Abstract
The natural abundance of stable carbon isotopes measured in bacterial nucleic acids extracted from estuarine bacterial concentrates was used to trace sources of organic matter for bacteria in aquatic environments. The stable carbon isotope ratios of Pseudomonas aeruginosa and nucleic acids extracted from cultures resembled those of the carbon source on which bacteria were grown. The carbon isotope discrimination between the substrate and total cell carbon from bacterial cultures averaged 2.3% +/- 0.6% (n = 13). Furthermore, the isotope discrimination between the substrate and nucleic acids extracted from bacterial cultures was 2.4% +/- 0.4% (n = 10), not significantly different from the discrimination between bacteria and the substrate. Estuarine water samples were prefiltered through 1-micron-pore-size cartridge filters. Bacterium-sized particles in the filtrates were concentrated with tangential-flow filtration and centrifugation, and nucleic acids were then extracted from these concentrates. Hybridization with 16S rRNA probes showed that approximately 90% of the nucleic acids extracted on two sample dates were of eubacterial origin. Bacteria and nucleic acids from incubation experiments using estuarine water samples enriched with dissolved organic matter from Spartina alterniflora and Cyclotella caspia had stable carbon isotope values similar to those of the substrate sources. In a survey that compared diverse estuarine environments, stable carbon isotopes of bacteria grown in incubation experiments ranged from -31.9 to -20.5%. The range in isotope values of nucleic acids extracted from indigenous bacteria from the same waters was similar, -27.9 to -20.2%. Generally, the