Abstract
1. Voltage-activated Ca2+ currents and caffeine (1 to 10 mM) were used to increase intracellular Ca2+ in rat cultured dorsal root ganglia (DRG) neurones. Elevation of intracellular Ca2+ resulted in activation of inward currents which were attenuated by increasing the Ca2+ buffering capacity of cells by raising the concentration of EGTA in the patch solution to 10 mM. Low and high voltage-activated Ca2+ currents gave rise to Cl- tail currents in cells loaded with CsCl patch solution. Outward Ca2+ channel currents activated at very depolarized potentials (Vc + 60 mV to + 100 mV) also activated Cl- tail currents, which were enhanced when extracellular Ca2+ was elevated from 2 mM to 4 mM. 2. The Ca(2+)-activated Cl- tail currents were identified by estimation of tail current reversal potential by use of a double pulse protocol and by sensitivity to the Cl- channel blocker 5-nitro 2-(3-phenyl-propylamino) benzoic acid (NPPB) applied at a concentration of 10 microM. 3. Cells loaded with Cs acetate patch solution and bathed in medium containing 4 mM Ca2+ also had prolonged Ca(2+)-dependent tail currents, however these smaller tail currents were insensitive to NPPB. Release of Ca2+ from intracellular stores by caffeine gave rise to sustained inward currents in cells loaded with Cs acetate. Both Ca(2+)-activated tail currents and caffeine-induced inward currents recorded from cells loaded with Cs acetate were