Abstract
1. The influence of different holding potentials (-120 to -70 mV) on the contraction of enzymatically dispersed myocytes from guinea-pig hearts was evaluated. Contractions were elicited by repetitive depolarizations to 0 mV at 0.5 Hz. 2. While ineffective at 140 and 5 mmol l-1 [Na+]o and pipette Na+, respectively, depolarization of the resting membrane with the holding potential increased myocyte shortening at reduced Na+ gradients ([Na+]o 70 or [Na+]i 10-15 mmol l-1). Elevated intracellular Na+ after Na(+)-pump inhibition with ouabain 1-10 mumol l-1 was similarly effective with regard to the inotropic response to different holding potentials. 3. At -70 mV holding potential, reduction of [Na+]o from 140 to 70 mmol l-1 increased myocyte shortening and induced an inwardly directed component of the holding current which peaked at -44 +/- 10 pA and declined thereafter in parallel with the inotropic effect. The relation of this inward current to [Ca2+]i was confirmed by experiments at high Ca2+ buffer capacity where [Na+]o reduction induced a Ni(2+)-insensitive, outwardly directed component (36 +/- 15 pA) of the holding current. The observed inward current is suggested to reflect the extrusion of [Ca2+]i in exchange for [Na+]o as a counter-regulatory mechanism which limits the increase of [Ca2+]i. 4. The interventions which increased the strength of the contraction also enhanced the transient tail current after repolarization, suggesting its close relation to [Ca2+]i. This finding confirmed the pattern found with cell shortening. 5. It is concluded that under certain conditions, voltage-dependent and Na(+)-dependent Na(+)-Ca2+ exchange during the interval between the contractions is relevant to the diastolic concentration of [Ca2+]i which in turn determines the accumulation of Ca2+ in the sarcoplasmic reticulum and the magnitude of the subsequent contraction.