lack of isotope discrimination between bacteria and nucleic acids that was noted in the laboratory was observed in the field.(ABSTRACT TRUNCATED AT 250 WORDS)
Full text
PDF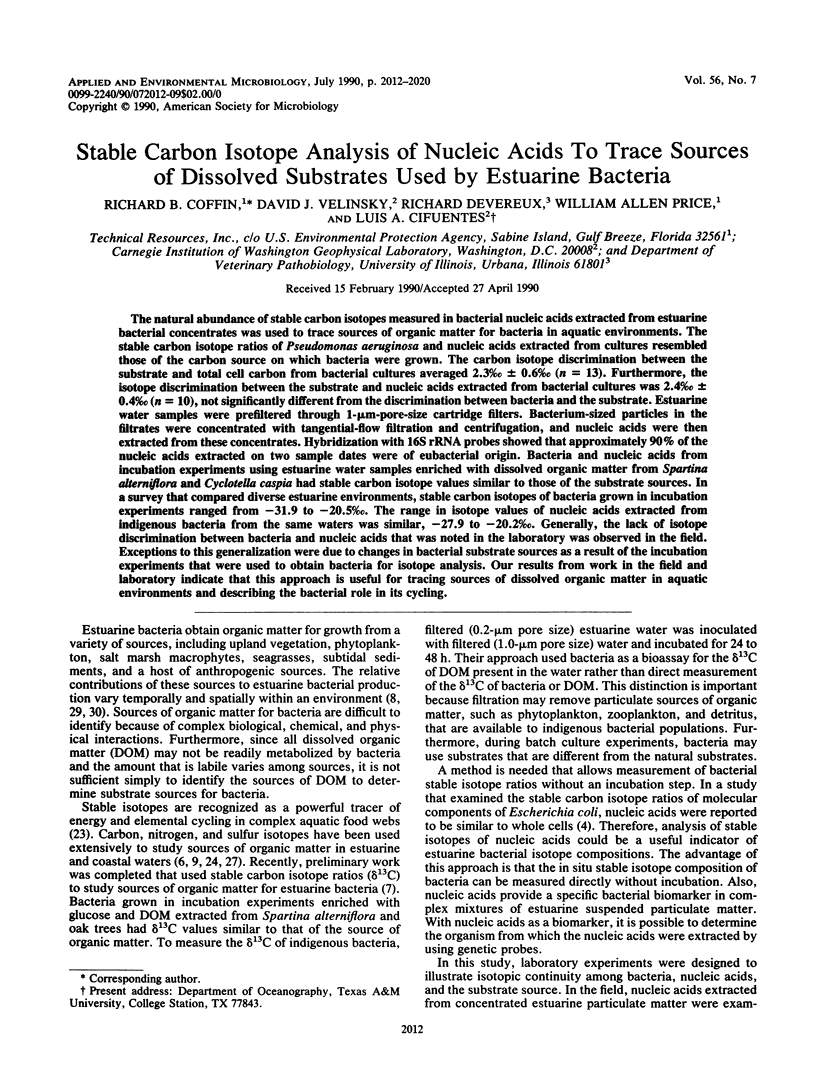
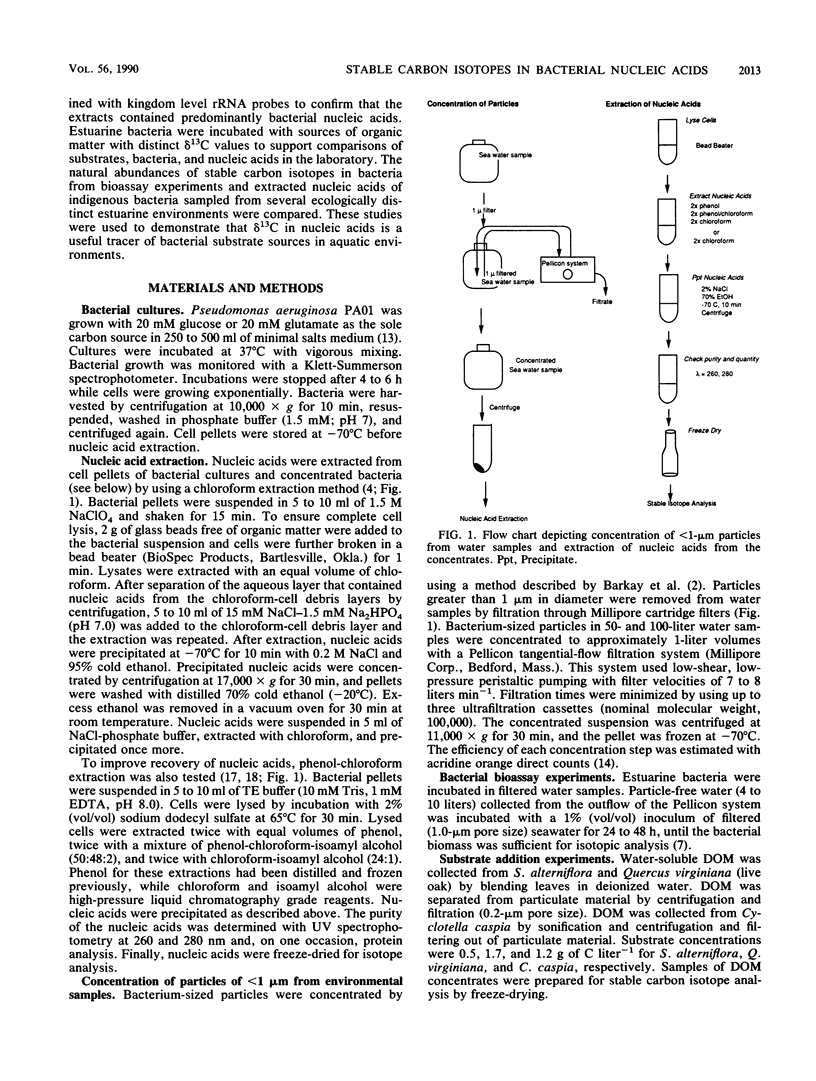
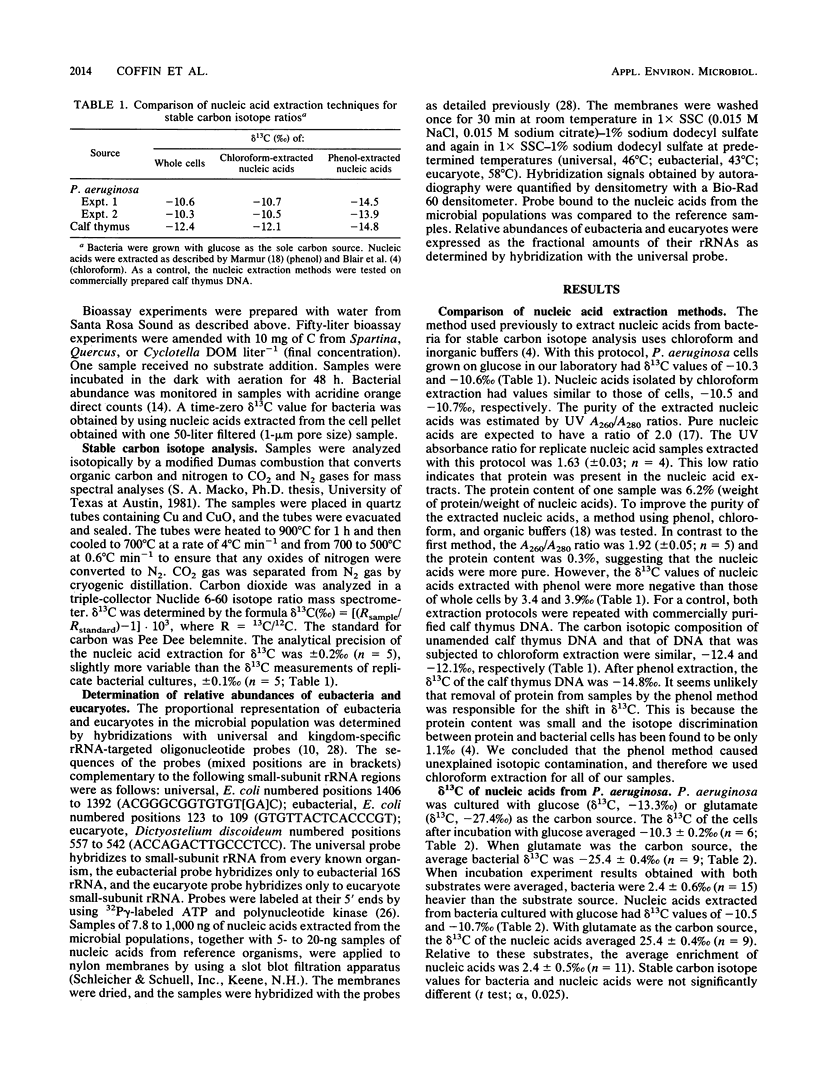
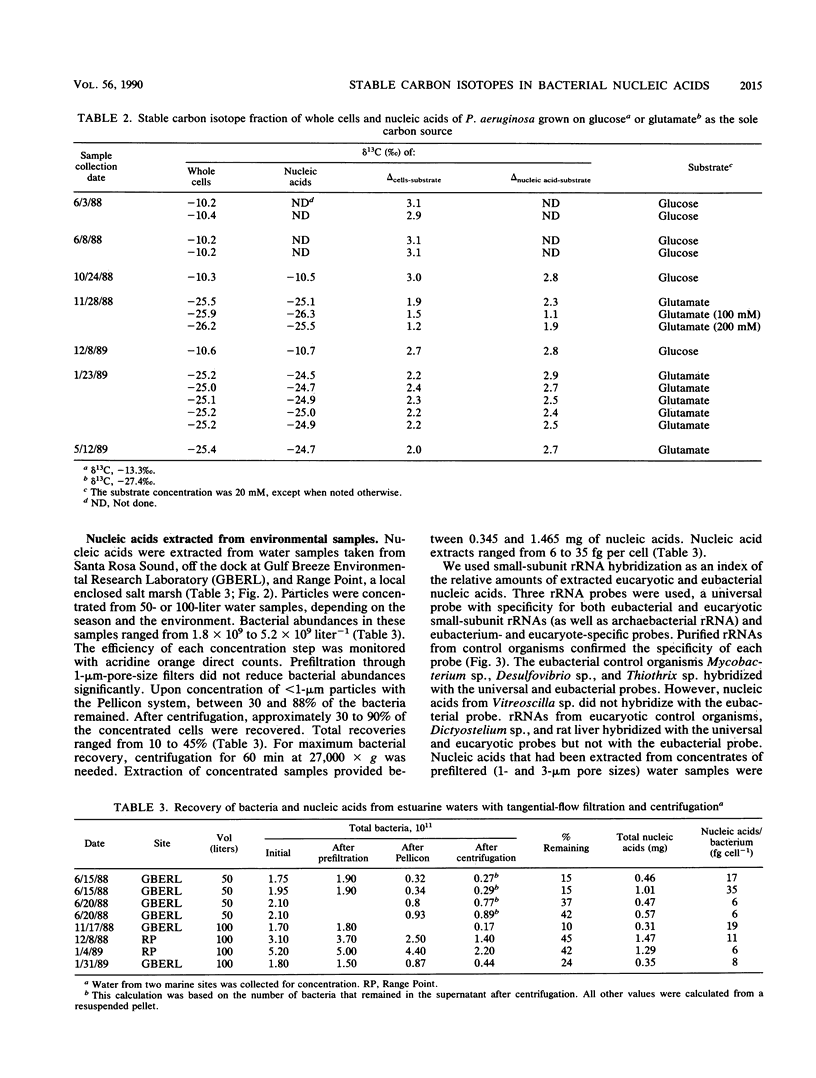
