Abstract
Washed cells of strain H18, a newly isolated ruminal selenomonad, decarboxylated succinate 25-fold faster than Selenomonas ruminantium HD4 (130 versus 5 nmol min-1 mg of protein-1, respectively). Batch cultures of strain H18 which were fermenting glucose did not utilize succinate, and glucose-limited continuous cultures were only able to decarboxylate significant amounts of succinate at slow (less than 0.1 h-1) dilution rates. Strain H18 grew more slowly on lactate than glucose (0.2 versus 0.4 h-1, respectively), and more than half of the lactate was initially converted to succinate. Succinate was only utilized after growth on lactate had ceased. Although nonenergized and glucose-energized cells had similar proton motive forces and ATP levels, glucose-energized cells were unable to transport succinate. Transport by nonenergized cells was decreased by small increases in osmotic strength, and it is possible that energy-dependent inhibition of succinate transport was related to changes in cell turgor. Since cells which were deenergized with 2-deoxyglucose or iodoacetate did not transport succinate, it appeared that glycogen metabolism was providing the driving force for succinate uptake. An artificial delta pH drove succinate transport in deenergized cells, but an artificial membrane potential (delta psi) could not serve as a driving force. Because succinate is nearly fully dissociated at pH 7.0 and the transport process was electroneutral, it appeared that succinate was taken up in symport with two protons. An Eadie-Hofstee plot indicated that the rate of uptake was unusually rapid at high substrate concentrations, but the low-velocity, high-affinity component could account for succinate utilization by stationary cultures.(ABSTRACT TRUNCATED AT 250 WORDS)
Full text
PDF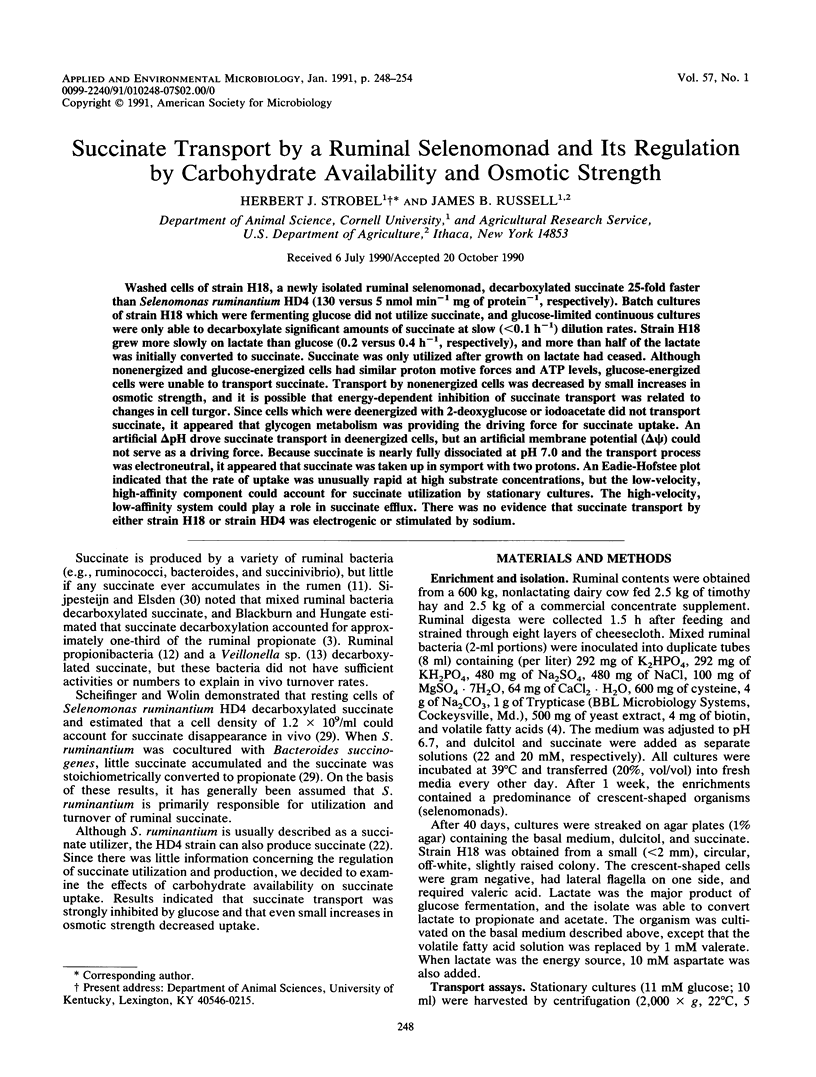
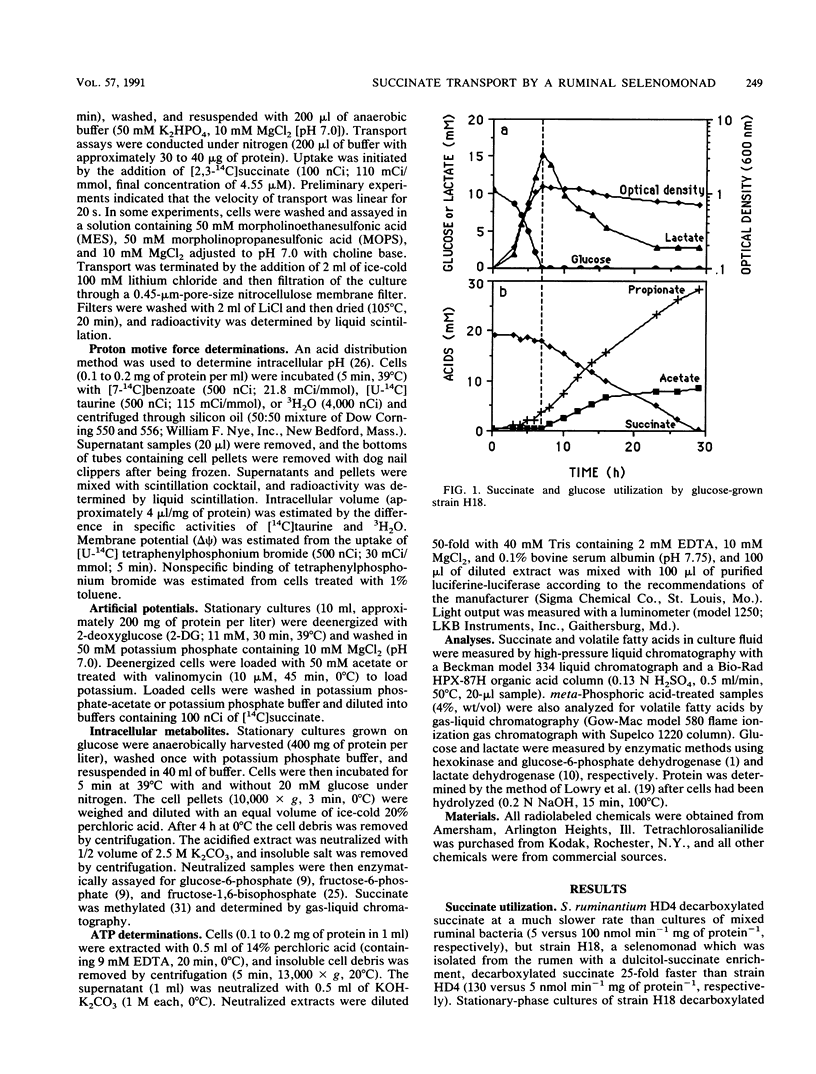
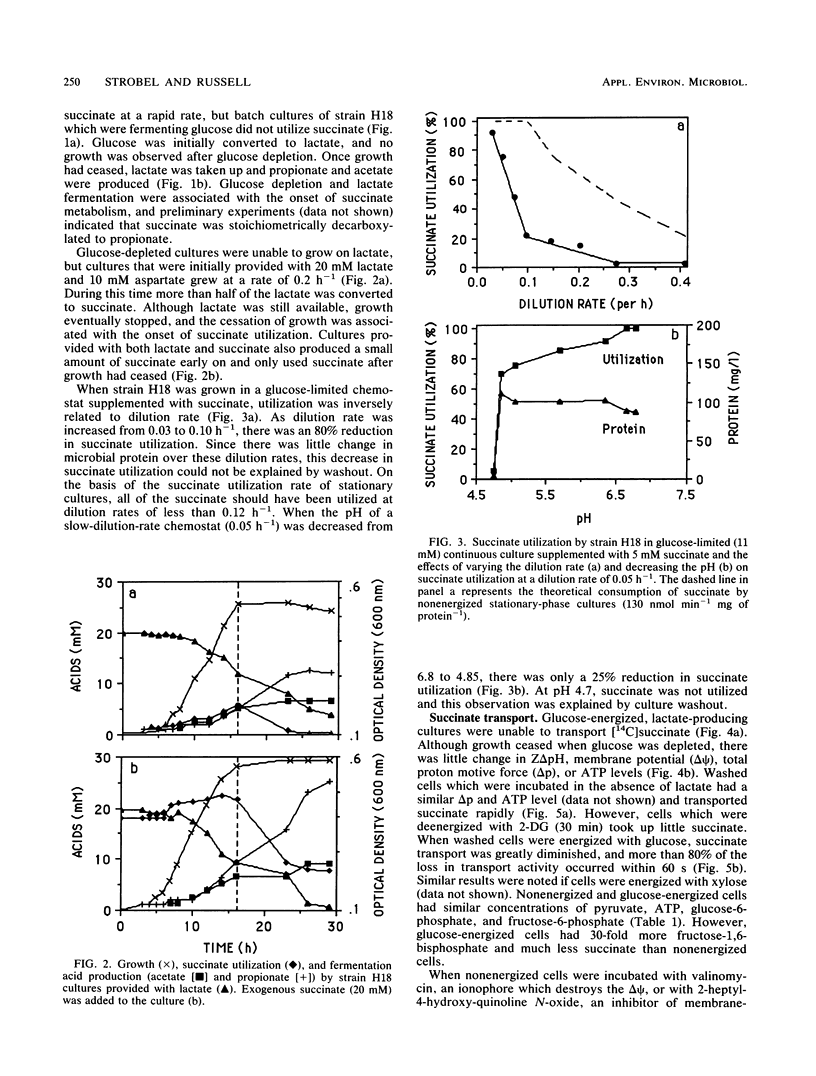
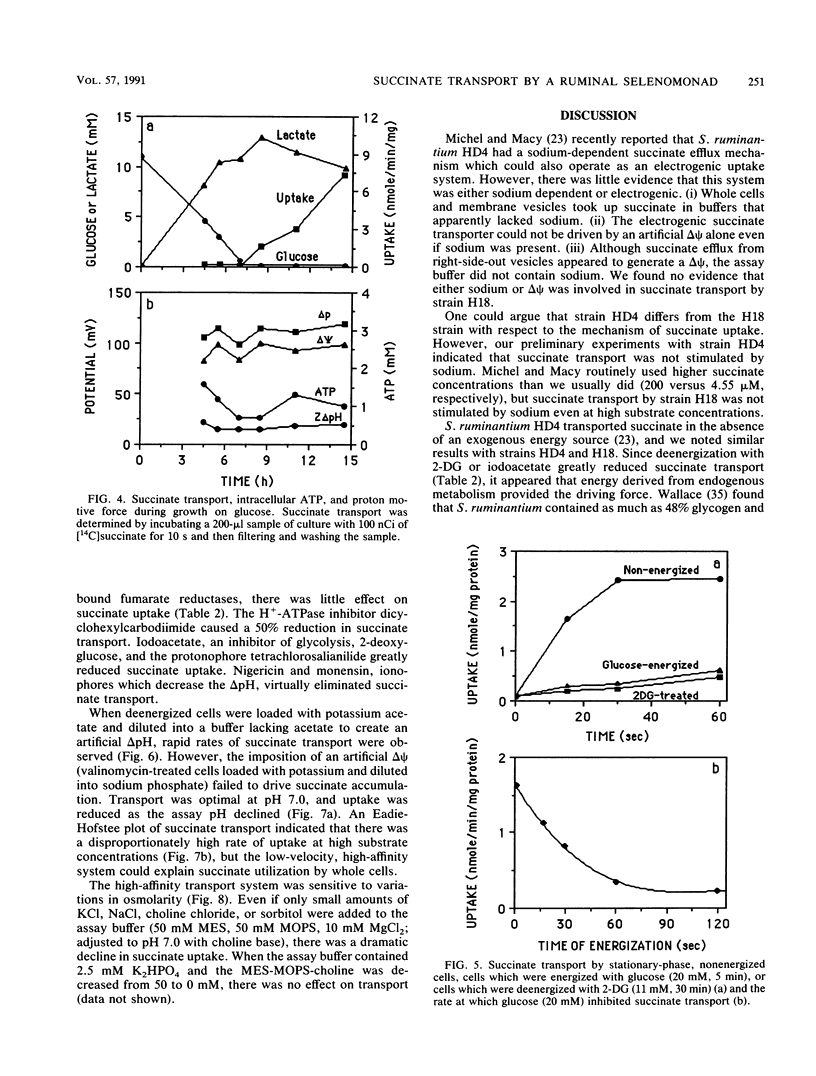
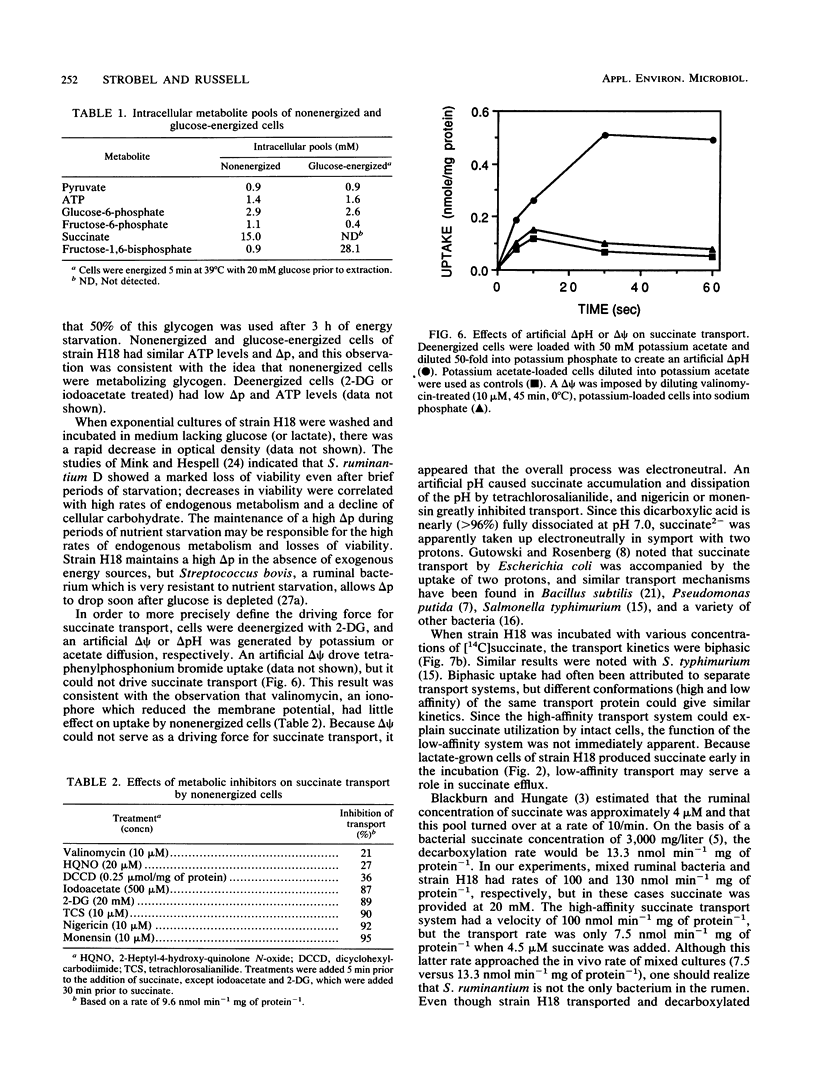
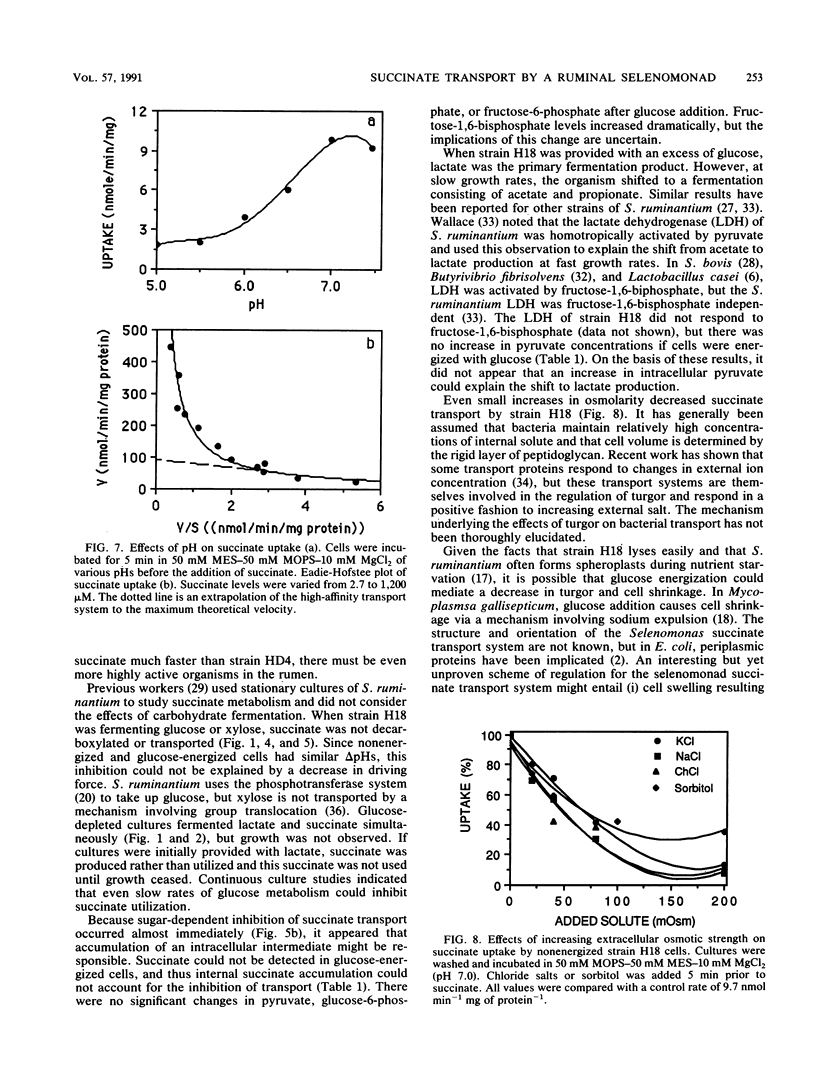
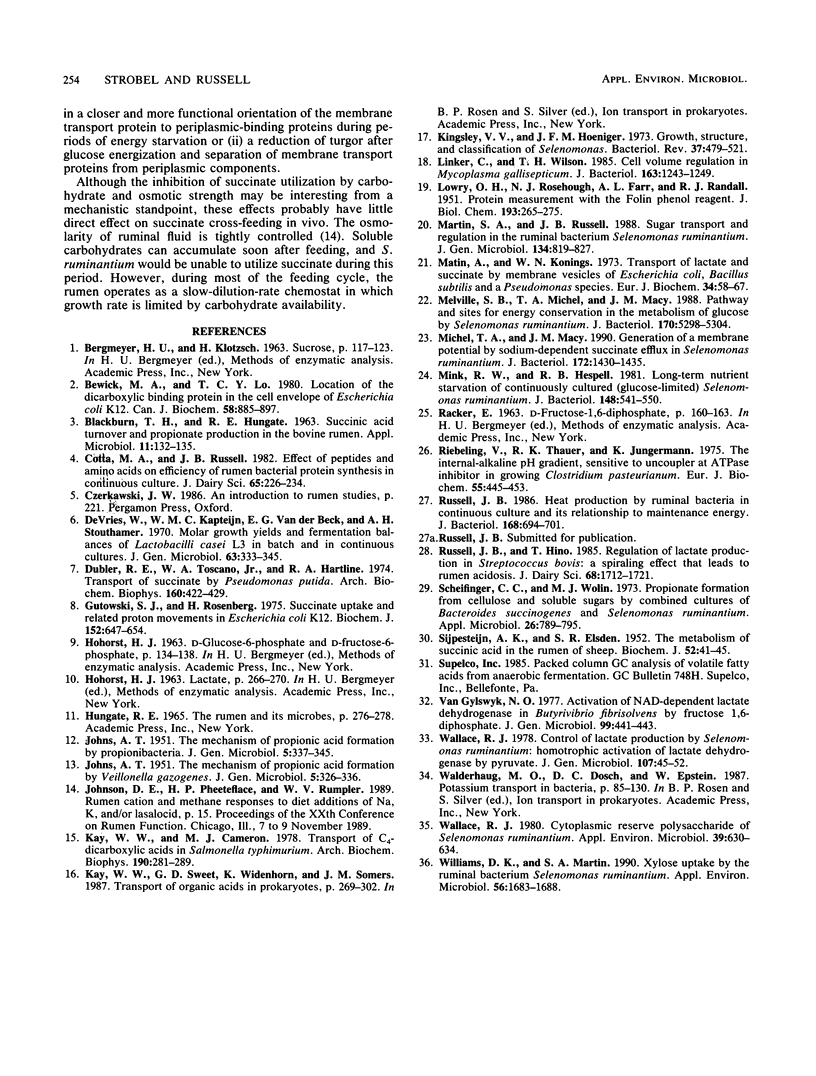
Selected References