Full text
PDF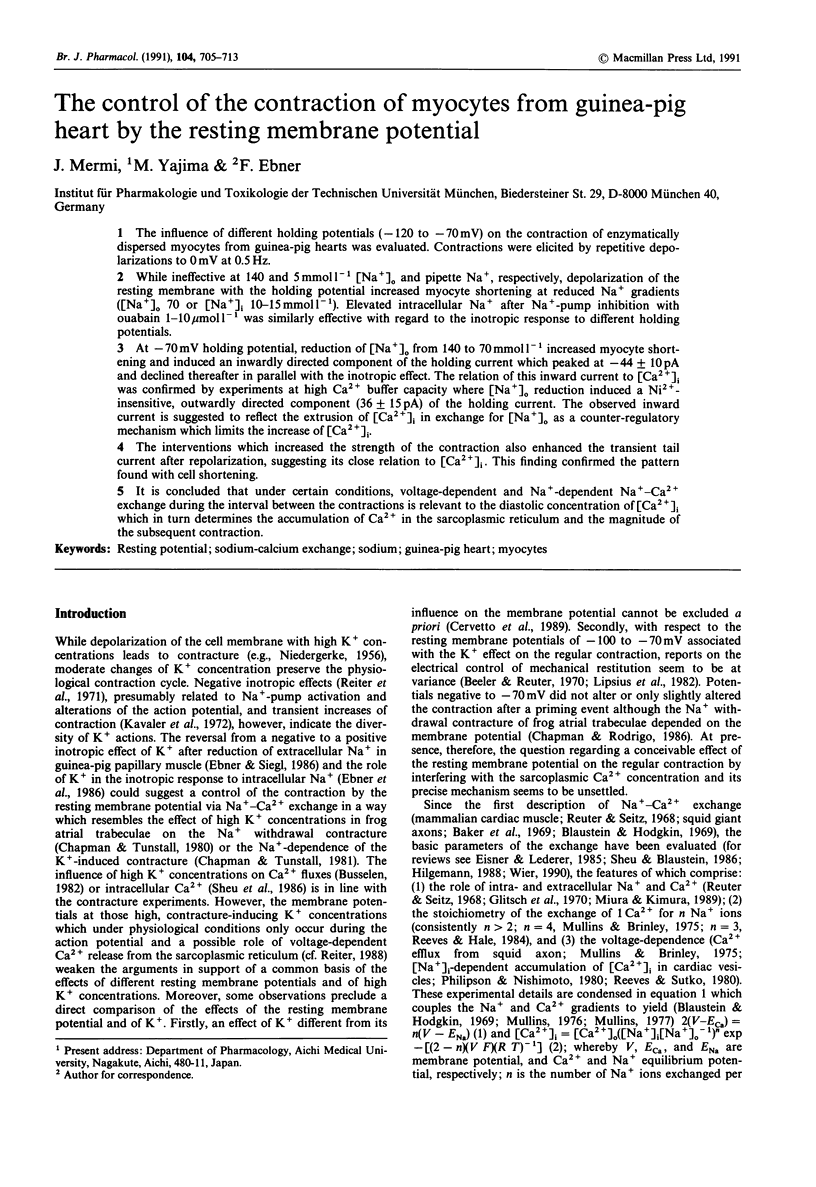
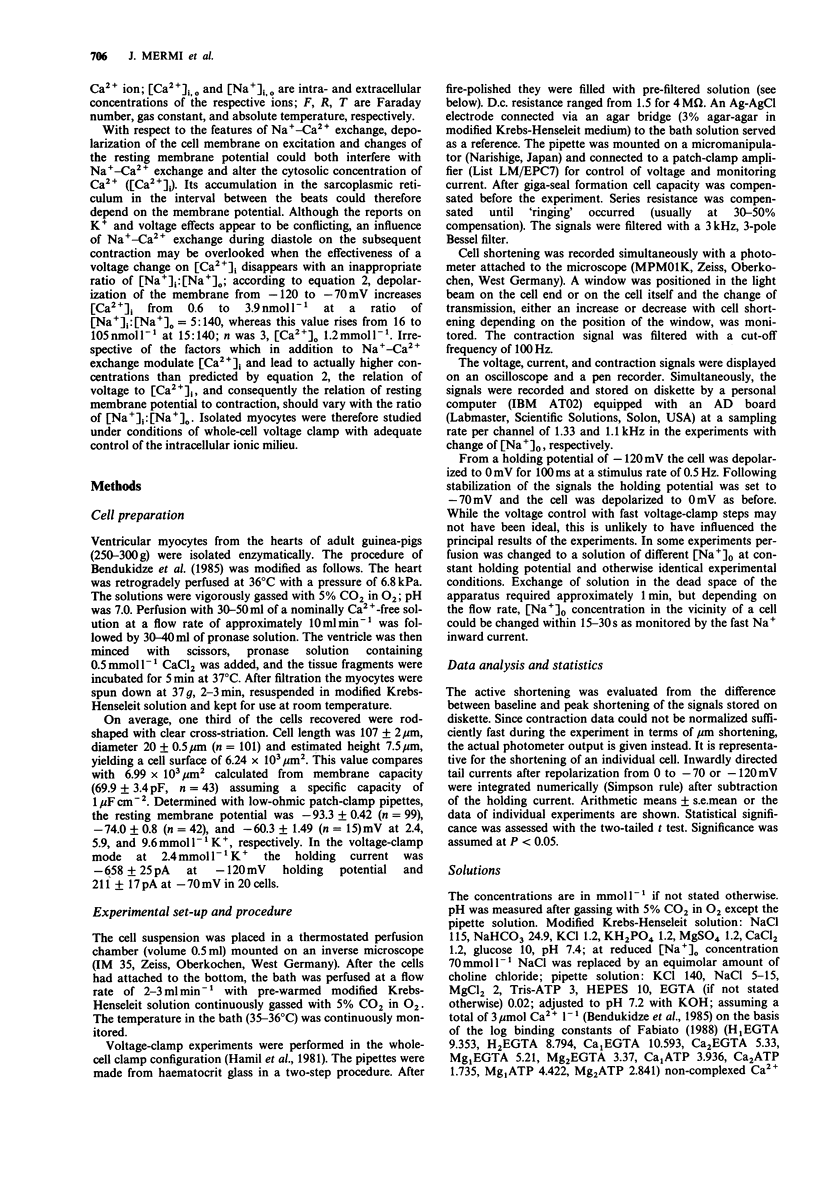
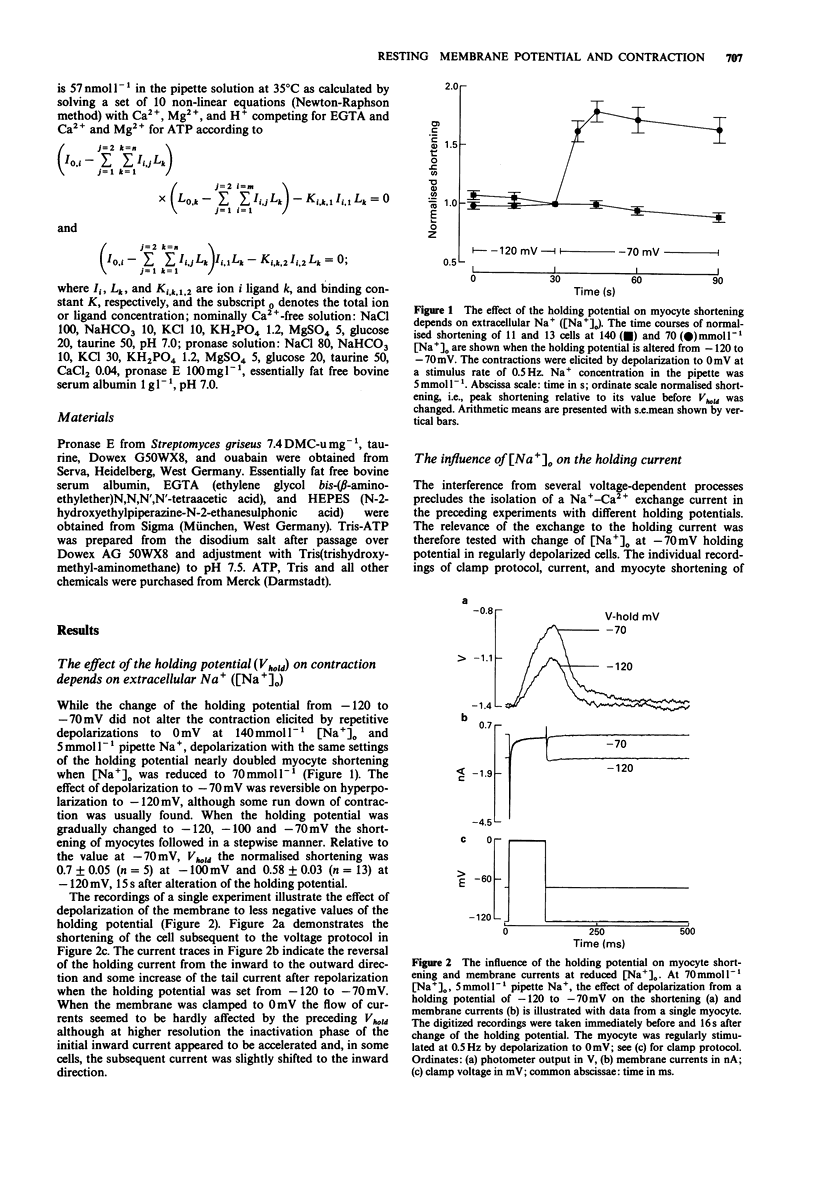
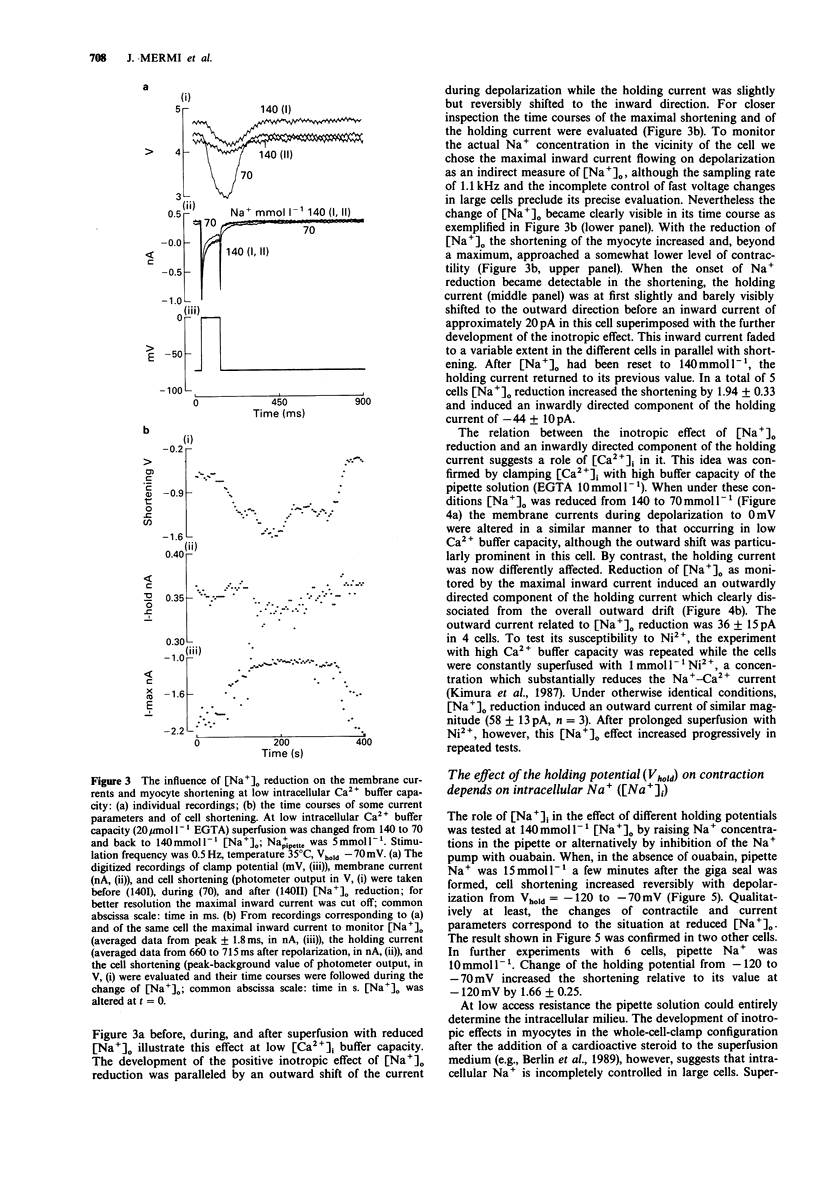
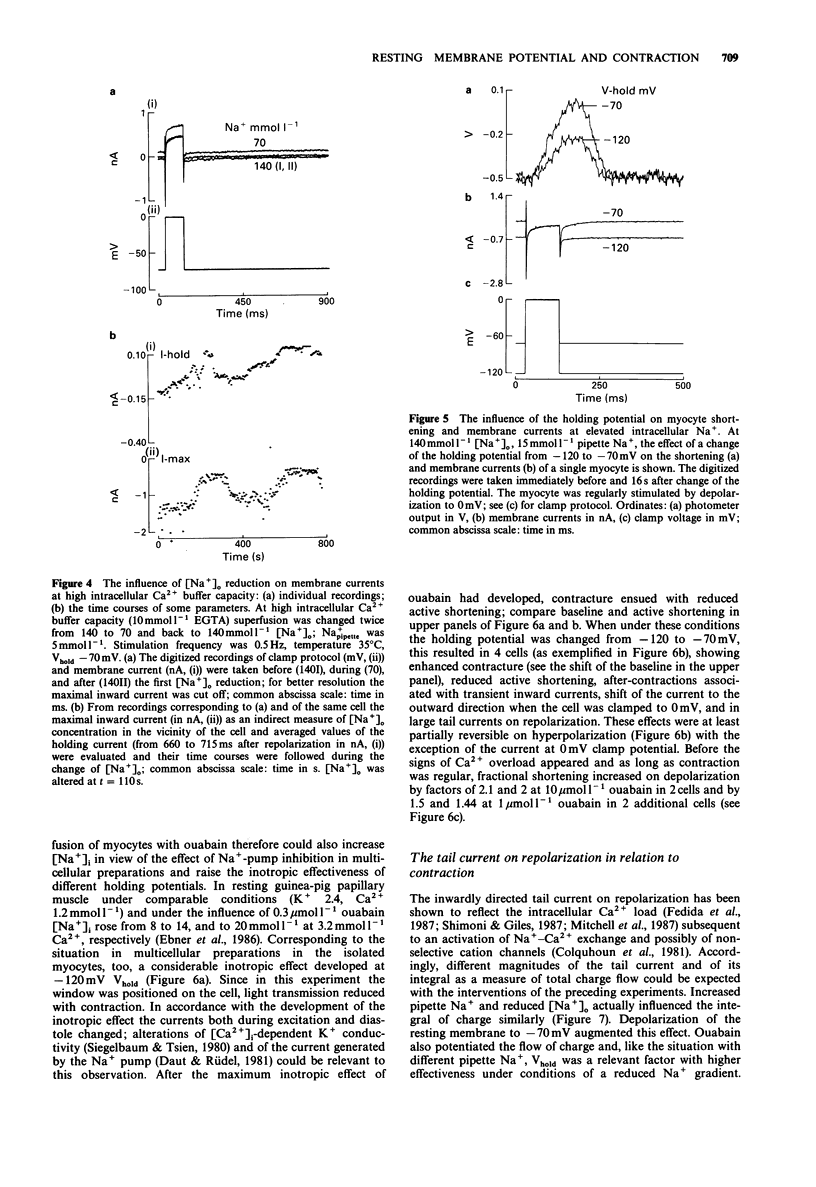
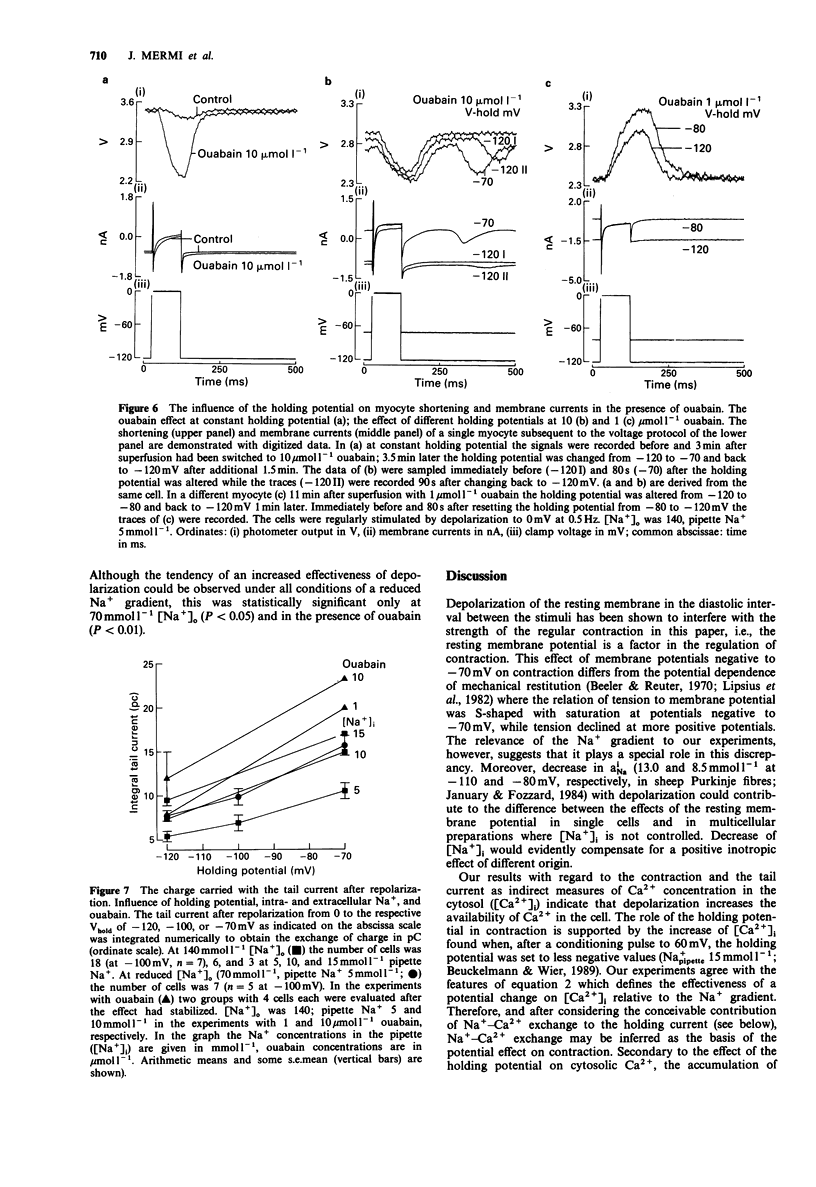
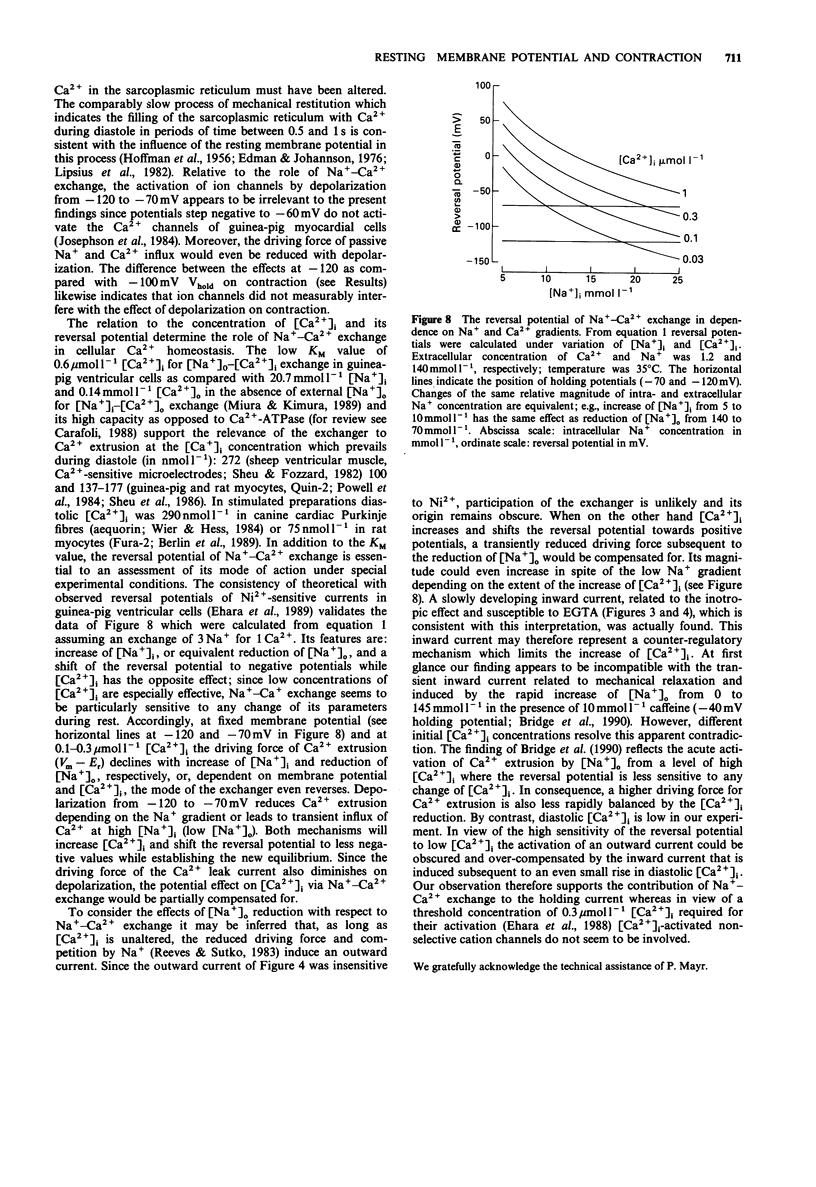
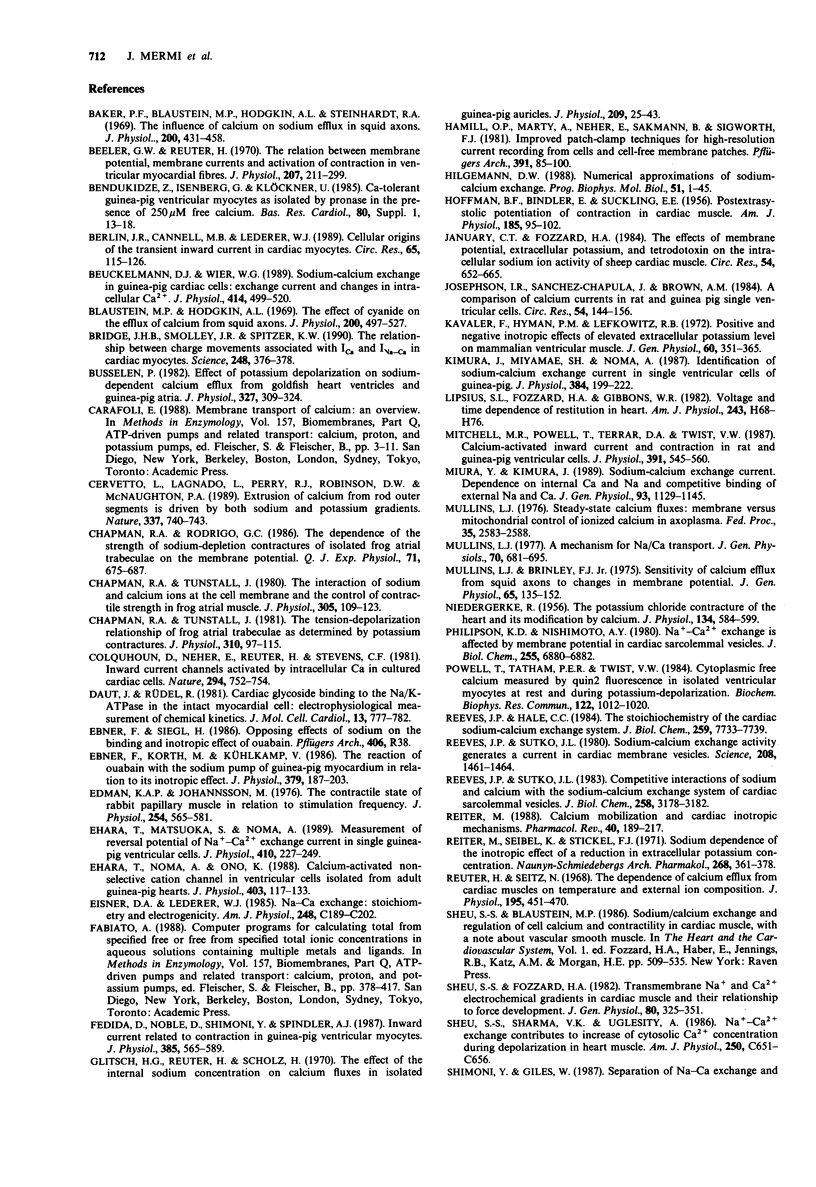
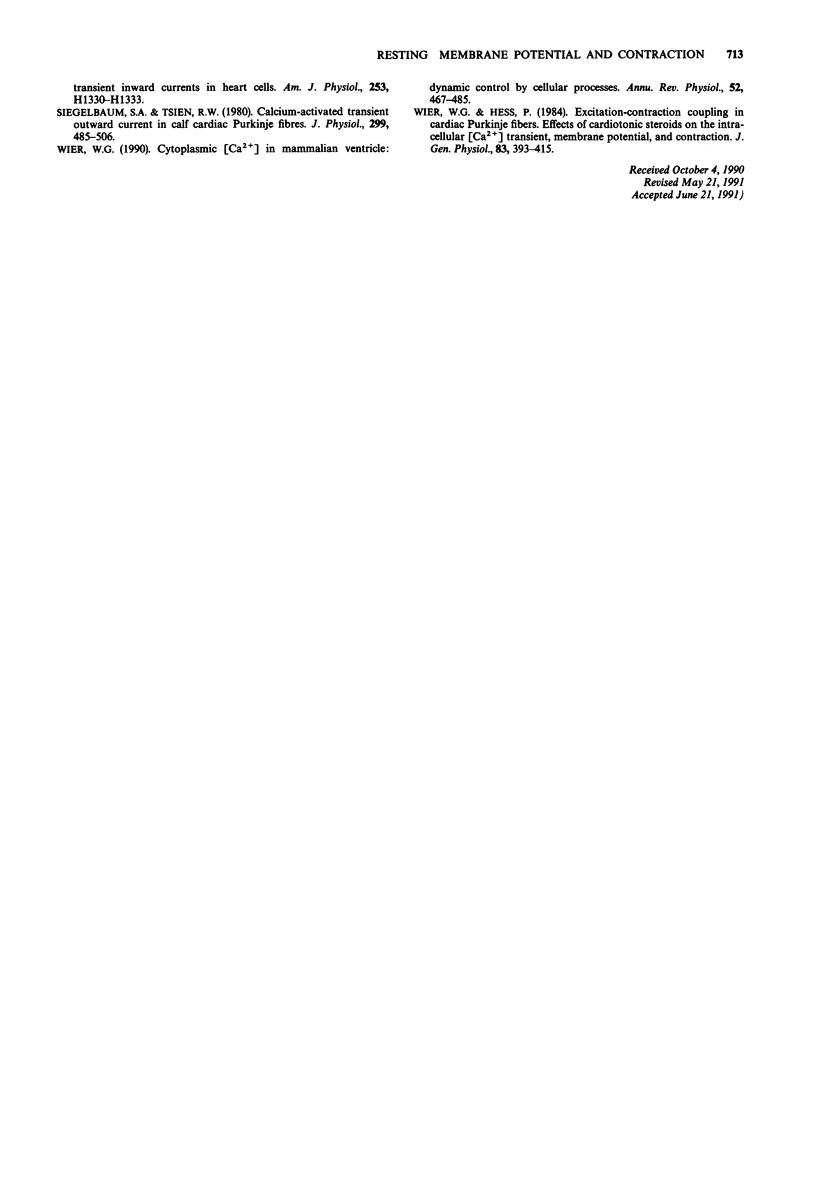
Selected References
These references are in PubMed. This may not be the complete list of references from this article.
- Baker P. F., Blaustein M. P., Hodgkin A. L., Steinhardt R. A. The influence of calcium on sodium efflux in squid axons. J Physiol. 1969 Feb;200(2):431–458. doi: 10.1113/jphysiol.1969.sp008702. [DOI] [PMC free article] [PubMed] [Google Scholar]
- Beeler G. W., Jr, Reuter H. The relation between membrane potential, membrane currents and activation of contraction in ventricular myocardial fibres. J Physiol. 1970 Mar;207(1):211–229. doi: 10.1113/jphysiol.1970.sp009057. [DOI] [PMC free article] [PubMed] [Google Scholar]
- Berlin J. R., Cannell M. B., Lederer W. J. Cellular origins of the transient inward current in cardiac myocytes. Role of fluctuations and waves of elevated intracellular calcium. Circ Res. 1989 Jul;65(1):115–126. doi: 10.1161/01.res.65.1.115. [DOI] [PubMed] [Google Scholar]
- Beuckelmann D. J., Wier W. G. Sodium-calcium exchange in guinea-pig cardiac cells: exchange current and changes in intracellular Ca2+. J Physiol. 1989 Jul;414:499–520. doi: 10.1113/jphysiol.1989.sp017700. [DOI] [PMC free article] [PubMed] [Google Scholar]
- Blaustein M. P., Hodgkin A. L. The effect of cyanide on the efflux of calcium from squid axons. J Physiol. 1969 Feb;200(2):497–527. doi: 10.1113/jphysiol.1969.sp008704. [DOI] [PMC free article] [PubMed] [Google Scholar]
