Abstract
The level of LamB protein in the outer membrane of Escherichia coli was derepressed in the absence of a known inducer (maltodextrins) under carbohydrate-limiting conditions in chemostats. LamB protein contributed to the ability of the bacteria to remove sugar from glucose-limited chemostats, and well-characterized lamB mutants with reduced stability constants for glucose were less growth competitive under glucose limitation than those with wild-type affinity. In turn, wild-type bacteria were less growth competitive than lamB mutants with enhanced sugar affinity. In contrast to an earlier report, we found that LamB- bacteria were less able to compete in carbohydrate-limited chemostats (with glucose, lactose, arabinose, or glycerol as the carbon and energy sources) when mixed with LamB+ bacteria. The transport Km for [14C]glucose was affected by the presence or affinity of LamB, but only in chemostat-grown bacteria, with their elevated LamB levels. The pattern of expression of LamB and the advantage it confers for growth on low concentrations of carbohydrates are consistent with a wider role in sugar permeation than simply maltosaccharide transport, and hence the well-known maltoporin activity of LamB is but one facet of its role as the general glycoporin of E. coli. A corollary of these findings is that OmpF/OmpC porins, present at high levels in carbon-limited bacteria, do not provide sufficient permeability to sugars or even glycerol to support high growth rates at low concentrations. Hence, the sugar-binding site of LamB protein is an important contributor to the permeability of the outer membrane to carbohydrates in habitats with low extracellular nutrient concentrations.
Full text
PDF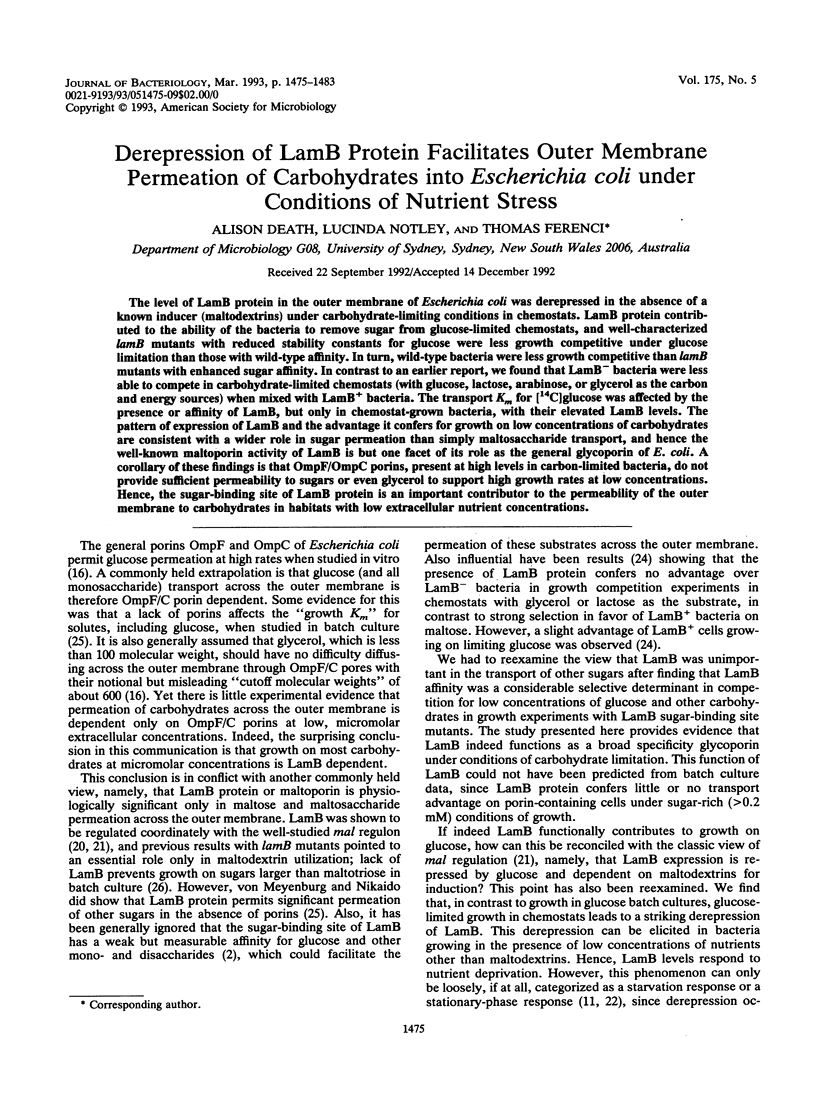
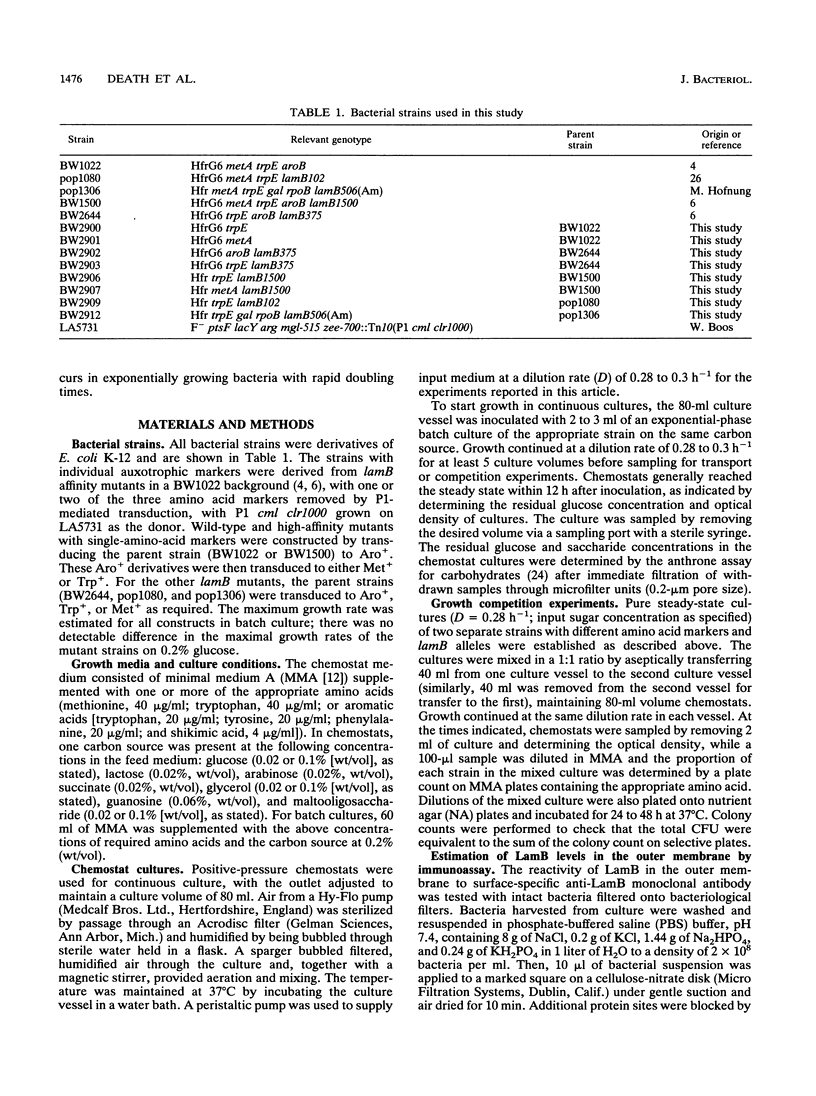
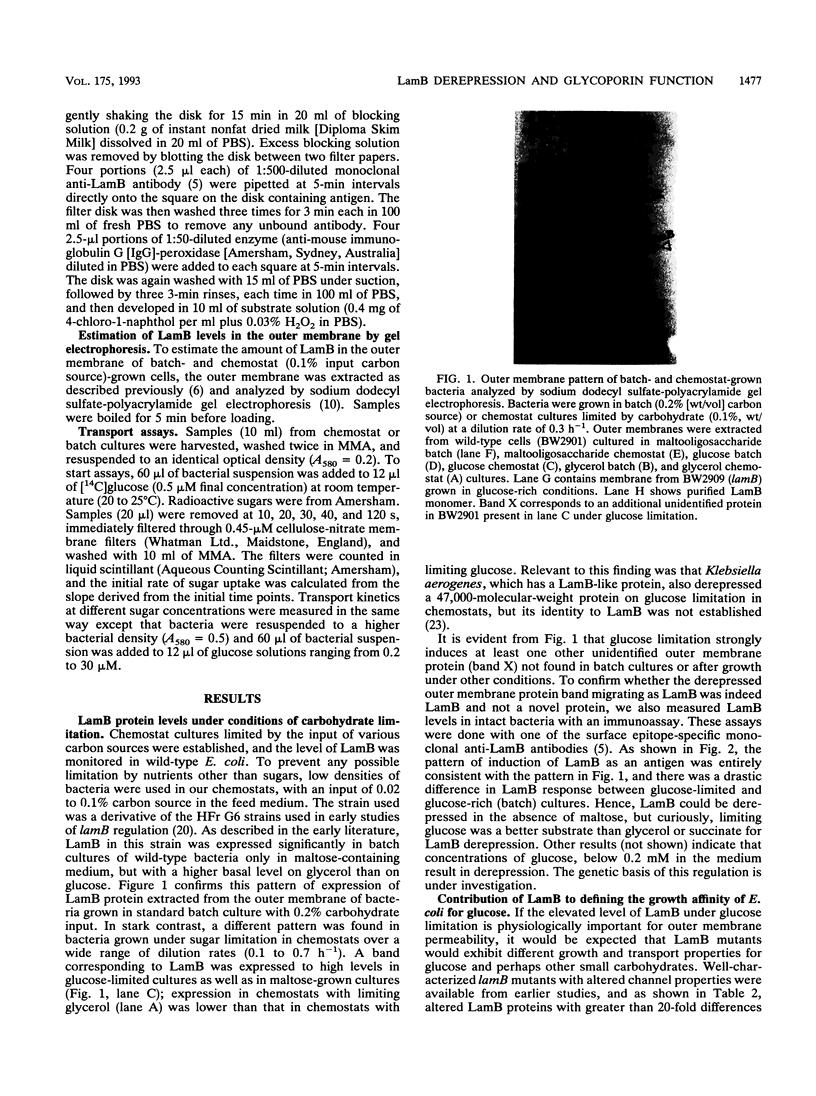
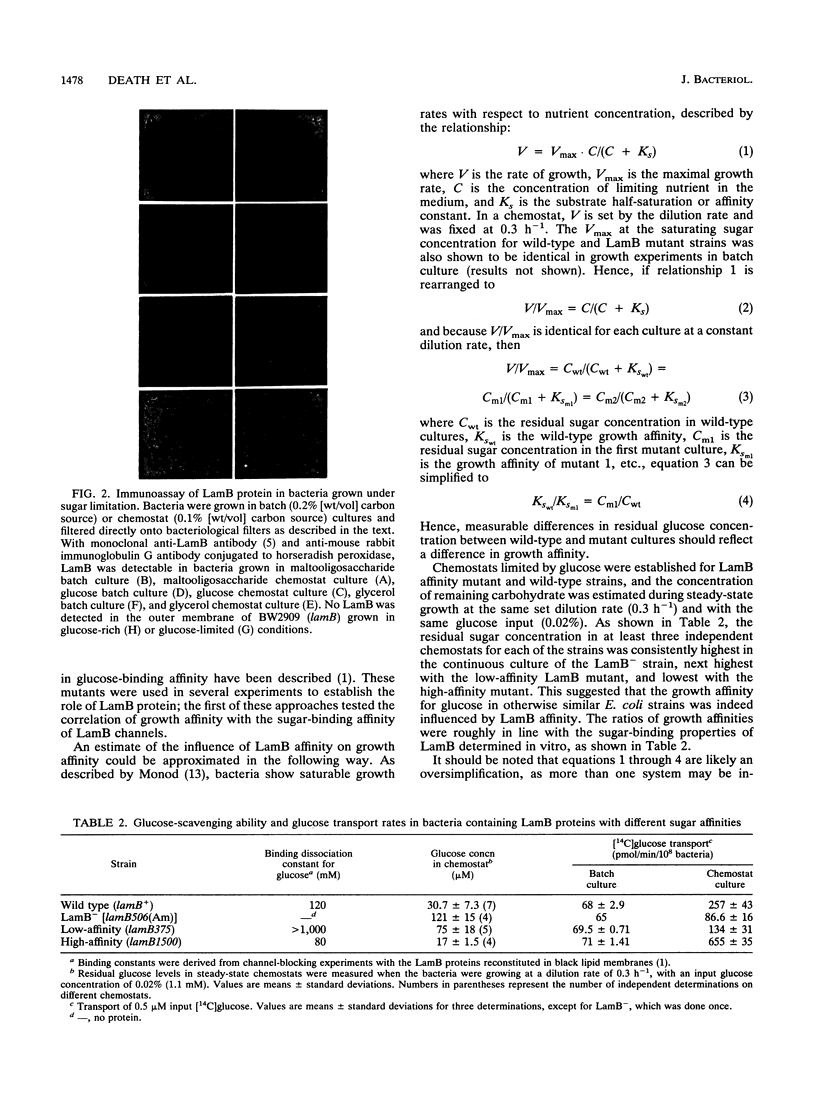
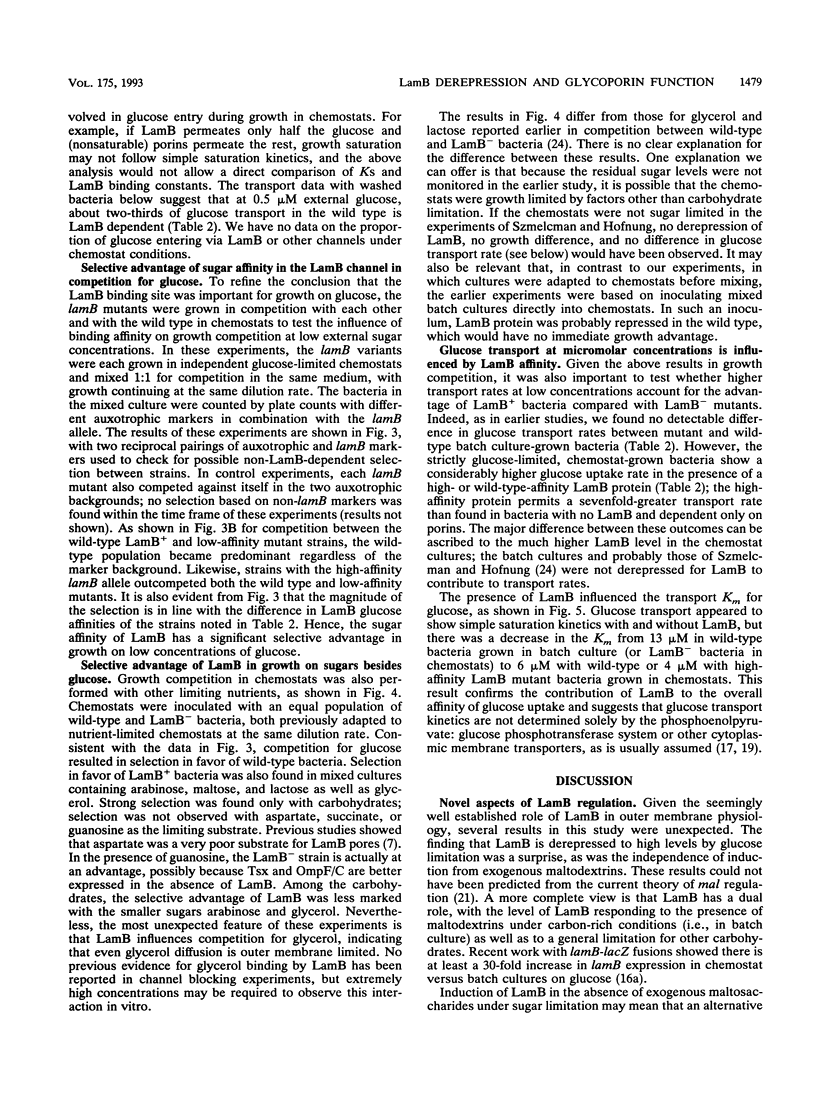
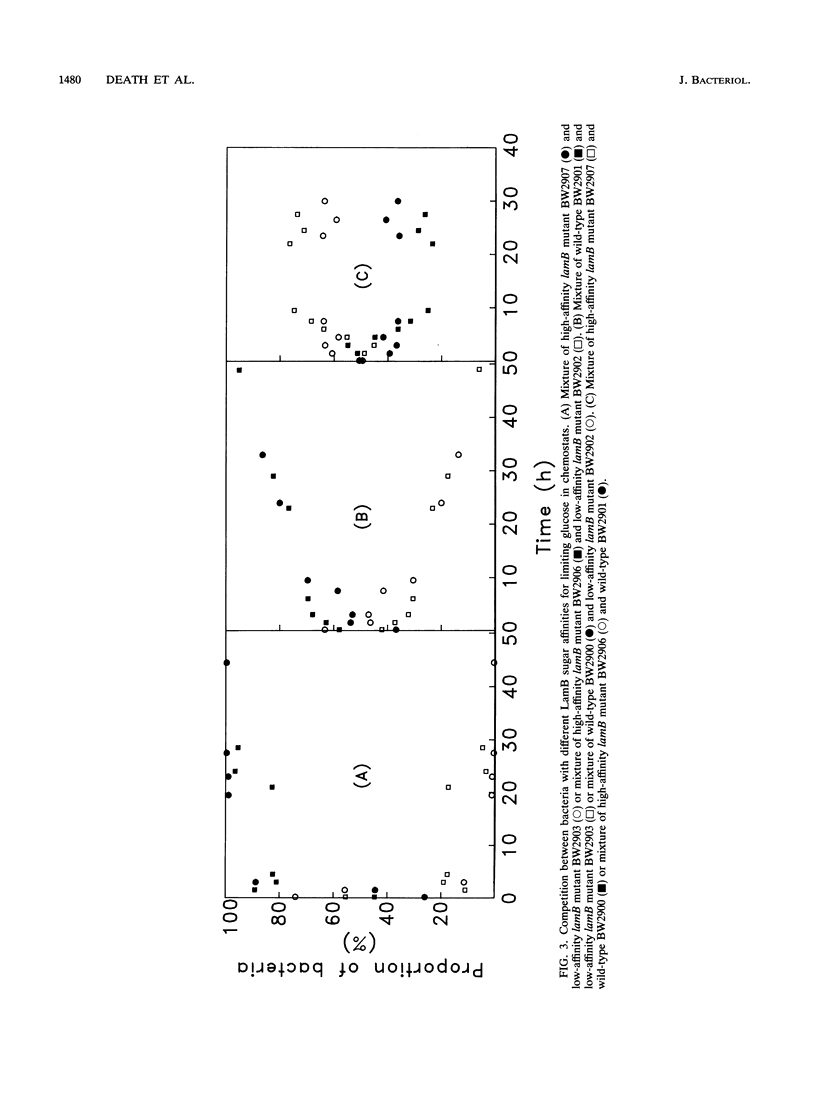