These references are in PubMed. This may not be the complete list of references from this article.
- BLACKBURN T. H., HUNGATE R. E. Succinic acid turnover and propionate production in the bovine rumen. Appl Microbiol. 1963 Mar;11:132–135. doi: 10.1128/am.11.2.132-135.1963. [DOI] [PMC free article] [PubMed] [Google Scholar]
- Bewick M. A., Lo T. C. Localization of the dicarboxylate binding protein in the cell envelope of Escherichia coli K12. Can J Biochem. 1980 Oct;58(10):885–897. doi: 10.1139/o80-123. [DOI] [PubMed] [Google Scholar]
- Dubler R. E., Toscano W. A., Jr, Hartline R. A. Transport of succinate by Pseudomonas putida. Arch Biochem Biophys. 1974 Feb;160(2):422–429. doi: 10.1016/0003-9861(74)90416-0. [DOI] [PubMed] [Google Scholar]
- Gutowski S. J., Rosenberg H. Succinate uptake and related proton movements in Escherichia coli K12. Biochem J. 1975 Dec;152(3):647–654. doi: 10.1042/bj1520647. [DOI] [PMC free article] [PubMed] [Google Scholar]
- JOHNS A. T. The mechanism of propionic acid formation by Veillonella gazogenes. J Gen Microbiol. 1951 May;5(2):326–336. doi: 10.1099/00221287-5-2-326. [DOI] [PubMed] [Google Scholar]
