Abstract
The physiology of Saccharomyces cerevisiae CBS 8066 was studied in glucose-limited chemostat cultures. Below a dilution rate of 0.30 h-1 glucose was completely respired, and biomass and CO2 were the only products formed. Above this dilution rate acetate and pyruvate appeared in the culture fluid, accompanied by disproportional increases in the rates of oxygen consumption and carbon dioxide production. This enhanced respiratory activity was accompanied by a drop in cell yield from 0.50 to 0.47 g (dry weight) g of glucose-1. At a dilution rate of 0.38 h-1 the culture reached its maximal oxidation capacity of 12 mmol of O2 g (dry weight)-1 h-1. A further increase in the dilution rate resulted in aerobic alcoholic fermentation in addition to respiration, accompanied by an additional decrease in cell yield from 0.47 to 0.16 g (dry weight) g of glucose-1. Since the high respiratory activity of the yeast at intermediary dilution rates would allow for full respiratory metabolism of glucose up to dilution rates close to mumax, we conclude that the occurrence of alcoholic fermentation is not primarily due to a limited respiratory capacity. Rather, organic acids produced by the organism may have an uncoupling effect on its respiration. As a result the respiratory activity is enhanced and reaches its maximum at a dilution rate of 0.38 h-1. An attempt was made to interpret the dilution rate-dependent formation of ethanol and acetate in glucose-limited chemostat cultures of S. cerevisiae CBS 8066 as an effect of overflow metabolism at the pyruvate level. Therefore, the