Abstract
Thienamycin (THM), the N-formimidoyl thienamycin derivative MK0787, and related carbapenem antibiotics were metabolized extensively in mice, rats, rabbits, dogs, rhesus monkeys, and chimpanzees. Urinary recovery of THM ranged from a low of 5% in dogs to 58% in rhesus monkeys. Renal clearance rates in dogs and chimpanzees were unusually low, less than glomerular filtration rates. The reduction in clearance of THM and MK0787 from plasma of rats and rabbits after ligation of renal arteries indicate that the kidneys are responsible for 35 and 92%, respectively, of metabolic drug clearance. Degradation was detected only in kidney homogenates. The enzyme activity was membrane bound and sensitive to inhibitors of Zn-metalloenzymes such as EDTA. A renal dipeptidase, dehydropeptidase-I (DHP-I), EC 3.4.13.11, was found to be responsible for the metabolism of the THM-class antibiotics, which exhibit a structural homology to dehydropeptides. A parallel increase in specific activity against THM and the substrate of DHP-I, glycyldehydrophenylalanine, was observed during solubilization and purification of the enzyme from porcine and human renal cortex. DHP-I was found to catalyze the hydrolysis of the beta-lactam ring in THM and MK0787. The products of the enzyme reaction were identical by high-powered liquid chromatography to their respective metabolites found in the urine. Nonbasic N-acylated THM and natural