Abstract
1. We used electrophysiological and binding techniques to determine the effects of oxygen free radicals (OFRs) generated by dihydroxyfumaric acid (DHF, 5 mM) on calcium current and dihydropyridine binding sites in guinea-pig isolated ventricular myocytes. 2. Binding of [3H]-PN200-110 to isolated ventricular myocytes revealed one population of binding sites with a KD of 0.11 +/- 0.01 nM and Bmax of 139.1 +/- 6.9 fmol mg-1 protein (n = 24). After 15 min of exposure to DHF, the density, but not the affinity of [3H]-PN200-110 binding sites was significantly (P < 0.01) reduced to 35% of the control value (Bmax = 49.4 +/- 3.7 fmol mg-1 protein, KD = 0.11 +/- 0.01 nM, n = 15). In the presence of superoxide dismutase (SOD) and catalase (CAT) the reduction in [3H]-PN200-110 binding sites was almost completely prevented (Bmax = 120.5 +/- 7.4 in control, n = 4 and 98.8 +/- 7.4 fmol mg-1 protein in DHF plus SOD and CAT, n = 4). KD values were not modified (0.08 +/- 0.01 in control and 0.09 +/- 0.01 nM in DHF plus SOD and CAT). 3. The time-course of the reduction of [3H]-PN200-110 binding sites by OFRs was paralleled