activities of pyruvate decarboxylase, NAD+- and NADP+-dependent acetaldehyde dehydrogenases, acetyl coenzyme A (acetyl-CoA) synthetase, and alcohol dehydrogenase were determined in extracts of cells grown at various dilution rates. From the enzyme profiles, substrate affinities, and calculated intracellular pyruvate concentrations, the following conclusions were drawn with respect to product formation of cells growing under glucose limitation. (i) Pyruvate decarboxylase, the key enzyme of alcoholic fermentation, probably already is operative under conditions in which alcoholic fermentation is absent. The acetaldehyde produced by the enzyme is then oxidized via acetaldehyde dehydrogenases and acetyl-CoA synthetase. The acetyl-CoA thus formed is further oxidized in the mitochondria. (ii) Acetate formation results from insufficient activity of acetyl-CoA synthetase, required for the complete oxidation of acetate. Ethanol formation results from insufficient activity of acetaldehyde dehydrogenases.(ABSTRACT TRUNCATED AT 400 WORDS)
Full text
PDF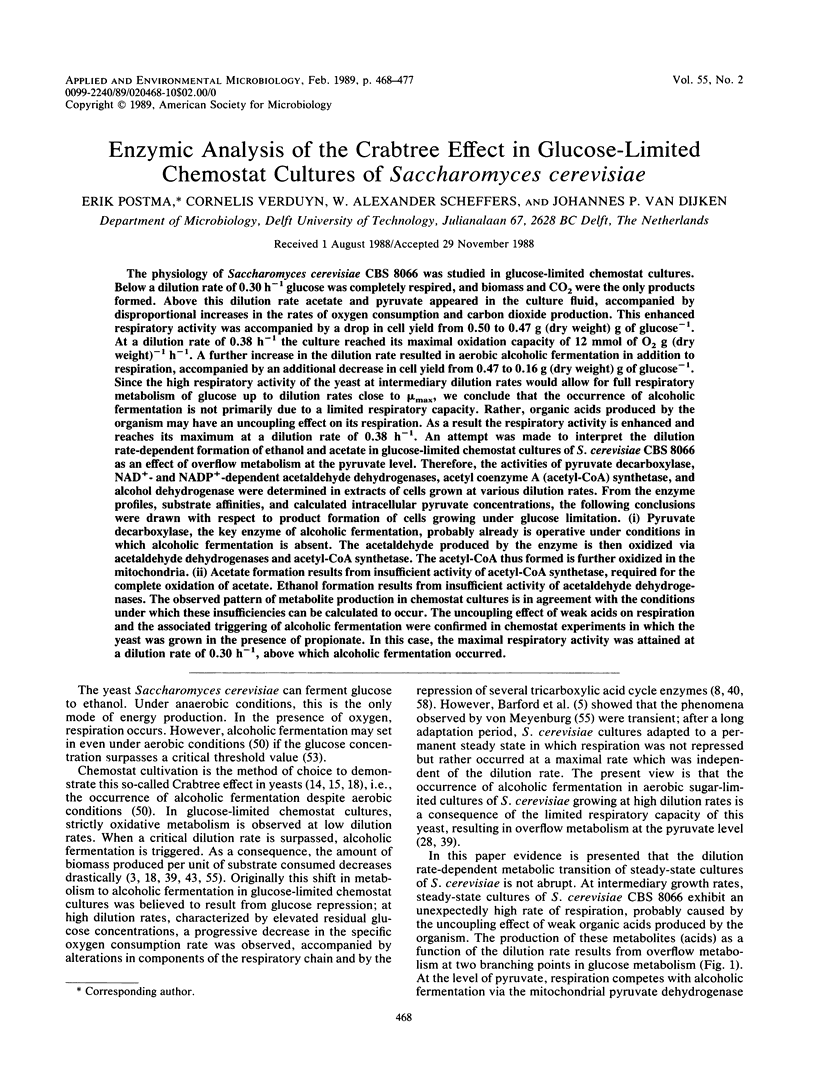
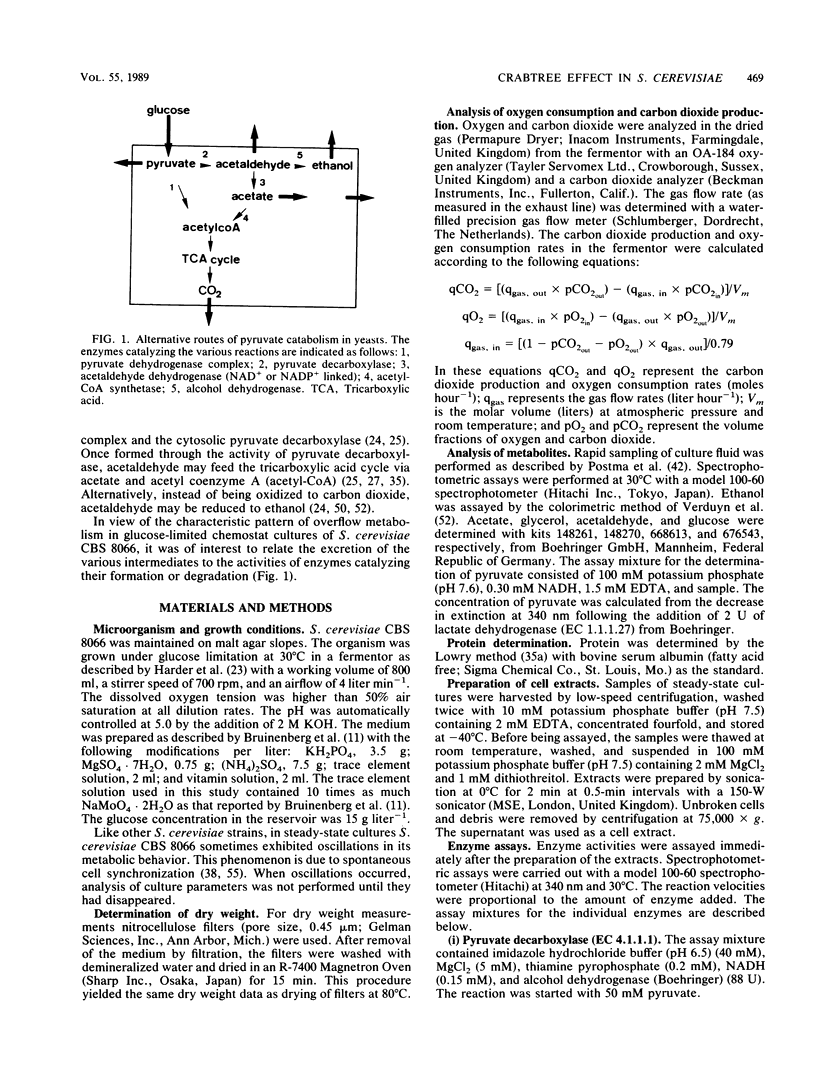
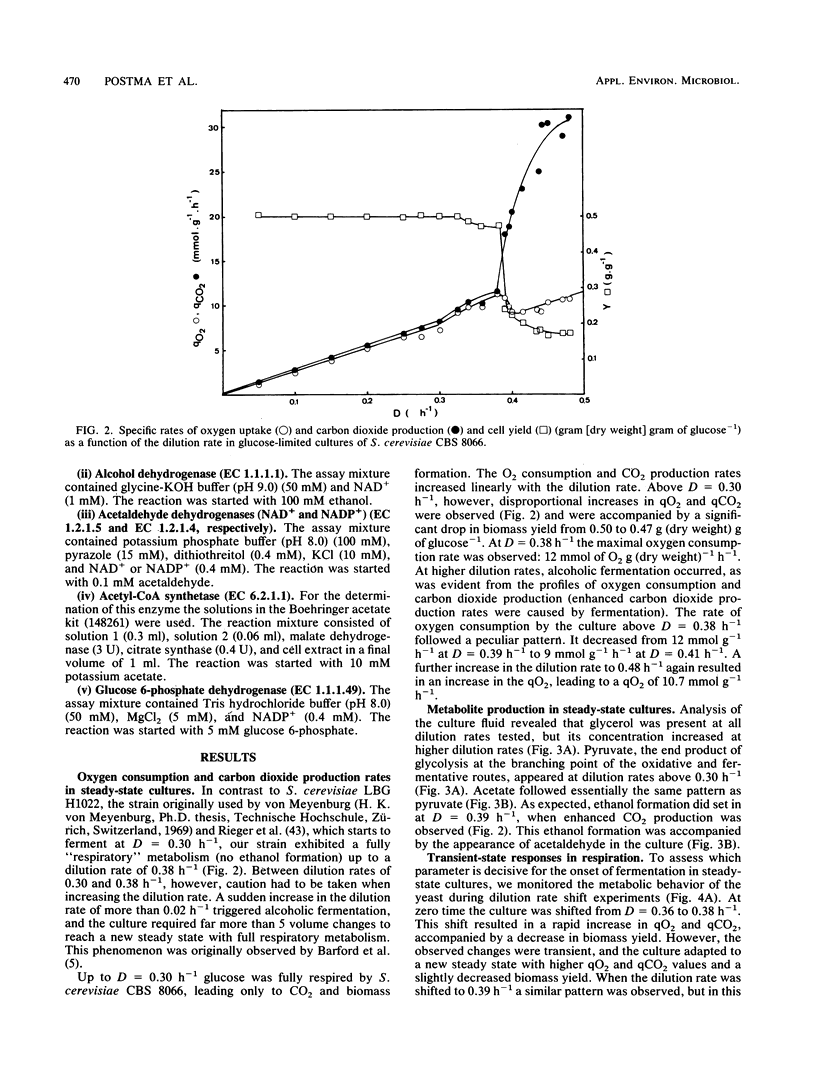
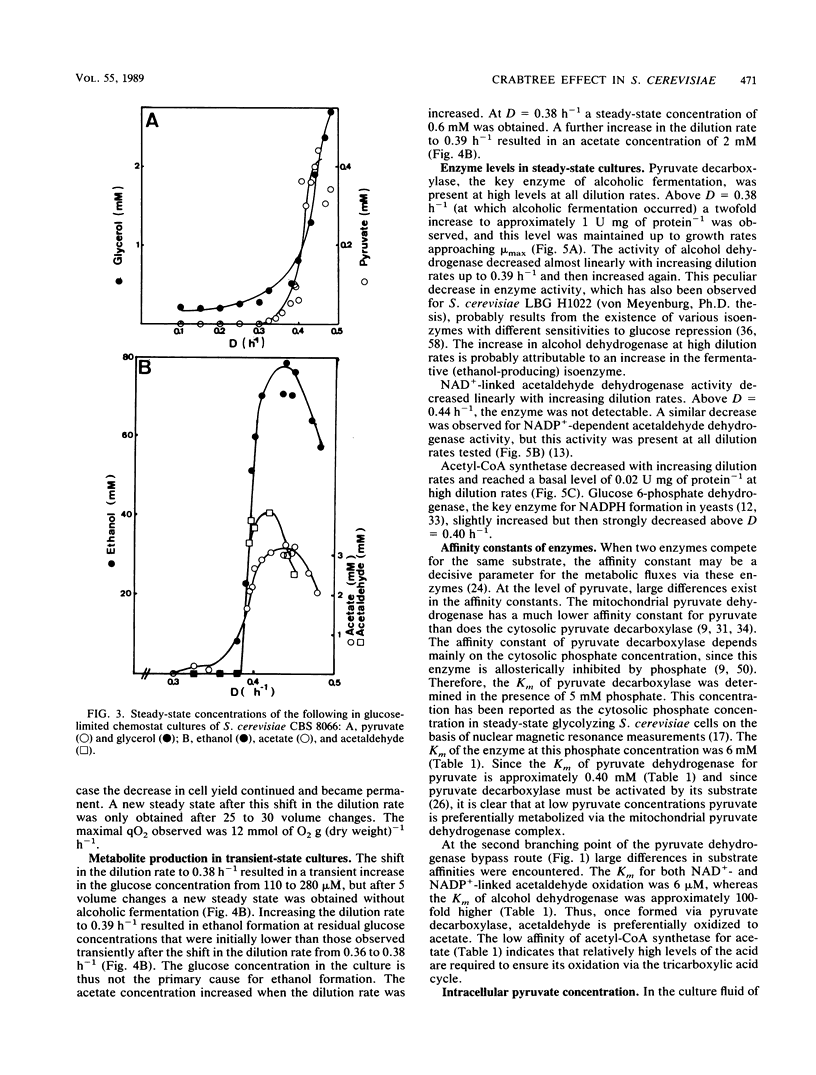
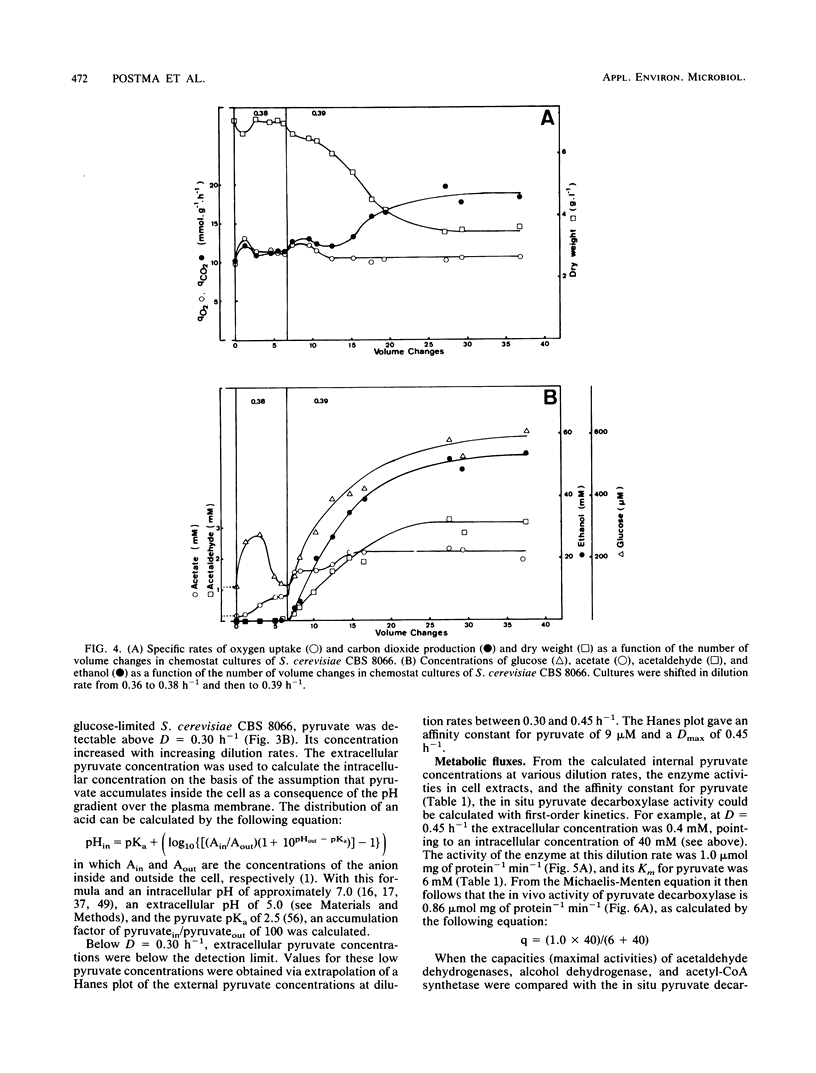
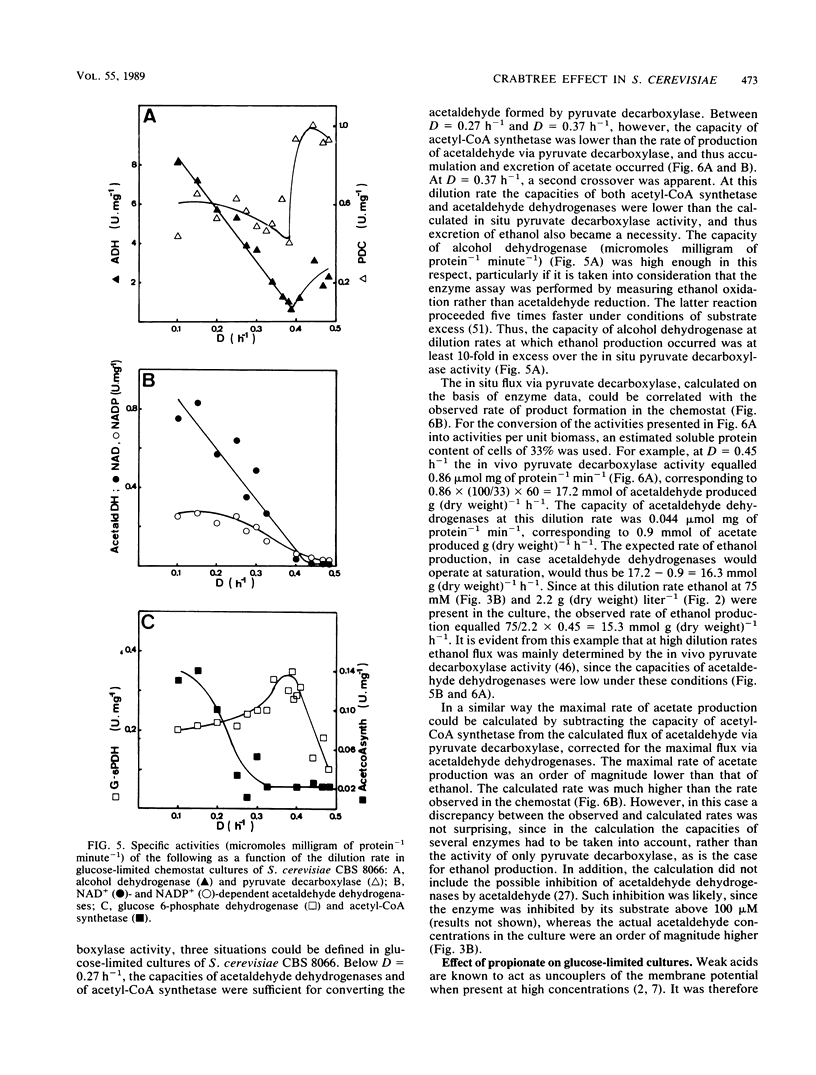
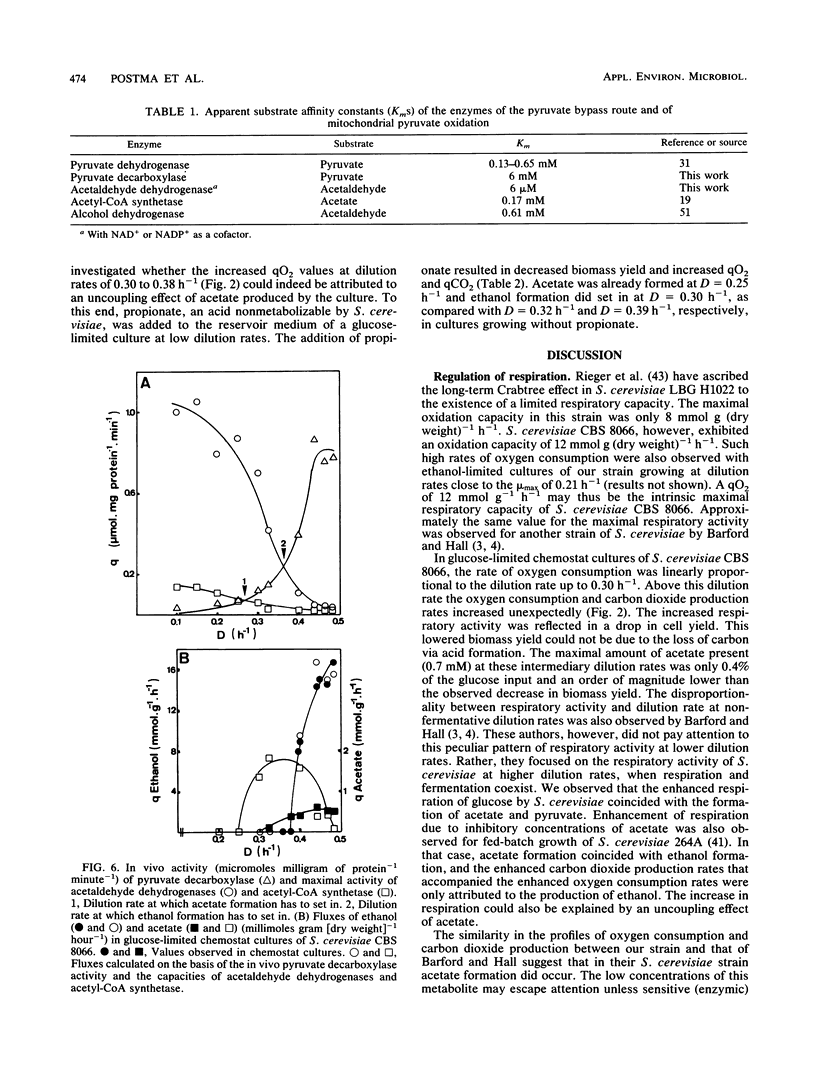
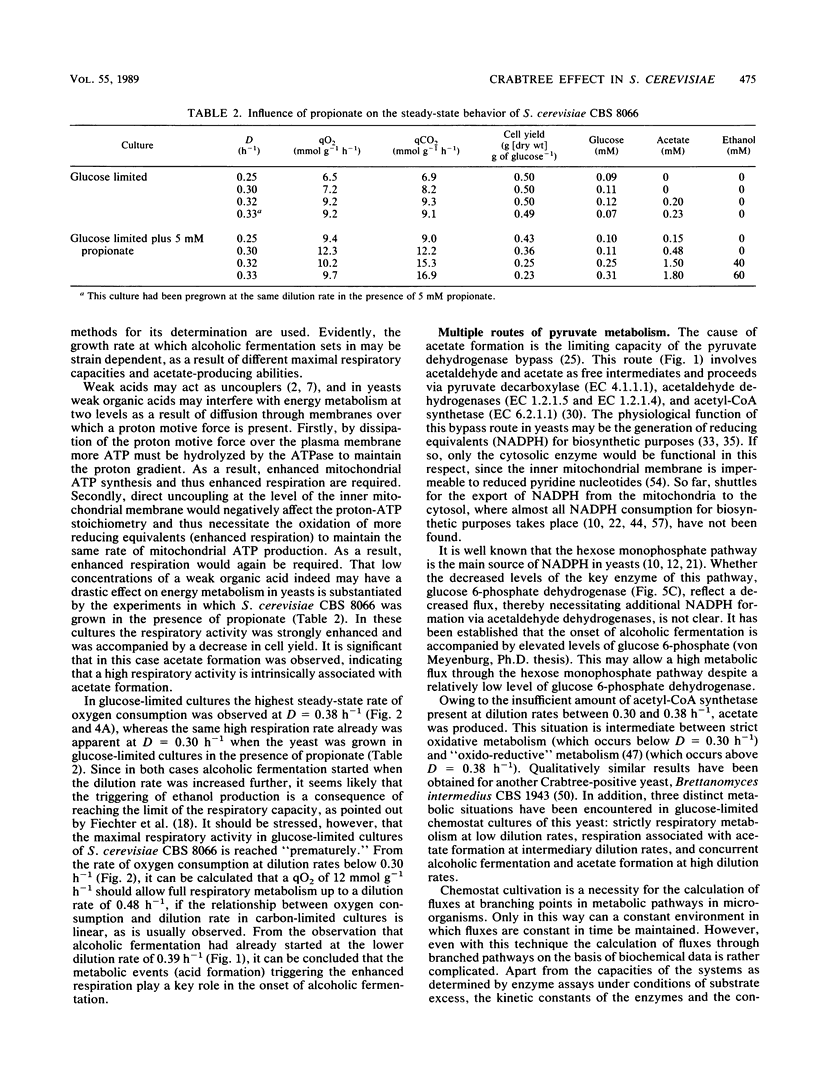
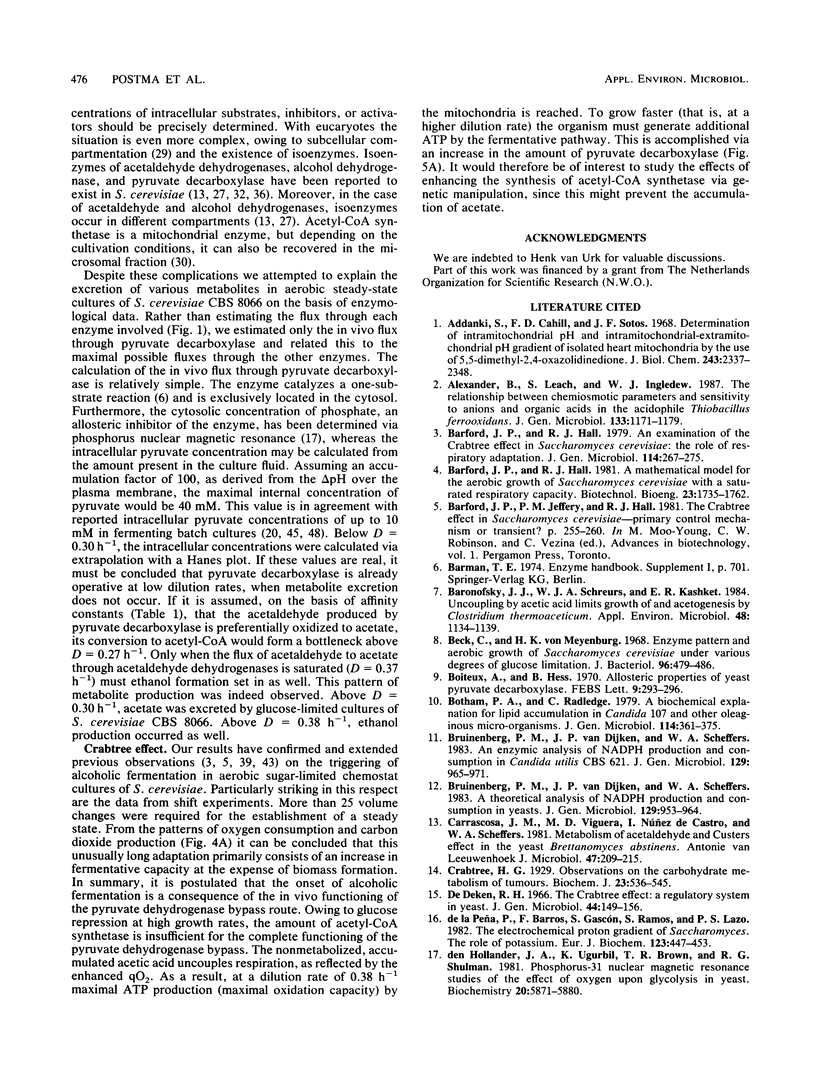
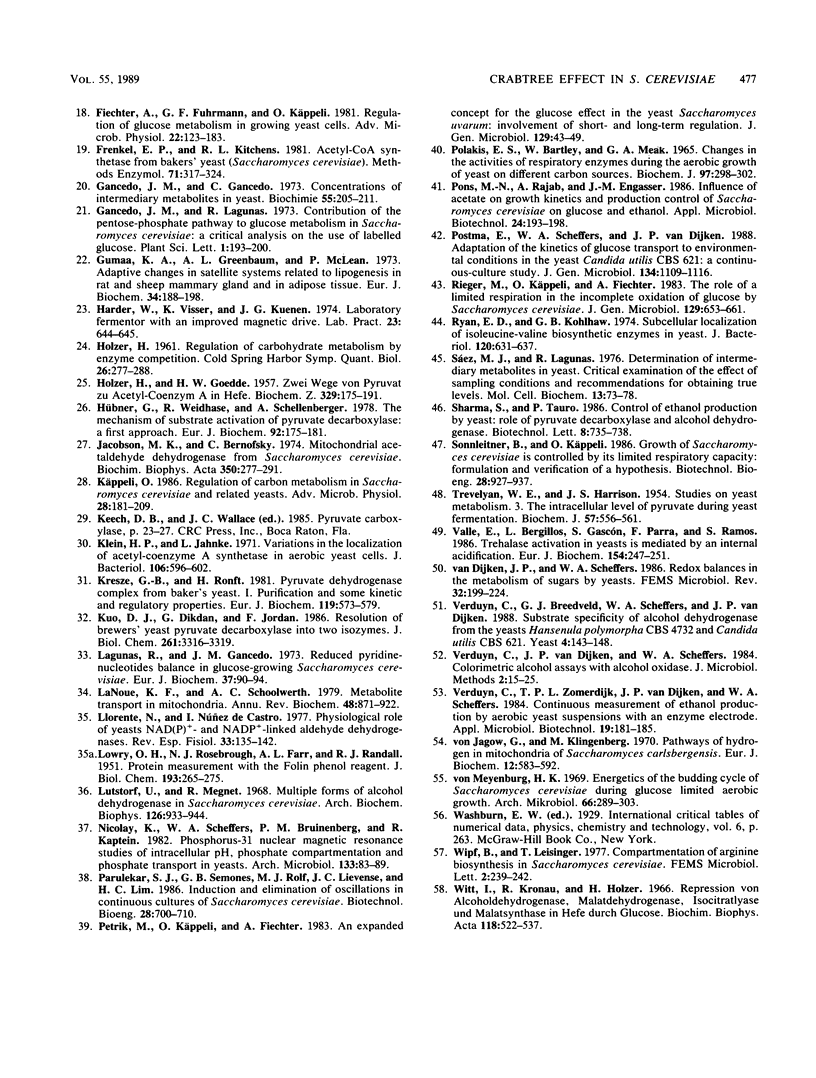
Selected References
These references are in PubMed. This may not be the complete list of references from this article.