attenuated by Tris based recording media, and had reversal potentials positive to 0 mV suggesting activity of Ca(2+)-activated cation channels.(ABSTRACT TRUNCATED AT 250 WORDS)
Full text
PDF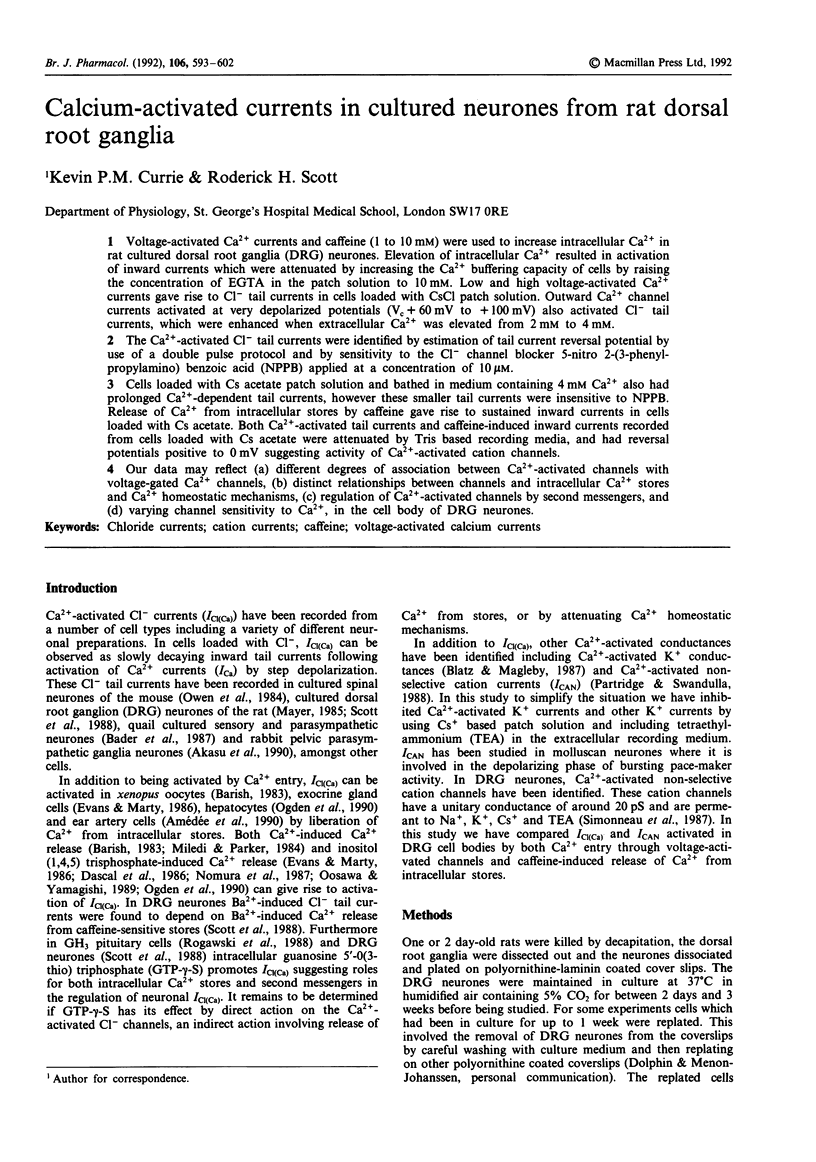
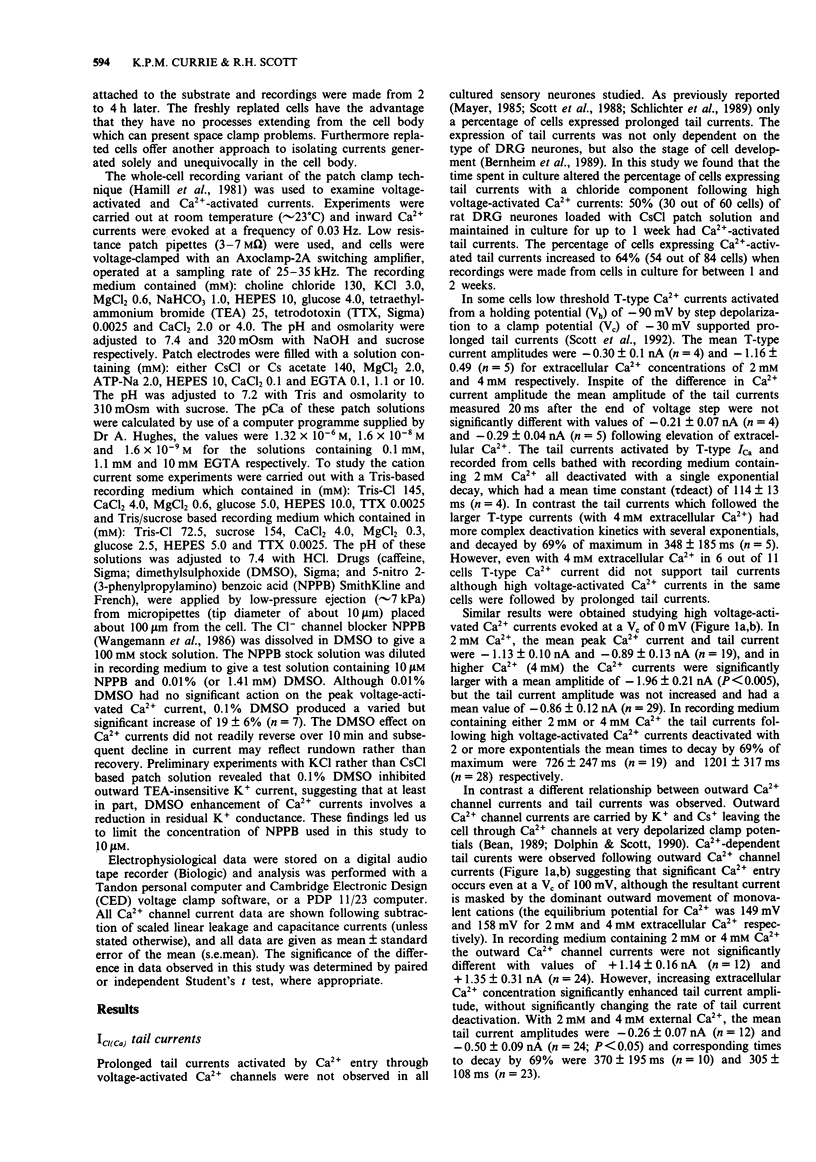
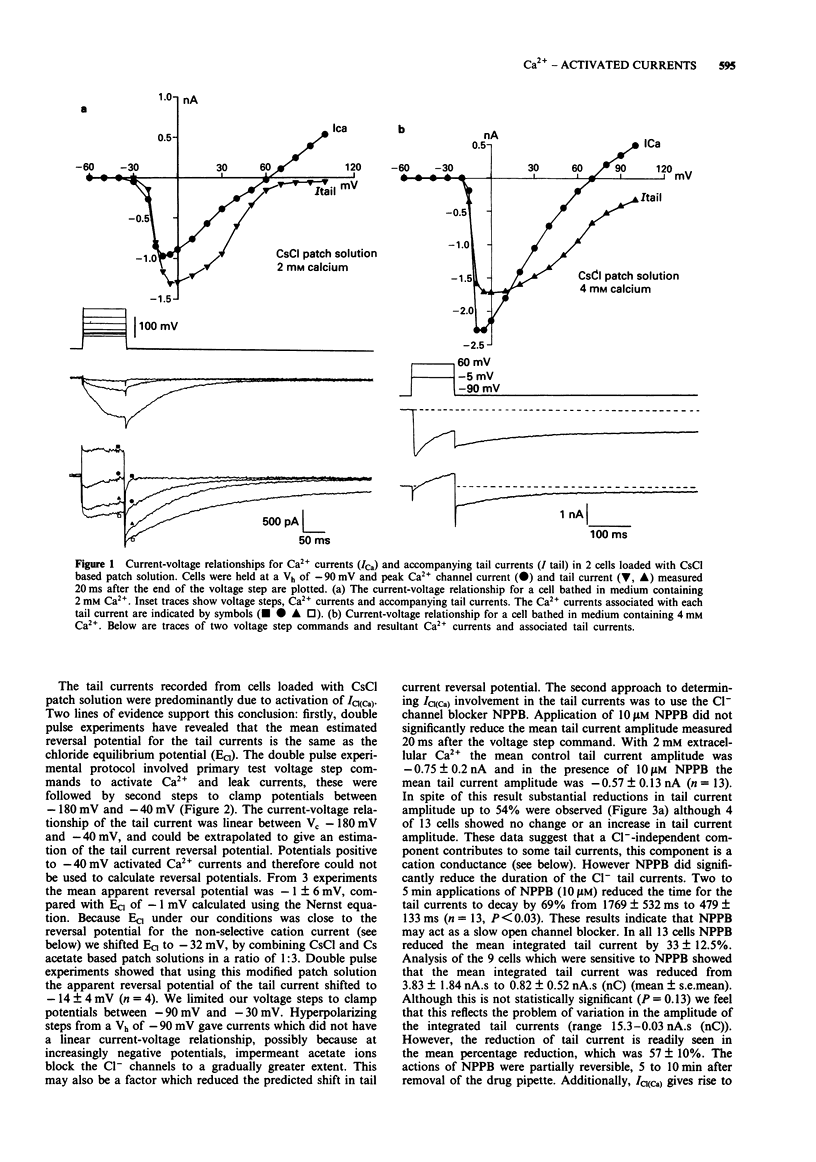
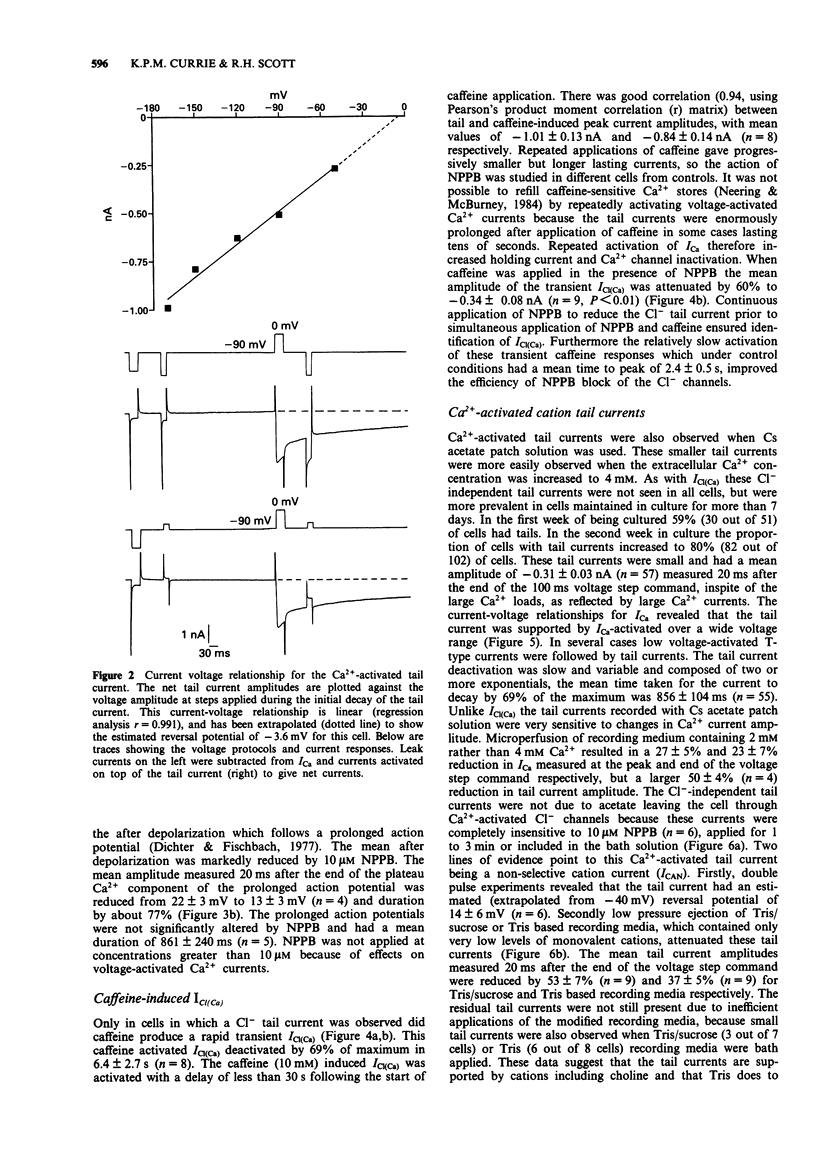