by the decrease in L-type calcium current (Ica,L) measured in patch-clamped guinea-pig ventricular myocytes either in the absence or in the presence of EGTA in the patch pipette. In the former conditions OFRs induced the appearance of calcium-dependent alterations, i.e. the transient inward current, within 10 min. After 30 min of incubation with DHF, [3H]-PN200-110 binding sites were reduced to 25% of the control value. 4. In myocytes incubated with the antilipoperoxidant agent, butylated hydroxytoluene (BHT, 50 microM), the decrease in [3H]-PN200-110 binding sites caused by DHF was partially prevented (Bmax values after 30 min exposure to DHF were 55.5 +/- 1.9 and 23.7 +/- 5.9 fmol mg-1 protein in the presence and in the absence of BHT respectively, P < 0.05). BHT did not affect the decrease in [3H]-PN200-110 binding sites during the first 15 min of exposure to DHF, but was able to prevent completely the further decrease occurring during the following 15 min of incubation with OFRs. 5. Our results demonstrate that the OFR-induced decrease in calcium current is associated with a reduction in DHP binding sites. The decrease in calcium current and in calcium channels may be implicated in the mechanical dysfunction associated with oxidative stress.
Full text
PDF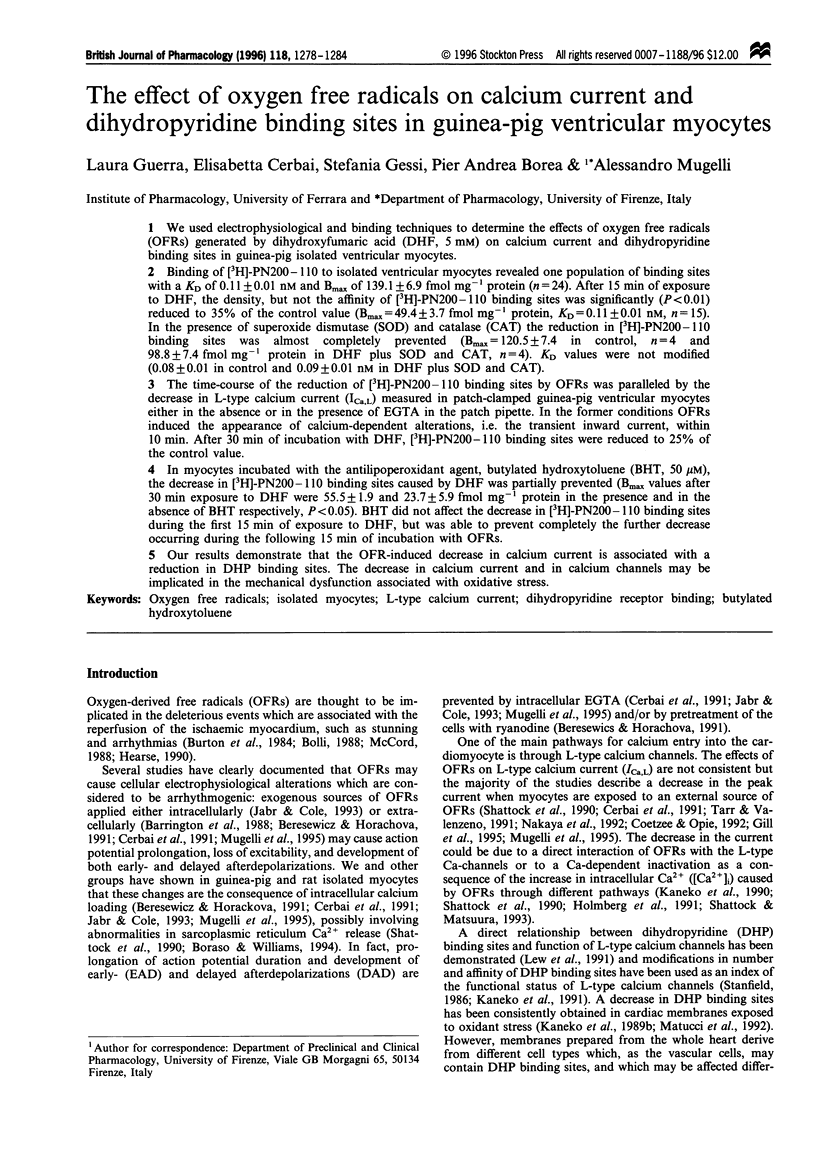
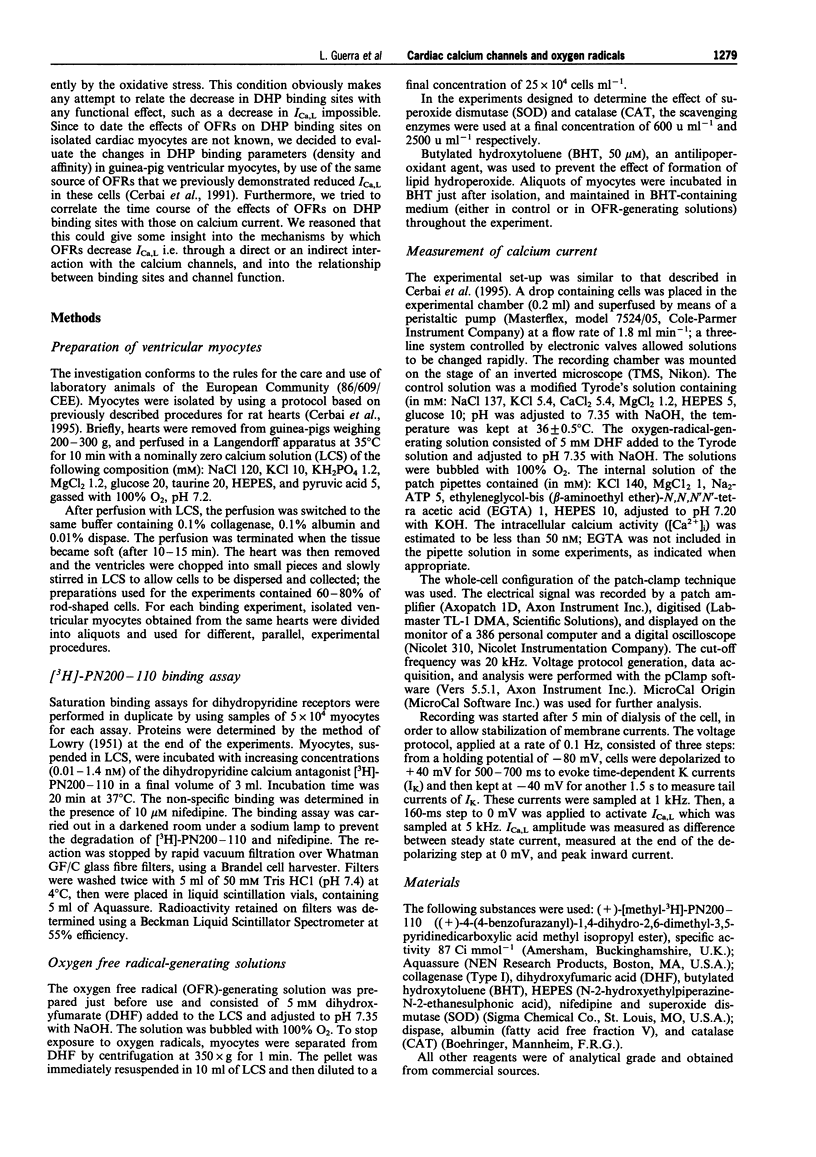
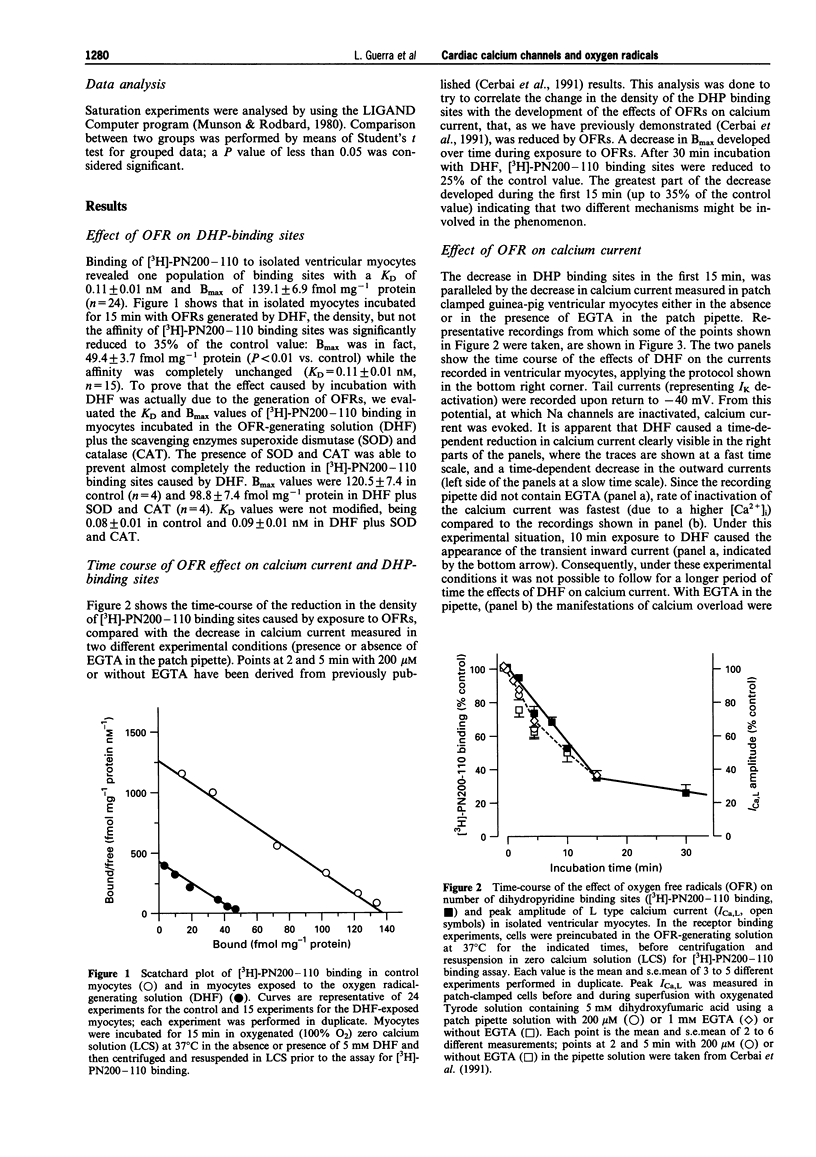
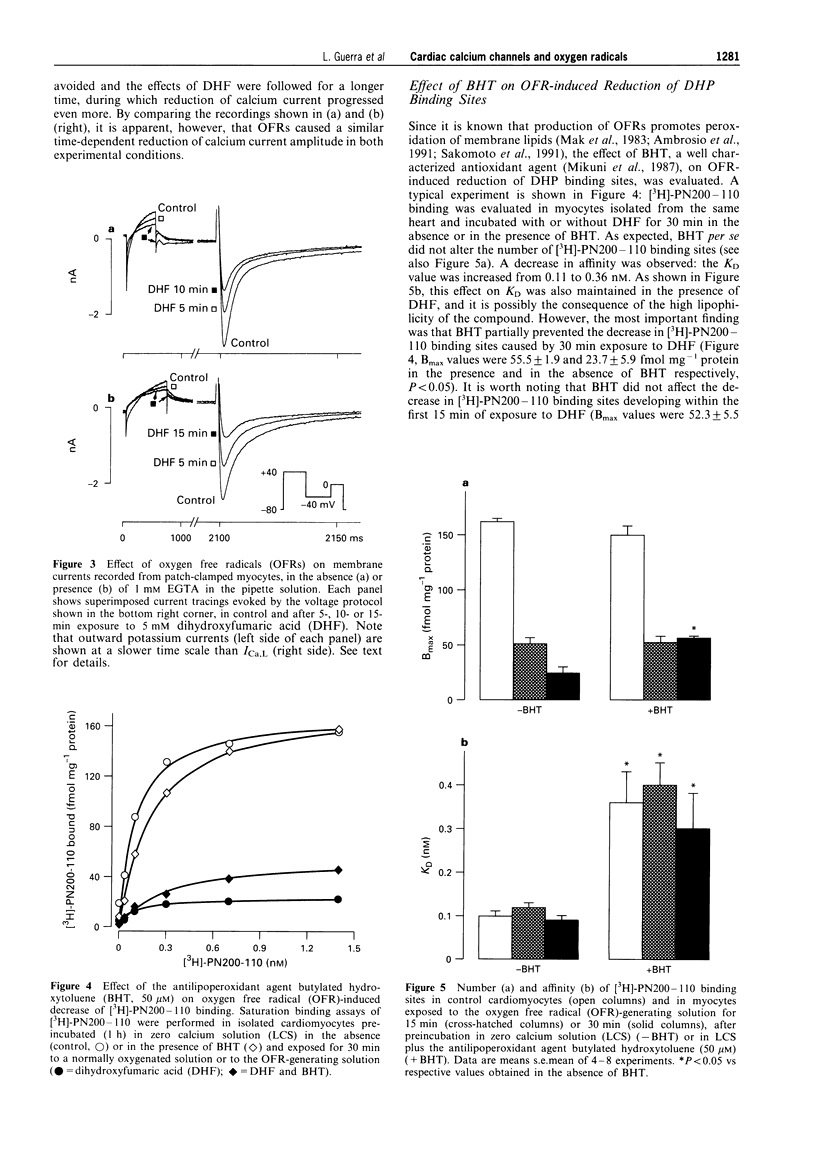
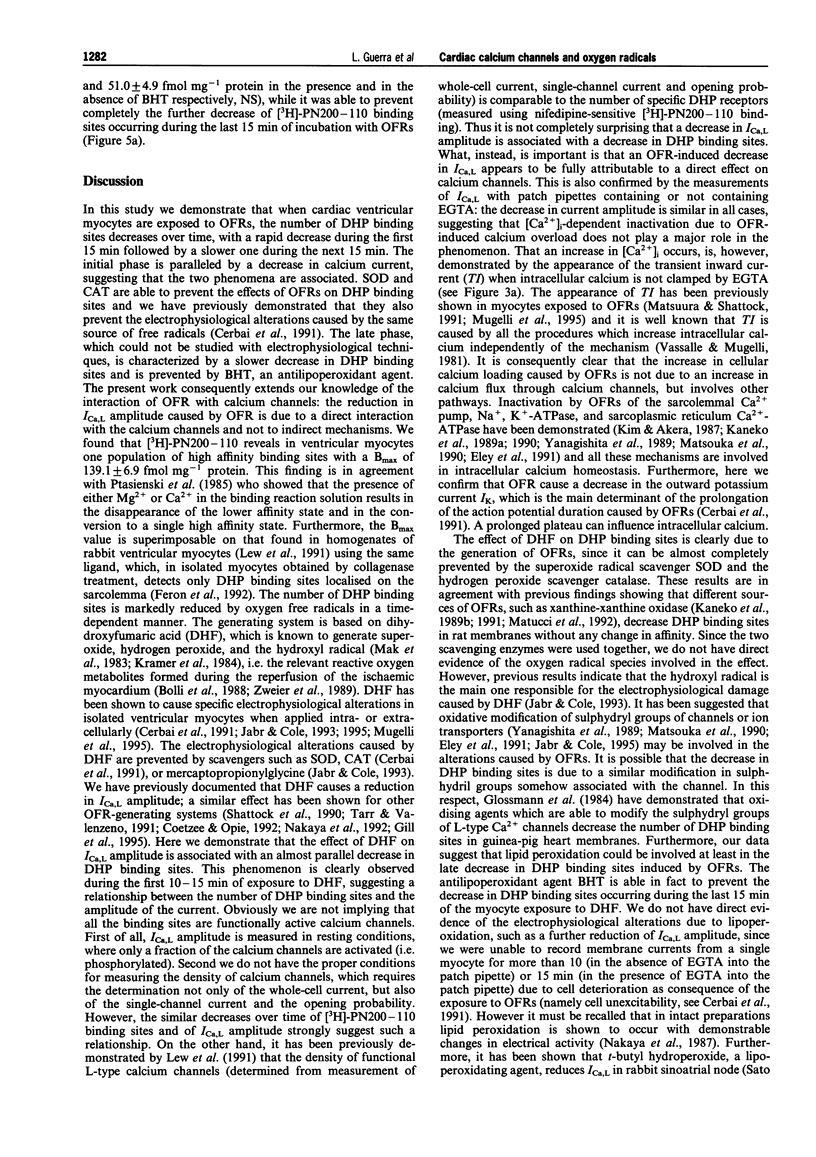
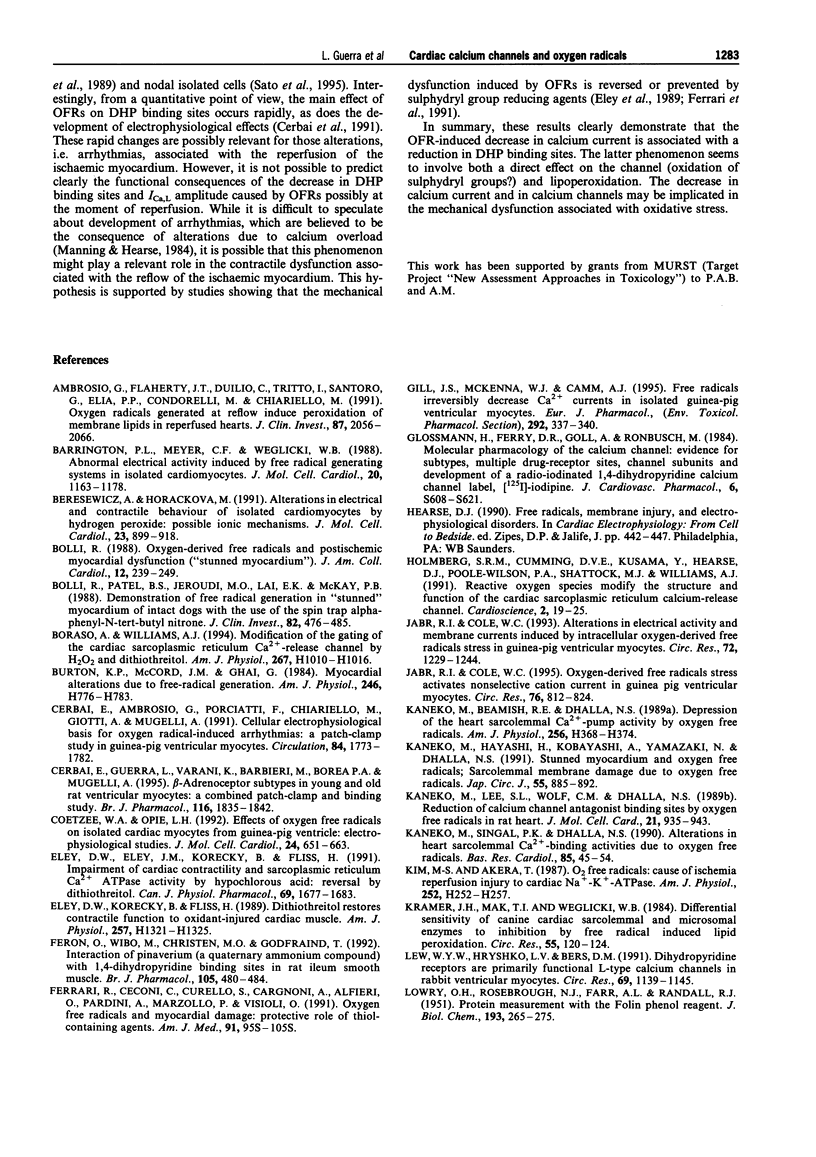
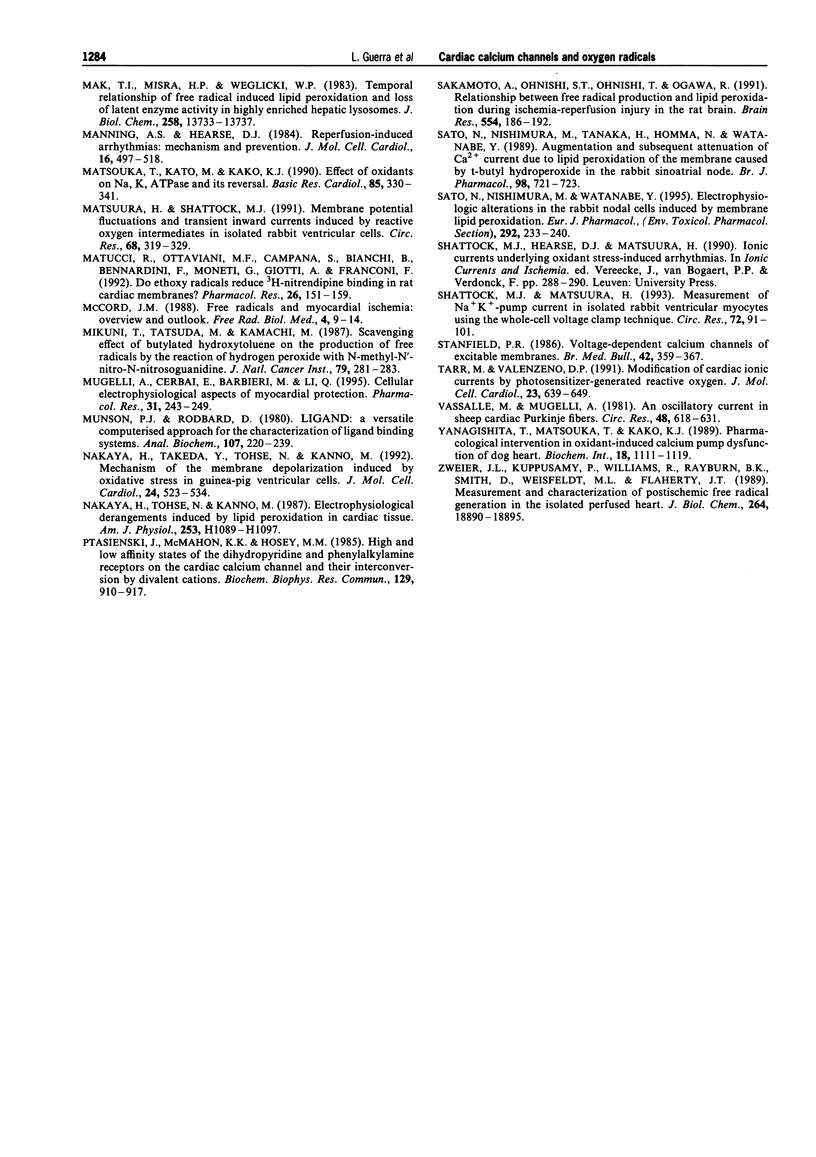
Selected References
These references are in PubMed. This may not be the complete list of references from this article.