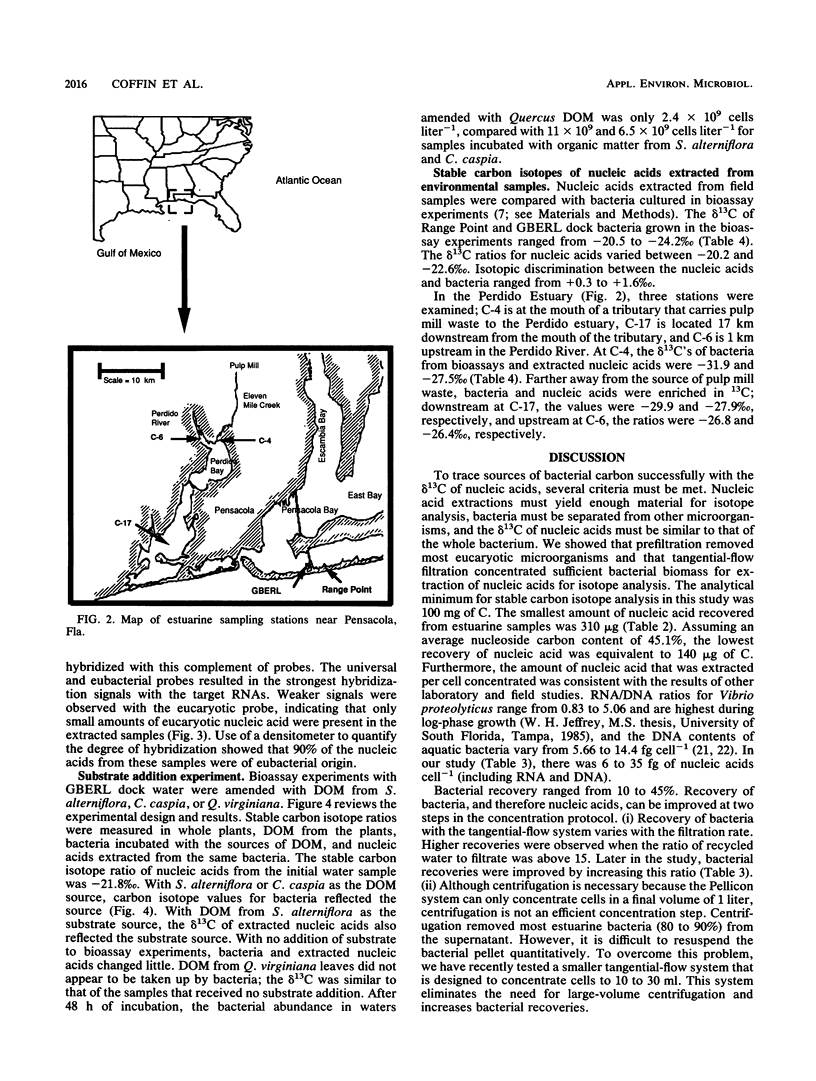
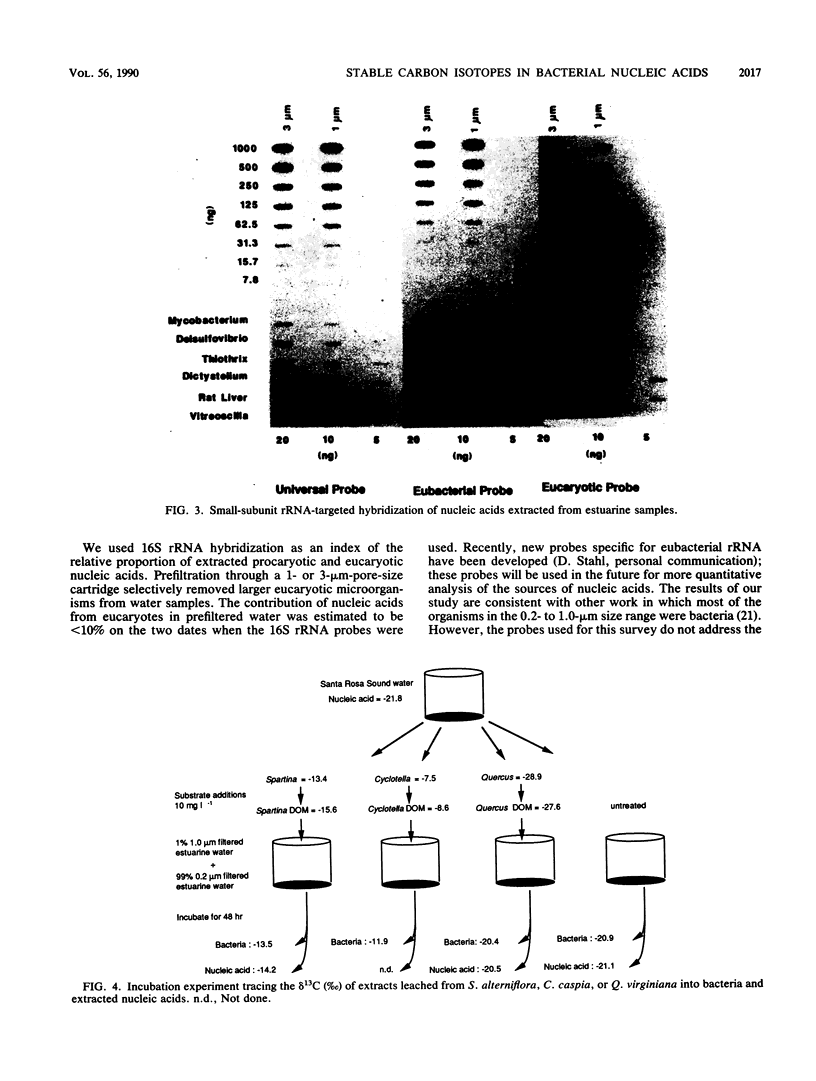
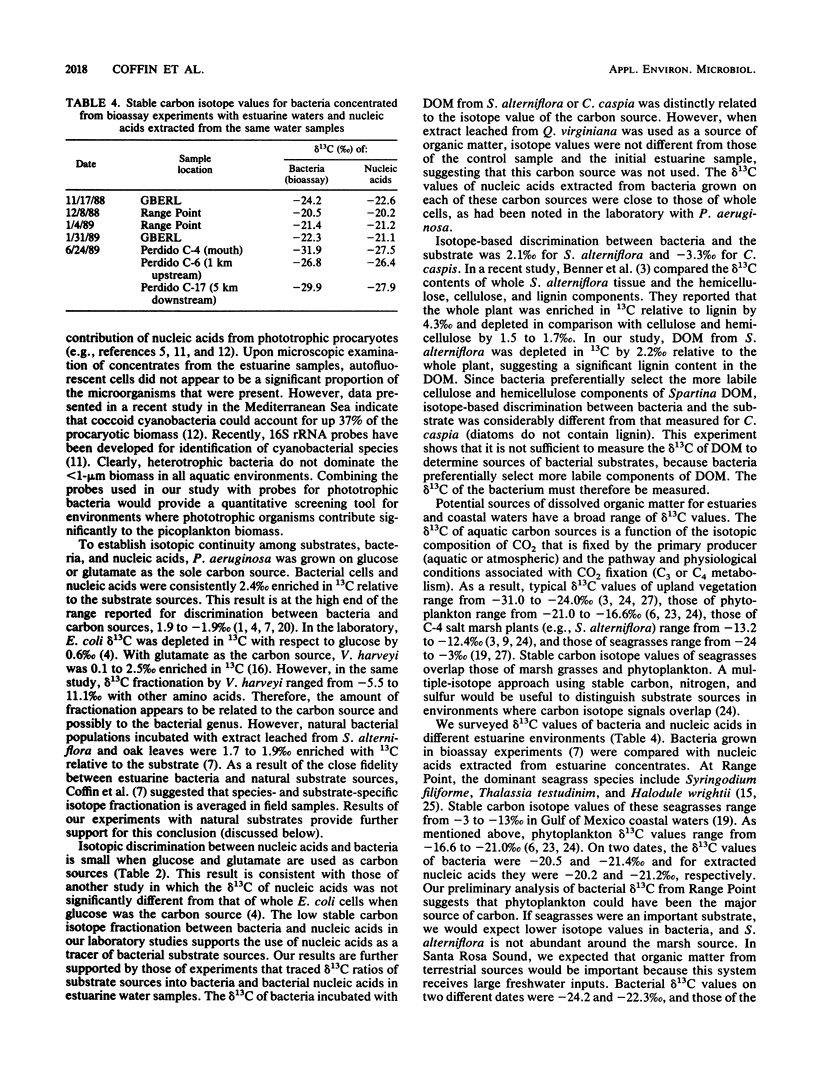
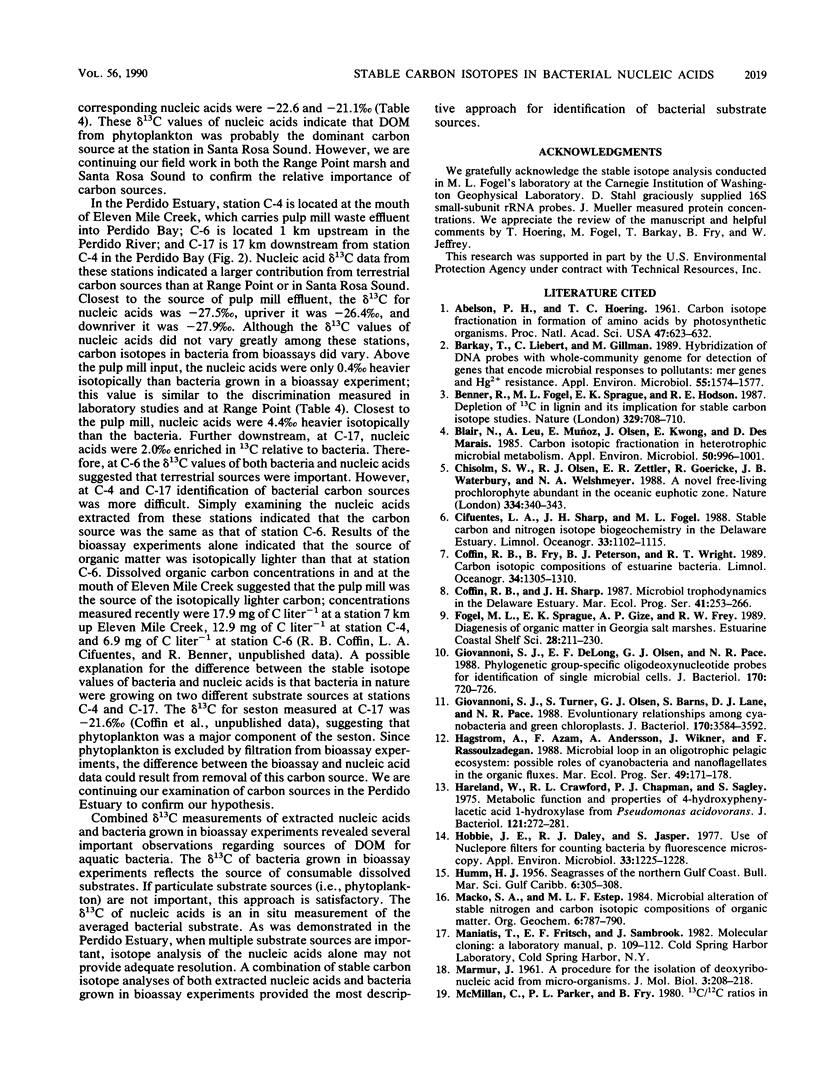
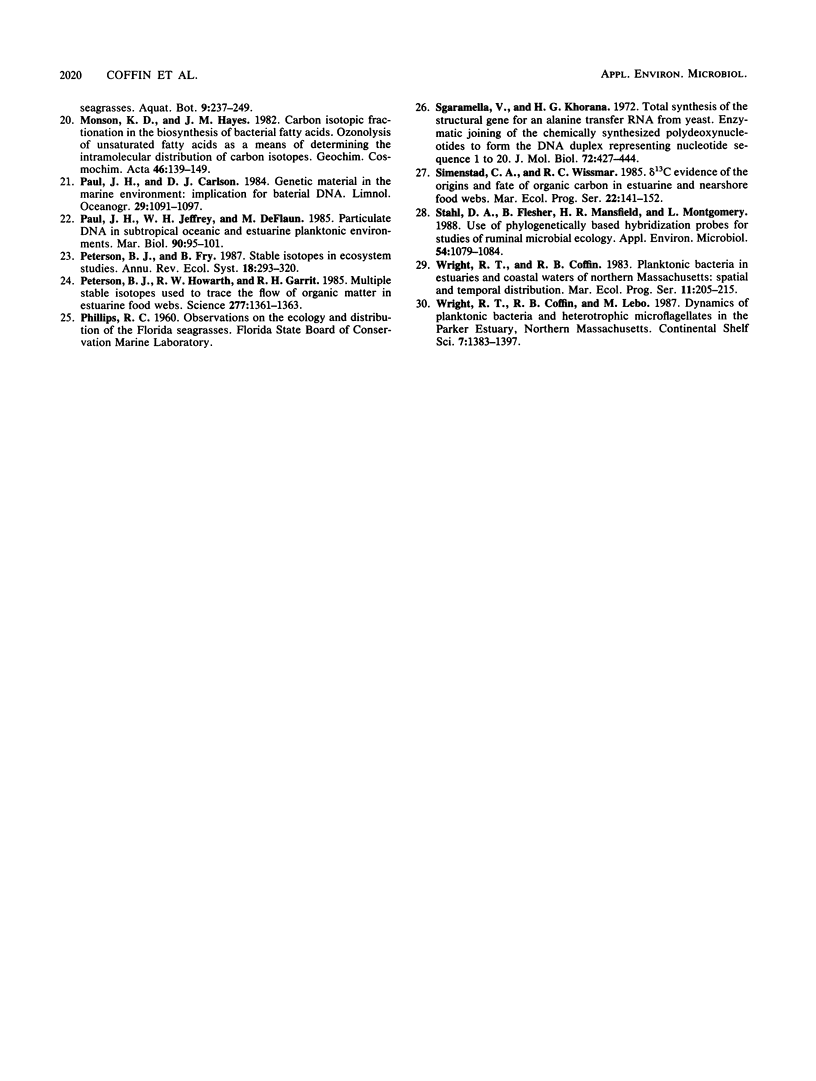
Images in this article
Selected References
These references are in PubMed. This may not be the complete list of references from this article.
