Abstract
It is generally accepted that the host microenvironment influences tumor biology. There are discrepancies in growth rate, metastatic potential, and efficacy of systemic treatment between ectopic and orthotopic tumors. Liver is the most common and critical site of distant metastasis of colorectal carcinoma. Tumorigenicity and efficacy of chemotherapeutic agents in colorectal tumors are different in liver and subcutaneous sites. Thus, we hypothesize that the liver (orthotopic) versus subcutaneous (ectopic) microenvironment would have different effects on the angiogenesis and maintenance of the microcirculation of colorectal tumor. To this end, we developed a new method to monitor and to quantify microcirculatory parameters in the tumor grown in the liver. Using this approach, we compared the microcirculation of LS174T, a human colon adenocarcinoma, metastasized to the liver with that of the host liver vessels and that of the same tumor grown in the subcutaneous space. In the liver metastasis model, 5 x 10(6) LS174T cells were injected into the spleen of nude mice. Four to eight weeks later, the liver with metastatic tumors was exteriorized and placed on a special stage and observed under an intravital fluorescence microscope. The dorsal skinfold chamber model was used to study the subcutaneous tumors. Red blood cell velocity, vessel diameter, density, permeability, and leukocyte-endothelial interactions were measured using fluorescence microscopy and image analysis. Vascular endothelial growth factor/ vascular permeability factor (VEGF/VPF) mRNA expression was determined by the Northern blot analysis. LS174T tumor foci in the liver had tortuous vascular architecture, heterogeneous blood flow, significantly lower vascular density, and significantly higher vascular permeability than normal liver tissue. Tumors grown in the liver had significantly lower vessel density, especially