- JOHNS A. T. The mechanism of propionic acid formation by propionibacteria. J Gen Microbiol. 1951 May;5(2):337–345. doi: 10.1099/00221287-5-2-337. [DOI] [PubMed] [Google Scholar]
- Kay W. W., Cameron M. J. Transport of C4-dicarboxylic acids in salmonella typhimurium. Arch Biochem Biophys. 1978 Sep;190(1):281–289. doi: 10.1016/0003-9861(78)90277-1. [DOI] [PubMed] [Google Scholar]
- Kingsley V. V., Hoeniger J. F. Growth, structure, and classification of Selenomonas. Bacteriol Rev. 1973 Dec;37(4):479–521. doi: 10.1128/br.37.4.479-521.1973. [DOI] [PMC free article] [PubMed] [Google Scholar]
- LOWRY O. H., ROSEBROUGH N. J., FARR A. L., RANDALL R. J. Protein measurement with the Folin phenol reagent. J Biol Chem. 1951 Nov;193(1):265–275. [PubMed] [Google Scholar]
- Linker C., Wilson T. H. Cell volume regulation in Mycoplasma gallisepticum. J Bacteriol. 1985 Sep;163(3):1243–1249. doi: 10.1128/jb.163.3.1243-1249.1985. [DOI] [PMC free article] [PubMed] [Google Scholar]