N-acylated carbapenems (epithienamycins and olivanic acids) were degraded 4- to 50-fold faster than THM when exposed to the enzymatic hydrolysis of DHP-I. Good correlations were obtained between the increased susceptibility of the carbapenem antibiotics to DHP-I as measured in the in vitro enzyme assay and the generally lower recoveries of active antibiotic in the urine of test animals. Despite this unusual degree of metabolism localized in the kidney, the plasma half-life of MK0787 and its efficacy against experimental systemic infections in animals remain satisfactory.
Full text
PDF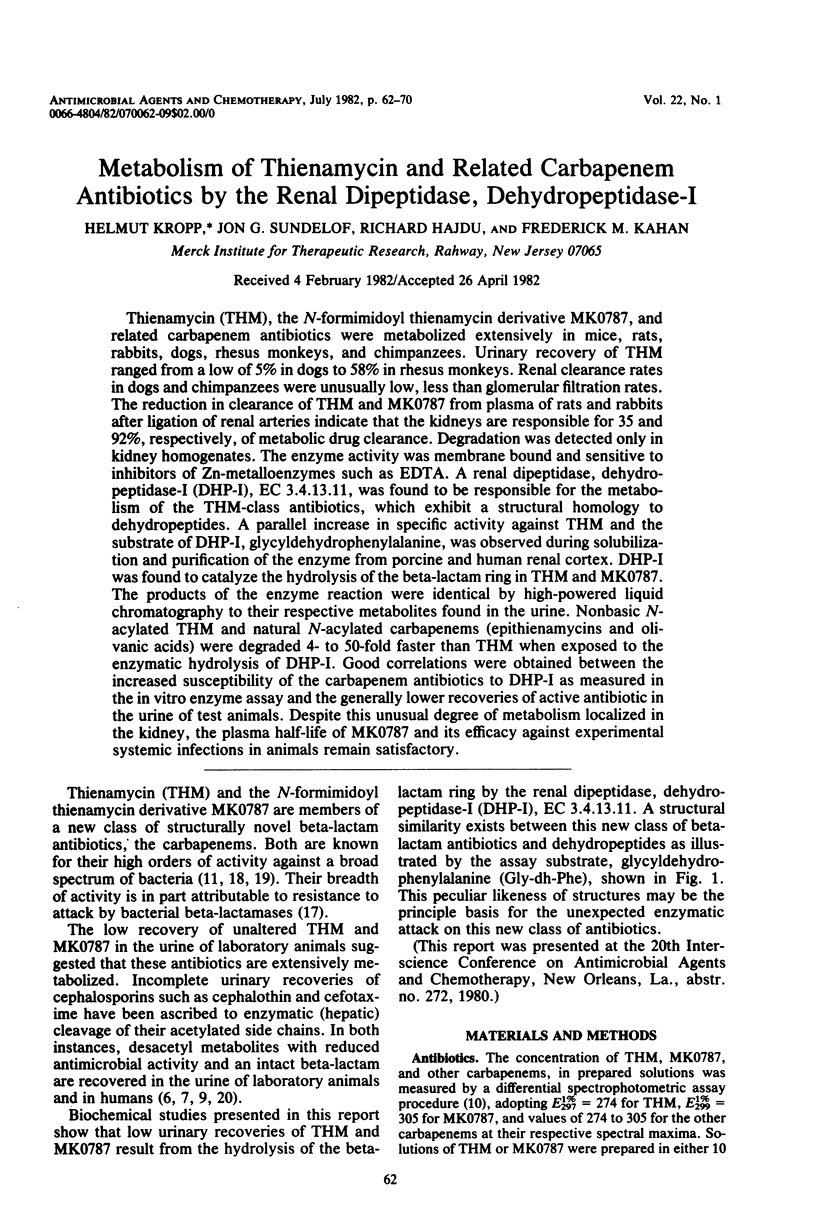
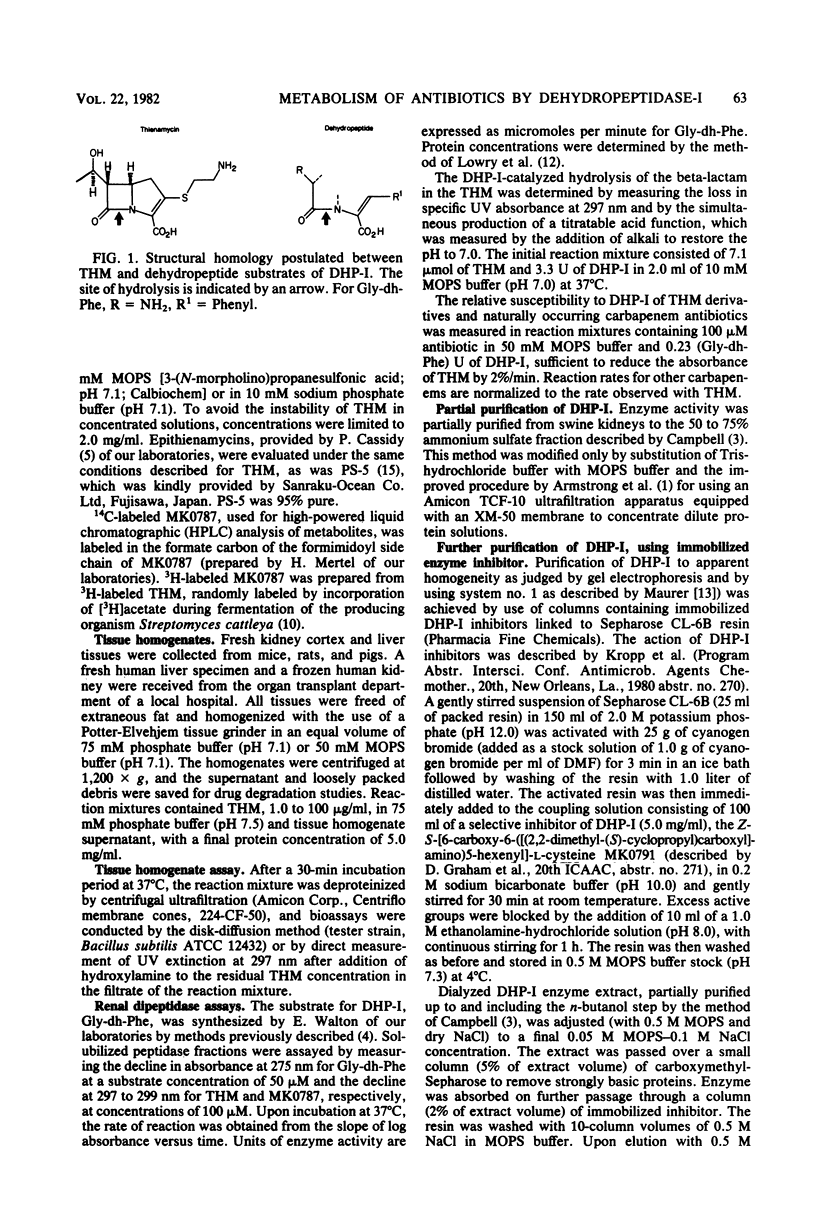
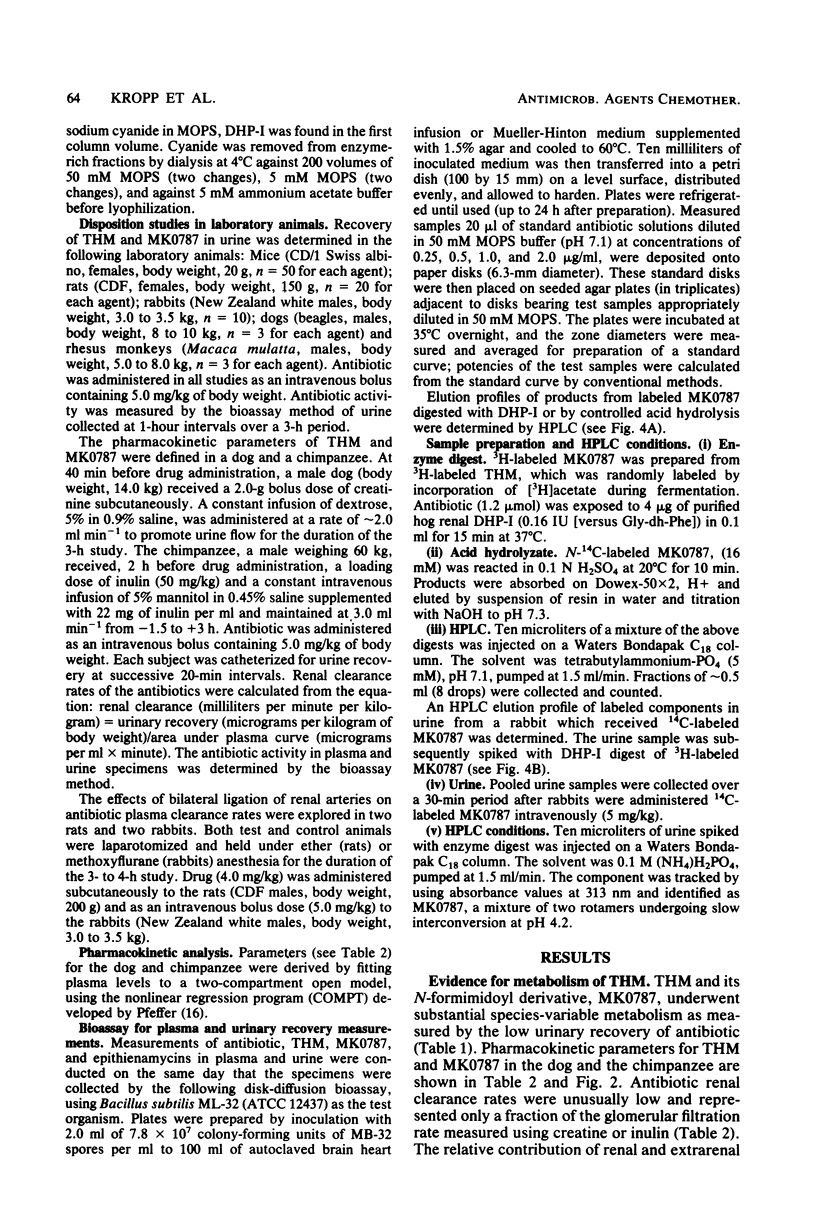