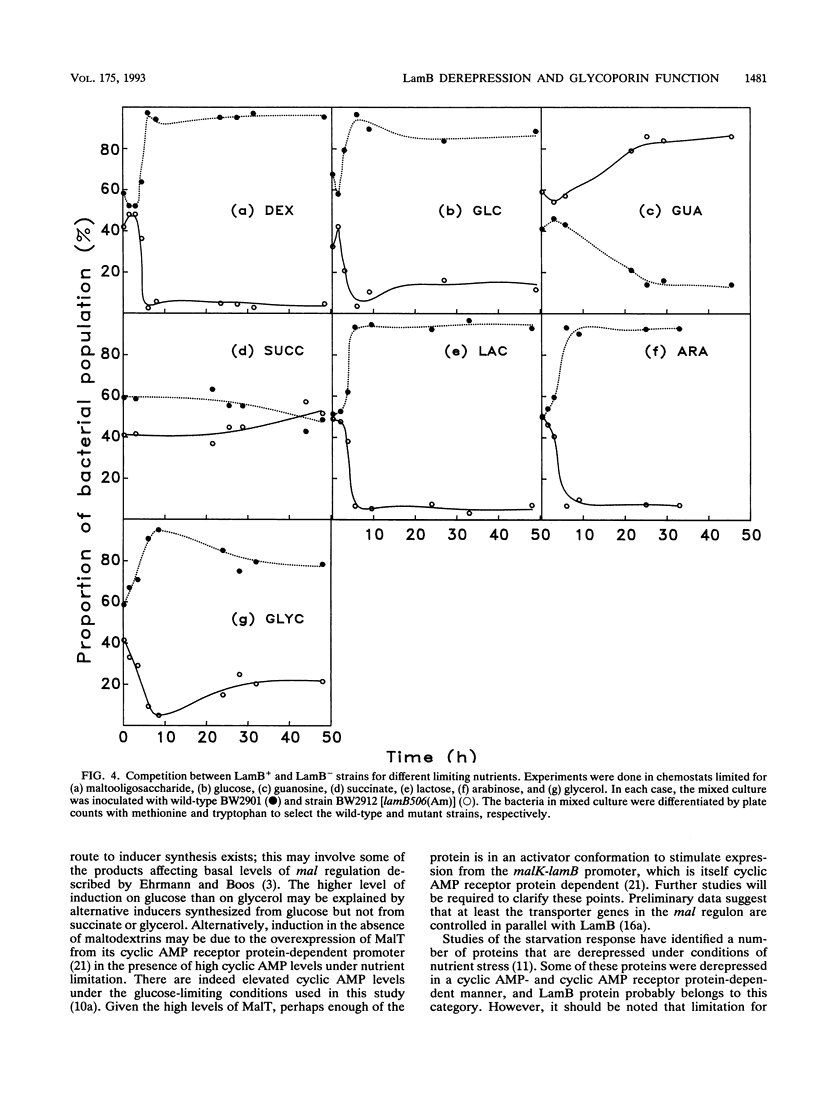
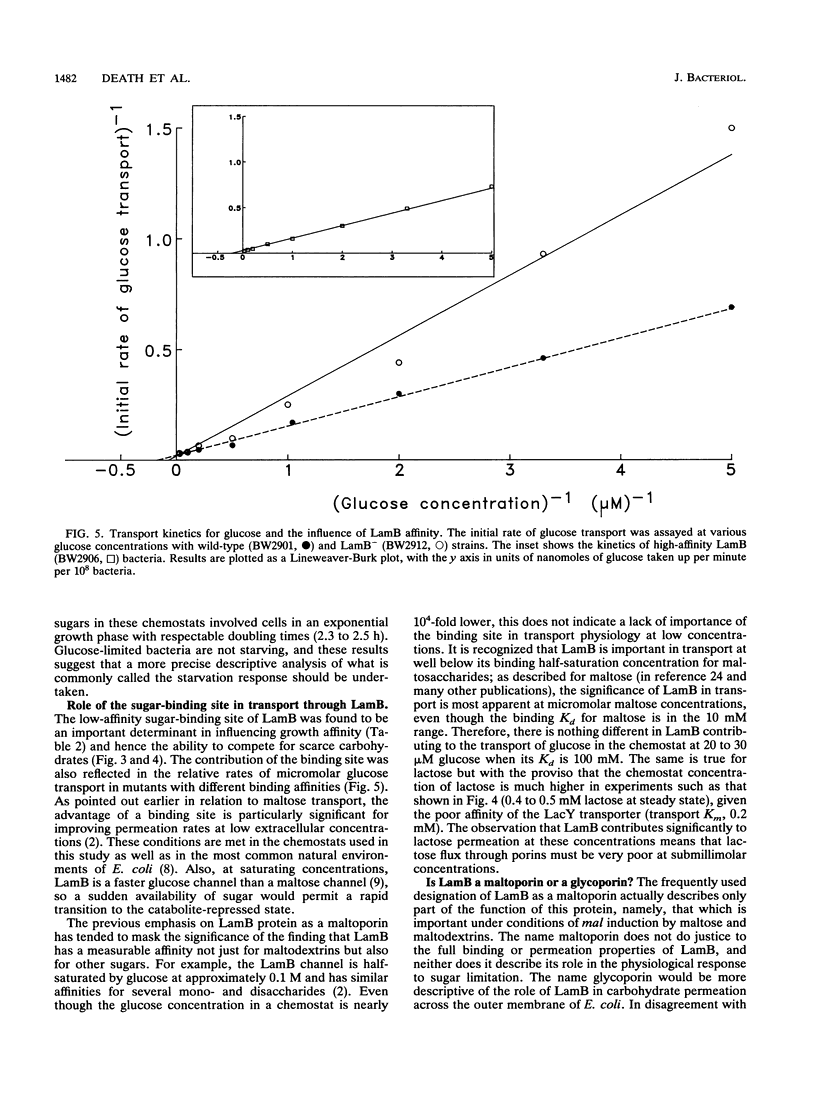
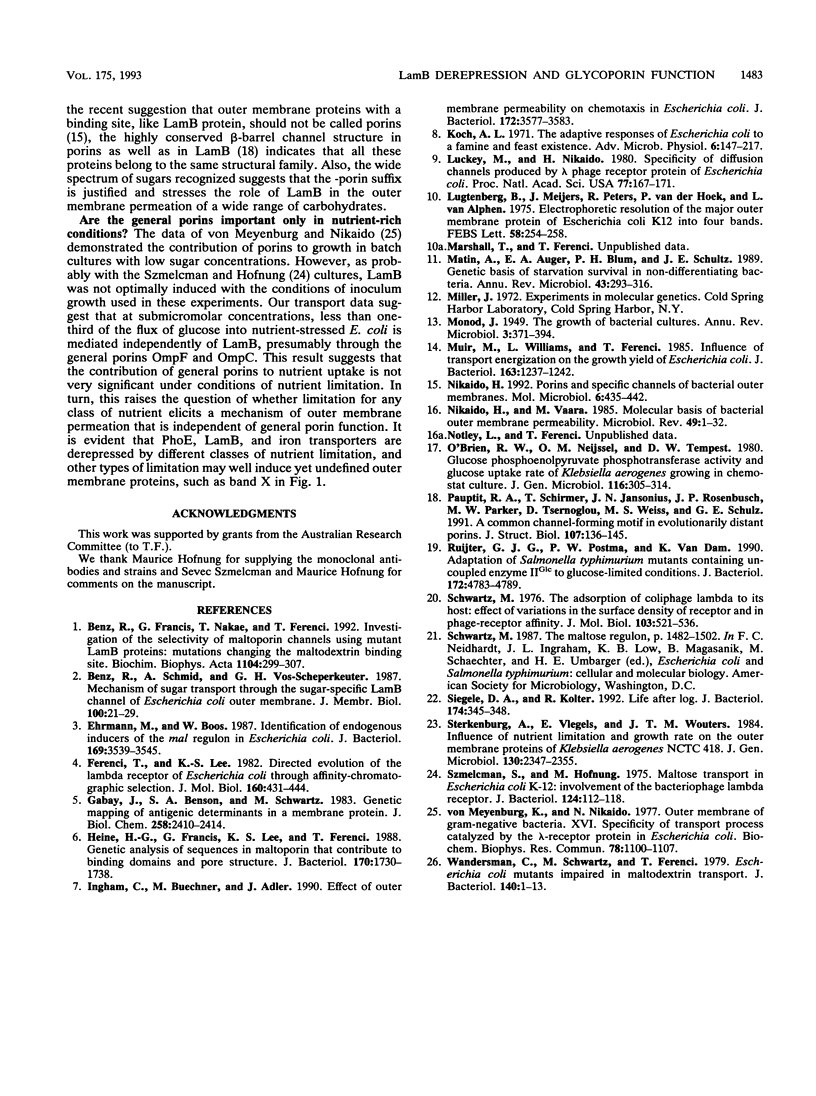
Images in this article
Selected References
These references are in PubMed. This may not be the complete list of references from this article.
- Benz R., Francis G., Nakae T., Ferenci T. Investigation of the selectivity of maltoporin channels using mutant LamB proteins: mutations changing the maltodextrin binding site. Biochim Biophys Acta. 1992 Mar 2;1104(2):299–307. doi: 10.1016/0005-2736(92)90044-m. [DOI] [PubMed] [Google Scholar]
- Benz R., Schmid A., Vos-Scheperkeuter G. H. Mechanism of sugar transport through the sugar-specific LamB channel of Escherichia coli outer membrane. J Membr Biol. 1987;100(1):21–29. doi: 10.1007/BF02209137. [DOI] [PubMed] [Google Scholar]
- Ehrmann M., Boos W. Identification of endogenous inducers of the mal regulon in Escherichia coli. J Bacteriol. 1987 Aug;169(8):3539–3545. doi: 10.1128/jb.169.8.3539-3545.1987. [DOI] [PMC free article] [PubMed] [Google Scholar]