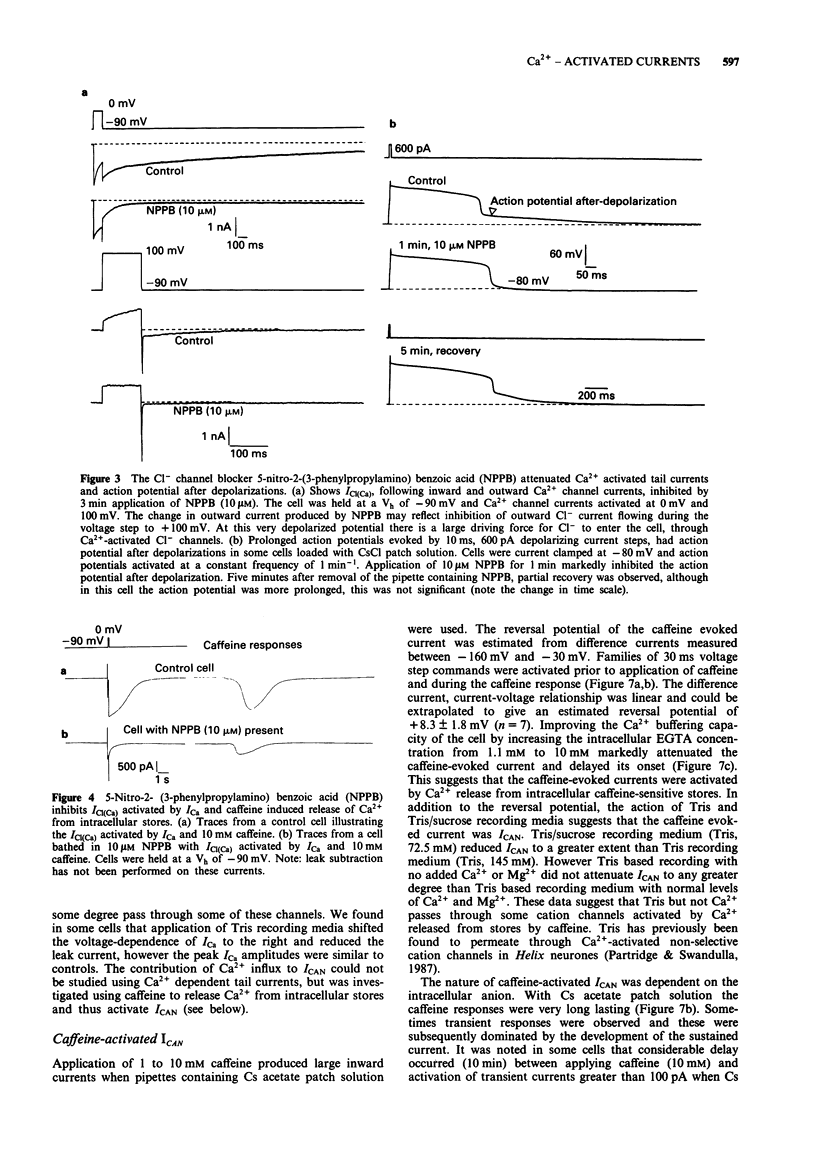
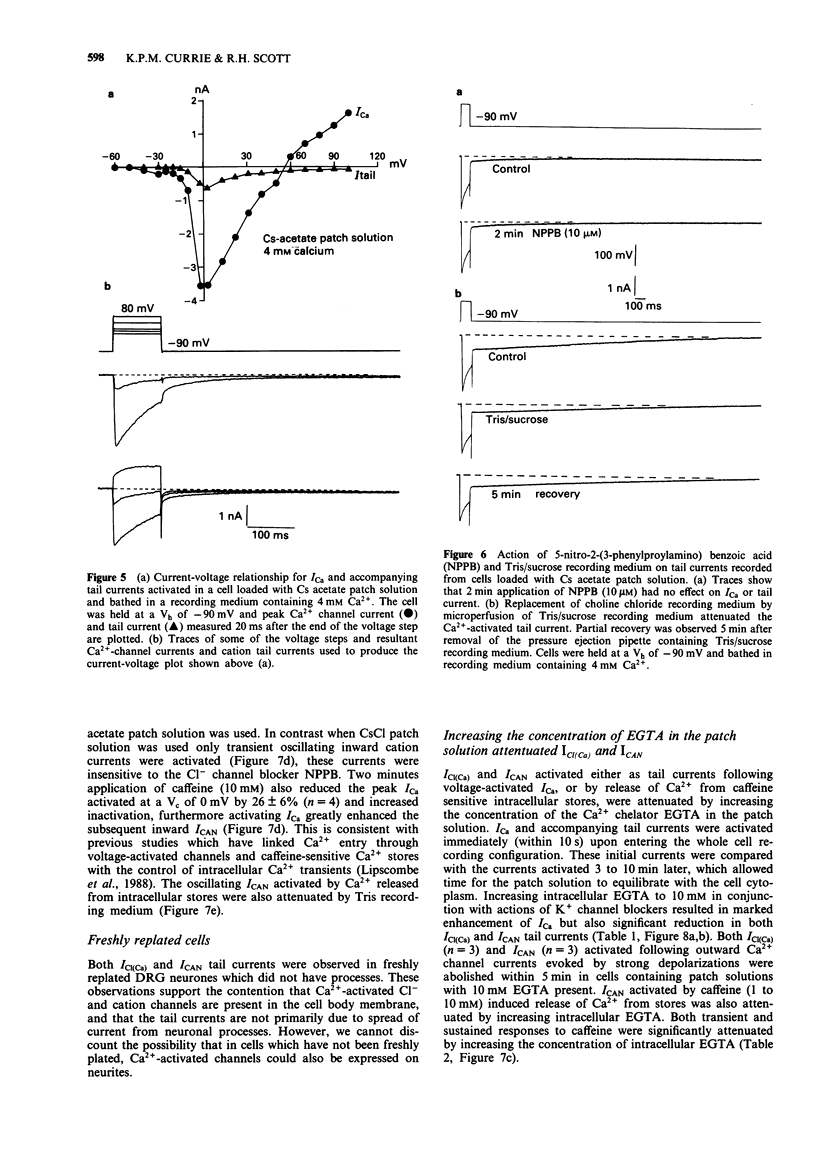
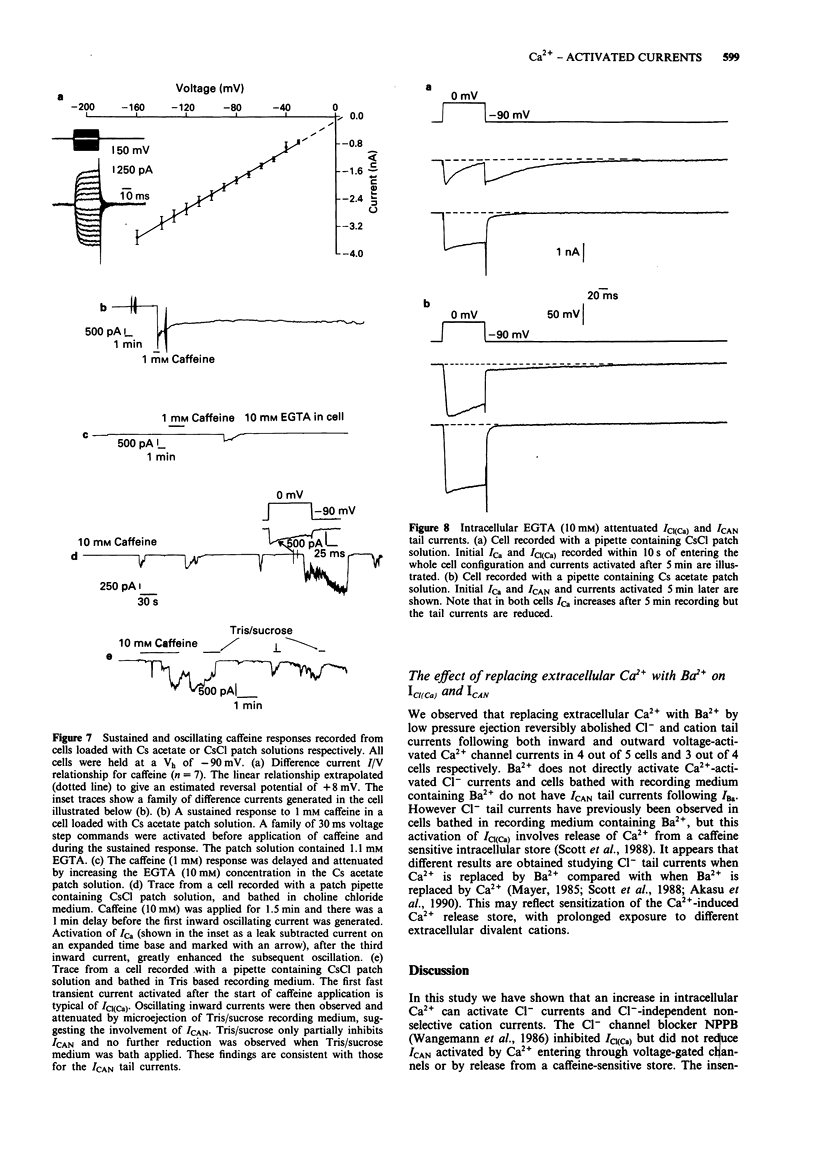
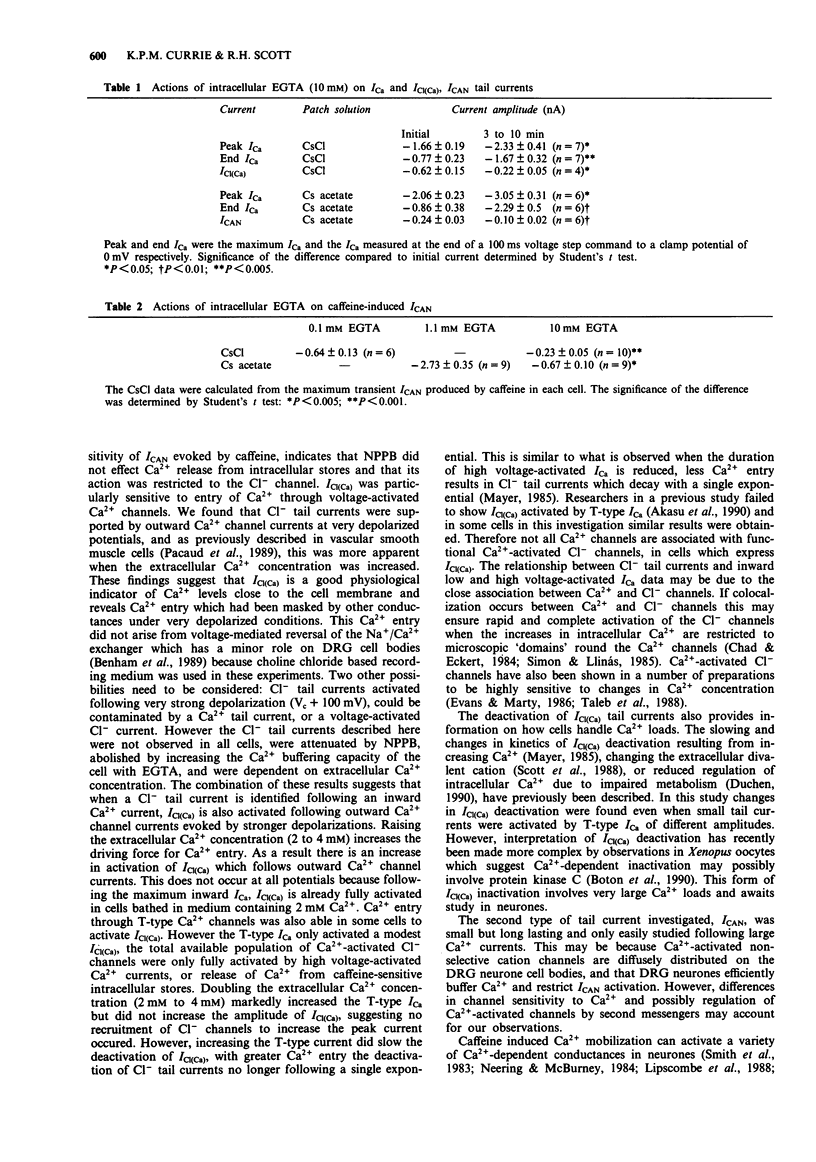
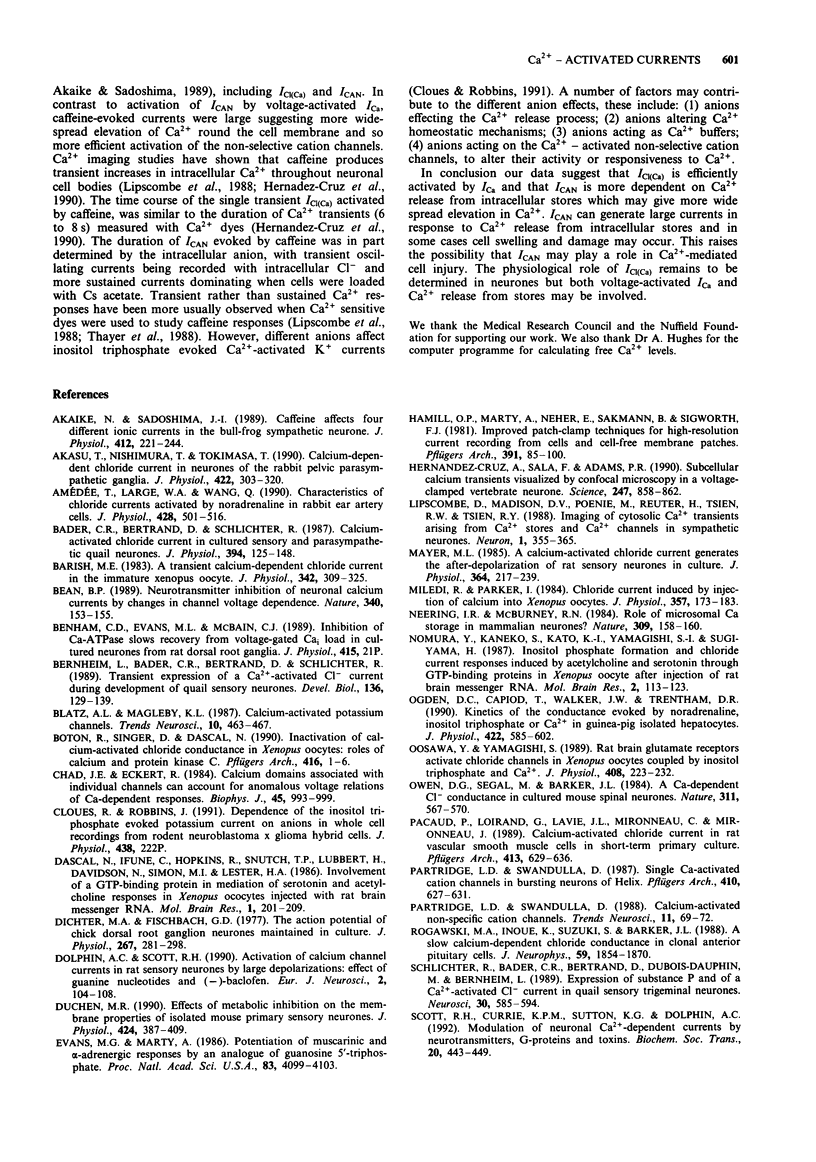
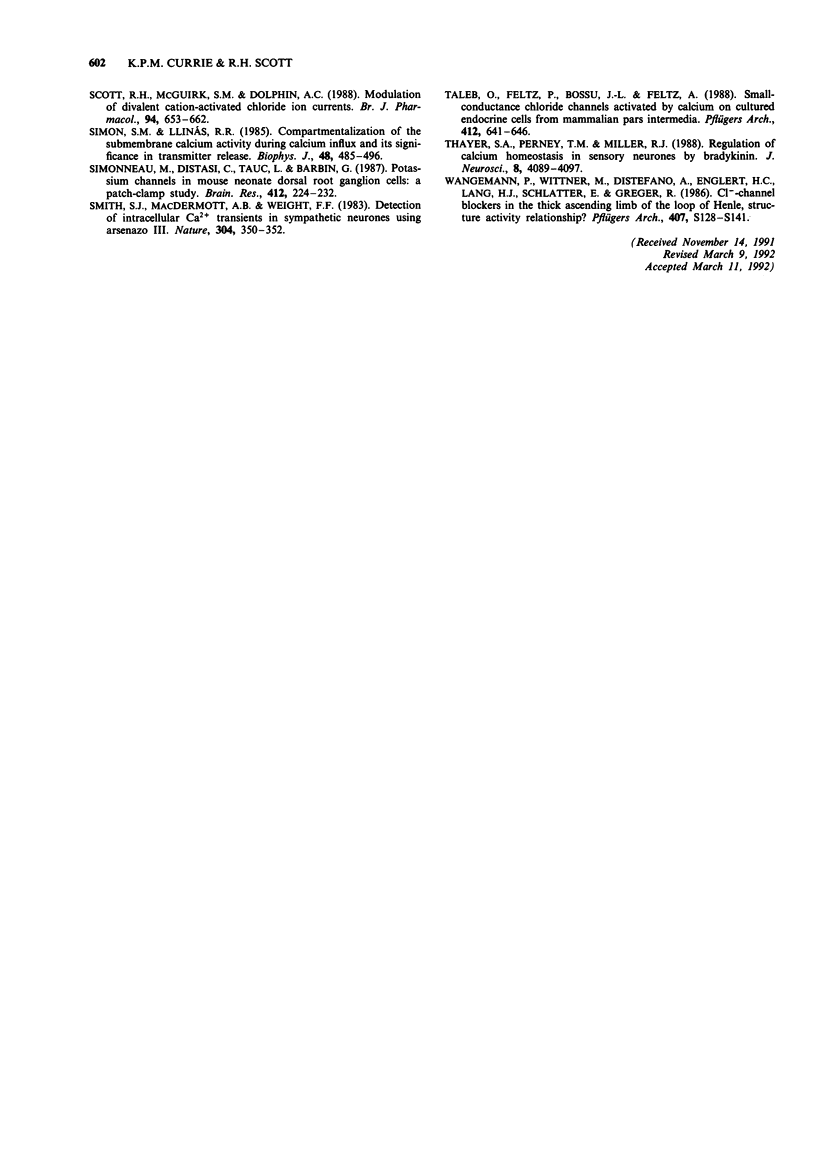
Selected References
These references are in PubMed. This may not be the complete list of references from this article.