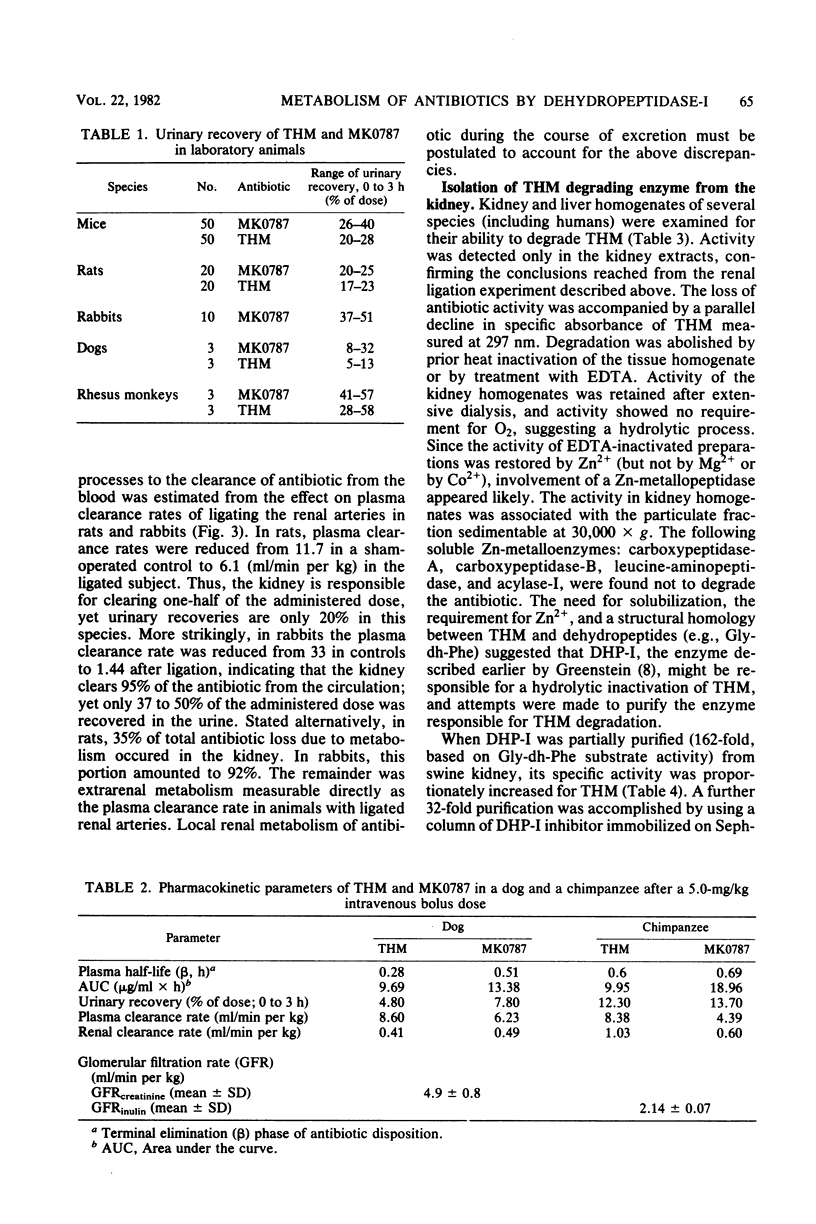
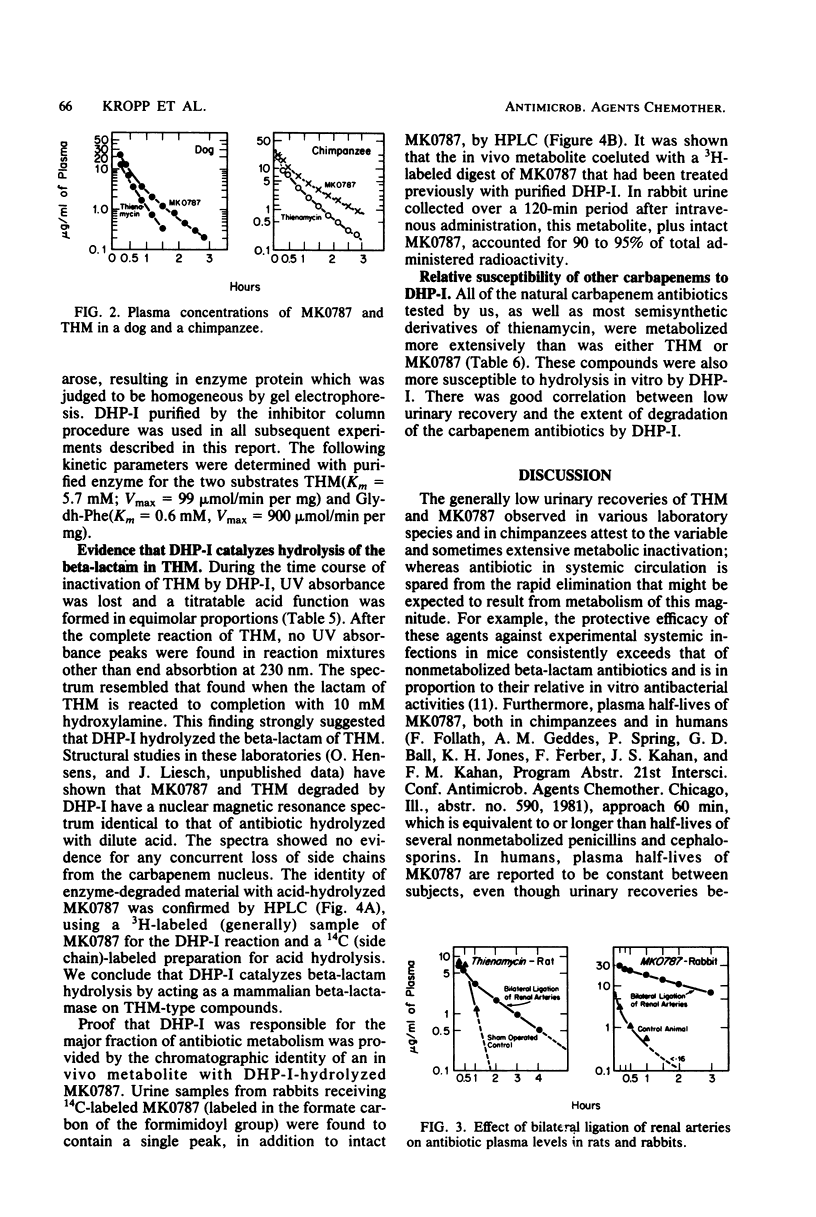
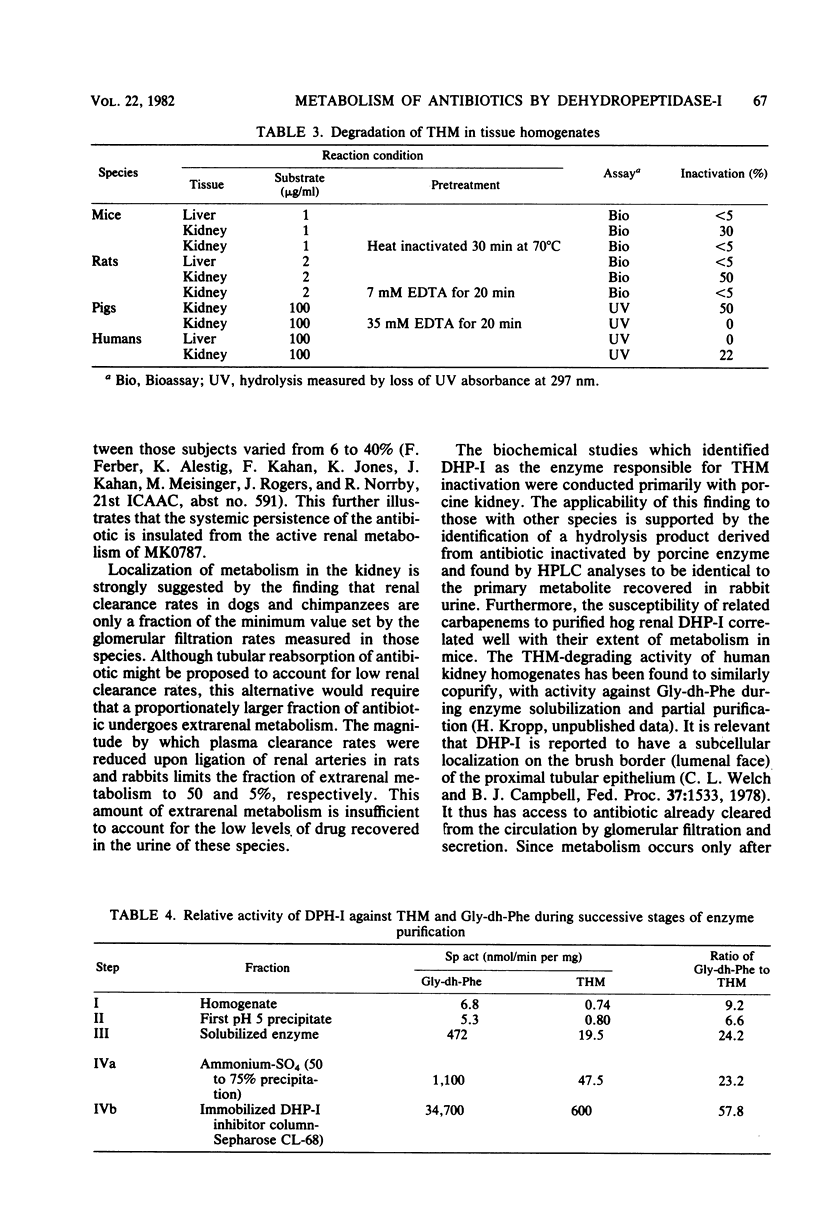
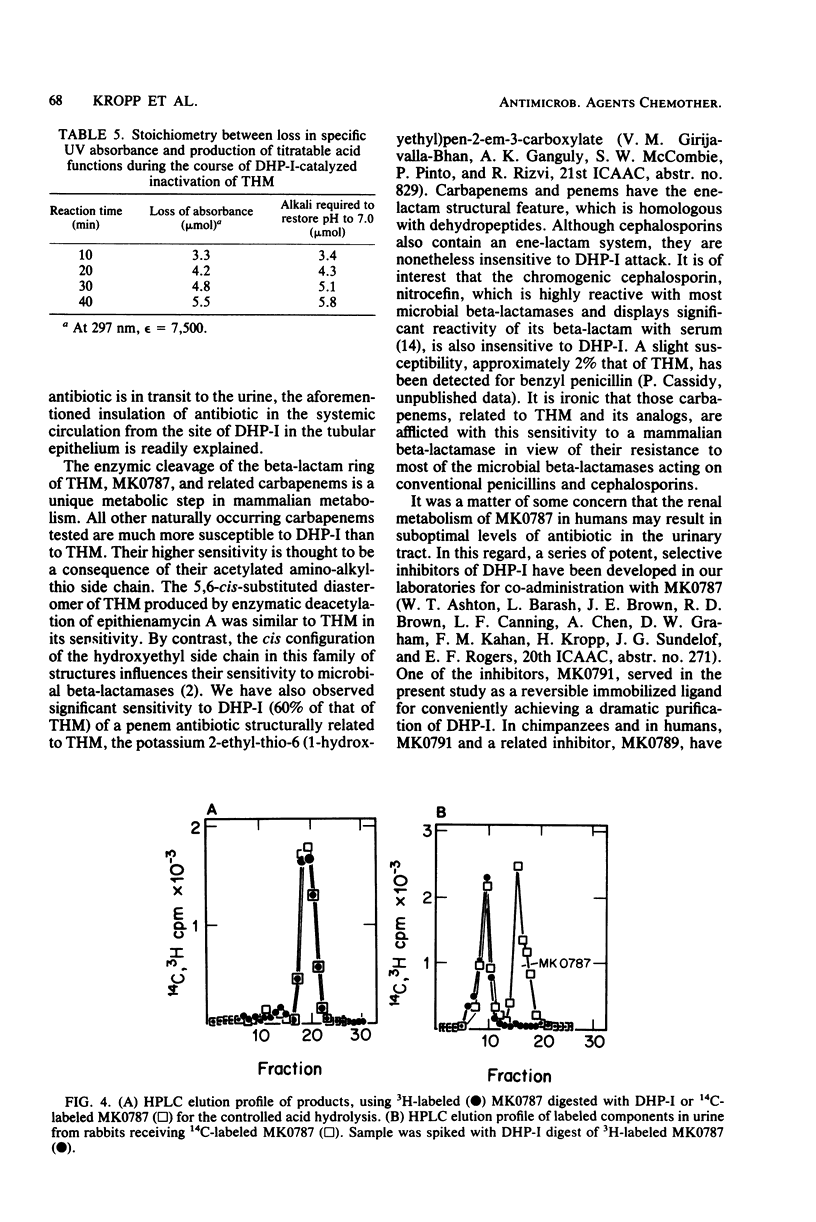
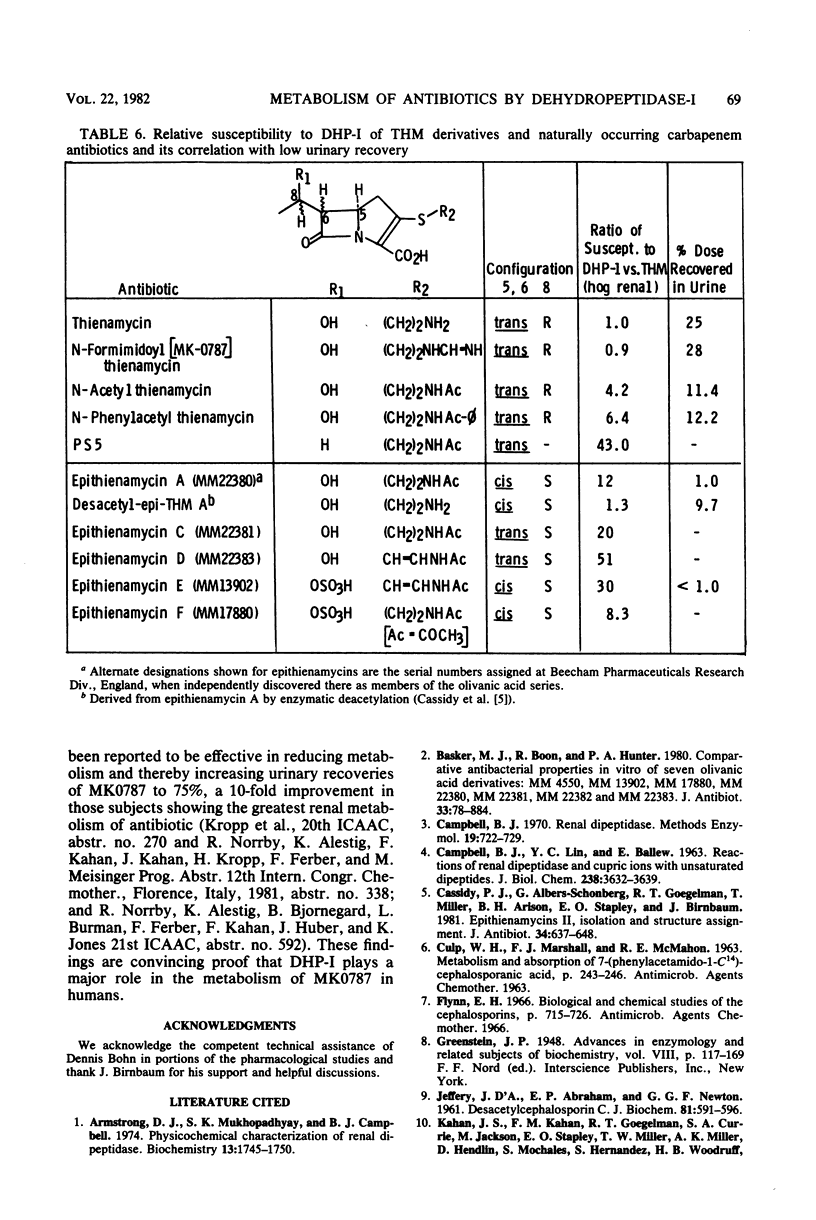
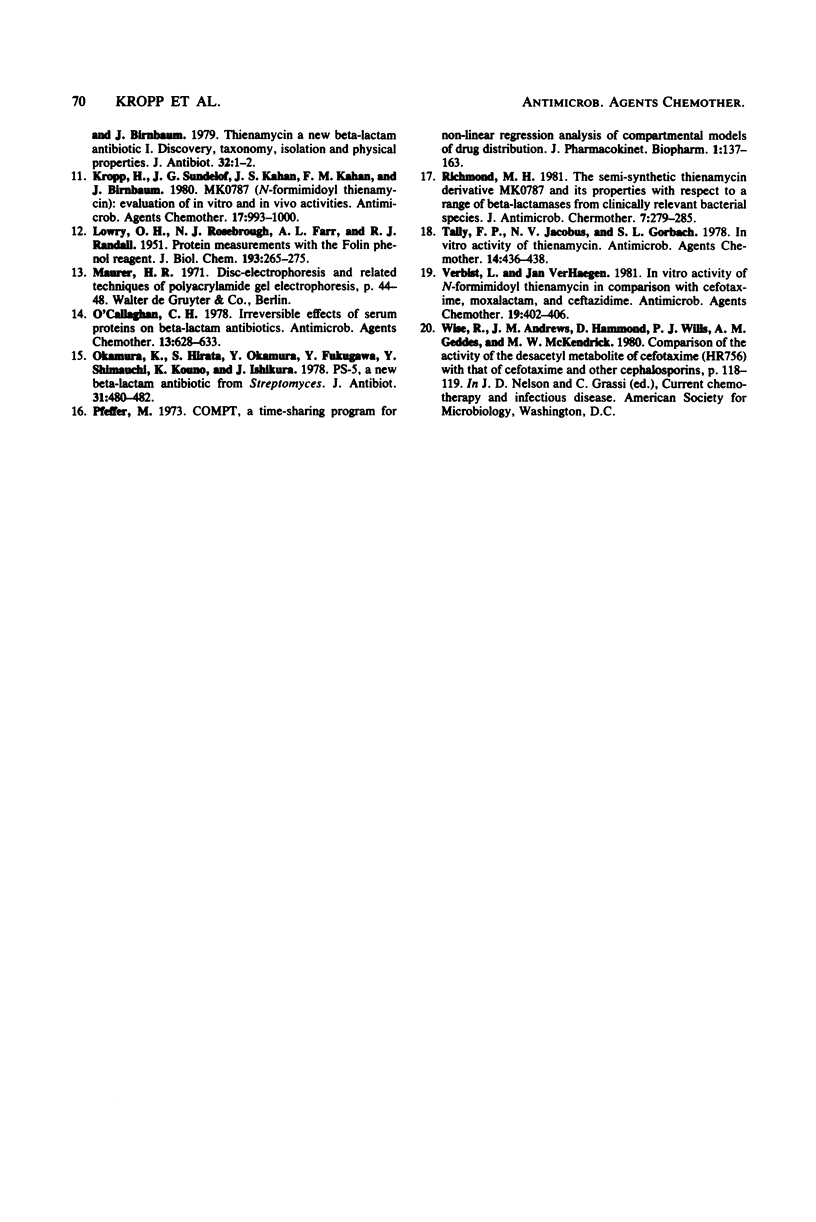
Selected References
These references are in PubMed. This may not be the complete list of references from this article.