- Ferenci T., Lee K. S. Directed evolution of the lambda receptor of Escherichia coli through affinity chromatographic selection. J Mol Biol. 1982 Sep 25;160(3):431–444. doi: 10.1016/0022-2836(82)90306-0. [DOI] [PubMed] [Google Scholar]
- Gabay J., Benson S., Schwartz M. Genetic mapping of antigenic determinants on a membrane protein. J Biol Chem. 1983 Feb 25;258(4):2410–2414. [PubMed] [Google Scholar]
- Heine H. G., Francis G., Lee K. S., Ferenci T. Genetic analysis of sequences in maltoporin that contribute to binding domains and pore structure. J Bacteriol. 1988 Apr;170(4):1730–1738. doi: 10.1128/jb.170.4.1730-1738.1988. [DOI] [PMC free article] [PubMed] [Google Scholar]
- Ingham C., Buechner M., Adler J. Effect of outer membrane permeability on chemotaxis in Escherichia coli. J Bacteriol. 1990 Jul;172(7):3577–3583. doi: 10.1128/jb.172.7.3577-3583.1990. [DOI] [PMC free article] [PubMed] [Google Scholar]
- Koch A. L. The adaptive responses of Escherichia coli to a feast and famine existence. Adv Microb Physiol. 1971;6:147–217. doi: 10.1016/s0065-2911(08)60069-7. [DOI] [PubMed] [Google Scholar]
- Luckey M., Nikaido H. Specificity of diffusion channels produced by lambda phage receptor protein of Escherichia coli. Proc Natl Acad Sci U S A. 1980 Jan;77(1):167–171. doi: 10.1073/pnas.77.1.167. [DOI] [PMC free article] [PubMed] [Google Scholar]
- Lugtenberg B., Meijers J., Peters R., van der Hoek P., van Alphen L. Electrophoretic resolution of the "major outer membrane protein" of Escherichia coli K12 into four bands. FEBS Lett. 1975 Oct 15;58(1):254–258. doi: 10.1016/0014-5793(75)80272-9. [DOI] [PubMed] [Google Scholar]
- Matin A., Auger E. A., Blum P. H., Schultz J. E. Genetic basis of starvation survival in nondifferentiating bacteria. Annu Rev Microbiol. 1989;43:293–316. doi: 10.1146/annurev.mi.43.100189.001453. [DOI] [PubMed] [Google Scholar]