- ABELSON P. H., HOERING T. C. Carbon isotope fractionation in formation of amino acids by photosynthetic organisms. Proc Natl Acad Sci U S A. 1961 May 15;47:623–632. doi: 10.1073/pnas.47.5.623. [DOI] [PMC free article] [PubMed] [Google Scholar]
- Barkay T., Liebert C., Gillman M. Hybridization of DNA probes with whole-community genome for detection of genes that encode microbial responses to pollutants: mer genes and Hg2+ resistance. Appl Environ Microbiol. 1989 Jun;55(6):1574–1577. doi: 10.1128/aem.55.6.1574-1577.1989. [DOI] [PMC free article] [PubMed] [Google Scholar]
- Blair N., Leu A., Muñoz E., Olsen J., Kwong E., Des Marais D. Carbon isotopic fractionation in heterotrophic microbial metabolism. Appl Environ Microbiol. 1985 Oct;50(4):996–1001. doi: 10.1128/aem.50.4.996-1001.1985. [DOI] [PMC free article] [PubMed] [Google Scholar]
- Giovannoni S. J., DeLong E. F., Olsen G. J., Pace N. R. Phylogenetic group-specific oligodeoxynucleotide probes for identification of single microbial cells. J Bacteriol. 1988 Feb;170(2):720–726. doi: 10.1128/jb.170.2.720-726.1988. [DOI] [PMC free article] [PubMed] [Google Scholar]
- Giovannoni S. J., Turner S., Olsen G. J., Barns S., Lane D. J., Pace N. R. Evolutionary relationships among cyanobacteria and green chloroplasts. J Bacteriol. 1988 Aug;170(8):3584–3592. doi: 10.1128/jb.170.8.3584-3592.1988. [DOI] [PMC free article] [PubMed] [Google Scholar]
- Hareland W. A., Crawford R. L., Chapman P. J., Dagley S. Metabolic function and properties of 4-hydroxyphenylacetic acid 1-hydroxylase from Pseudomonas acidovorans. J Bacteriol. 1975 Jan;121(1):272–285. doi: 10.1128/jb.121.1.272-285.1975. [DOI] [PMC free article] [PubMed] [Google Scholar]
- Hobbie J. E., Daley R. J., Jasper S. Use of nuclepore filters for counting bacteria by fluorescence microscopy. Appl Environ Microbiol. 1977 May;33(5):1225–1228. doi: 10.1128/aem.33.5.1225-1228.1977. [DOI] [PMC free article] [PubMed] [Google Scholar]
- Peterson B. J., Howarth R. W., Garritt R. H. Multiple stable isotopes used to trace the flow of organic matter in estuarine food webs. Science. 1985 Mar 15;227(4692):1361–1363. doi: 10.1126/science.227.4692.1361. [DOI] [PubMed] [Google Scholar]
- Sgaramella V., Khorana H. G. CXII. Total synthesis of the structural gene for an alanine transfer RNA from yeast. Enzymic joining of the chemically synthesized polydeoxynucleotides to form the DNA duplex representing nucleotide sequence 1 to 20. J Mol Biol. 1972 Dec 28;72(2):427–444. doi: 10.1016/0022-2836(72)90155-6. [DOI] [PubMed] [Google Scholar]
- Stahl D. A., Flesher B., Mansfield H. R., Montgomery L. Use of phylogenetically based hybridization probes for studies of ruminal microbial ecology. Appl Environ Microbiol. 1988 May;54(5):1079–1084. doi: 10.1128/aem.54.5.1079-1084.1988. [DOI] [PMC free article] [PubMed] [Google Scholar]