- Bridge J. H., Smolley J. R., Spitzer K. W. The relationship between charge movements associated with ICa and INa-Ca in cardiac myocytes. Science. 1990 Apr 20;248(4953):376–378. doi: 10.1126/science.2158147. [DOI] [PubMed] [Google Scholar]
- Busselen P. Effect of potassium depolarization on sodium-dependent calcium efflux from goldfish heart ventricles and guinea-pig atria. J Physiol. 1982 Jun;327:309–324. doi: 10.1113/jphysiol.1982.sp014233. [DOI] [PMC free article] [PubMed] [Google Scholar]
- Carafoli E. Membrane transport of calcium: an overview. Methods Enzymol. 1988;157:3–11. doi: 10.1016/0076-6879(88)57063-5. [DOI] [PubMed] [Google Scholar]
- Cervetto L., Lagnado L., Perry R. J., Robinson D. W., McNaughton P. A. Extrusion of calcium from rod outer segments is driven by both sodium and potassium gradients. Nature. 1989 Feb 23;337(6209):740–743. doi: 10.1038/337740a0. [DOI] [PubMed] [Google Scholar]
- Chapman R. A., Rodrigo G. C. The dependence of the strength of sodium-depletion contractures of isolated frog atrial trabeculae on the membrane potential. Q J Exp Physiol. 1986 Oct;71(4):675–687. doi: 10.1113/expphysiol.1986.sp003026. [DOI] [PubMed] [Google Scholar]
- Chapman R. A., Tunstall J. The interaction of sodium and calcium ions at the cell membrane and the control of contractile strength in frog atrial muscle. J Physiol. 1980 Aug;305:109–123. doi: 10.1113/jphysiol.1980.sp013353. [DOI] [PMC free article] [PubMed] [Google Scholar]
- Chapman R. A., Tunstall J. The tension-depolarization relationship of frog atrial trabeculae as determined by potassium contractures. J Physiol. 1981 Jan;310:97–115. doi: 10.1113/jphysiol.1981.sp013539. [DOI] [PMC free article] [PubMed] [Google Scholar]
