Abstract
Bacteroides ovatus NCTC 11153 was grown in a two-stage continuous culture system at various growth rates (vessel 1, D = 0.06 to 0.19 h-1; vessel 2, D = 0.03 to 0.09 h-1) on media containing mixtures of starch and arabinogalactan as carbon sources. The cell-associated enzyme activities needed to hydrolyze both substrates (amylase, arabinogalactanase, alpha-glucosidase, beta-galactosidase, and alpha-arabinofuranosidase) were variously influenced by growth rate and polysaccharide availability but were detected under all growth conditions tested. Measurements of residual carbohydrate in spent culture media showed that both polysaccharides were co-utilized during growth under putative C-limited conditions. The arabinogalactan was partly depolymerized in N-limited chemostats, and significant amounts of arabinose- and galactose-containing oligosaccharides accumulated in the cultures, indicating that starch was being preferentially utilized. Acetate, propionate, and succinate were the major fermentation products formed by C-limited bacteria, but under N limitation, lactate was also produced. Molar ratios of succinate increased concomitantly with the dilution rate in C-limited chemostats, whereas molar ratios of propionate decreased. During N-limited growth, however, decarboxylation of succinate to propionate was relatively independent of growth rate. Cell viability was higher in C-limited cultures compared with those grown under N limitation and was greatest at high dilution rates, irrespective of nutrient limitation.
Full text
PDF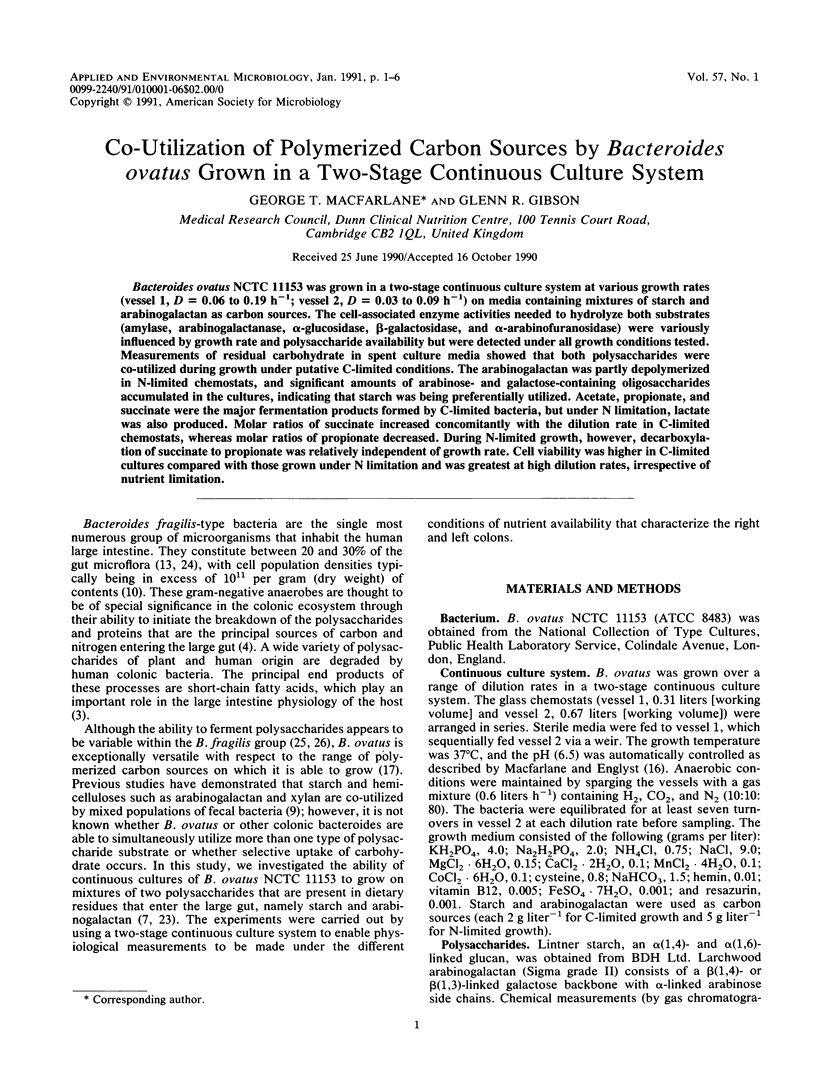
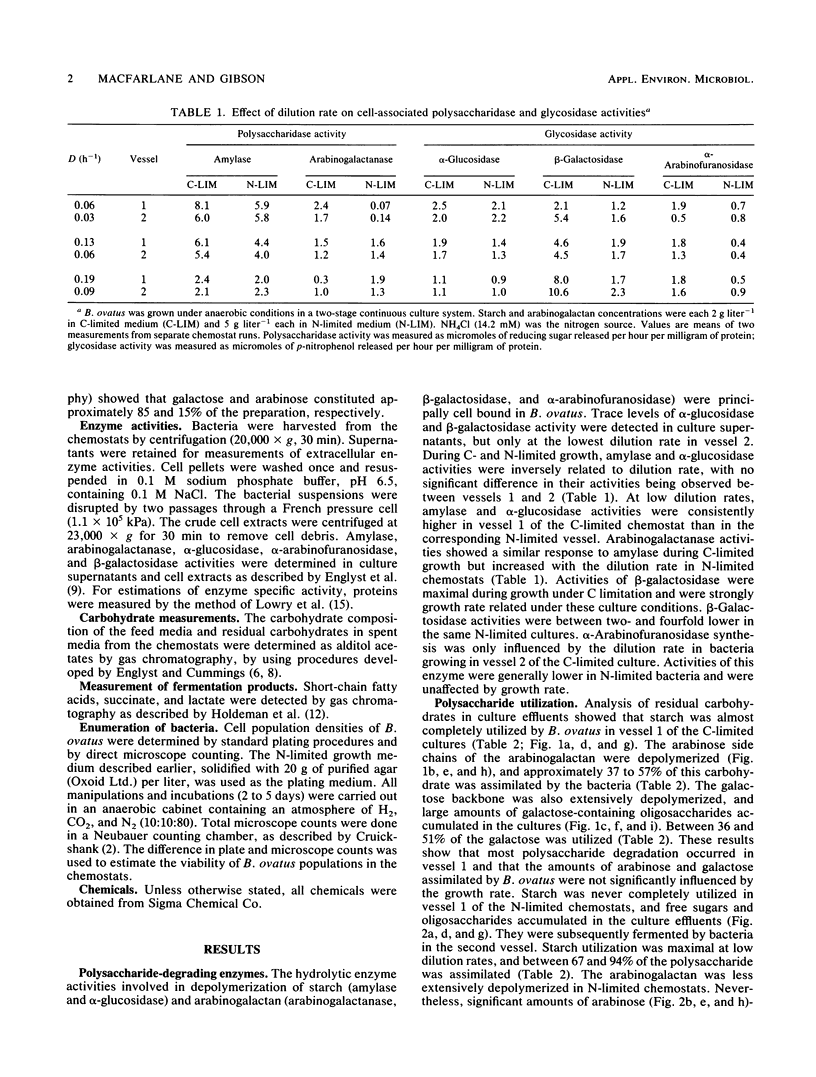
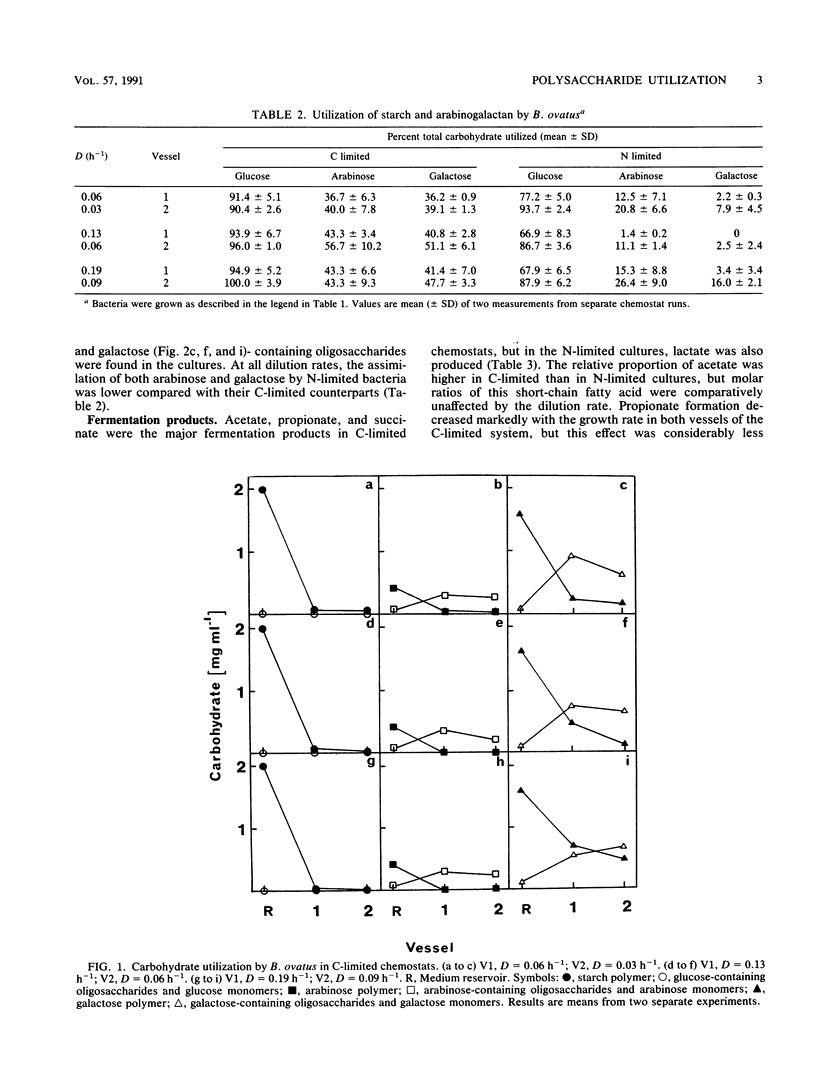
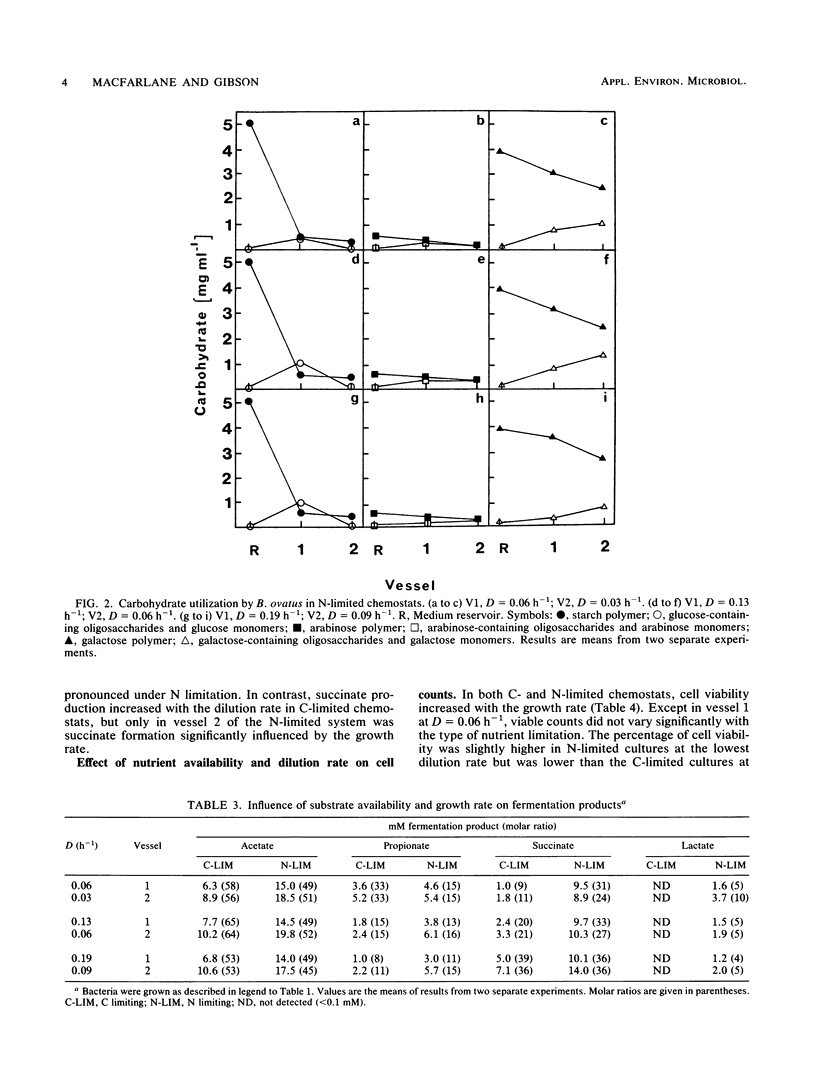
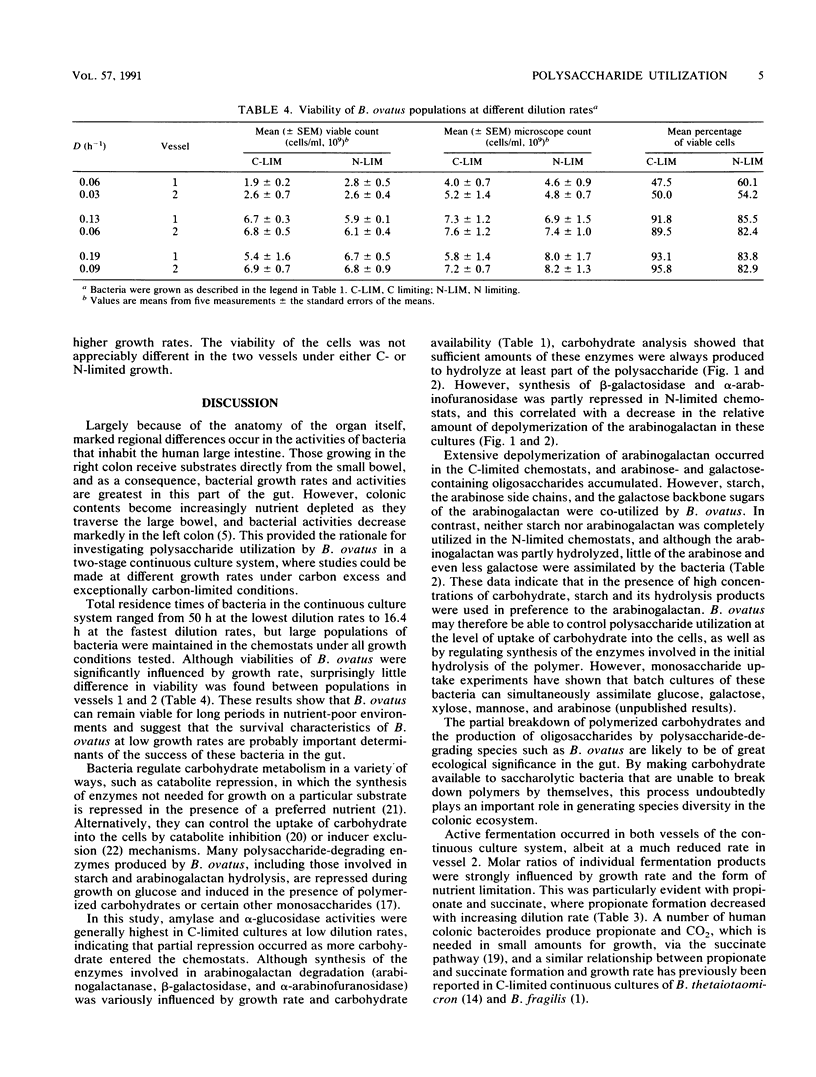
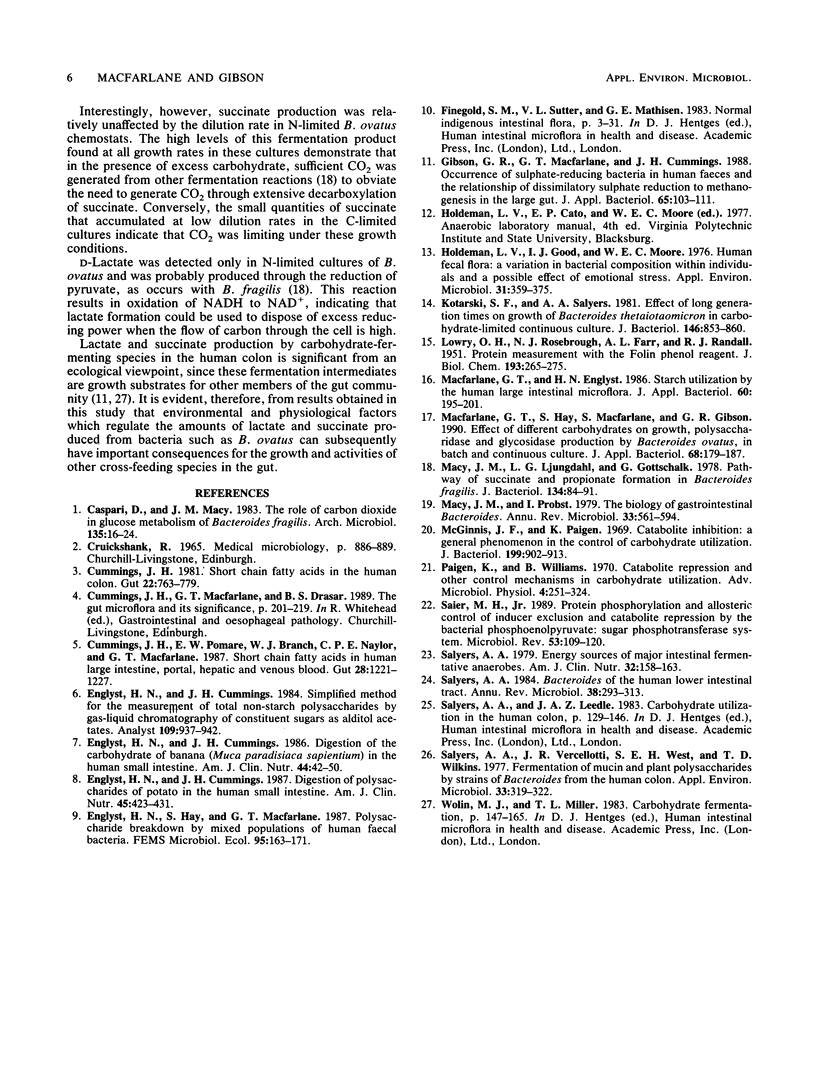
Selected References
These references are in PubMed. This may not be the complete list of references from this article.
- Caspari D., Macy J. M. The role of carbon dioxide in glucose metabolism of Bacteroides fragilis. Arch Microbiol. 1983 Aug;135(1):16–24. doi: 10.1007/BF00419476. [DOI] [PubMed] [Google Scholar]