- Ambrosio G., Flaherty J. T., Duilio C., Tritto I., Santoro G., Elia P. P., Condorelli M., Chiariello M. Oxygen radicals generated at reflow induce peroxidation of membrane lipids in reperfused hearts. J Clin Invest. 1991 Jun;87(6):2056–2066. doi: 10.1172/JCI115236. [DOI] [PMC free article] [PubMed] [Google Scholar]
- Barrington P. L., Meier C. F., Jr, Weglicki W. B. Abnormal electrical activity induced by free radical generating systems in isolated cardiocytes. J Mol Cell Cardiol. 1988 Dec;20(12):1163–1178. doi: 10.1016/0022-2828(88)90596-2. [DOI] [PubMed] [Google Scholar]
- Beresewicz A., Horackova M. Alterations in electrical and contractile behavior of isolated cardiomyocytes by hydrogen peroxide: possible ionic mechanisms. J Mol Cell Cardiol. 1991 Aug;23(8):899–918. doi: 10.1016/0022-2828(91)90133-7. [DOI] [PubMed] [Google Scholar]
- Bolli R. Oxygen-derived free radicals and postischemic myocardial dysfunction ("stunned myocardium"). J Am Coll Cardiol. 1988 Jul;12(1):239–249. doi: 10.1016/0735-1097(88)90381-6. [DOI] [PubMed] [Google Scholar]
- Bolli R., Patel B. S., Jeroudi M. O., Lai E. K., McCay P. B. Demonstration of free radical generation in "stunned" myocardium of intact dogs with the use of the spin trap alpha-phenyl N-tert-butyl nitrone. J Clin Invest. 1988 Aug;82(2):476–485. doi: 10.1172/JCI113621. [DOI] [PMC free article] [PubMed] [Google Scholar]
- Boraso A., Williams A. J. Modification of the gating of the cardiac sarcoplasmic reticulum Ca(2+)-release channel by H2O2 and dithiothreitol. Am J Physiol. 1994 Sep;267(3 Pt 2):H1010–H1016. doi: 10.1152/ajpheart.1994.267.3.H1010. [DOI] [PubMed] [Google Scholar]
- Burton K. P., McCord J. M., Ghai G. Myocardial alterations due to free-radical generation. Am J Physiol. 1984 Jun;246(6 Pt 2):H776–H783. doi: 10.1152/ajpheart.1984.246.6.H776. [DOI] [PubMed] [Google Scholar]
- Cerbai E., Ambrosio G., Porciatti F., Chiariello M., Giotti A., Mugelli A. Cellular electrophysiological basis for oxygen radical-induced arrhythmias. A patch-clamp study in guinea pig ventricular myocytes. Circulation. 1991 Oct;84(4):1773–1782. doi: 10.1161/01.cir.84.4.1773. [DOI] [PubMed] [Google Scholar]
- Cerbai E., Guerra L., Varani K., Barbieri M., Borea P. A., Mugelli A. Beta-adrenoceptor subtypes in young and old rat ventricular myocytes: a combined patch-clamp and binding study. Br J Pharmacol. 1995 Sep;116(2):1835–1842. doi: 10.1111/j.1476-5381.1995.tb16671.x. [DOI] [PMC free article] [PubMed] [Google Scholar]
- Coetzee W. A., Opie L. H. Effects of oxygen free radicals on isolated cardiac myocytes from guinea-pig ventricle: electrophysiological studies. J Mol Cell Cardiol. 1992 Jun;24(6):651–663. doi: 10.1016/0022-2828(92)91049-b. [DOI] [PubMed] [Google Scholar]
- Eley D. W., Eley J. M., Korecky B., Fliss H. Impairment of cardiac contractility and sarcoplasmic reticulum Ca2+ ATPase activity by hypochlorous acid: reversal by dithiothreitol. Can J Physiol Pharmacol. 1991 Nov;69(11):1677–1685. doi: 10.1139/y91-249. [DOI] [PubMed] [Google Scholar]
- Eley D. W., Korecky B., Fliss H. Dithiothreitol restores contractile function to oxidant-injured cardiac muscle. Am J Physiol. 1989 Oct;257(4 Pt 2):H1321–H1325. doi: 10.1152/ajpheart.1989.257.4.H1321. [DOI] [PubMed] [Google Scholar]
- Feron O., Wibo M., Christen M. O., Godfraind T. Interaction of pinaverium (a quaternary ammonium compound) with 1,4-dihydropyridine binding sites in rat ileum smooth muscle. Br J Pharmacol. 1992 Feb;105(2):480–484. doi: 10.1111/j.1476-5381.1992.tb14279.x. [DOI] [PMC free article] [PubMed] [Google Scholar]
- Ferrari R., Ceconi C., Curello S., Cargnoni A., Alfieri O., Pardini A., Marzollo P., Visioli O. Oxygen free radicals and myocardial damage: protective role of thiol-containing agents. Am J Med. 1991 Sep 30;91(3C):95S–105S. doi: 10.1016/0002-9343(91)90291-5. [DOI] [PubMed] [Google Scholar]
- Gill J. S., McKenna W. J., Camm A. J. Free radicals irreversibly decrease Ca2+ currents in isolated guinea-pig ventricular myocytes. Eur J Pharmacol. 1995 Mar 16;292(3-4):337–340. doi: 10.1016/0926-6917(95)90042-x. [DOI] [PubMed] [Google Scholar]