- Colquhoun D., Neher E., Reuter H., Stevens C. F. Inward current channels activated by intracellular Ca in cultured cardiac cells. Nature. 1981 Dec 24;294(5843):752–754. doi: 10.1038/294752a0. [DOI] [PubMed] [Google Scholar]
- Daut J., Rüdel R. Cardiac glycoside binding to the Na/K-ATPase in the intact myocardial cell: electrophysiological measurement of chemical kinetics. J Mol Cell Cardiol. 1981 Aug;13(8):777–782. doi: 10.1016/0022-2828(81)90260-1. [DOI] [PubMed] [Google Scholar]
- Ebner F., Korth M., Kühlkamp V. The reaction of ouabain with the sodium pump of guinea-pig myocardium in relation to its inotropic effect. J Physiol. 1986 Oct;379:187–203. doi: 10.1113/jphysiol.1986.sp016247. [DOI] [PMC free article] [PubMed] [Google Scholar]
- Edman K. A., Jóhannsson M. The contractile state of rabbit papillary muscle in relation to stimulation frequency. J Physiol. 1976 Jan;254(3):565–581. doi: 10.1113/jphysiol.1976.sp011247. [DOI] [PMC free article] [PubMed] [Google Scholar]
- Ehara T., Matsuoka S., Noma A. Measurement of reversal potential of Na+-Ca2+ exchange current in single guinea-pig ventricular cells. J Physiol. 1989 Mar;410:227–249. doi: 10.1113/jphysiol.1989.sp017530. [DOI] [PMC free article] [PubMed] [Google Scholar]
- Ehara T., Noma A., Ono K. Calcium-activated non-selective cation channel in ventricular cells isolated from adult guinea-pig hearts. J Physiol. 1988 Sep;403:117–133. doi: 10.1113/jphysiol.1988.sp017242. [DOI] [PMC free article] [PubMed] [Google Scholar]
- Eisner D. A., Lederer W. J. Na-Ca exchange: stoichiometry and electrogenicity. Am J Physiol. 1985 Mar;248(3 Pt 1):C189–C202. doi: 10.1152/ajpcell.1985.248.3.C189. [DOI] [PubMed] [Google Scholar]