- Matin A., Konings W. N. Transport of lactate and succinate by membrane vesicles of Escherichia coli, Bacillus subtilis and a pseudomonas species. Eur J Biochem. 1973 Apr 2;34(1):58–67. doi: 10.1111/j.1432-1033.1973.tb02728.x. [DOI] [PubMed] [Google Scholar]
- Melville S. B., Michel T. A., Macy J. M. Pathway and sites for energy conservation in the metabolism of glucose by Selenomonas ruminantium. J Bacteriol. 1988 Nov;170(11):5298–5304. doi: 10.1128/jb.170.11.5298-5304.1988. [DOI] [PMC free article] [PubMed] [Google Scholar]
- Michel T. A., Macy J. M. Generation of a membrane potential by sodium-dependent succinate efflux in Selenomonas ruminantium. J Bacteriol. 1990 Mar;172(3):1430–1435. doi: 10.1128/jb.172.3.1430-1435.1990. [DOI] [PMC free article] [PubMed] [Google Scholar]
- Mink R. W., Hespell R. B. Long-term nutrient starvation of continuously cultured (glucose-limited) Selenomonas ruminantium. J Bacteriol. 1981 Nov;148(2):541–550. doi: 10.1128/jb.148.2.541-550.1981. [DOI] [PMC free article] [PubMed] [Google Scholar]