- Akaike N., Sadoshima J. Caffeine affects four different ionic currents in the bull-frog sympathetic neurone. J Physiol. 1989 May;412:221–244. doi: 10.1113/jphysiol.1989.sp017612. [DOI] [PMC free article] [PubMed] [Google Scholar]
- Akasu T., Nishimura T., Tokimasa T. Calcium-dependent chloride current in neurones of the rabbit pelvic parasympathetic ganglia. J Physiol. 1990 Mar;422:303–320. doi: 10.1113/jphysiol.1990.sp017985. [DOI] [PMC free article] [PubMed] [Google Scholar]
- Amédée T., Large W. A., Wang Q. Characteristics of chloride currents activated by noradrenaline in rabbit ear artery cells. J Physiol. 1990 Sep;428:501–516. doi: 10.1113/jphysiol.1990.sp018224. [DOI] [PMC free article] [PubMed] [Google Scholar]
- Bader C. R., Bertrand D., Schlichter R. Calcium-activated chloride current in cultured sensory and parasympathetic quail neurones. J Physiol. 1987 Dec;394:125–148. doi: 10.1113/jphysiol.1987.sp016863. [DOI] [PMC free article] [PubMed] [Google Scholar]
- Barish M. E. A transient calcium-dependent chloride current in the immature Xenopus oocyte. J Physiol. 1983 Sep;342:309–325. doi: 10.1113/jphysiol.1983.sp014852. [DOI] [PMC free article] [PubMed] [Google Scholar]
- Bean B. P. Neurotransmitter inhibition of neuronal calcium currents by changes in channel voltage dependence. Nature. 1989 Jul 13;340(6229):153–156. doi: 10.1038/340153a0. [DOI] [PubMed] [Google Scholar]
- Bernheim L., Bader C. R., Bertrand D., Schlichter R. Transient expression of a Ca2+-activated Cl- current during development of quail sensory neurons. Dev Biol. 1989 Nov;136(1):129–139. doi: 10.1016/0012-1606(89)90136-x. [DOI] [PubMed] [Google Scholar]
- Boton R., Singer D., Dascal N. Inactivation of calcium-activated chloride conductance in Xenopus oocytes: roles of calcium and protein kinase C. Pflugers Arch. 1990 Apr;416(1-2):1–6. doi: 10.1007/BF00370214. [DOI] [PubMed] [Google Scholar]
- Chad J. E., Eckert R. Calcium domains associated with individual channels can account for anomalous voltage relations of CA-dependent responses. Biophys J. 1984 May;45(5):993–999. doi: 10.1016/S0006-3495(84)84244-7. [DOI] [PMC free article] [PubMed] [Google Scholar]
- Dascal N., Ifune C., Hopkins R., Snutch T. P., Lübbert H., Davidson N., Simon M. I., Lester H. A. Involvement of a GTP-binding protein in mediation of serotonin and acetylcholine responses in Xenopus oocytes injected with rat brain messenger RNA. Brain Res. 1986 Dec;387(3):201–209. doi: 10.1016/0169-328x(86)90026-4. [DOI] [PubMed] [Google Scholar]
- Dichter M. A., Fischbach G. D. The action potential of chick dorsal root ganglion neurones maintained in cell culture. J Physiol. 1977 May;267(2):281–298. doi: 10.1113/jphysiol.1977.sp011813. [DOI] [PMC free article] [PubMed] [Google Scholar]
- Dolphin A. C., Scott R. H. Activation of calcium channel currents in rat sensory neurons by large depolarizations: effect of Guanine nucleotides and (-)-baclofen. Eur J Neurosci. 1990;2(1):104–108. doi: 10.1111/j.1460-9568.1990.tb00386.x. [DOI] [PubMed] [Google Scholar]
- Duchen M. R. Effects of metabolic inhibition on the membrane properties of isolated mouse primary sensory neurones. J Physiol. 1990 May;424:387–409. doi: 10.1113/jphysiol.1990.sp018073. [DOI] [PMC free article] [PubMed] [Google Scholar]
- Evans M. G., Marty A. Potentiation of muscarinic and alpha-adrenergic responses by an analogue of guanosine 5'-triphosphate. Proc Natl Acad Sci U S A. 1986 Jun;83(11):4099–4103. doi: 10.1073/pnas.83.11.4099. [DOI] [PMC free article] [PubMed] [Google Scholar]
- Hamill O. P., Marty A., Neher E., Sakmann B., Sigworth F. J. Improved patch-clamp techniques for high-resolution current recording from cells and cell-free membrane patches. Pflugers Arch. 1981 Aug;391(2):85–100. doi: 10.1007/BF00656997. [DOI] [PubMed] [Google Scholar]
- Hernández-Cruz A., Sala F., Adams P. R. Subcellular calcium transients visualized by confocal microscopy in a voltage-clamped vertebrate neuron. Science. 1990 Feb 16;247(4944):858–862. doi: 10.1126/science.2154851. [DOI] [PubMed] [Google Scholar]
- Lipscombe D., Madison D. V., Poenie M., Reuter H., Tsien R. W., Tsien R. Y. Imaging of cytosolic Ca2+ transients arising from Ca2+ stores and Ca2+ channels in sympathetic neurons. Neuron. 1988 Jul;1(5):355–365. doi: 10.1016/0896-6273(88)90185-7. [DOI] [PubMed] [Google Scholar]
- Mayer M. L. A calcium-activated chloride current generates the after-depolarization of rat sensory neurones in culture. J Physiol. 1985 Jul;364:217–239. doi: 10.1113/jphysiol.1985.sp015740. [DOI] [PMC free article] [PubMed] [Google Scholar]