- Addanki A., Cahill F. D., Sotos J. F. Determination of intramitochondrial pH and intramitochondrial-extramitochondrial pH gradient of isolated heart mitochondria by the use of 5,5-dimethyl-2,4-oxazolidinedione. I. Changes during respiration and adenosine triphosphate-dependent transport of Ca++, Mg++, and Zn++. J Biol Chem. 1968 May 10;243(9):2337–2348. [PubMed] [Google Scholar]
- Baronofsky J. J., Schreurs W. J., Kashket E. R. Uncoupling by Acetic Acid Limits Growth of and Acetogenesis by Clostridium thermoaceticum. Appl Environ Microbiol. 1984 Dec;48(6):1134–1139. doi: 10.1128/aem.48.6.1134-1139.1984. [DOI] [PMC free article] [PubMed] [Google Scholar]
- Beck C., von Meyenburg H. K. Enzyme pattern and aerobic growth of Saccharomyces cerevisiae under various degrees of glucose limitation. J Bacteriol. 1968 Aug;96(2):479–486. doi: 10.1128/jb.96.2.479-486.1968. [DOI] [PMC free article] [PubMed] [Google Scholar]
- Boiteux A., Hess B. Allosteric properties of yeast pyruvate decarboxylase. FEBS Lett. 1970 Aug 31;9(5):293–296. doi: 10.1016/0014-5793(70)80381-7. [DOI] [PubMed] [Google Scholar]
- Botham P. A., Ratledge C. A biochemical explanation for lipid accumulation in Candida 107 and other oleaginous micro-organisms. J Gen Microbiol. 1979 Oct;114(2):361–375. doi: 10.1099/00221287-114-2-361. [DOI] [PubMed] [Google Scholar]
- Bruinenberg P. M., van Dijken J. P., Scheffers W. A. An enzymic analysis of NADPH production and consumption in Candida utilis. J Gen Microbiol. 1983 Apr;129(4):965–971. doi: 10.1099/00221287-129-4-965. [DOI] [PubMed] [Google Scholar]
- Carrascosa J. M., Viguera M. D., Núez de Castro I., Scheffers W. A. Metabolism of acetaldehyde and custers effect in the yeast. Antonie Van Leeuwenhoek. 1981;47(3):209–215. doi: 10.1007/BF00403392. [DOI] [PubMed] [Google Scholar]
- Crabtree H. G. Observations on the carbohydrate metabolism of tumours. Biochem J. 1929;23(3):536–545. doi: 10.1042/bj0230536. [DOI] [PMC free article] [PubMed] [Google Scholar]
- De Deken R. H. The Crabtree effect: a regulatory system in yeast. J Gen Microbiol. 1966 Aug;44(2):149–156. doi: 10.1099/00221287-44-2-149. [DOI] [PubMed] [Google Scholar]
- Fiechter A., Fuhrmann G. F., Käppeli O. Regulation of glucose metabolism in growing yeast cells. Adv Microb Physiol. 1981;22:123–183. doi: 10.1016/s0065-2911(08)60327-6. [DOI] [PubMed] [Google Scholar]
- Frenkel E. P., Kitchens R. L. Acetyl-CoA synthetase from bakers' yeast (Saccharomyces cerevisiae). Methods Enzymol. 1981;71(Pt 100):317–324. doi: 10.1016/0076-6879(81)71040-1. [DOI] [PubMed] [Google Scholar]
- Gancedo J. M., Gancedo C. Concentrations of intermediary metabolites in yeast. Biochimie. 1973;55(2):205–211. doi: 10.1016/s0300-9084(73)80393-1. [DOI] [PubMed] [Google Scholar]
- Gumaa K. A., Greenbaum A. L., McLean P. Adaptive changes in satellite systems related to lipogenesis in rat and sheep mammary gland and in adipose tissue. Eur J Biochem. 1973 Apr 2;34(1):188–198. doi: 10.1111/j.1432-1033.1973.tb02745.x. [DOI] [PubMed] [Google Scholar]
- HOLZER H., GOEDDE H. W. Zwei Wege von Pyruvat zu Acetyl-Coenzym A in Hefe. Biochem Z. 1957;329(3):175–191. [PubMed] [Google Scholar]
- HOLZER H. Regulation of carbohydrate metabolism by enzyme competition. Cold Spring Harb Symp Quant Biol. 1961;26:277–288. doi: 10.1101/sqb.1961.026.01.034. [DOI] [PubMed] [Google Scholar]
- Harder W., Visser K., Kuenen J. G. Laboratory fermenter with an improved magnetic drive. Lab Pract. 1974 Nov;23(11):644–645. [PubMed] [Google Scholar]
- Hübner G., Weidhase R., Schellenberger A. The mechanism of substrate activation of pyruvate decarboxylase: a first approach. Eur J Biochem. 1978 Dec 1;92(1):175–181. doi: 10.1111/j.1432-1033.1978.tb12735.x. [DOI] [PubMed] [Google Scholar]
- Jacobson M. K., Bernofsky C. Mitochondrial acetaldehyde dehydrogenase from Saccharomyces cerevisiae. Biochim Biophys Acta. 1974 Jun 18;350(2):277–291. doi: 10.1016/0005-2744(74)90502-6. [DOI] [PubMed] [Google Scholar]
- Kaspar von Meyenburg H. Energetics of the budding cycle of Saccharomyces cerevisiae during glucose limited aerobic growth. Arch Mikrobiol. 1969;66(4):289–303. doi: 10.1007/BF00414585. [DOI] [PubMed] [Google Scholar]