- Fabiato A. Computer programs for calculating total from specified free or free from specified total ionic concentrations in aqueous solutions containing multiple metals and ligands. Methods Enzymol. 1988;157:378–417. doi: 10.1016/0076-6879(88)57093-3. [DOI] [PubMed] [Google Scholar]
- Fedida D., Noble D., Shimoni Y., Spindler A. J. Inward current related to contraction in guinea-pig ventricular myocytes. J Physiol. 1987 Apr;385:565–589. doi: 10.1113/jphysiol.1987.sp016508. [DOI] [PMC free article] [PubMed] [Google Scholar]
- Glitsch H. G., Reuter H., Scholz H. The effect of the internal sodium concentration on calcium fluxes in isolated guinea-pig auricles. J Physiol. 1970 Jul;209(1):25–43. doi: 10.1113/jphysiol.1970.sp009153. [DOI] [PMC free article] [PubMed] [Google Scholar]
- HOFFMAN B. F., BINDLER E., SUCKLING E. E. Postextrasystolic potentiation of contraction in cardiac muscle. Am J Physiol. 1956 Apr;185(1):95–102. doi: 10.1152/ajplegacy.1956.185.1.95. [DOI] [PubMed] [Google Scholar]
- Hamill O. P., Marty A., Neher E., Sakmann B., Sigworth F. J. Improved patch-clamp techniques for high-resolution current recording from cells and cell-free membrane patches. Pflugers Arch. 1981 Aug;391(2):85–100. doi: 10.1007/BF00656997. [DOI] [PubMed] [Google Scholar]
- Hilgemann D. W. Numerical approximations of sodium-calcium exchange. Prog Biophys Mol Biol. 1988;51(1):1–45. doi: 10.1016/0079-6107(88)90009-0. [DOI] [PubMed] [Google Scholar]
- January C. T., Fozzard H. A. The effects of membrane potential, extracellular potassium, and tetrodotoxin on the intracellular sodium ion activity of sheep cardiac muscle. Circ Res. 1984 Jun;54(6):652–665. doi: 10.1161/01.res.54.6.652. [DOI] [PubMed] [Google Scholar]
- Josephson I. R., Sanchez-Chapula J., Brown A. M. A comparison of calcium currents in rat and guinea pig single ventricular cells. Circ Res. 1984 Feb;54(2):144–156. doi: 10.1161/01.res.54.2.144. [DOI] [PubMed] [Google Scholar]