- Riebeling V., Thauer R. K., Jungermann K. The internal-alkaline pH gradient, sensitive to uncoupler and ATPase inhibitor, in growing Clostridium pasteurianum. Eur J Biochem. 1975 Jul 1;55(2):445–453. doi: 10.1111/j.1432-1033.1975.tb02181.x. [DOI] [PubMed] [Google Scholar]
- Russell J. B. Heat production by ruminal bacteria in continuous culture and its relationship to maintenance energy. J Bacteriol. 1986 Nov;168(2):694–701. doi: 10.1128/jb.168.2.694-701.1986. [DOI] [PMC free article] [PubMed] [Google Scholar]
- SIJPESTEIJN A. K., ELSDEN S. R. The metabolism of succinic acid in the rumen of the sheep. Biochem J. 1952 Sep;52(1):41–45. doi: 10.1042/bj0520041. [DOI] [PMC free article] [PubMed] [Google Scholar]
- Scheifinger C. C., Wolin M. J. Propionate formation from cellulose and soluble sugars by combined cultures of Bacteroides succinogenes and Selenomonas ruminantium. Appl Microbiol. 1973 Nov;26(5):789–795. doi: 10.1128/am.26.5.789-795.1973. [DOI] [PMC free article] [PubMed] [Google Scholar]
- Wallace R. J. Control of lactate production by Selenomonas ruminantium: homotropic activation of lactate dehydrogenase by pyruvate. J Gen Microbiol. 1978 Jul;107(1):45–52. doi: 10.1099/00221287-107-1-45. [DOI] [PubMed] [Google Scholar]