- Muir M., Williams L., Ferenci T. Influence of transport energization on the growth yield of Escherichia coli. J Bacteriol. 1985 Sep;163(3):1237–1242. doi: 10.1128/jb.163.3.1237-1242.1985. [DOI] [PMC free article] [PubMed] [Google Scholar]
- Nikaido H. Porins and specific channels of bacterial outer membranes. Mol Microbiol. 1992 Feb;6(4):435–442. doi: 10.1111/j.1365-2958.1992.tb01487.x. [DOI] [PubMed] [Google Scholar]
- Nikaido H., Vaara M. Molecular basis of bacterial outer membrane permeability. Microbiol Rev. 1985 Mar;49(1):1–32. doi: 10.1128/mr.49.1.1-32.1985. [DOI] [PMC free article] [PubMed] [Google Scholar]
- O'Brien R. W., Neijssel O. M., Tempest D. W. Glucose phosphoenolpyruvate phosphotransferase activity and glucose uptake rate of Klebsiella aerogenes growing in chemostat culture. J Gen Microbiol. 1980 Feb;116(2):305–314. doi: 10.1099/00221287-116-2-305. [DOI] [PubMed] [Google Scholar]
- Pauptit R. A., Schirmer T., Jansonius J. N., Rosenbusch J. P., Parker M. W., Tucker A. D., Tsernoglou D., Weiss M. S., Schultz G. E. A common channel-forming motif in evolutionarily distant porins. J Struct Biol. 1991 Oct;107(2):136–145. doi: 10.1016/1047-8477(91)90017-q. [DOI] [PubMed] [Google Scholar]
- Ruijter G. J., Postma P. W., van Dam K. Adaptation of Salmonella typhimurium mutants containing uncoupled enzyme IIGlc to glucose-limited conditions. J Bacteriol. 1990 Sep;172(9):4783–4789. doi: 10.1128/jb.172.9.4783-4789.1990. [DOI] [PMC free article] [PubMed] [Google Scholar]
- Schwartz M. The adsorption of coliphage lambda to its host: effect of variations in the surface density of receptor and in phage-receptor affinity. J Mol Biol. 1976 May 25;103(3):521–536. doi: 10.1016/0022-2836(76)90215-1. [DOI] [PubMed] [Google Scholar]
- Siegele D. A., Kolter R. Life after log. J Bacteriol. 1992 Jan;174(2):345–348. doi: 10.1128/jb.174.2.345-348.1992. [DOI] [PMC free article] [PubMed] [Google Scholar]
