doi: 10.1128/jb.172.8.4464-4471.1990. [DOI] [PMC free article] [PubMed] [Google Scholar]
- Daniel S. L., Keith E. S., Yang H., Lin Y. S., Drake H. L. Utilization of methoxylated aromatic compounds by the acetogen Clostridium thermoaceticum: expression and specificity of the co-dependent O-demethylating activity. Biochem Biophys Res Commun. 1991 Oct 15;180(1):416–422. doi: 10.1016/s0006-291x(05)81309-9. [DOI] [PubMed] [Google Scholar]
- Dawson K. A., Allison M. J., Hartman P. A. Isolation and some characteristics of anaerobic oxalate-degrading bacteria from the rumen. Appl Environ Microbiol. 1980 Oct;40(4):833–839. doi: 10.1128/aem.40.4.833-839.1980. [DOI] [PMC free article] [PubMed] [Google Scholar]
- Dimroth P. Sodium ion transport decarboxylases and other aspects of sodium ion cycling in bacteria. Microbiol Rev. 1987 Sep;51(3):320–340. doi: 10.1128/mr.51.3.320-340.1987. [DOI] [PMC free article] [PubMed] [Google Scholar]
- Drake H. L. Occurrence of nickel in carbon monoxide dehydrogenase from Clostridium pasteurianum and Clostridium thermoaceticum. J Bacteriol. 1982 Feb;149(2):561–566. doi: 10.1128/jb.149.2.561-566.1982. [DOI] [PMC free article] [PubMed] [Google Scholar]
- Fontaine F. E., Peterson W. H., McCoy E., Johnson M. J., Ritter G. J. A New Type of Glucose Fermentation by Clostridium thermoaceticum. J Bacteriol. 1942 Jun;43(6):701–715. doi: 10.1128/jb.43.6.701-715.1942. [DOI] [PMC free article] [PubMed] [Google Scholar]
- Hilpert W., Schink B., Dimroth P. Life by a new decarboxylation-dependent energy conservation mechanism with Na as coupling ion. EMBO J. 1984 Aug;3(8):1665–1670. doi: 10.1002/j.1460-2075.1984.tb02030.x. [DOI] [PMC free article] [PubMed] [Google Scholar]
- Hsu T. D., Lux M. F., Drake H. L. Expression of an aromatic-dependent decarboxylase which provides growth-essential CO2 equivalents for the acetogenic (Wood) pathway of Clostridium thermoaceticum. J Bacteriol. 1990 Oct;172(10):5901–5907. doi: 10.1128/jb.172.10.5901-5907.1990. [DOI] [PMC free article] [PubMed] [Google Scholar]
- Hsu T., Daniel S. L., Lux M. F., Drake H. L. Biotransformations of carboxylated aromatic compounds by the acetogen Clostridium thermoaceticum: generation of growth-supportive CO2 equivalents under CO2-limited conditions. J Bacteriol. 1990 Jan;172(1):212–217. doi: 10.1128/jb.172.1.212-217.1990. [DOI] [PMC free article] [PubMed] [Google Scholar]
- Hugenholtz J., Ljungdahl L. G. Metabolism and energy generation in homoacetogenic clostridia. FEMS Microbiol Rev. 1990 Dec;7(3-4):383–389. doi: 10.1111/j.1574-6968.1990.tb04941.x. [DOI] [PubMed] [Google Scholar]
- Laemmli U. K. Cleavage of structural proteins during the assembly of the head of bacteriophage T4. Nature. 1970 Aug 15;227(5259):680–685. doi: 10.1038/227680a0. [DOI] [PubMed] [Google Scholar]
- Laubinger W., Dimroth P. Characterization of the ATP synthase of Propionigenium modestum as a primary sodium pump. Biochemistry. 1988 Sep 20;27(19):7531–7537. doi: 10.1021/bi00419a053. [DOI] [PubMed] [Google Scholar]
- Ljungdahl L. G. The autotrophic pathway of acetate synthesis in acetogenic bacteria. Annu Rev Microbiol. 1986;40:415–450. doi: 10.1146/annurev.mi.40.100186.002215. [DOI] [PubMed] [Google Scholar]
- Lundie L. L., Jr, Drake H. L. Development of a minimally defined medium for the acetogen Clostridium thermoaceticum. J Bacteriol. 1984 Aug;159(2):700–703. doi: 10.1128/jb.159.2.700-703.1984. [DOI] [PMC free article] [PubMed] [Google Scholar]
- Lundie L. L., Jr, Yang H. C., Heinonen J. K., Dean S. I., Drake H. L. Energy-dependent, high-affinity transport of nickel by the acetogen Clostridium thermoaceticum. J Bacteriol. 1988 Dec;170(12):5705–5708. doi: 10.1128/jb.170.12.5705-5708.1988. [DOI] [PMC free article] [PubMed] [Google Scholar]
- Lux M. F., Drake H. L. Re-examination of the metabolic potentials of the acetogens Clostridium aceticum and Clostridium formicoaceticum: chemolithoautotrophic and aromatic-dependent growth. FEMS Microbiol Lett. 1992 Aug 1;74(1):49–56. doi: 10.1016/0378-1097(92)90735-7. [DOI] [PubMed] [Google Scholar]
- Maloney P. C., Anantharam V., Allison M. J. Measurement of the substrate dissociation constant of a solubilized membrane carrier. Substrate stabilization of OxlT, the anion exchange protein of Oxalobacter formigenes. J Biol Chem. 1992 May 25;267(15):10531–10536. [PubMed] [Google Scholar]
- Matthies C., Schink B. Energy conservation in fermentative glutarate degradation by the bacterial strain WoG13. FEMS Microbiol Lett. 1992 Dec 15;100(1-3):221–225. doi: 10.1111/j.1574-6968.1992.tb14044.x. [DOI] [PubMed] [Google Scholar]
- POSTGATE J. R. A STRAIN OF DESULFOVIBRIO ABLE TO USE OXAMATE. Arch Mikrobiol. 1963 Sep 16;46:287–295. doi: 10.1007/BF00422189. [DOI] [PubMed] [Google Scholar]
- Ragsdale S. W. Enzymology of the acetyl-CoA pathway of CO2 fixation. Crit Rev Biochem Mol Biol. 1991;26(3-4):261–300. doi: 10.3109/10409239109114070. [DOI] [PubMed] [Google Scholar]
- Ruan Z. S., Anantharam V., Crawford I. T., Ambudkar S. V., Rhee S. Y., Allison M. J., Maloney P. C. Identification, purification, and reconstitution of OxlT, the oxalate: formate antiport protein of Oxalobacter formigenes. J Biol Chem. 1992 May 25;267(15):10537–10543. [PubMed] [Google Scholar]
- Savage M. D., Drake H. L. Adaptation of the acetogen Clostridium thermoautotrophicum to minimal medium. J Bacteriol. 1986 Jan;165(1):315–318. doi: 10.1128/jb.165.1.315-318.1986. [DOI] [PMC free article] [PubMed] [Google Scholar]
- Smith R. L., Oremland R. S. Anaerobic oxalate degradation: widespread natural occurrence in aquatic sediments. Appl Environ Microbiol. 1983 Jul;46(1):106–113. doi: 10.1128/aem.46.1.106-113.1983. [DOI] [PMC free article] [PubMed] [Google Scholar]
- Wood H. G. Life with CO or CO2 and H2 as a source of carbon and energy. FASEB J. 1991 Feb;5(2):156–163. doi: 10.1096/fasebj.5.2.1900793. [DOI] [PubMed] [Google Scholar]