Abstract
The degree and temporal context of variations in ribosome content during nutrient starvation of two copiotrophic marine bacteria, Vibrio alginolyticus and Vibrio furnissii, have been examined. The organisms were starved either by nutritional shift-down or by consumption of limiting nutrients resulting from growth into stationary phase. Measurements of the amount of hybridization to 16S rRNA-specific probes revealed that the cells retained between 10 and 26% of their original rRNA content after 15 days of starvation. In V. alginolyticus, losses in stationary-phase cells occurred rapidly (1 to 2 days), whereas cells shifted into starvation remained larger and retained considerably more rRNA. The ability of V. alginolyticus to recover from starvation was assessed after cells were maintained for 2, 8, and 15 days in nutrient-depleted medium. The pattern of recovery at the level of rRNA accumulation depended upon the duration of nutrient deprivation and the manner in which it was imposed. Stationary-phase cells starved for 2 days had only slight relative increases in rRNA levels after excess nutrients were added. As the duration of starvation lengthened to 8 and 15 days, increasingly greater amounts of rRNA (30 and 70 times preenrichment values, respectively) were transcribed after nutrient enrichment. Shift-down cells recovered from 2 and 8 days of starvation without extensive rRNA production. After 15 days, nutrient enrichment caused 16S rRNA levels to increase 30-fold. The results indicate that the mechanisms controlling starvation-survival in these marine bacterial species are linked to the physiological state at the onset of starvation and that the subsequent pattern of recovery will depend upon how starvation was initiated.
Full text
PDF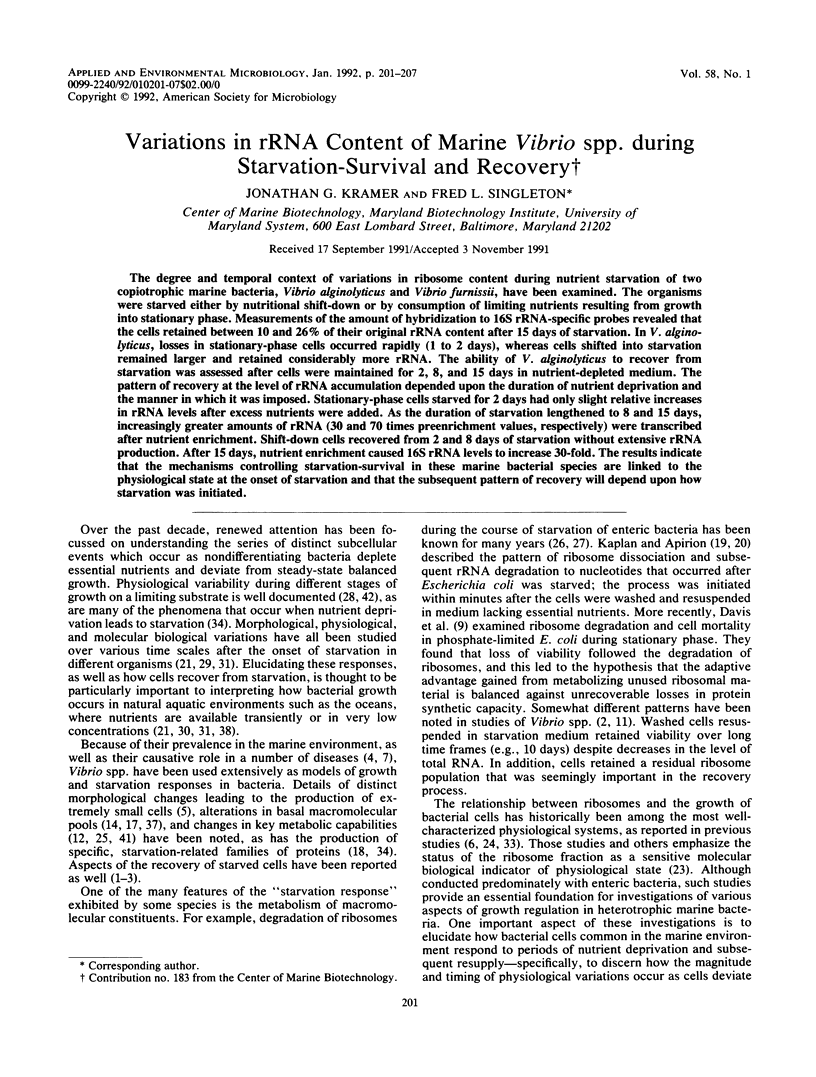