- Glossmann H., Ferry D. R., Goll A., Rombusch M. Molecular pharmacology of the calcium channel: evidence for subtypes, multiple drug-receptor sites, channel subunits, and the development of a radioiodinated 1,4-dihydropyridine calcium channel label, [125I]iodipine. J Cardiovasc Pharmacol. 1984;6 (Suppl 4):S608–S621. [PubMed] [Google Scholar]
- Holmberg S. R., Cumming D. V., Kusama Y., Hearse D. J., Poole-Wilson P. A., Shattock M. J., Williams A. J. Reactive oxygen species modify the structure and function of the cardiac sarcoplasmic reticulum calcium-release channel. Cardioscience. 1991 Mar;2(1):19–25. [PubMed] [Google Scholar]
- Jabr R. I., Cole W. C. Alterations in electrical activity and membrane currents induced by intracellular oxygen-derived free radical stress in guinea pig ventricular myocytes. Circ Res. 1993 Jun;72(6):1229–1244. doi: 10.1161/01.res.72.6.1229. [DOI] [PubMed] [Google Scholar]
- Jabr R. I., Cole W. C. Oxygen-derived free radical stress activates nonselective cation current in guinea pig ventricular myocytes. Role of sulfhydryl groups. Circ Res. 1995 May;76(5):812–824. doi: 10.1161/01.res.76.5.812. [DOI] [PubMed] [Google Scholar]
- Kaneko M., Beamish R. E., Dhalla N. S. Depression of heart sarcolemmal Ca2+-pump activity by oxygen free radicals. Am J Physiol. 1989 Feb;256(2 Pt 2):H368–H374. doi: 10.1152/ajpheart.1989.256.2.H368. [DOI] [PubMed] [Google Scholar]
- Kaneko M., Hayashi H., Kobayashi A., Yamazaki N., Dhalla N. S. Stunned myocardium and oxygen free radicals--sarcolemmal membrane damage due to oxygen free radicals. Jpn Circ J. 1991 Sep;55(9):885–892. doi: 10.1253/jcj.55.885. [DOI] [PubMed] [Google Scholar]
- Kaneko M., Lee S. L., Wolf C. M., Dhalla N. S. Reduction of calcium channel antagonist binding sites by oxygen free radicals in rat heart. J Mol Cell Cardiol. 1989 Sep;21(9):935–943. doi: 10.1016/0022-2828(89)90761-x. [DOI] [PubMed] [Google Scholar]
- Kaneko M., Singal P. K., Dhalla N. S. Alterations in heart sarcolemmal Ca2(+)-ATPase and Ca2(+)-binding activities due to oxygen free radicals. Basic Res Cardiol. 1990 Jan-Feb;85(1):45–54. doi: 10.1007/BF01907013. [DOI] [PubMed] [Google Scholar]
- Kim M. S., Akera T. O2 free radicals: cause of ischemia-reperfusion injury to cardiac Na+-K+-ATPase. Am J Physiol. 1987 Feb;252(2 Pt 2):H252–H257. doi: 10.1152/ajpheart.1987.252.2.H252. [DOI] [PubMed] [Google Scholar]
- Kramer J. H., Mak I. T., Weglicki W. B. Differential sensitivity of canine cardiac sarcolemmal and microsomal enzymes to inhibition by free radical-induced lipid peroxidation. Circ Res. 1984 Jul;55(1):120–124. doi: 10.1161/01.res.55.1.120. [DOI] [PubMed] [Google Scholar]
- LOWRY O. H., ROSEBROUGH N. J., FARR A. L., RANDALL R. J. Protein measurement with the Folin phenol reagent. J Biol Chem. 1951 Nov;193(1):265–275. [PubMed] [Google Scholar]
- Lew W. Y., Hryshko L. V., Bers D. M. Dihydropyridine receptors are primarily functional L-type calcium channels in rabbit ventricular myocytes. Circ Res. 1991 Oct;69(4):1139–1145. doi: 10.1161/01.res.69.4.1139. [DOI] [PubMed] [Google Scholar]
- Mak I. T., Misra H. P., Weglicki W. B. Temporal relationship of free radical-induced lipid peroxidation and loss of latent enzyme activity in highly enriched hepatic lysosomes. J Biol Chem. 1983 Nov 25;258(22):13733–13737. [PubMed] [Google Scholar]
- Manning A. S., Hearse D. J. Reperfusion-induced arrhythmias: mechanisms and prevention. J Mol Cell Cardiol. 1984 Jun;16(6):497–518. doi: 10.1016/s0022-2828(84)80638-0. [DOI] [PubMed] [Google Scholar]
- Matsuoka T., Kato M., Kako K. J. Effect of oxidants on Na,K,ATPase and its reversal. Basic Res Cardiol. 1990 Jul-Aug;85(4):330–341. doi: 10.1007/BF01907126. [DOI] [PubMed] [Google Scholar]
- Matsuura H., Shattock M. J. Membrane potential fluctuations and transient inward currents induced by reactive oxygen intermediates in isolated rabbit ventricular cells. Circ Res. 1991 Feb;68(2):319–329. doi: 10.1161/01.res.68.2.319. [DOI] [PubMed] [Google Scholar]