- Kavaler F., Hyman P. M., Lefkowitz R. B. Positive and negative inotropic effects of elevated extracellular potassium level on mammalian ventricular muscle. J Gen Physiol. 1972 Sep;60(3):351–365. doi: 10.1085/jgp.60.3.351. [DOI] [PMC free article] [PubMed] [Google Scholar]
- Kimura J., Miyamae S., Noma A. Identification of sodium-calcium exchange current in single ventricular cells of guinea-pig. J Physiol. 1987 Mar;384:199–222. doi: 10.1113/jphysiol.1987.sp016450. [DOI] [PMC free article] [PubMed] [Google Scholar]
- Lipsius S. L., Fozzard H. A., Gibbons W. R. Voltage and time dependence of restitution in heart. Am J Physiol. 1982 Jul;243(1):H68–H76. doi: 10.1152/ajpheart.1982.243.1.H68. [DOI] [PubMed] [Google Scholar]
- Mitchell M. R., Powell T., Terrar D. A., Twist V. W. Calcium-activated inward current and contraction in rat and guinea-pig ventricular myocytes. J Physiol. 1987 Oct;391:545–560. doi: 10.1113/jphysiol.1987.sp016755. [DOI] [PMC free article] [PubMed] [Google Scholar]
- Miura Y., Kimura J. Sodium-calcium exchange current. Dependence on internal Ca and Na and competitive binding of external Na and Ca. J Gen Physiol. 1989 Jun;93(6):1129–1145. doi: 10.1085/jgp.93.6.1129. [DOI] [PMC free article] [PubMed] [Google Scholar]
- Mullins L. J. A mechanism for Na/Ca transport. J Gen Physiol. 1977 Dec;70(6):681–695. doi: 10.1085/jgp.70.6.681. [DOI] [PMC free article] [PubMed] [Google Scholar]
- Mullins L. J., Brinley F. J., Jr Sensitivity of calcium efflux from squid axons to changes in membrane potential. J Gen Physiol. 1975 Feb;65(2):135–152. doi: 10.1085/jgp.65.2.135. [DOI] [PMC free article] [PubMed] [Google Scholar]