- Armstrong D. J., Mukhopadhyay S. K., Campbell B. J. Physicochemical characterization of renal dipeptidase. Biochemistry. 1974 Apr 9;13(8):1745–1750. doi: 10.1021/bi00705a029. [DOI] [PubMed] [Google Scholar]
- Basker M. J., Boon R. J., Hunter P. A. Comparative antibacterial properties in vitro of seven olivanic acid derivatives: MM 4550, MM 13902, MM 17880, MM 22380, MM 22381, MM 22382 and MM 22383. J Antibiot (Tokyo) 1980 Aug;33(8):878–884. doi: 10.7164/antibiotics.33.878. [DOI] [PubMed] [Google Scholar]
- CAMPBELL B. J., LIN Y. C., BIRD M. E. RENAL AMINOPEPTIDASE- AND COPPER-ACTIVATED PEPTIDE HYDROLYSIS. J Biol Chem. 1963 Nov;238:3632–3639. [PubMed] [Google Scholar]
- CULP H. W., MARSHALL F. J., MCMAHON R. E. METABOLISM AND ABSORPTION OF 7-(PHENYLACETAMIDO-1-C14)-CEPHALOSPORANIC ACID. Antimicrob Agents Chemother (Bethesda) 1963;161:243–246. [PubMed] [Google Scholar]
- Cassidy P. J., Albers-Schonberg G., Goegelman R. T., Miller T., Arison B., Stapley E. O., Birnbaum J. Epithienamycins. II. Isolation and structure assignment. J Antibiot (Tokyo) 1981 Jun;34(6):637–648. doi: 10.7164/antibiotics.34.637. [DOI] [PubMed] [Google Scholar]
- Flynn E. H. Biological and chemical studies of the cephalosporins. Antimicrob Agents Chemother (Bethesda) 1966;6:715–726. [PubMed] [Google Scholar]
- JEFFERY J. D., ABRAHAM E. P., NEWTON G. G. Deacetylcephalosporin C. Biochem J. 1961 Dec;81:591–596. doi: 10.1042/bj0810591. [DOI] [PMC free article] [PubMed] [Google Scholar]
- Kropp H., Sundelof J. G., Kahan J. S., Kahan F. M., Birnbaum J. MK0787 (N-formimidoyl thienamycin): evaluation of in vitro and in vivo activities. Antimicrob Agents Chemother. 1980 Jun;17(6):993–1000. doi: 10.1128/aac.17.6.993. [DOI] [PMC free article] [PubMed] [Google Scholar]
- LOWRY O. H., ROSEBROUGH N. J., FARR A. L., RANDALL R. J. Protein measurement with the Folin phenol reagent. J Biol Chem. 1951 Nov;193(1):265–275. [PubMed] [Google Scholar]
- O'Callaghan C. H. Irreversible effects of serum proteins on beta-lactam antibiotics. Antimicrob Agents Chemother. 1978 Apr;13(4):628–633. doi: 10.1128/aac.13.4.628. [DOI] [PMC free article] [PubMed] [Google Scholar]
- Okamura K., Hirata S., Okumura Y., Fukagawa Y., Shimauchi Y., Kouno K., Ishikura T. PS-5, a new beta-lactam antibiotic from Streptomyces. J Antibiot (Tokyo) 1978 May;31(5):480–482. doi: 10.7164/antibiotics.31.480. [DOI] [PubMed] [Google Scholar]
- Pfeffer M. COMPT, a time-sharing program for nonlinear regression analysis of compartmental models of drug distribution. J Pharmacokinet Biopharm. 1973 Apr;1(2):137–163. doi: 10.1007/BF01059627. [DOI] [PubMed] [Google Scholar]
- Tally F. P., Jacobus N. V., Gorbach S. L. In vitro activity of thienamycin. Antimicrob Agents Chemother. 1978 Sep;14(3):436–438. doi: 10.1128/aac.14.3.436. [DOI] [PMC free article] [PubMed] [Google Scholar]
- Verbist L., Verhaegen J. In vitro activity of N-formimidoyl thienamycin in comparison with cefotaxime, moxalactam, and ceftazidime. Antimicrob Agents Chemother. 1981 Mar;19(3):402–406. doi: 10.1128/aac.19.3.402. [DOI] [PMC free article] [PubMed] [Google Scholar]