- Sterkenburg A., Vlegels E., Wouters J. T. Influence of nutrient limitation and growth rate on the outer membrane proteins of Klebsiella aerogenes NCTC 418. J Gen Microbiol. 1984 Sep;130(9):2347–2355. doi: 10.1099/00221287-130-9-2347. [DOI] [PubMed] [Google Scholar]
- Szmelcman S., Hofnung M. Maltose transport in Escherichia coli K-12: involvement of the bacteriophage lambda receptor. J Bacteriol. 1975 Oct;124(1):112–118. doi: 10.1128/jb.124.1.112-118.1975. [DOI] [PMC free article] [PubMed] [Google Scholar]
- Wandersman C., Schwartz M., Ferenci T. Escherichia coli mutants impaired in maltodextrin transport. J Bacteriol. 1979 Oct;140(1):1–13. doi: 10.1128/jb.140.1.1-13.1979. [DOI] [PMC free article] [PubMed] [Google Scholar]
- von Meyenburg K., Nikaido H. Outer membrane of gram-negative bacteria. XVII. Secificity of transport process catalyzed by the lambda-receptor protein in Escherichia coli. Biochem Biophys Res Commun. 1977 Oct 10;78(3):1100–1107. doi: 10.1016/0006-291x(77)90534-4. [DOI] [PubMed] [Google Scholar]