- Miledi R., Parker I. Chloride current induced by injection of calcium into Xenopus oocytes. J Physiol. 1984 Dec;357:173–183. doi: 10.1113/jphysiol.1984.sp015495. [DOI] [PMC free article] [PubMed] [Google Scholar]
- Neering I. R., McBurney R. N. Role for microsomal Ca storage in mammalian neurones? Nature. 1984 May 10;309(5964):158–160. doi: 10.1038/309158a0. [DOI] [PubMed] [Google Scholar]
- Ogden D. C., Capiod T., Walker J. W., Trentham D. R. Kinetics of the conductance evoked by noradrenaline, inositol trisphosphate or Ca2+ in guinea-pig isolated hepatocytes. J Physiol. 1990 Mar;422:585–602. doi: 10.1113/jphysiol.1990.sp018002. [DOI] [PMC free article] [PubMed] [Google Scholar]
- Oosawa Y., Yamagishi S. Rat brain glutamate receptors activate chloride channels in Xenopus oocytes coupled by inositol trisphosphate and Ca2+. J Physiol. 1989 Jan;408:223–232. doi: 10.1113/jphysiol.1989.sp017456. [DOI] [PMC free article] [PubMed] [Google Scholar]
- Owen D. G., Segal M., Barker J. L. A Ca-dependent Cl- conductance in cultured mouse spinal neurones. Nature. 1984 Oct 11;311(5986):567–570. doi: 10.1038/311567a0. [DOI] [PubMed] [Google Scholar]
- Pacaud P., Loirand G., Lavie J. L., Mironneau C., Mironneau J. Calcium-activated chloride current in rat vascular smooth muscle cells in short-term primary culture. Pflugers Arch. 1989 Apr;413(6):629–636. doi: 10.1007/BF00581813. [DOI] [PubMed] [Google Scholar]
- Partridge L. D., Swandulla D. Calcium-activated non-specific cation channels. Trends Neurosci. 1988 Feb;11(2):69–72. doi: 10.1016/0166-2236(88)90167-1. [DOI] [PubMed] [Google Scholar]
- Partridge L. D., Swandulla D. Single Ca-activated cation channels in bursting neurons of Helix. Pflugers Arch. 1987 Dec;410(6):627–631. doi: 10.1007/BF00581323. [DOI] [PubMed] [Google Scholar]
- Rogawski M. A., Inoue K., Suzuki S., Barker J. L. A slow calcium-dependent chloride conductance in clonal anterior pituitary cells. J Neurophysiol. 1988 Jun;59(6):1854–1870. doi: 10.1152/jn.1988.59.6.1854. [DOI] [PubMed] [Google Scholar]
- Schlichter R., Bader C. R., Bertrand D., Dubois-Dauphin M., Bernheim L. Expression of substance P and of a Ca2+-activated Cl- current in quail sensory trigeminal neurons. Neuroscience. 1989;30(3):585–594. doi: 10.1016/0306-4522(89)90153-x. [DOI] [PubMed] [Google Scholar]
- Scott R. H., Currie K. P., Sutton K. G., Dolphin A. C. Modulation of neuronal Ca(2+)-dependent currents by neurotransmitters, G-proteins and toxins. Biochem Soc Trans. 1992 May;20(2):443–449. doi: 10.1042/bst0200443. [DOI] [PubMed] [Google Scholar]
- Scott R. H., McGuirk S. M., Dolphin A. C. Modulation of divalent cation-activated chloride ion currents. Br J Pharmacol. 1988 Jul;94(3):653–662. doi: 10.1111/j.1476-5381.1988.tb11572.x. [DOI] [PMC free article] [PubMed] [Google Scholar]
- Simon S. M., Llinás R. R. Compartmentalization of the submembrane calcium activity during calcium influx and its significance in transmitter release. Biophys J. 1985 Sep;48(3):485–498. doi: 10.1016/S0006-3495(85)83804-2. [DOI] [PMC free article] [PubMed] [Google Scholar]
- Simonneau M., Distasi C., Tauc L., Barbin G. Potassium channels in mouse neonate dorsal root ganglion cells: a patch-clamp study. Brain Res. 1987 Jun 2;412(2):224–232. doi: 10.1016/0006-8993(87)91128-0. [DOI] [PubMed] [Google Scholar]
- Smith S. J., MacDermott A. B., Weight F. F. Detection of intracellular Ca2+ transients in sympathetic neurones using arsenazo III. 1983 Jul 28-Aug 3Nature. 304(5924):350–352. doi: 10.1038/304350a0. [DOI] [PubMed] [Google Scholar]
- Taleb O., Feltz P., Bossu J. L., Feltz A. Small-conductance chloride channels activated by calcium on cultured endocrine cells from mammalian pars intermedia. Pflugers Arch. 1988 Oct;412(6):641–646. doi: 10.1007/BF00583766. [DOI] [PubMed] [Google Scholar]
- Thayer S. A., Perney T. M., Miller R. J. Regulation of calcium homeostasis in sensory neurons by bradykinin. J Neurosci. 1988 Nov;8(11):4089–4097. doi: 10.1523/JNEUROSCI.08-11-04089.1988. [DOI] [PMC free article] [PubMed] [Google Scholar]
- Wangemann P., Wittner M., Di Stefano A., Englert H. C., Lang H. J., Schlatter E., Greger R. Cl(-)-channel blockers in the thick ascending limb of the loop of Henle. Structure activity relationship. Pflugers Arch. 1986;407 (Suppl 2):S128–S141. doi: 10.1007/BF00584942. [DOI] [PubMed] [Google Scholar]