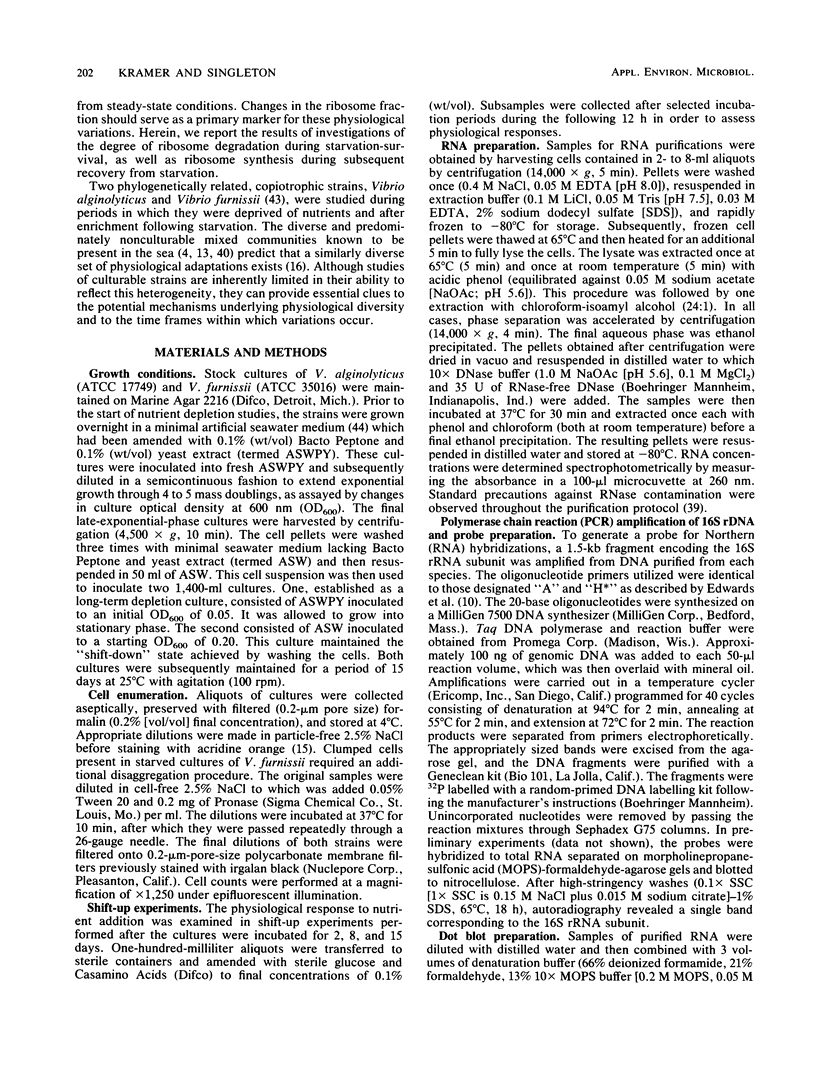
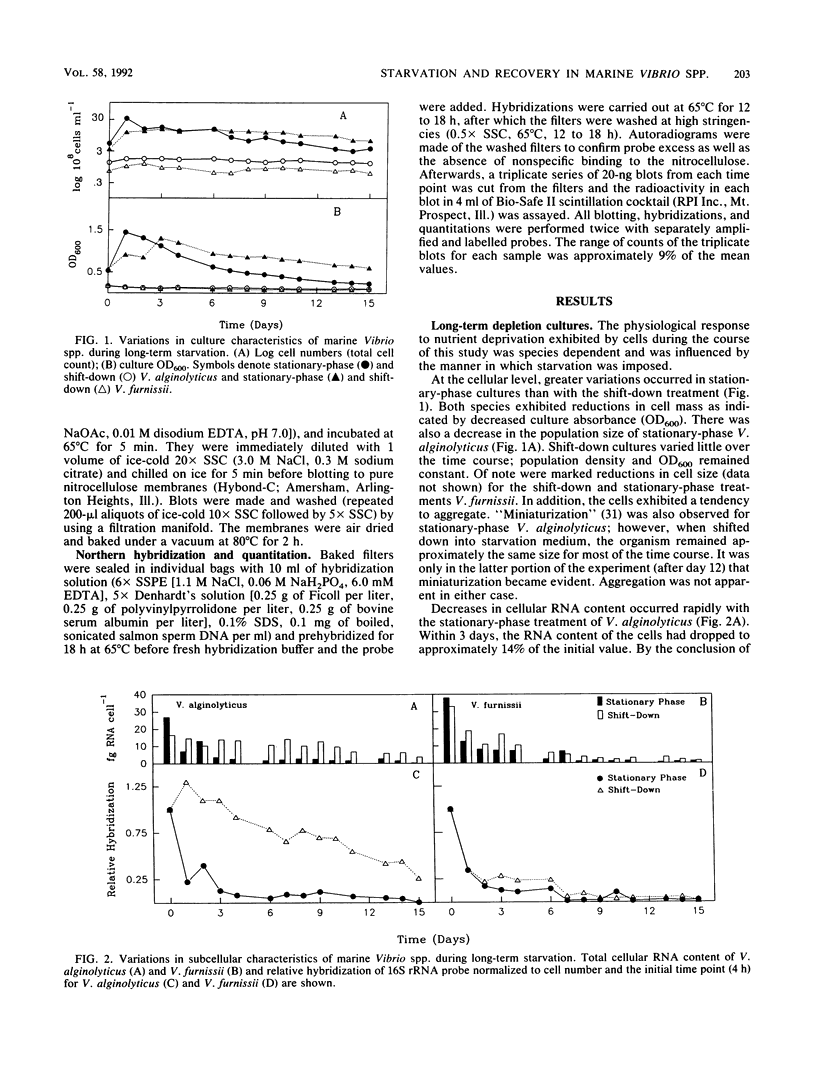
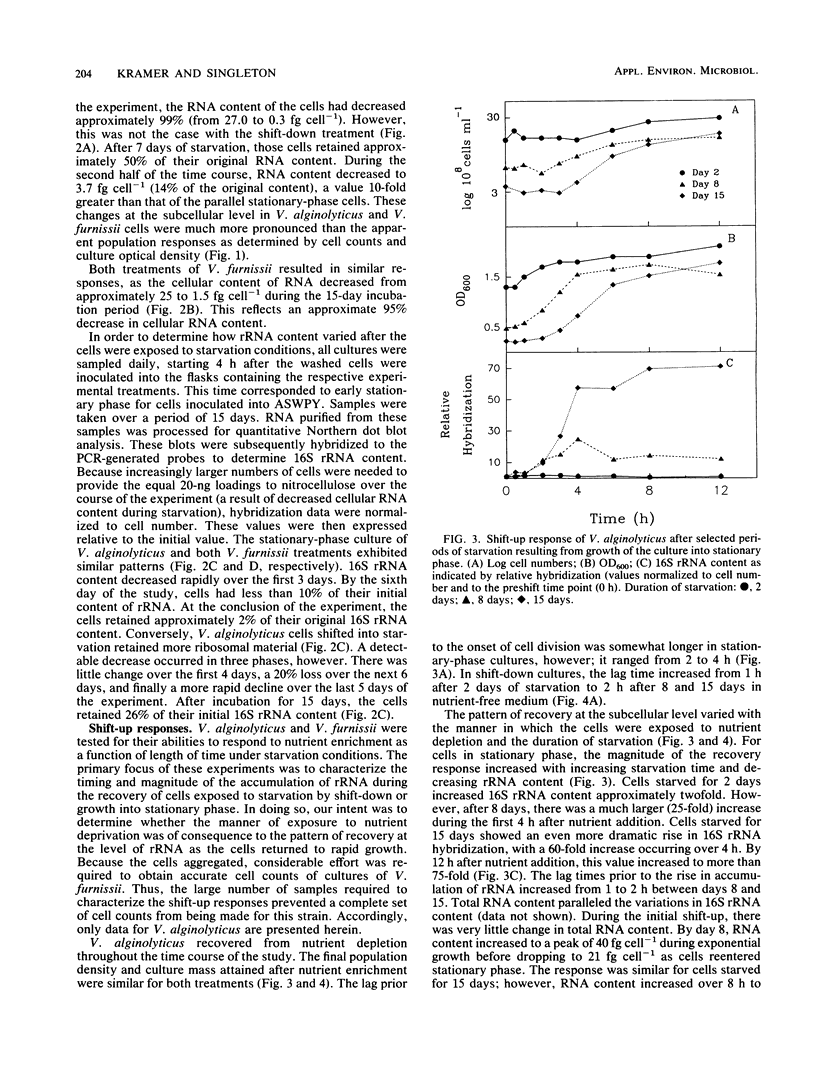
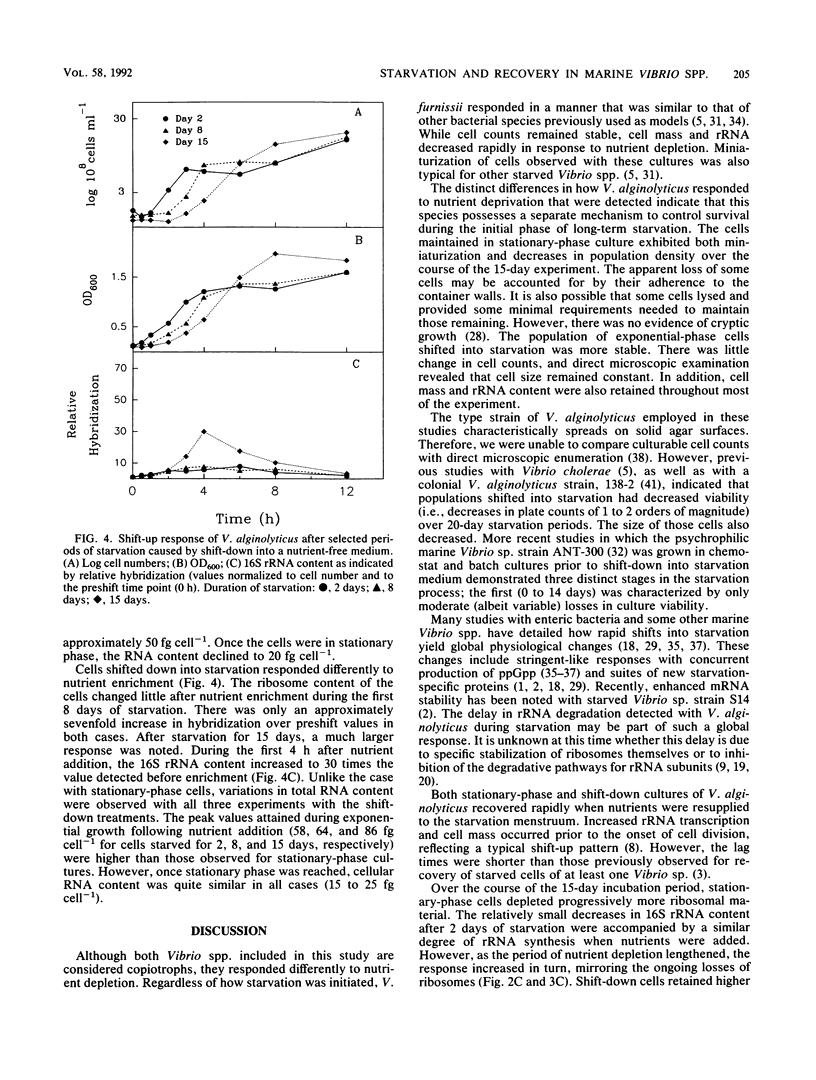
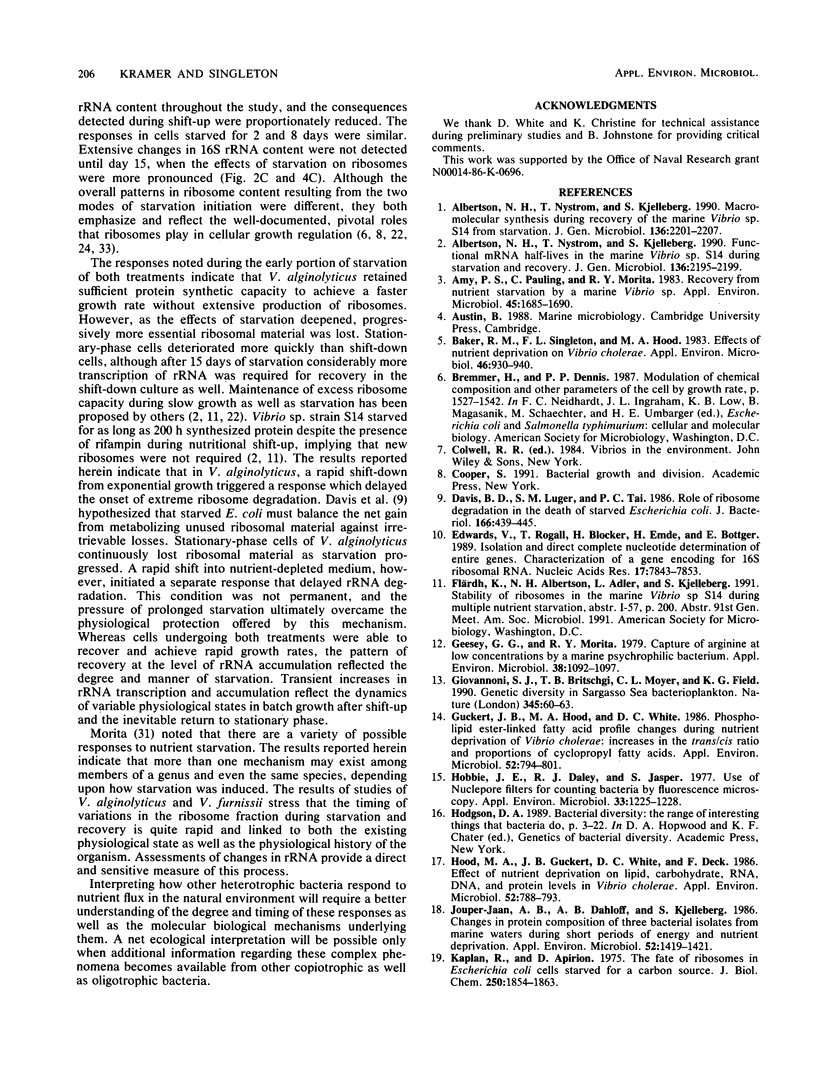
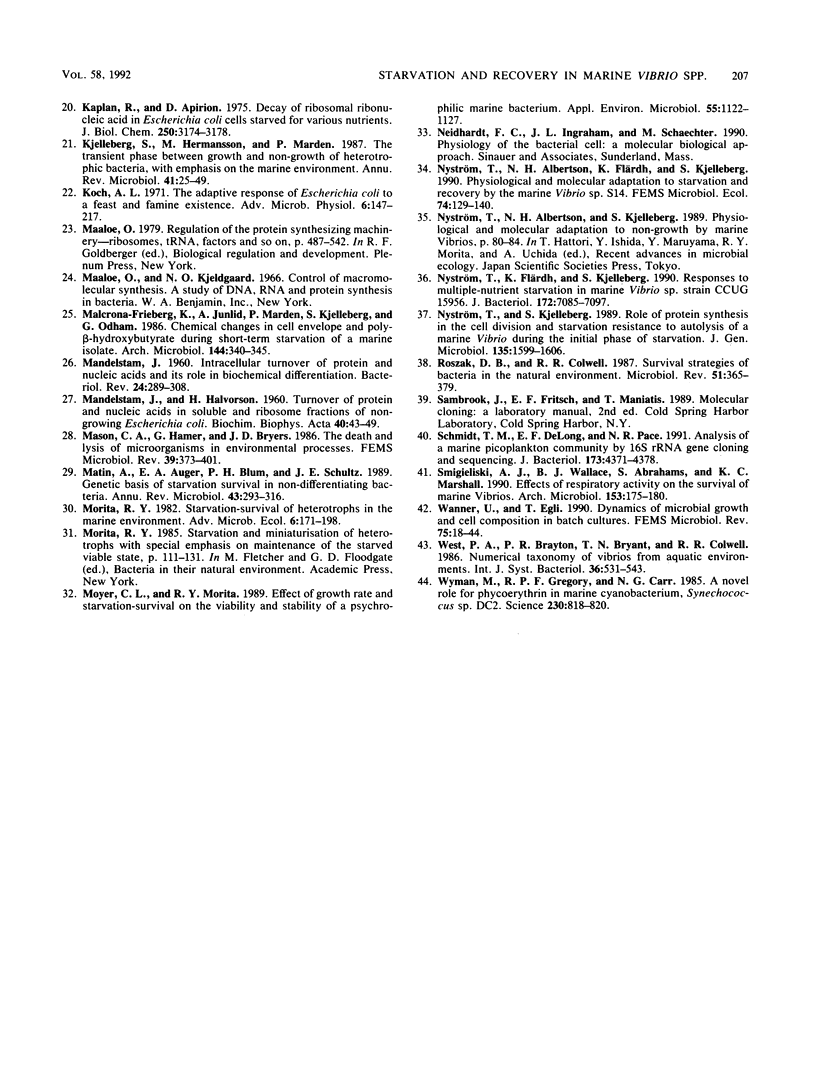
Selected References
These references are in PubMed. This may not be the complete list of references from this article.