- Mullins L. J. Steady-state calcium fluxes: membrane versus mitochondrial control of ionized calcium in axoplasm. Fed Proc. 1976 Dec;35(14):2583–2588. [PubMed] [Google Scholar]
- NIEDERGERKE R. The potassium chloride contracture of the heart and its modification by calcium. J Physiol. 1956 Dec 28;134(3):584–599. doi: 10.1113/jphysiol.1956.sp005667. [DOI] [PMC free article] [PubMed] [Google Scholar]
- Philipson K. D., Nishimoto A. Y. Na+-Ca2+ exchange is affected by membrane potential in cardiac sarcolemmal vesicles. J Biol Chem. 1980 Jul 25;255(14):6880–6882. [PubMed] [Google Scholar]
- Powell T., Tatham P. E., Twist V. W. Cytoplasmic free calcium measured by quin2 fluorescence in isolated ventricular myocytes at rest and during potassium-depolarization. Biochem Biophys Res Commun. 1984 Aug 16;122(3):1012–1020. doi: 10.1016/0006-291x(84)91192-6. [DOI] [PubMed] [Google Scholar]
- Reeves J. P., Hale C. C. The stoichiometry of the cardiac sodium-calcium exchange system. J Biol Chem. 1984 Jun 25;259(12):7733–7739. [PubMed] [Google Scholar]
- Reeves J. P., Sutko J. L. Competitive interactions of sodium and calcium with the sodium-calcium exchange system of cardiac sarcolemmal vesicles. J Biol Chem. 1983 Mar 10;258(5):3178–3182. [PubMed] [Google Scholar]
- Reeves J. P., Sutko J. L. Sodium-calcium exchange activity generates a current in cardiac membrane vesicles. Science. 1980 Jun 27;208(4451):1461–1464. doi: 10.1126/science.7384788. [DOI] [PubMed] [Google Scholar]
- Reiter M. Calcium mobilization and cardiac inotropic mechanisms. Pharmacol Rev. 1988 Sep;40(3):189–217. [PubMed] [Google Scholar]
- Reiter M., Seibel K., Stickel F. J. Sodium dependence of the inotropic effect of a reduction in extracellular potassium concentration. Naunyn Schmiedebergs Arch Pharmakol. 1971;268(4):361–378. doi: 10.1007/BF00997062. [DOI] [PubMed] [Google Scholar]