- Cummings J. H., Pomare E. W., Branch W. J., Naylor C. P., Macfarlane G. T. Short chain fatty acids in human large intestine, portal, hepatic and venous blood. Gut. 1987 Oct;28(10):1221–1227. doi: 10.1136/gut.28.10.1221. [DOI] [PMC free article] [PubMed] [Google Scholar]
- Cummings J. H. Short chain fatty acids in the human colon. Gut. 1981 Sep;22(9):763–779. doi: 10.1136/gut.22.9.763. [DOI] [PMC free article] [PubMed] [Google Scholar]
- Englyst H. N., Cummings J. H. Digestion of polysaccharides of potato in the small intestine of man. Am J Clin Nutr. 1987 Feb;45(2):423–431. doi: 10.1093/ajcn/45.2.423. [DOI] [PubMed] [Google Scholar]
- Englyst H. N., Cummings J. H. Digestion of the carbohydrates of banana (Musa paradisiaca sapientum) in the human small intestine. Am J Clin Nutr. 1986 Jul;44(1):42–50. doi: 10.1093/ajcn/44.1.42. [DOI] [PubMed] [Google Scholar]
- Gibson G. R., Macfarlane G. T., Cummings J. H. Occurrence of sulphate-reducing bacteria in human faeces and the relationship of dissimilatory sulphate reduction to methanogenesis in the large gut. J Appl Bacteriol. 1988 Aug;65(2):103–111. doi: 10.1111/j.1365-2672.1988.tb01498.x. [DOI] [PubMed] [Google Scholar]
- Holdeman L. V., Good I. J., Moore W. E. Human fecal flora: variation in bacterial composition within individuals and a possible effect of emotional stress. Appl Environ Microbiol. 1976 Mar;31(3):359–375. doi: 10.1128/aem.31.3.359-375.1976. [DOI] [PMC free article] [PubMed] [Google Scholar]
- Kotarski S. F., Salyers A. A. Effect of long generation times on growth of Bacteroides thetaiotaomicron in carbohydrate-induced continuous culture. J Bacteriol. 1981 Jun;146(3):853–860. doi: 10.1128/jb.146.3.853-860.1981. [DOI] [PMC free article] [PubMed] [Google Scholar]
- LOWRY O. H., ROSEBROUGH N. J., FARR A. L., RANDALL R. J. Protein measurement with the Folin phenol reagent. J Biol Chem. 1951 Nov;193(1):265–275. [PubMed] [Google Scholar]