- Matucci R., Ottaviani M. F., Campana S., Bianchi B., Bennardini F., Moneti G., Giotti A., Franconi F. Do ethoxy radicals reduce 3H-nitrendipine binding in rat cardiac membranes? Pharmacol Res. 1992 Sep;26(2):151–159. doi: 10.1016/s1043-6618(05)80128-8. [DOI] [PubMed] [Google Scholar]
- McCord J. M. Free radicals and myocardial ischemia: overview and outlook. Free Radic Biol Med. 1988;4(1):9–14. doi: 10.1016/0891-5849(88)90005-6. [DOI] [PubMed] [Google Scholar]
- Mikuni T., Tatsuta M., Kamachi M. Scavenging effect of butylated hydroxytoluene on the production of free radicals by the reaction of hydrogen peroxide with N-methyl-N'-nitro-N-nitrosoguanidine. J Natl Cancer Inst. 1987 Aug;79(2):281–283. [PubMed] [Google Scholar]
- Mugelli A., Cerbai E., Barbieri M., Li Q. Cellular electrophysiological aspects of myocardial protection. Pharmacol Res. 1995 Mar-Apr;31(3-4):243–249. doi: 10.1016/1043-6618(95)80025-5. [DOI] [PubMed] [Google Scholar]
- Munson P. J., Rodbard D. Ligand: a versatile computerized approach for characterization of ligand-binding systems. Anal Biochem. 1980 Sep 1;107(1):220–239. doi: 10.1016/0003-2697(80)90515-1. [DOI] [PubMed] [Google Scholar]
- Nakaya H., Takeda Y., Tohse N., Kanno M. Mechanism of the membrane depolarization induced by oxidative stress in guinea-pig ventricular cells. J Mol Cell Cardiol. 1992 May;24(5):523–534. doi: 10.1016/0022-2828(92)91841-r. [DOI] [PubMed] [Google Scholar]
- Nakaya H., Tohse N., Kanno M. Electrophysiological derangements induced by lipid peroxidation in cardiac tissue. Am J Physiol. 1987 Nov;253(5 Pt 2):H1089–H1097. doi: 10.1152/ajpheart.1987.253.5.H1089. [DOI] [PubMed] [Google Scholar]
- Ptasienski J., McMahon K. K., Hosey M. M. High and low affinity states of the dihydropyridine and phenylalkylamine receptors on the cardiac calcium channel and their interconversion by divalent cations. Biochem Biophys Res Commun. 1985 Jun 28;129(3):910–917. doi: 10.1016/0006-291x(85)91978-3. [DOI] [PubMed] [Google Scholar]
- Sakamoto A., Ohnishi S. T., Ohnishi T., Ogawa R. Relationship between free radical production and lipid peroxidation during ischemia-reperfusion injury in the rat brain. Brain Res. 1991 Jul 19;554(1-2):186–192. doi: 10.1016/0006-8993(91)90187-z. [DOI] [PubMed] [Google Scholar]
- Sato N., Nishimura M., Tanaka H., Homma N., Watanabe Y. Augmentation and subsequent attenuation of Ca2+ current due to lipid peroxidation of the membrane caused by t-butyl hydroperoxide in the rabbit sinoatrial node. Br J Pharmacol. 1989 Nov;98(3):721–723. doi: 10.1111/j.1476-5381.1989.tb14598.x. [DOI] [PMC free article] [PubMed] [Google Scholar]
- Satoh N., Nishimura M., Watanabe Y. Electrophysiologic alterations in the rabbit nodal cells induced by membrane lipid peroxidation. Eur J Pharmacol. 1995 Mar 16;292(3-4):233–240. doi: 10.1016/0926-6917(95)90027-6. [DOI] [PubMed] [Google Scholar]
- Shattock M. J., Matsuura H. Measurement of Na(+)-K+ pump current in isolated rabbit ventricular myocytes using the whole-cell voltage-clamp technique. Inhibition of the pump by oxidant stress. Circ Res. 1993 Jan;72(1):91–101. doi: 10.1161/01.res.72.1.91. [DOI] [PubMed] [Google Scholar]
- Stanfield P. R. Voltage-dependent calcium channels of excitable membranes. Br Med Bull. 1986 Oct;42(4):359–367. doi: 10.1093/oxfordjournals.bmb.a072153. [DOI] [PubMed] [Google Scholar]
- Tarr M., Valenzeno D. P. Modification of cardiac ionic currents by photosensitizer-generated reactive oxygen. J Mol Cell Cardiol. 1991 May;23(5):639–649. doi: 10.1016/0022-2828(91)90055-q. [DOI] [PubMed] [Google Scholar]
- Vassalle M., Mugelli A. An oscillatory current in sheep cardiac Purkinje fibers. Circ Res. 1981 May;48(5):618–631. doi: 10.1161/01.res.48.5.618. [DOI] [PubMed] [Google Scholar]
- Yanagishita T., Matsuoka T., Kako K. J. Pharmacological intervention in oxidant-induced calcium pump dysfunction of dog heart. Biochem Int. 1989 Jun;18(6):1111–1119. [PubMed] [Google Scholar]
- Zweier J. L., Kuppusamy P., Williams R., Rayburn B. K., Smith D., Weisfeldt M. L., Flaherty J. T. Measurement and characterization of postischemic free radical generation in the isolated perfused heart. J Biol Chem. 1989 Nov 15;264(32):18890–18895. [PubMed] [Google Scholar]