Abstract
The ruminal selenomonad strain H18 grew rapidly (mu = 0.50 h-1) in a defined medium containing glucose, ammonia, purified amino acids, and sodium (95 mM); little if any ammonia was utilized as a nitrogen source. When the sodium salts were replaced by potassium salts (0.13 mM sodium), there was a small reduction in growth rate (mu = 0.34 h-1), and under these conditions greater than 95% of the cell nitrogen was derived from ammonia. No growth was observed when the medium lacked sodium (less than 0.35 mM) and amino acids were the only nitrogen source. At least six amino acid transport systems (aspartate, glutamine, lysine, phenylalanine, serine, and valine) were sodium dependent, and these systems could be driven by an electrical potential (delta psi) or a chemical gradient of sodium. H18 utilized lactate as an energy source for growth, but only when sodium and aspartate were added to the medium. Malate or fumarate was able to replace aspartate, and when these acids were added, sodium was no longer required. Glucose-grown cells accumulated large amounts of polysaccharide (64% of dry weight), and when the exogenous glucose was depleted, this material was converted to acetate and propionate as long as sodium was present. When the cells were incubated in buffers lacking sodium, succinate accumulated and exogenous succinate could not be decarboxylated. Because sodium had little effect on the transmembrane pH gradient at pH 6.7 to 4.5, it did not appear that sodium was required for intracellular pH regulation.