in the center coincident with central necrosis, than the subcutaneous tumors. The frequency distribution of vessel diameters of liver tumor was slightly shifted to smaller size compared with that of subcutaneous tumor. Leukocyte rolling in liver tumor was twofold lower than that in subcutaneous tumor. These physiological findings were consistent with the measurement of VEGF/VPF in that the VEGF/VPF mRNA level was lower in the liver tumor than that in the subcutaneous tumor. However, macromolecular vascular permeability in the liver tumor was significantly higher than in the subcutaneous tumor. Liver sinusoidal endothelial cells, the origin of liver tumor vessel endothelium, are known to be fenestrated and not to have a basement membrane, suggesting that the difference in endothelial cell origin may explain the difference in tumor vascular permeability in two sites. These findings demonstrate that liver microenvironment has different effects on some aspects of the tumor angiogenesis and microcirculation compared with the subcutaneous tissues. The new model/method described in this paper has significant implications in two research areas: 1) the liver microenvironment and its effect on tumor pathophysiology in conjunction with cytokine/ growth factor regulation and 2) the delivery of drugs, cells, and genes to liver tumors.
Full text
PDF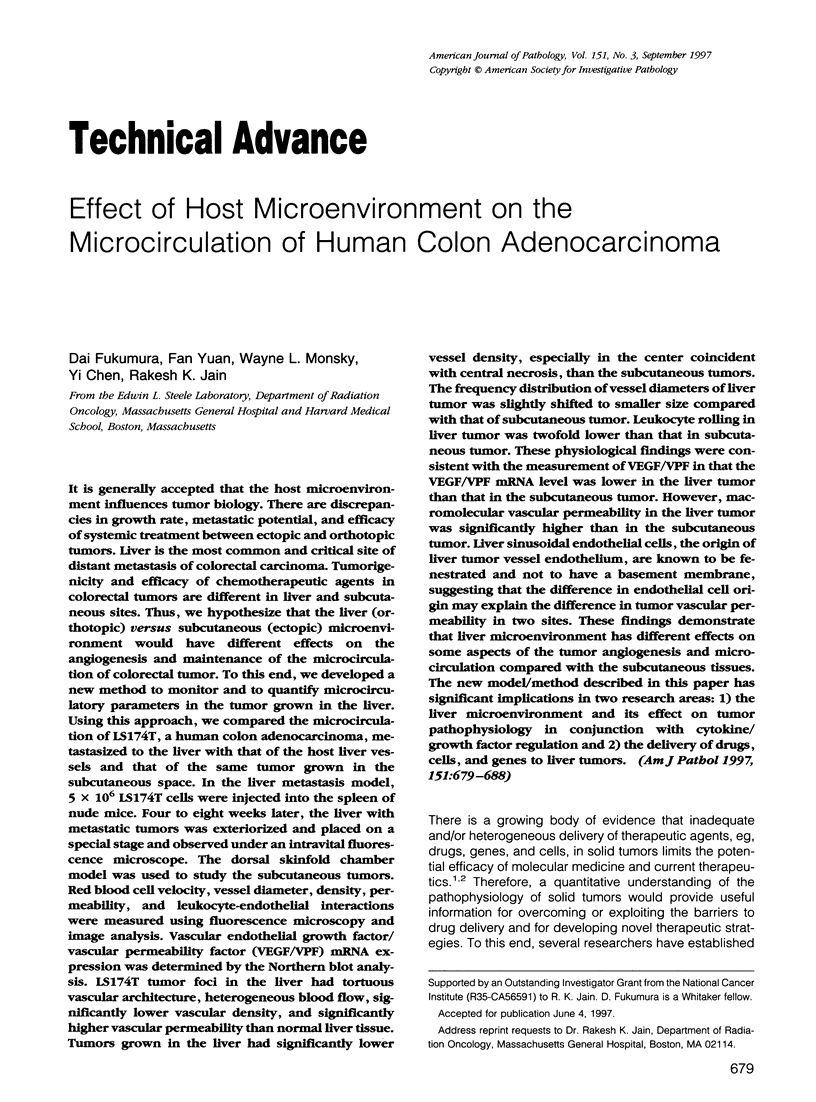
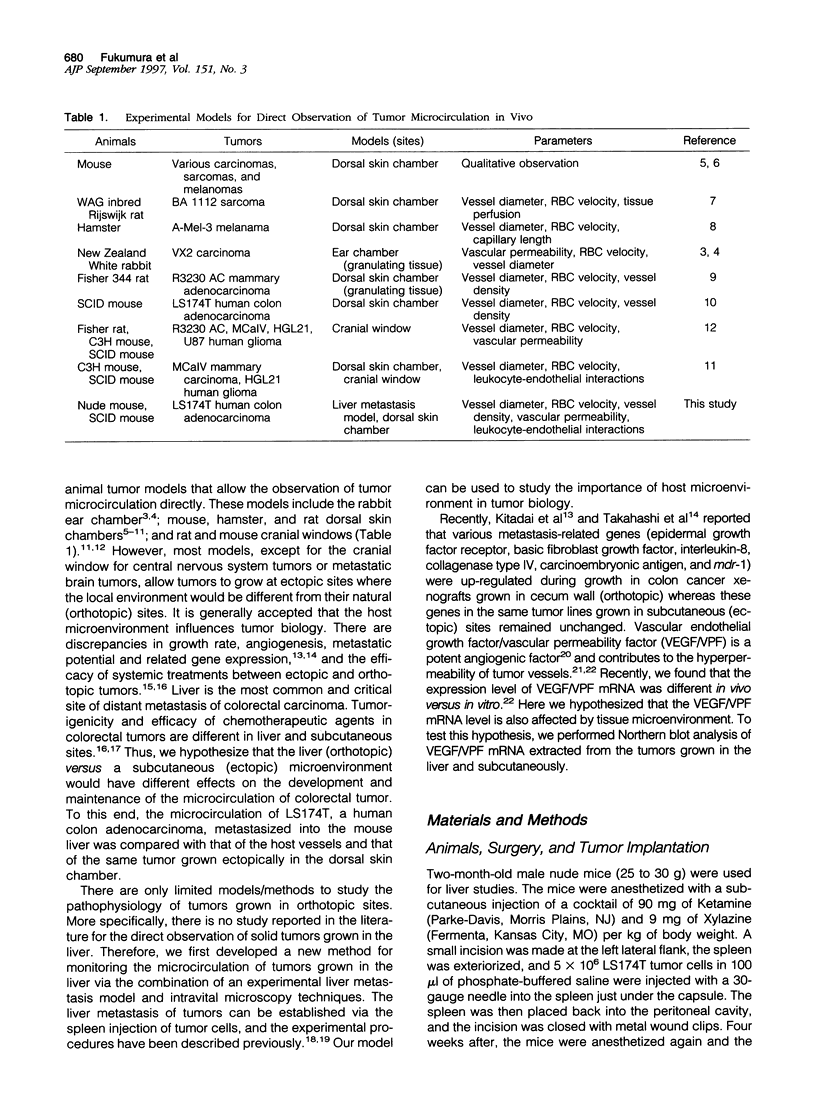
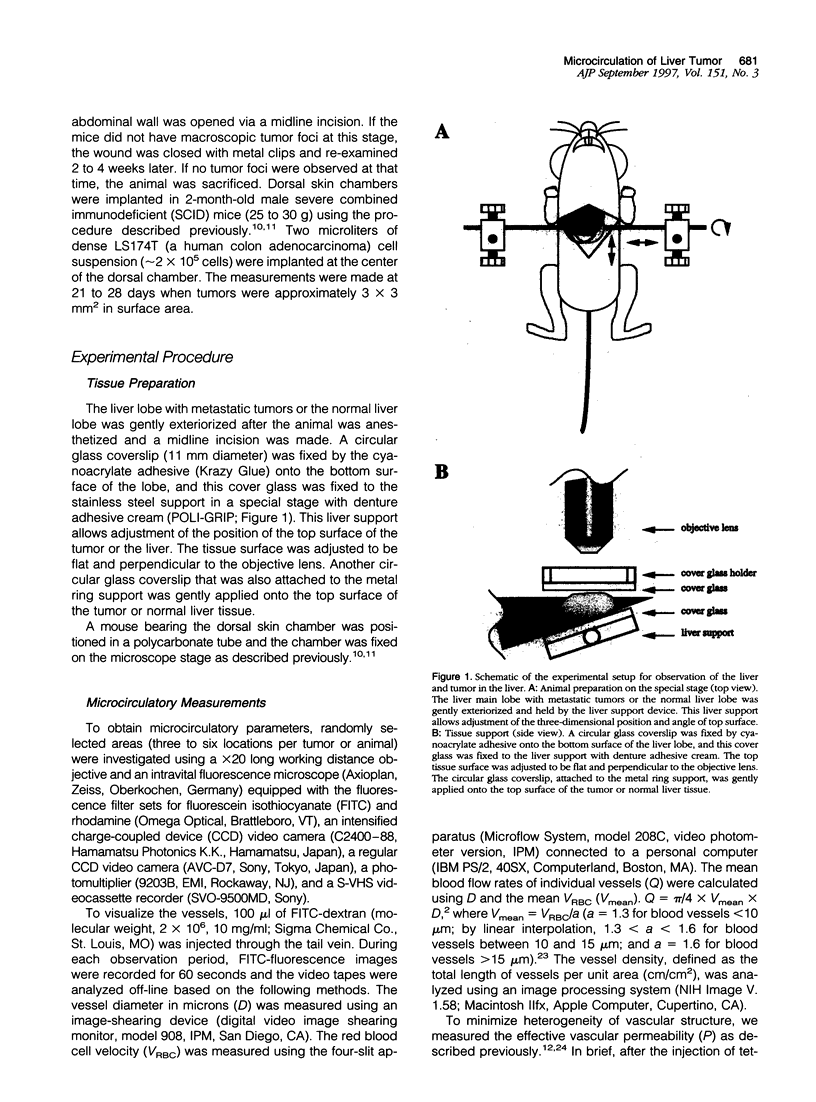
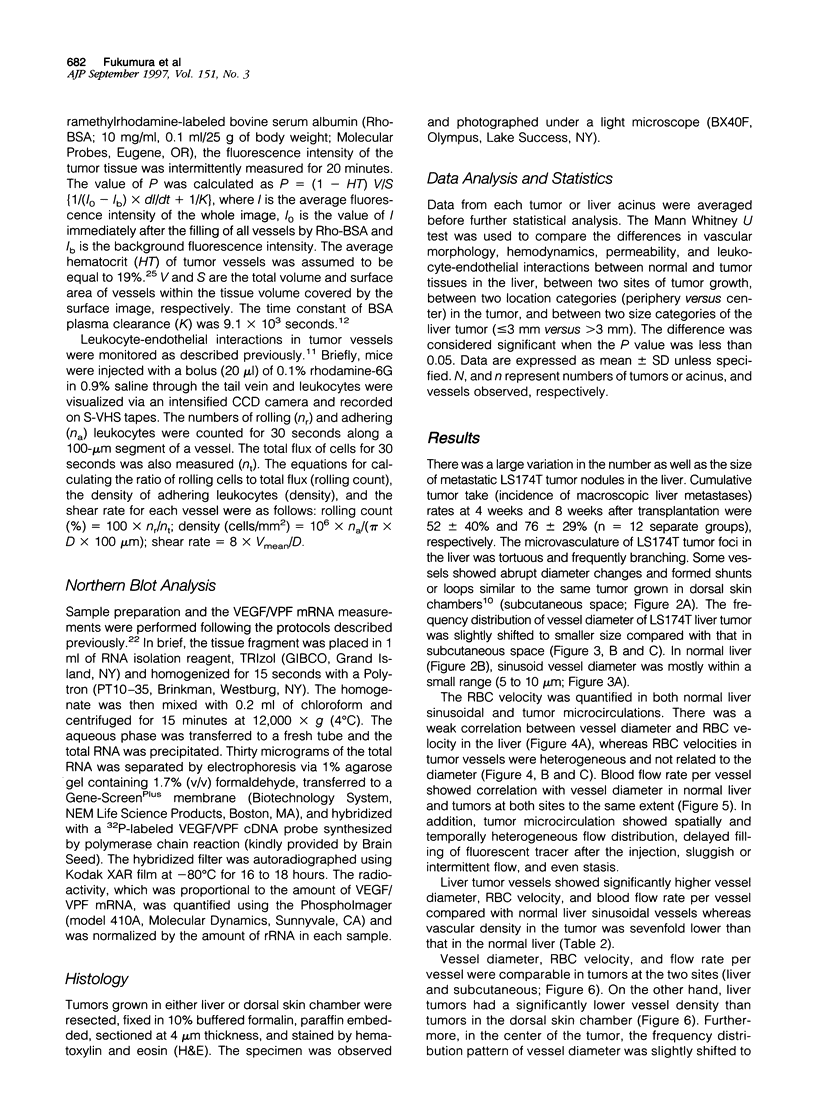
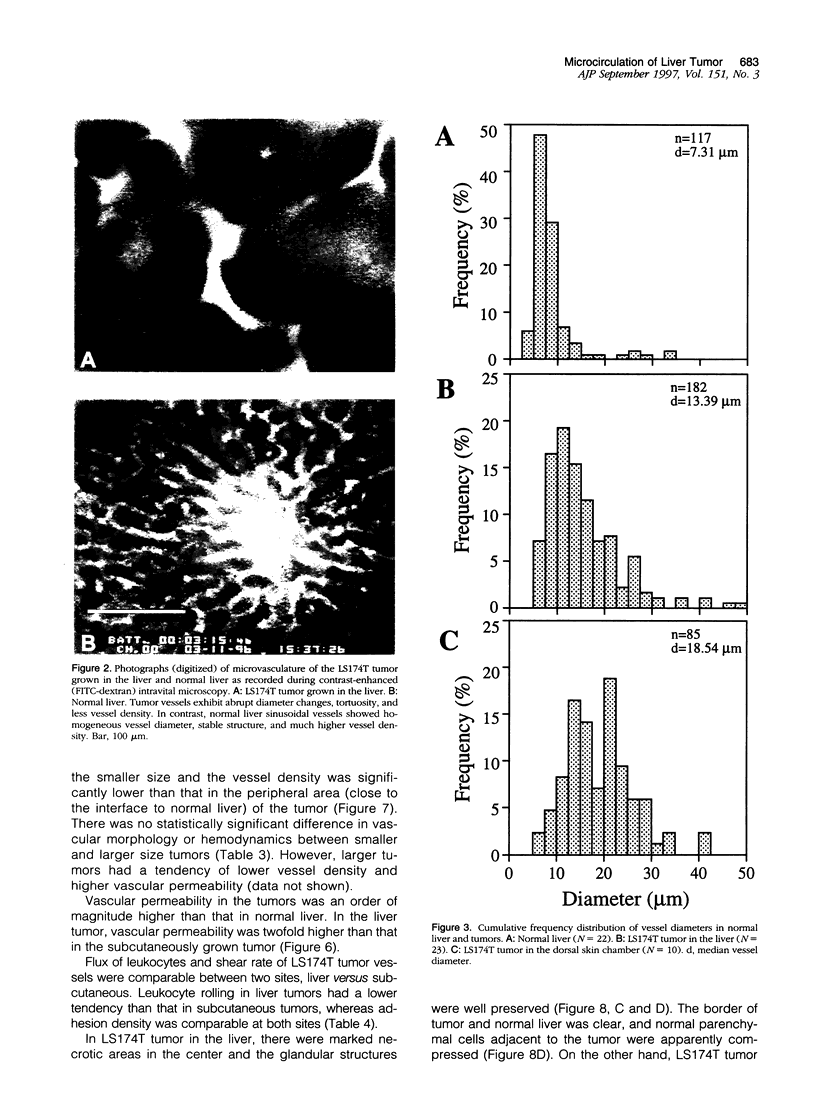
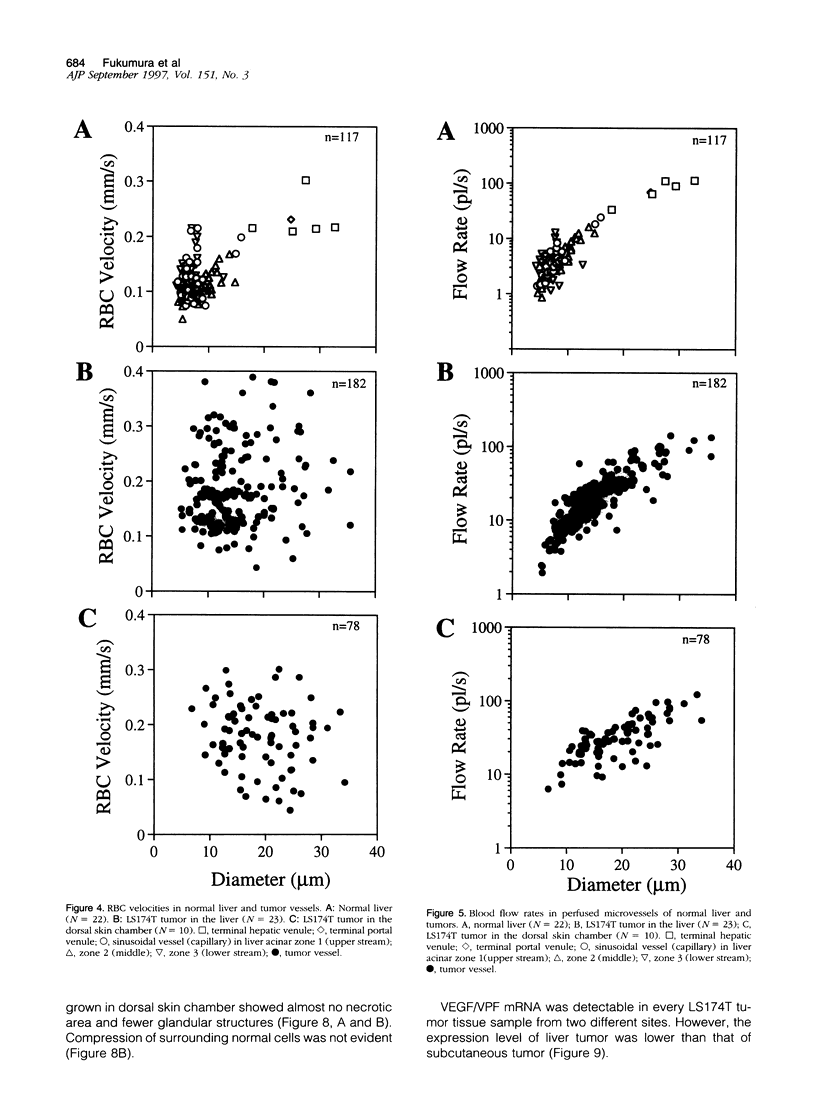
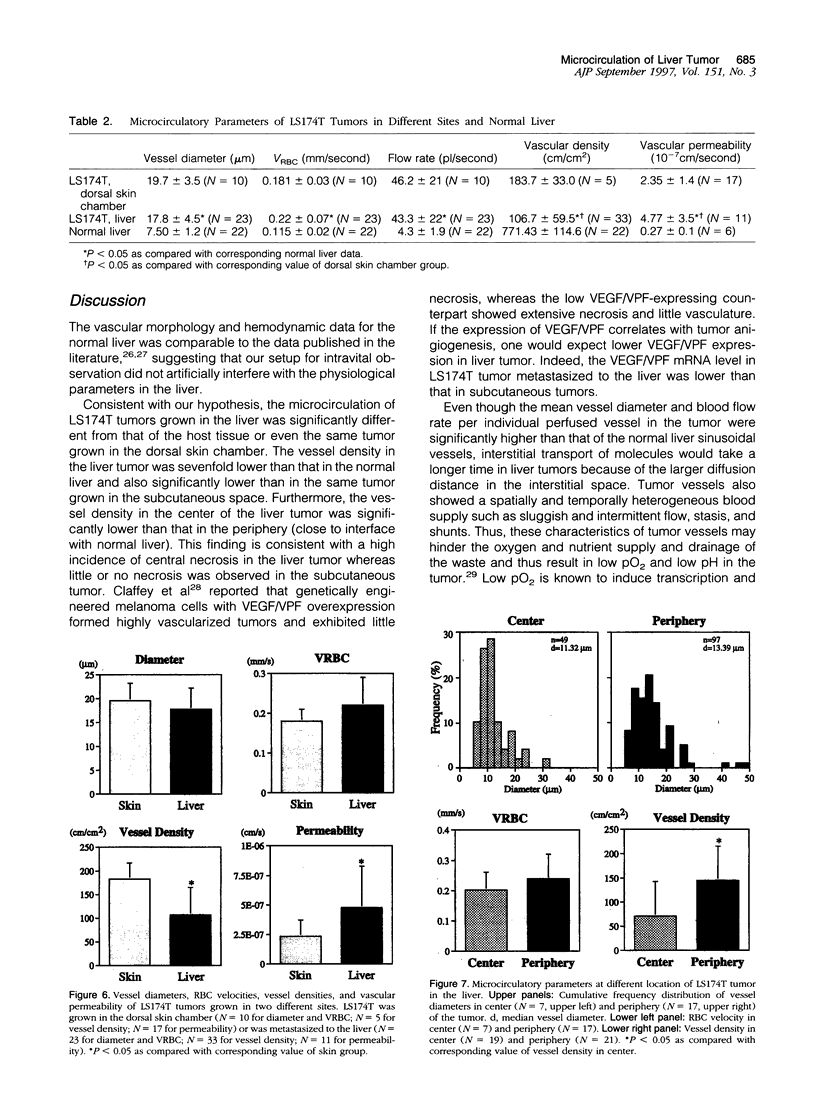
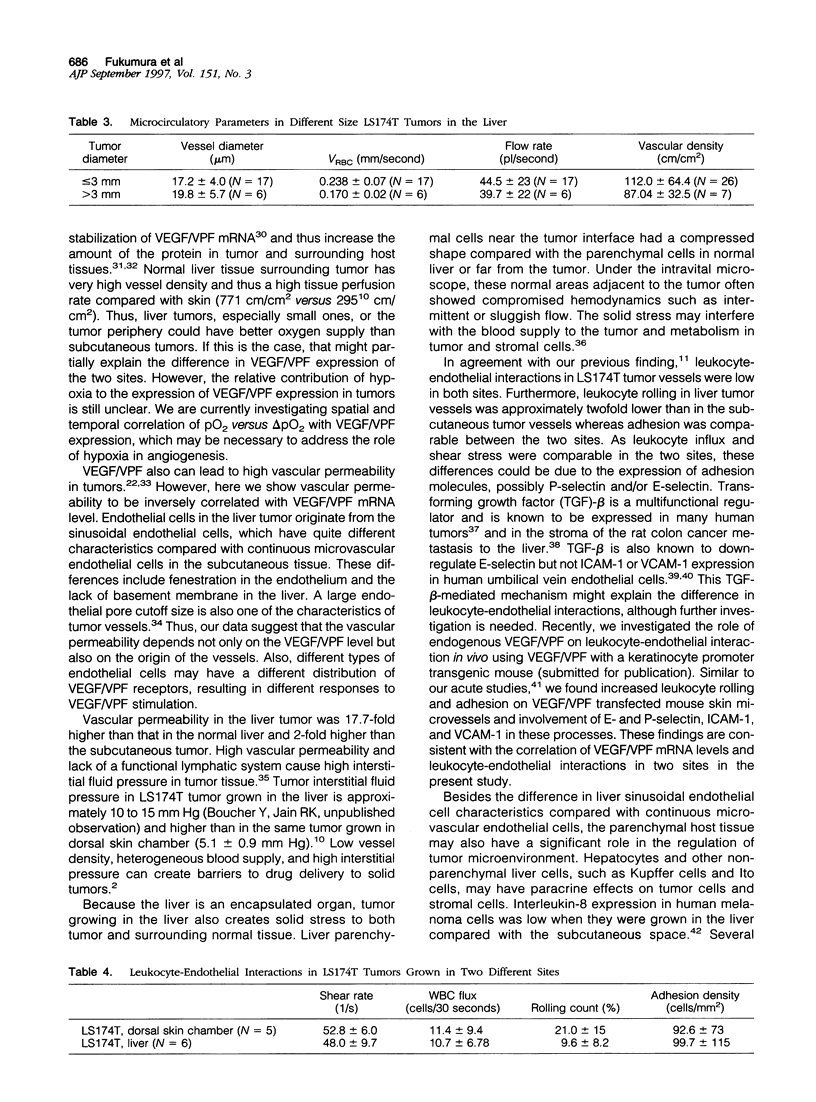
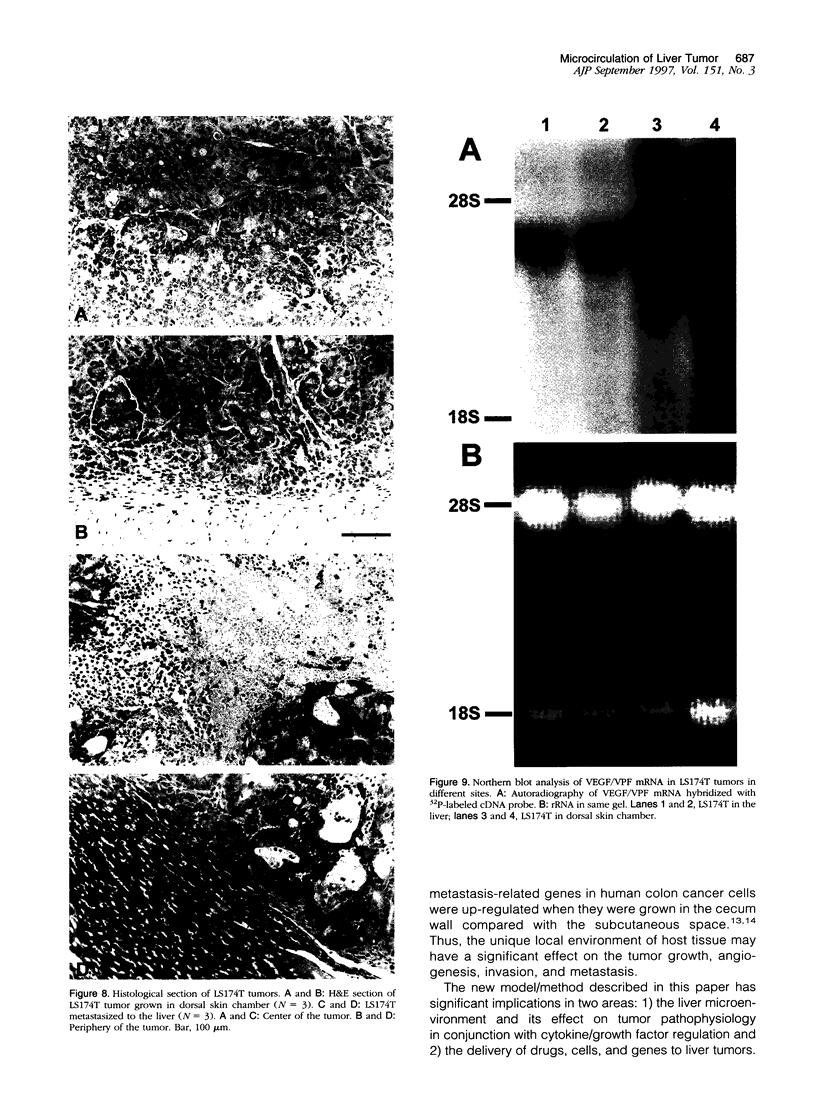
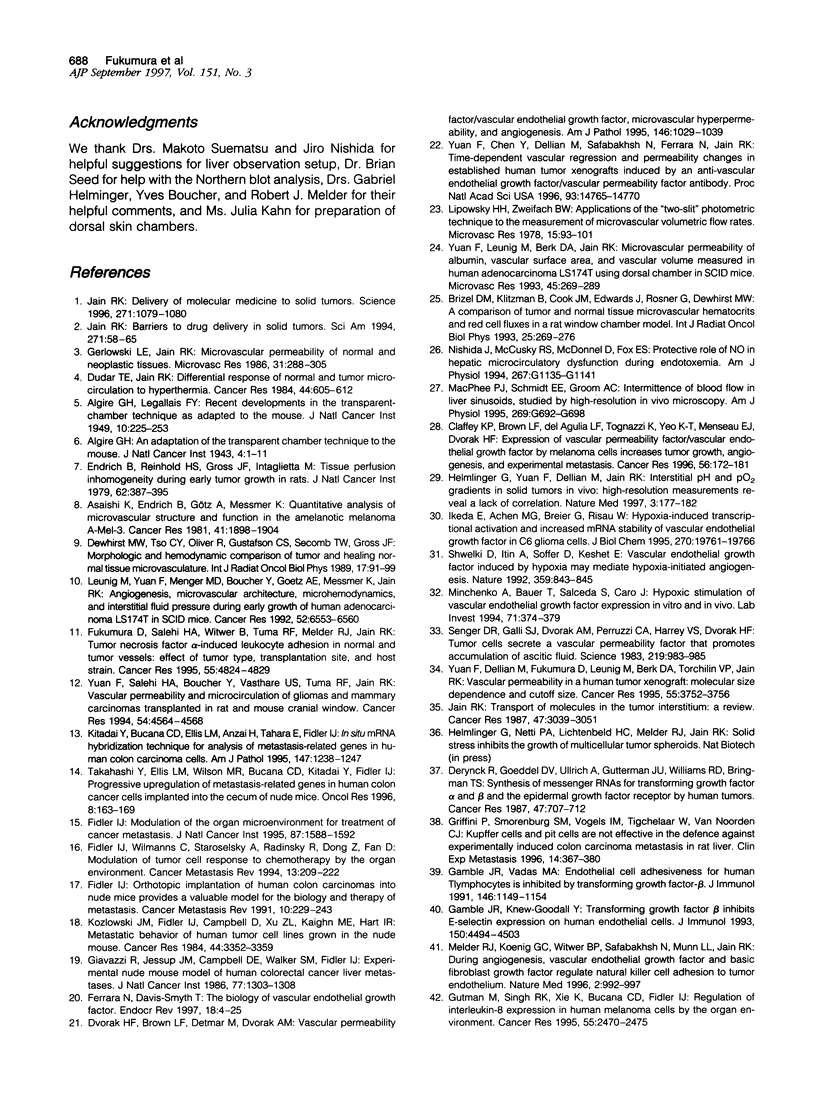
Images in this article
Selected References
These references are in PubMed. This may not be the complete list of references from this article.