- Macfarlane G. T., Englyst H. N. Starch utilization by the human large intestinal microflora. J Appl Bacteriol. 1986 Mar;60(3):195–201. doi: 10.1111/j.1365-2672.1986.tb01073.x. [DOI] [PubMed] [Google Scholar]
- Macfarlane G. T., Hay S., Macfarlane S., Gibson G. R. Effect of different carbohydrates on growth, polysaccharidase and glycosidase production by Bacteroides ovatus, in batch and continuous culture. J Appl Bacteriol. 1990 Feb;68(2):179–187. doi: 10.1111/j.1365-2672.1990.tb02564.x. [DOI] [PubMed] [Google Scholar]
- Macy J. M., Ljungdahl L. G., Gottschalk G. Pathway of succinate and propionate formation in Bacteroides fragilis. J Bacteriol. 1978 Apr;134(1):84–91. doi: 10.1128/jb.134.1.84-91.1978. [DOI] [PMC free article] [PubMed] [Google Scholar]
- Macy J. M., Probst I. The biology of gastrointestinal bacteroides. Annu Rev Microbiol. 1979;33:561–594. doi: 10.1146/annurev.mi.33.100179.003021. [DOI] [PubMed] [Google Scholar]
- McGinnis J. F., Paigen K. Catabolite inhibition: a general phenomenon in the control of carbohydrate utilization. J Bacteriol. 1969 Nov;100(2):902–913. doi: 10.1128/jb.100.2.902-913.1969. [DOI] [PMC free article] [PubMed] [Google Scholar]
- Saier M. H., Jr Protein phosphorylation and allosteric control of inducer exclusion and catabolite repression by the bacterial phosphoenolpyruvate: sugar phosphotransferase system. Microbiol Rev. 1989 Mar;53(1):109–120. doi: 10.1128/mr.53.1.109-120.1989. [DOI] [PMC free article] [PubMed] [Google Scholar]
- Salyers A. A. Bacteroides of the human lower intestinal tract. Annu Rev Microbiol. 1984;38:293–313. doi: 10.1146/annurev.mi.38.100184.001453. [DOI] [PubMed] [Google Scholar]
- Salyers A. A. Energy sources of major intestinal fermentative anaerobes. Am J Clin Nutr. 1979 Jan;32(1):158–163. doi: 10.1093/ajcn/32.1.158. [DOI] [PubMed] [Google Scholar]
- Salyers A. A., Vercellotti J. R., West S. E., Wilkins T. D. Fermentation of mucin and plant polysaccharides by strains of Bacteroides from the human colon. Appl Environ Microbiol. 1977 Feb;33(2):319–322. doi: 10.1128/aem.33.2.319-322.1977. [DOI] [PMC free article] [PubMed] [Google Scholar]