Full text
PDF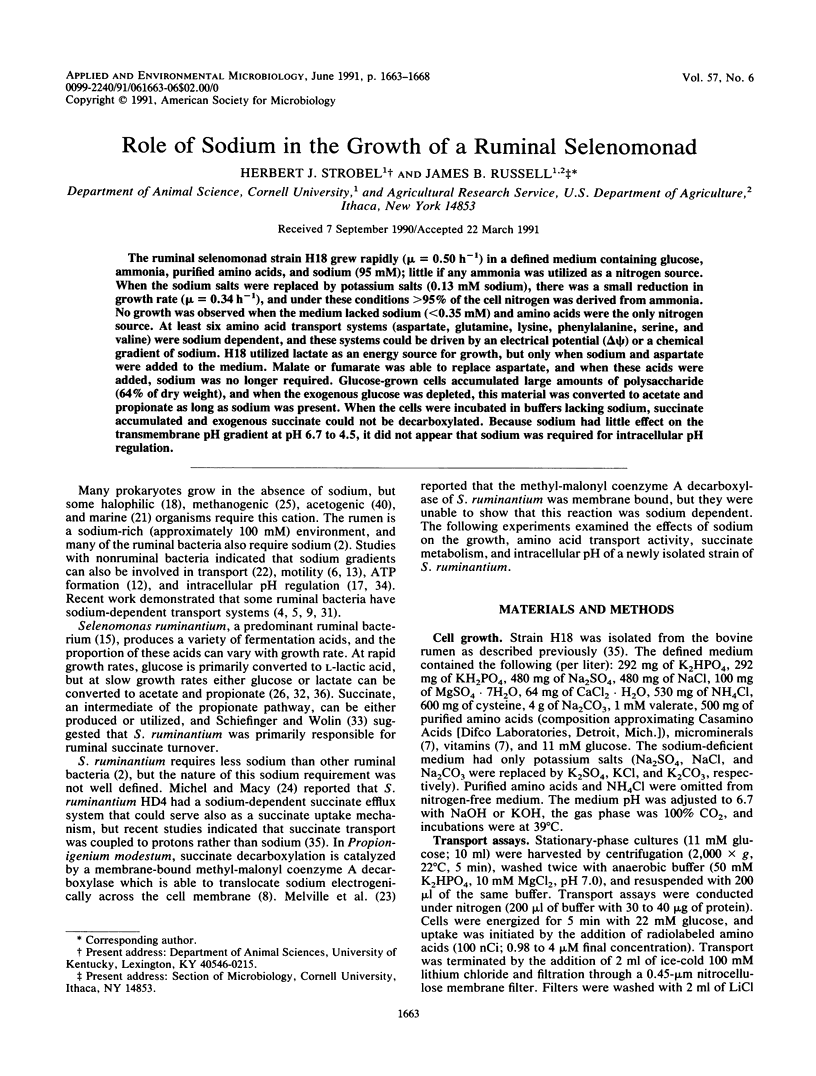
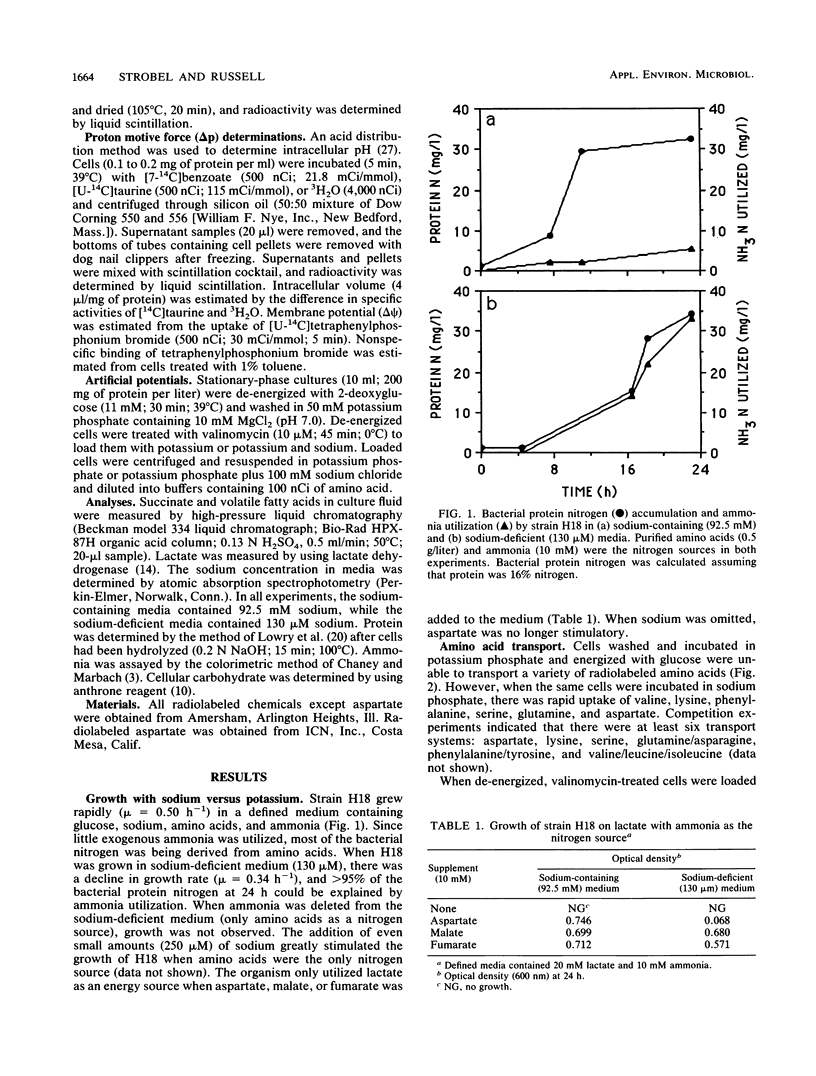
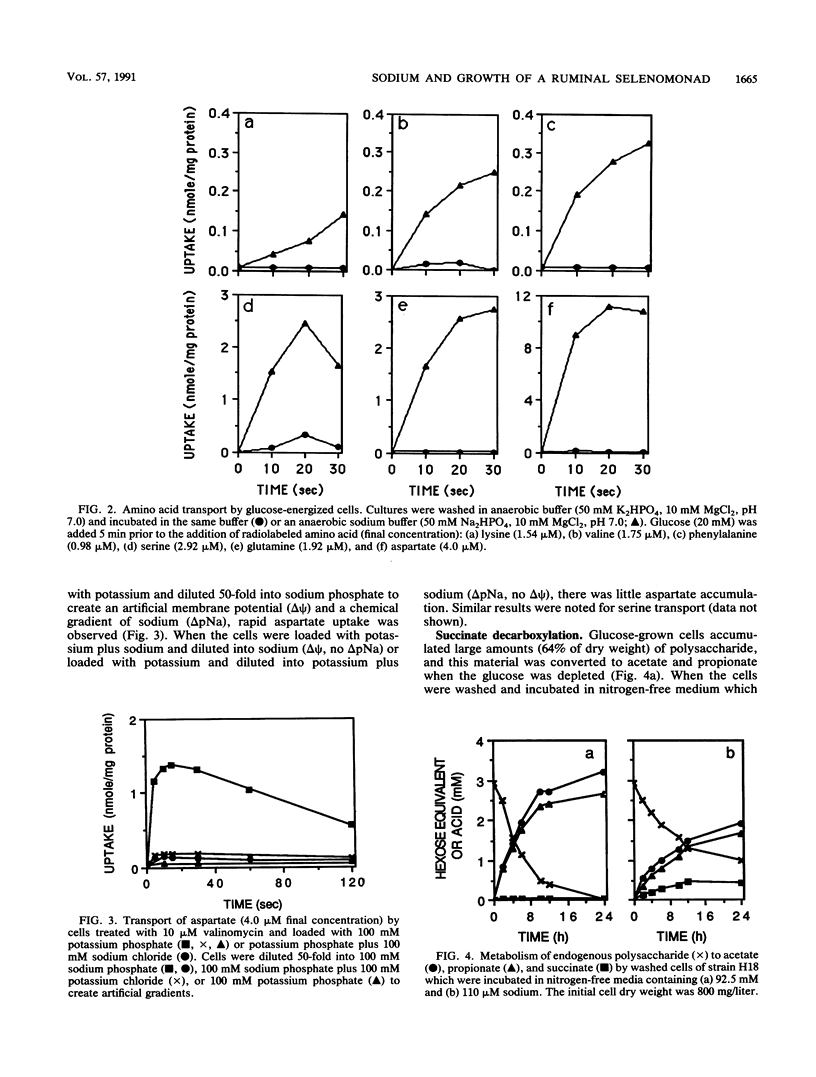
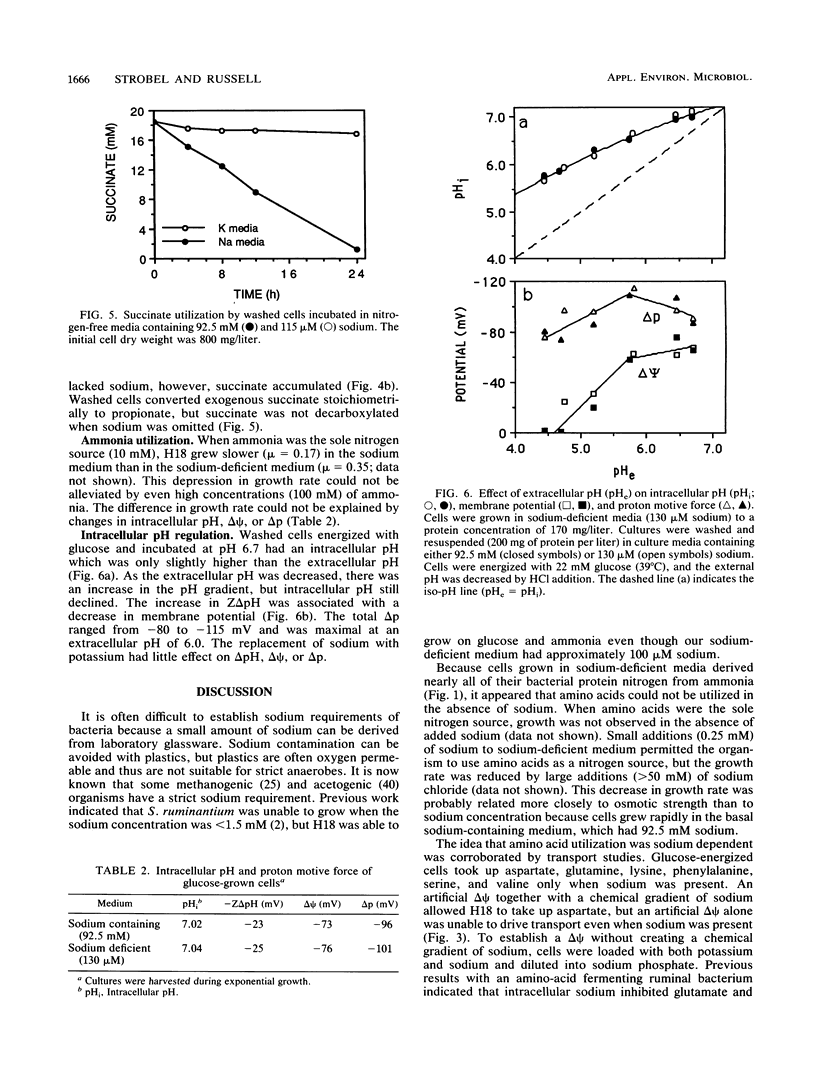
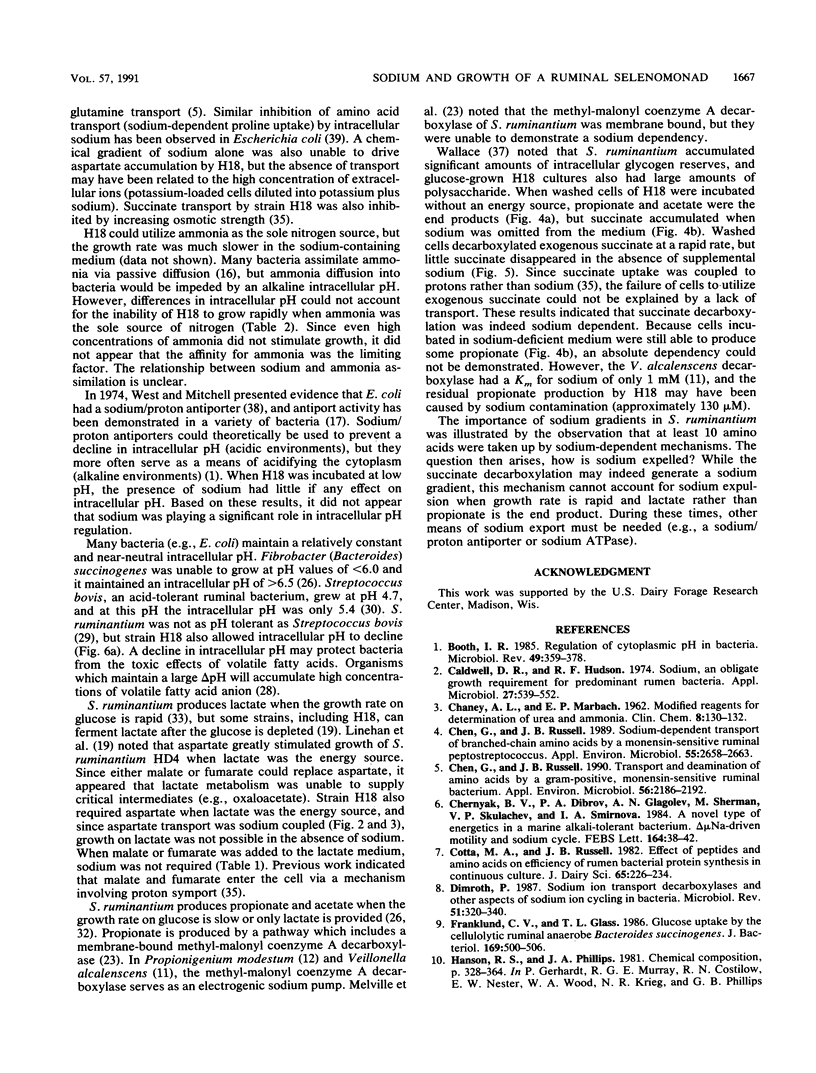
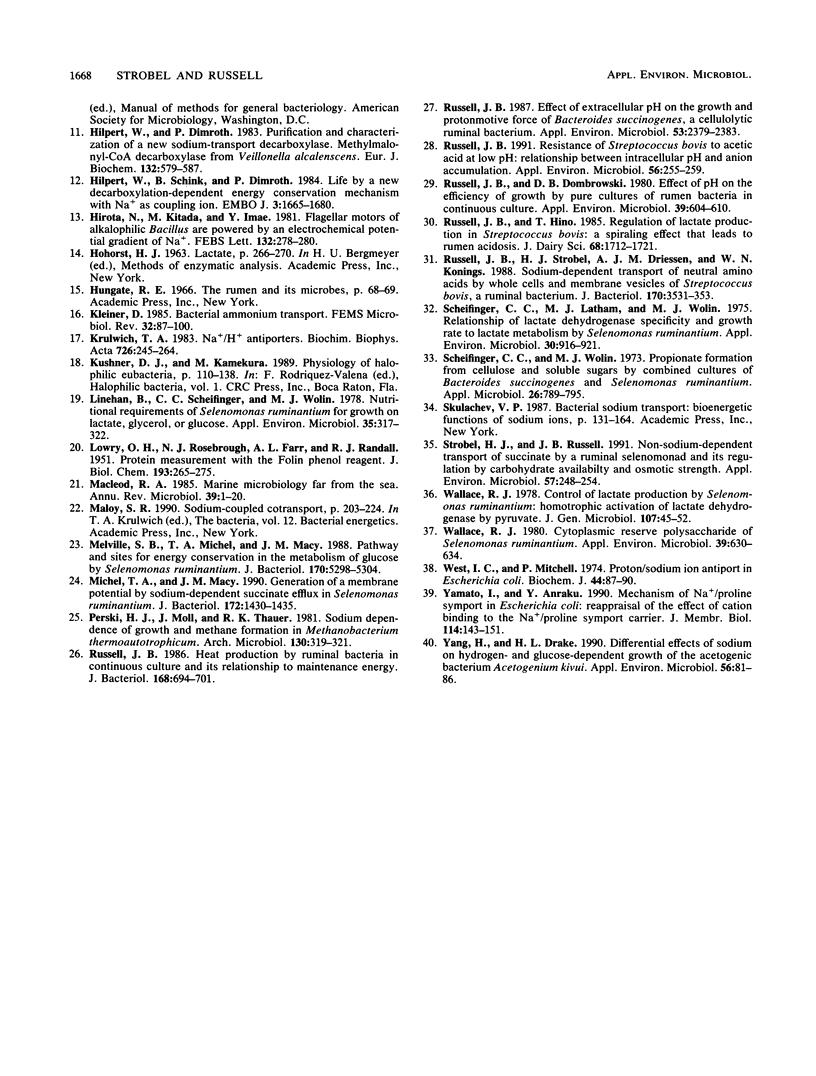
Selected References
These references are in PubMed. This may not be the complete list of references from this article.