- ALGIRE G. H., LEGALLAIS F. Y. Recent developments in the transparent-chamber technique as adapted to the mouse. J Natl Cancer Inst. 1949 Oct;10(2):225-53, incl 8 pl. [PubMed] [Google Scholar]
- Asaishi K., Endrich B., Götz A., Messmer K. Quantitative analysis of microvascular structure and function in the amelanotic melanoma A-Mel-3. Cancer Res. 1981 May;41(5):1898–1904. [PubMed] [Google Scholar]
- Brizel D. M., Klitzman B., Cook J. M., Edwards J., Rosner G., Dewhirst M. W. A comparison of tumor and normal tissue microvascular hematocrits and red cell fluxes in a rat window chamber model. Int J Radiat Oncol Biol Phys. 1993 Jan 15;25(2):269–276. doi: 10.1016/0360-3016(93)90348-y. [DOI] [PubMed] [Google Scholar]
- Claffey K. P., Brown L. F., del Aguila L. F., Tognazzi K., Yeo K. T., Manseau E. J., Dvorak H. F. Expression of vascular permeability factor/vascular endothelial growth factor by melanoma cells increases tumor growth, angiogenesis, and experimental metastasis. Cancer Res. 1996 Jan 1;56(1):172–181. [PubMed] [Google Scholar]
- Derynck R., Goeddel D. V., Ullrich A., Gutterman J. U., Williams R. D., Bringman T. S., Berger W. H. Synthesis of messenger RNAs for transforming growth factors alpha and beta and the epidermal growth factor receptor by human tumors. Cancer Res. 1987 Feb 1;47(3):707–712. [PubMed] [Google Scholar]
- Dewhirst M. W., Tso C. Y., Oliver R., Gustafson C. S., Secomb T. W., Gross J. F. Morphologic and hemodynamic comparison of tumor and healing normal tissue microvasculature. Int J Radiat Oncol Biol Phys. 1989 Jul;17(1):91–99. doi: 10.1016/0360-3016(89)90375-1. [DOI] [PubMed] [Google Scholar]
- Dudar T. E., Jain R. K. Differential response of normal and tumor microcirculation to hyperthermia. Cancer Res. 1984 Feb;44(2):605–612. [PubMed] [Google Scholar]
- Dvorak H. F., Brown L. F., Detmar M., Dvorak A. M. Vascular permeability factor/vascular endothelial growth factor, microvascular hyperpermeability, and angiogenesis. Am J Pathol. 1995 May;146(5):1029–1039. [PMC free article] [PubMed] [Google Scholar]
- Endrich B., Reinhold H. S., Gross J. F., Intaglietta M. Tissue perfusion inhomogeneity during early tumor growth in rats. J Natl Cancer Inst. 1979 Feb;62(2):387–395. [PubMed] [Google Scholar]
- Ferrara N., Davis-Smyth T. The biology of vascular endothelial growth factor. Endocr Rev. 1997 Feb;18(1):4–25. doi: 10.1210/edrv.18.1.0287. [DOI] [PubMed] [Google Scholar]
- Fidler I. J. Modulation of the organ microenvironment for treatment of cancer metastasis. J Natl Cancer Inst. 1995 Nov 1;87(21):1588–1592. doi: 10.1093/jnci/87.21.1588. [DOI] [PubMed] [Google Scholar]
- Fidler I. J. Orthotopic implantation of human colon carcinomas into nude mice provides a valuable model for the biology and therapy of metastasis. Cancer Metastasis Rev. 1991 Oct;10(3):229–243. doi: 10.1007/BF00050794. [DOI] [PubMed] [Google Scholar]
- Fidler I. J., Wilmanns C., Staroselsky A., Radinsky R., Dong Z., Fan D. Modulation of tumor cell response to chemotherapy by the organ environment. Cancer Metastasis Rev. 1994 Jun;13(2):209–222. doi: 10.1007/BF00689637. [DOI] [PubMed] [Google Scholar]
- Fukumura D., Salehi H. A., Witwer B., Tuma R. F., Melder R. J., Jain R. K. Tumor necrosis factor alpha-induced leukocyte adhesion in normal and tumor vessels: effect of tumor type, transplantation site, and host strain. Cancer Res. 1995 Nov 1;55(21):4824–4829. [PubMed] [Google Scholar]
- Gamble J. R., Khew-Goodall Y., Vadas M. A. Transforming growth factor-beta inhibits E-selectin expression on human endothelial cells. J Immunol. 1993 May 15;150(10):4494–4503. [PubMed] [Google Scholar]
- Gamble J. R., Vadas M. A. Endothelial cell adhesiveness for human T lymphocytes is inhibited by transforming growth factor-beta 1. J Immunol. 1991 Feb 15;146(4):1149–1154. [PubMed] [Google Scholar]
- Gerlowski L. E., Jain R. K. Microvascular permeability of normal and neoplastic tissues. Microvasc Res. 1986 May;31(3):288–305. doi: 10.1016/0026-2862(86)90018-x. [DOI] [PubMed] [Google Scholar]
- Giavazzi R., Jessup J. M., Campbell D. E., Walker S. M., Fidler I. J. Experimental nude mouse model of human colorectal cancer liver metastases. J Natl Cancer Inst. 1986 Dec;77(6):1303–1308. [PubMed] [Google Scholar]
- Griffini P., Smorenburg S. M., Vogels I. M., Tigchelaar W., Van Noorden C. J. Kupffer cells and pit cells are not effective in the defense against experimentally induced colon carcinoma metastasis in rat liver. Clin Exp Metastasis. 1996 Sep;14(4):367–380. doi: 10.1007/BF00123396. [DOI] [PubMed] [Google Scholar]
- Gutman M., Singh R. K., Xie K., Bucana C. D., Fidler I. J. Regulation of interleukin-8 expression in human melanoma cells by the organ environment. Cancer Res. 1995 Jun 1;55(11):2470–2475. [PubMed] [Google Scholar]