- Klein H. P., Jahnke L. Variations in the localization of acetyl-coenzyme A synthetase in aerobic yeast cells. J Bacteriol. 1971 May;106(2):596–602. doi: 10.1128/jb.106.2.596-602.1971. [DOI] [PMC free article] [PubMed] [Google Scholar]
- Kresze G. B., Ronft H. Pyruvate dehydrogenase complex from baker's yeast. 1. Purification and some kinetic and regulatory properties. Eur J Biochem. 1981 Oct;119(3):573–579. doi: 10.1111/j.1432-1033.1981.tb05646.x. [DOI] [PubMed] [Google Scholar]
- Kuo D. J., Dikdan G., Jordan F. Resolution of brewers' yeast pyruvate decarboxylase into two isozymes. J Biol Chem. 1986 Mar 5;261(7):3316–3319. [PubMed] [Google Scholar]
- Käppeli O. Regulation of carbon metabolism in Saccharomyces cerevisiae and related yeasts. Adv Microb Physiol. 1986;28:181–209. doi: 10.1016/s0065-2911(08)60239-8. [DOI] [PubMed] [Google Scholar]
- LOWRY O. H., ROSEBROUGH N. J., FARR A. L., RANDALL R. J. Protein measurement with the Folin phenol reagent. J Biol Chem. 1951 Nov;193(1):265–275. [PubMed] [Google Scholar]
- LaNoue K. F., Schoolwerth A. C. Metabolite transport in mitochondria. Annu Rev Biochem. 1979;48:871–922. doi: 10.1146/annurev.bi.48.070179.004255. [DOI] [PubMed] [Google Scholar]
- Lagunas R., Gancedo J. M. Reduced pyridine-nucleotides balance in glucose-growing Saccharomyces cerevisiae. Eur J Biochem. 1973 Aug 1;37(1):90–94. doi: 10.1111/j.1432-1033.1973.tb02961.x. [DOI] [PubMed] [Google Scholar]
- Llorente N., de Castro I. N. Physiological role of yeasts NAD(P)+ and NADP+-linked aldehyde dehydrogenases. Rev Esp Fisiol. 1977 Jun;33(2):135–142. [PubMed] [Google Scholar]
- Lutstorf U., Megnet R. Multiple forms of alcohol dehydrogenase in Saccharomyces cerevisiae. I. Physiological control of ADH-2 and properties of ADH-2 and ADH-4. Arch Biochem Biophys. 1968 Sep 10;126(3):933–944. doi: 10.1016/0003-9861(68)90487-6. [DOI] [PubMed] [Google Scholar]
- Polakis E. S., Bartley W., Meek G. A. Changes in the activities of respiratory enzymes during the aerobic growth of yeast on different carbon sources. Biochem J. 1965 Oct;97(1):298–302. doi: 10.1042/bj0970298. [DOI] [PMC free article] [PubMed] [Google Scholar]
- Ryan E. D., Kohlhaw G. B. Subcellular localization of isoleucine-valine biosynthetic enzymes in yeast. J Bacteriol. 1974 Nov;120(2):631–637. doi: 10.1128/jb.120.2.631-637.1974. [DOI] [PMC free article] [PubMed] [Google Scholar]
- Sáez M. J., Lagunas R. Determination of intermediary metabolites in yeast. Critical examination of the effect of sampling conditions and recommendations for obtaining true levels. Mol Cell Biochem. 1976 Nov 30;13(2):73–78. doi: 10.1007/BF01837056. [DOI] [PubMed] [Google Scholar]
- TREVELYAN W. E., HARRISON J. S. Studies on yeast metabolism. III. The intracellular level of pyruvate during yeast fermentation. Biochem J. 1954 Aug;57(4):556–561. doi: 10.1042/bj0570556. [DOI] [PMC free article] [PubMed] [Google Scholar]
- Valle E., Bergillos L., Gascón S., Parra F., Ramos S. Trehalase activation in yeasts is mediated by an internal acidification. Eur J Biochem. 1986 Jan 15;154(2):247–251. doi: 10.1111/j.1432-1033.1986.tb09389.x. [DOI] [PubMed] [Google Scholar]
- Witt I., Kronau R., Holzer H. Repression von Alkoholdehydrogenase, Malatdehydrogenase, Isocitratlyase und Malatsynthase in Hefe durch Glucose. Biochim Biophys Acta. 1966 Jun 15;118(3):522–537. [PubMed] [Google Scholar]
- de la Peña P., Barros F., Gascón S., Ramos S., Lazo P. S. The electrochemical proton gradient of Saccharomyces. The role of potassium. Eur J Biochem. 1982 Apr 1;123(2):447–453. doi: 10.1111/j.1432-1033.1982.tb19788.x. [DOI] [PubMed] [Google Scholar]
- den Hollander J. A., Ugurbil K., Brown T. R., Shulman R. G. Phosphorus-31 nuclear magnetic resonance studies of the effect of oxygen upon glycolysis in yeast. Biochemistry. 1981 Sep 29;20(20):5871–5880. doi: 10.1021/bi00523a034. [DOI] [PubMed] [Google Scholar]
- von Jagow G., Klingenberg M. Pathways of hydrogen in mitochondria of Saccharomyces carlsbergensis. Eur J Biochem. 1970 Feb;12(3):583–592. doi: 10.1111/j.1432-1033.1970.tb00890.x. [DOI] [PubMed] [Google Scholar]