- Booth I. R. Regulation of cytoplasmic pH in bacteria. Microbiol Rev. 1985 Dec;49(4):359–378. doi: 10.1128/mr.49.4.359-378.1985. [DOI] [PMC free article] [PubMed] [Google Scholar]
- CHANEY A. L., MARBACH E. P. Modified reagents for determination of urea and ammonia. Clin Chem. 1962 Apr;8:130–132. [PubMed] [Google Scholar]
- Caldwell D. R., Hudson R. F. Sodium, an obligate growth requirement for predominant rumen bacteria. Appl Microbiol. 1974 Mar;27(3):549–552. doi: 10.1128/am.27.3.549-552.1974. [DOI] [PMC free article] [PubMed] [Google Scholar]
- Chen G. J., Russell J. B. Sodium-dependent transport of branched-chain amino acids by a monensin-sensitive ruminal peptostreptococcus. Appl Environ Microbiol. 1989 Oct;55(10):2658–2663. doi: 10.1128/aem.55.10.2658-2663.1989. [DOI] [PMC free article] [PubMed] [Google Scholar]
- Chen G., Russell J. B. Transport and deamination of amino acids by a gram-positive, monensin-sensitive ruminal bacterium. Appl Environ Microbiol. 1990 Jul;56(7):2186–2192. doi: 10.1128/aem.56.7.2186-2192.1990. [DOI] [PMC free article] [PubMed] [Google Scholar]
- Dimroth P. Sodium ion transport decarboxylases and other aspects of sodium ion cycling in bacteria. Microbiol Rev. 1987 Sep;51(3):320–340. doi: 10.1128/mr.51.3.320-340.1987. [DOI] [PMC free article] [PubMed] [Google Scholar]
- Franklund C. V., Glass T. L. Glucose uptake by the cellulolytic ruminal anaerobe Bacteroides succinogenes. J Bacteriol. 1987 Feb;169(2):500–506. doi: 10.1128/jb.169.2.500-506.1987. [DOI] [PMC free article] [PubMed] [Google Scholar]
- Hilpert W., Dimroth P. Purification and characterization of a new sodium-transport decarboxylase. Methylmalonyl-CoA decarboxylase from Veillonella alcalescens. Eur J Biochem. 1983 May 16;132(3):579–587. doi: 10.1111/j.1432-1033.1983.tb07403.x. [DOI] [PubMed] [Google Scholar]
- Hilpert W., Schink B., Dimroth P. Life by a new decarboxylation-dependent energy conservation mechanism with Na as coupling ion. EMBO J. 1984 Aug;3(8):1665–1670. doi: 10.1002/j.1460-2075.1984.tb02030.x. [DOI] [PMC free article] [PubMed] [Google Scholar]
- Krulwich T. A. Na+/H+ antiporters. Biochim Biophys Acta. 1983 Dec 30;726(4):245–264. doi: 10.1016/0304-4173(83)90011-3. [DOI] [PubMed] [Google Scholar]
- LOWRY O. H., ROSEBROUGH N. J., FARR A. L., RANDALL R. J. Protein measurement with the Folin phenol reagent. J Biol Chem. 1951 Nov;193(1):265–275. [PubMed] [Google Scholar]
- Linehan B., Scheifinger C. C., Wolin M. J. Nutritional Requirements of Selenomonas ruminantium for Growth on Lactate, Glycerol, or Glucose. Appl Environ Microbiol. 1978 Feb;35(2):317–322. doi: 10.1128/aem.35.2.317-322.1978. [DOI] [PMC free article] [PubMed] [Google Scholar]
- MacLeod R. A. Marine microbiology far from the sea. Annu Rev Microbiol. 1985;39:1–20. doi: 10.1146/annurev.mi.39.100185.000245. [DOI] [PubMed] [Google Scholar]
- Melville S. B., Michel T. A., Macy J. M. Pathway and sites for energy conservation in the metabolism of glucose by Selenomonas ruminantium. J Bacteriol. 1988 Nov;170(11):5298–5304. doi: 10.1128/jb.170.11.5298-5304.1988. [DOI] [PMC free article] [PubMed] [Google Scholar]