- Helmlinger G., Yuan F., Dellian M., Jain R. K. Interstitial pH and pO2 gradients in solid tumors in vivo: high-resolution measurements reveal a lack of correlation. Nat Med. 1997 Feb;3(2):177–182. doi: 10.1038/nm0297-177. [DOI] [PubMed] [Google Scholar]
- Ikeda E., Achen M. G., Breier G., Risau W. Hypoxia-induced transcriptional activation and increased mRNA stability of vascular endothelial growth factor in C6 glioma cells. J Biol Chem. 1995 Aug 25;270(34):19761–19766. doi: 10.1074/jbc.270.34.19761. [DOI] [PubMed] [Google Scholar]
- Jain R. K. Barriers to drug delivery in solid tumors. Sci Am. 1994 Jul;271(1):58–65. doi: 10.1038/scientificamerican0794-58. [DOI] [PubMed] [Google Scholar]
- Jain R. K. Delivery of molecular medicine to solid tumors. Science. 1996 Feb 23;271(5252):1079–1080. doi: 10.1126/science.271.5252.1079. [DOI] [PubMed] [Google Scholar]
- Jain R. K. Transport of molecules in the tumor interstitium: a review. Cancer Res. 1987 Jun 15;47(12):3039–3051. [PubMed] [Google Scholar]
- Kitadai Y., Bucana C. D., Ellis L. M., Anzai H., Tahara E., Fidler I. J. In situ mRNA hybridization technique for analysis of metastasis-related genes in human colon carcinoma cells. Am J Pathol. 1995 Nov;147(5):1238–1247. [PMC free article] [PubMed] [Google Scholar]
- Leunig M., Yuan F., Menger M. D., Boucher Y., Goetz A. E., Messmer K., Jain R. K. Angiogenesis, microvascular architecture, microhemodynamics, and interstitial fluid pressure during early growth of human adenocarcinoma LS174T in SCID mice. Cancer Res. 1992 Dec 1;52(23):6553–6560. [PubMed] [Google Scholar]
- Lipowsky H. H., Zweifach B. W. Application of the "two-slit" photometric technique to the measurement of microvascular volumetric flow rates. Microvasc Res. 1978 Jan;15(1):93–101. doi: 10.1016/0026-2862(78)90009-2. [DOI] [PubMed] [Google Scholar]
- MacPhee P. J., Schmidt E. E., Groom A. C. Intermittence of blood flow in liver sinusoids, studied by high-resolution in vivo microscopy. Am J Physiol. 1995 Nov;269(5 Pt 1):G692–G698. doi: 10.1152/ajpgi.1995.269.5.G692. [DOI] [PubMed] [Google Scholar]
- Melder R. J., Koenig G. C., Witwer B. P., Safabakhsh N., Munn L. L., Jain R. K. During angiogenesis, vascular endothelial growth factor and basic fibroblast growth factor regulate natural killer cell adhesion to tumor endothelium. Nat Med. 1996 Sep;2(9):992–997. doi: 10.1038/nm0996-992. [DOI] [PubMed] [Google Scholar]
- Minchenko A., Bauer T., Salceda S., Caro J. Hypoxic stimulation of vascular endothelial growth factor expression in vitro and in vivo. Lab Invest. 1994 Sep;71(3):374–379. [PubMed] [Google Scholar]
- Senger D. R., Galli S. J., Dvorak A. M., Perruzzi C. A., Harvey V. S., Dvorak H. F. Tumor cells secrete a vascular permeability factor that promotes accumulation of ascites fluid. Science. 1983 Feb 25;219(4587):983–985. doi: 10.1126/science.6823562. [DOI] [PubMed] [Google Scholar]
- Shweiki D., Itin A., Soffer D., Keshet E. Vascular endothelial growth factor induced by hypoxia may mediate hypoxia-initiated angiogenesis. Nature. 1992 Oct 29;359(6398):843–845. doi: 10.1038/359843a0. [DOI] [PubMed] [Google Scholar]
- Takahashi Y., Ellis L. M., Wilson M. R., Bucana C. D., Kitadai Y., Fidler I. J. Progressive upregulation of metastasis-related genes in human colon cancer cells implanted into the cecum of nude mice. Oncol Res. 1996;8(4):163–169. [PubMed] [Google Scholar]
- Yuan F., Chen Y., Dellian M., Safabakhsh N., Ferrara N., Jain R. K. Time-dependent vascular regression and permeability changes in established human tumor xenografts induced by an anti-vascular endothelial growth factor/vascular permeability factor antibody. Proc Natl Acad Sci U S A. 1996 Dec 10;93(25):14765–14770. doi: 10.1073/pnas.93.25.14765. [DOI] [PMC free article] [PubMed] [Google Scholar]
- Yuan F., Dellian M., Fukumura D., Leunig M., Berk D. A., Torchilin V. P., Jain R. K. Vascular permeability in a human tumor xenograft: molecular size dependence and cutoff size. Cancer Res. 1995 Sep 1;55(17):3752–3756. [PubMed] [Google Scholar]
- Yuan F., Leunig M., Berk D. A., Jain R. K. Microvascular permeability of albumin, vascular surface area, and vascular volume measured in human adenocarcinoma LS174T using dorsal chamber in SCID mice. Microvasc Res. 1993 May;45(3):269–289. doi: 10.1006/mvre.1993.1024. [DOI] [PubMed] [Google Scholar]
- Yuan F., Salehi H. A., Boucher Y., Vasthare U. S., Tuma R. F., Jain R. K. Vascular permeability and microcirculation of gliomas and mammary carcinomas transplanted in rat and mouse cranial windows. Cancer Res. 1994 Sep 1;54(17):4564–4568. [PubMed] [Google Scholar]