- Reuter H., Seitz N. The dependence of calcium efflux from cardiac muscle on temperature and external ion composition. J Physiol. 1968 Mar;195(2):451–470. doi: 10.1113/jphysiol.1968.sp008467. [DOI] [PMC free article] [PubMed] [Google Scholar]
- Sheu S. S., Fozzard H. A. Transmembrane Na+ and Ca2+ electrochemical gradients in cardiac muscle and their relationship to force development. J Gen Physiol. 1982 Sep;80(3):325–351. doi: 10.1085/jgp.80.3.325. [DOI] [PMC free article] [PubMed] [Google Scholar]
- Sheu S. S., Sharma V. K., Uglesity A. Na+-Ca2+ exchange contributes to increase of cytosolic Ca2+ concentration during depolarization in heart muscle. Am J Physiol. 1986 Apr;250(4 Pt 1):C651–C656. doi: 10.1152/ajpcell.1986.250.4.C651. [DOI] [PubMed] [Google Scholar]
- Shimoni Y., Giles W. Separation of Na-Ca exchange and transient inward currents in heart cells. Am J Physiol. 1987 Nov;253(5 Pt 2):H1330–H1333. doi: 10.1152/ajpheart.1987.253.5.H1330. [DOI] [PubMed] [Google Scholar]
- Siegelbaum S. A., Tsien R. W. Calcium-activated transient outward current in calf cardiac Purkinje fibres. J Physiol. 1980 Feb;299:485–506. doi: 10.1113/jphysiol.1980.sp013138. [DOI] [PMC free article] [PubMed] [Google Scholar]
- Wier W. G. Cytoplasmic [Ca2+] in mammalian ventricle: dynamic control by cellular processes. Annu Rev Physiol. 1990;52:467–485. doi: 10.1146/annurev.ph.52.030190.002343. [DOI] [PubMed] [Google Scholar]
- Wier W. G., Hess P. Excitation-contraction coupling in cardiac Purkinje fibers. Effects of cardiotonic steroids on the intracellular [Ca2+] transient, membrane potential, and contraction. J Gen Physiol. 1984 Mar;83(3):395–415. doi: 10.1085/jgp.83.3.395. [DOI] [PMC free article] [PubMed] [Google Scholar]