- Wallace R. J. Cytoplasmic reserve polysaccharide of Selenomonas ruminantium. Appl Environ Microbiol. 1980 Mar;39(3):630–634. doi: 10.1128/aem.39.3.630-634.1980. [DOI] [PMC free article] [PubMed] [Google Scholar]
- Williams D. K., Martin S. A. Xylose uptake by the ruminal bacterium Selenomonas ruminantium. Appl Environ Microbiol. 1990 Jun;56(6):1683–1688. doi: 10.1128/aem.56.6.1683-1688.1990. [DOI] [PMC free article] [PubMed] [Google Scholar]
- de Vries W., Kapteijn W. M., van der Beek E. G., Stouthamer A. H. Molar growth yields and fermentation balances of Lactobacillus casei L3 in batch cultures and in continuous cultures. J Gen Microbiol. 1970 Nov;63(3):333–345. doi: 10.1099/00221287-63-3-333. [DOI] [PubMed] [Google Scholar]
- van Gylswyk N. O. Activation of NAD-dependent lactate dehydrogenase in Butyrivibrio fibrisolvens by fructose 1,6-diphosphate. J Gen Microbiol. 1977 Apr;99(2):441–443. doi: 10.1099/00221287-99-2-441. [DOI] [PubMed] [Google Scholar]