- Michel T. A., Macy J. M. Generation of a membrane potential by sodium-dependent succinate efflux in Selenomonas ruminantium. J Bacteriol. 1990 Mar;172(3):1430–1435. doi: 10.1128/jb.172.3.1430-1435.1990. [DOI] [PMC free article] [PubMed] [Google Scholar]
- Russell J. B., Dombrowski D. B. Effect of pH on the efficiency of growth by pure cultures of rumen bacteria in continuous culture. Appl Environ Microbiol. 1980 Mar;39(3):604–610. doi: 10.1128/aem.39.3.604-610.1980. [DOI] [PMC free article] [PubMed] [Google Scholar]
- Russell J. B. Effect of extracellular pH on growth and proton motive force of Bacteroides succinogenes, a cellulolytic ruminal bacterium. Appl Environ Microbiol. 1987 Oct;53(10):2379–2383. doi: 10.1128/aem.53.10.2379-2383.1987. [DOI] [PMC free article] [PubMed] [Google Scholar]
- Russell J. B. Heat production by ruminal bacteria in continuous culture and its relationship to maintenance energy. J Bacteriol. 1986 Nov;168(2):694–701. doi: 10.1128/jb.168.2.694-701.1986. [DOI] [PMC free article] [PubMed] [Google Scholar]
- Russell J. B. Resistance of Streptococcus bovis to acetic acid at low pH: relationship between intracellular pH and anion accumulation. Appl Environ Microbiol. 1991 Jan;57(1):255–259. doi: 10.1128/aem.57.1.255-259.1991. [DOI] [PMC free article] [PubMed] [Google Scholar]
- Russell J. B., Strobel H. J., Driessen A. J., Konings W. N. Sodium-dependent transport of neutral amino acids by whole cells and membrane vesicles of Streptococcus bovis, a ruminal bacterium. J Bacteriol. 1988 Aug;170(8):3531–3536. doi: 10.1128/jb.170.8.3531-3536.1988. [DOI] [PMC free article] [PubMed] [Google Scholar]
- Scheifinger C. C., Wolin M. J. Propionate formation from cellulose and soluble sugars by combined cultures of Bacteroides succinogenes and Selenomonas ruminantium. Appl Microbiol. 1973 Nov;26(5):789–795. doi: 10.1128/am.26.5.789-795.1973. [DOI] [PMC free article] [PubMed] [Google Scholar]
- Strobel H. J., Russell J. B. Succinate transport by a ruminal selenomonad and its regulation by carbohydrate availability and osmotic strength. Appl Environ Microbiol. 1991 Jan;57(1):248–254. doi: 10.1128/aem.57.1.248-254.1991. [DOI] [PMC free article] [PubMed] [Google Scholar]
- Wallace R. J. Control of lactate production by Selenomonas ruminantium: homotropic activation of lactate dehydrogenase by pyruvate. J Gen Microbiol. 1978 Jul;107(1):45–52. doi: 10.1099/00221287-107-1-45. [DOI] [PubMed] [Google Scholar]
- Wallace R. J. Cytoplasmic reserve polysaccharide of Selenomonas ruminantium. Appl Environ Microbiol. 1980 Mar;39(3):630–634. doi: 10.1128/aem.39.3.630-634.1980. [DOI] [PMC free article] [PubMed] [Google Scholar]
- West I. C., Mitchell P. Proton/sodium ion antiport in Escherichia coli. Biochem J. 1974 Oct;144(1):87–90. doi: 10.1042/bj1440087. [DOI] [PMC free article] [PubMed] [Google Scholar]
- Yamato I., Anraku Y. Mechanism of Na+/proline symport in Escherichia coli: reappraisal of the effect of cation binding to the Na+/proline symport carrier. J Membr Biol. 1990 Mar;114(2):143–151. doi: 10.1007/BF01869095. [DOI] [PubMed] [Google Scholar]
- Yang H. C., Drake H. L. Differential effects of sodium on hydrogen- and glucose-dependent growth of the acetogenic bacterium Acetogenium kivui. Appl Environ Microbiol. 1990 Jan;56(1):81–86. doi: 10.1128/aem.56.1.81-86.1990. [DOI] [PMC free article] [PubMed] [Google Scholar]