- Amy P. S., Pauling C., Morita R. Y. Recovery from nutrient starvation by a marine Vibrio sp. Appl Environ Microbiol. 1983 May;45(5):1685–1690. doi: 10.1128/aem.45.5.1685-1690.1983. [DOI] [PMC free article] [PubMed] [Google Scholar]
- Baker R. M., Singleton F. L., Hood M. A. Effects of nutrient deprivation on Vibrio cholerae. Appl Environ Microbiol. 1983 Oct;46(4):930–940. doi: 10.1128/aem.46.4.930-940.1983. [DOI] [PMC free article] [PubMed] [Google Scholar]
- Davis B. D., Luger S. M., Tai P. C. Role of ribosome degradation in the death of starved Escherichia coli cells. J Bacteriol. 1986 May;166(2):439–445. doi: 10.1128/jb.166.2.439-445.1986. [DOI] [PMC free article] [PubMed] [Google Scholar]
- Edwards U., Rogall T., Blöcker H., Emde M., Böttger E. C. Isolation and direct complete nucleotide determination of entire genes. Characterization of a gene coding for 16S ribosomal RNA. Nucleic Acids Res. 1989 Oct 11;17(19):7843–7853. doi: 10.1093/nar/17.19.7843. [DOI] [PMC free article] [PubMed] [Google Scholar]
- Geesey G. G., Morita R. Y. Capture of arginine at low concentrations by a marine psychrophilic bacterium. Appl Environ Microbiol. 1979 Dec;38(6):1092–1097. doi: 10.1128/aem.38.6.1092-1097.1979. [DOI] [PMC free article] [PubMed] [Google Scholar]
- Giovannoni S. J., Britschgi T. B., Moyer C. L., Field K. G. Genetic diversity in Sargasso Sea bacterioplankton. Nature. 1990 May 3;345(6270):60–63. doi: 10.1038/345060a0. [DOI] [PubMed] [Google Scholar]
- Guckert J. B., Hood M. A., White D. C. Phospholipid ester-linked fatty acid profile changes during nutrient deprivation of Vibrio cholerae: increases in the trans/cis ratio and proportions of cyclopropyl fatty acids. Appl Environ Microbiol. 1986 Oct;52(4):794–801. doi: 10.1128/aem.52.4.794-801.1986. [DOI] [PMC free article] [PubMed] [Google Scholar]
- Hobbie J. E., Daley R. J., Jasper S. Use of nuclepore filters for counting bacteria by fluorescence microscopy. Appl Environ Microbiol. 1977 May;33(5):1225–1228. doi: 10.1128/aem.33.5.1225-1228.1977. [DOI] [PMC free article] [PubMed] [Google Scholar]
- Hood M. A., Guckert J. B., White D. C., Deck F. Effect of nutrient deprivation on lipid, carbohydrate, DNA, RNA, and protein levels in Vibrio cholerae. Appl Environ Microbiol. 1986 Oct;52(4):788–793. doi: 10.1128/aem.52.4.788-793.1986. [DOI] [PMC free article] [PubMed] [Google Scholar]
- Jaan A. J., Dahllöf B., Kjelleberg S. Changes in Protein Composition of Three Bacterial Isolates from Marine Waters during Short Periods of Energy and Nutrient Deprivation. Appl Environ Microbiol. 1986 Dec;52(6):1419–1421. doi: 10.1128/aem.52.6.1419-1421.1986. [DOI] [PMC free article] [PubMed] [Google Scholar]
- Kaplan R., Apirion D. Decay of ribosomal ribonucleic acid in Escherichia coli cells starved for various nutrients. J Biol Chem. 1975 Apr 25;250(8):3174–3178. [PubMed] [Google Scholar]
- Kaplan R., Apirion D. The fate of ribosomes in Escherichia coli cells starved for a carbon source. J Biol Chem. 1975 Mar 10;250(5):1854–1863. [PubMed] [Google Scholar]
- Kjelleberg S., Hermansson M., Mårdén P., Jones G. W. The transient phase between growth and nongrowth of heterotrophic bacteria, with emphasis on the marine environment. Annu Rev Microbiol. 1987;41:25–49. doi: 10.1146/annurev.mi.41.100187.000325. [DOI] [PubMed] [Google Scholar]
- Koch A. L. The adaptive responses of Escherichia coli to a feast and famine existence. Adv Microb Physiol. 1971;6:147–217. doi: 10.1016/s0065-2911(08)60069-7. [DOI] [PubMed] [Google Scholar]
- MANDELSTAM J. The intracellular turnover of protein and nucleic acids and its role in biochemical differentiation. Bacteriol Rev. 1960 Sep;24(3):289–308. doi: 10.1128/br.24.3.289-308.1960. [DOI] [PMC free article] [PubMed] [Google Scholar]
- Matin A., Auger E. A., Blum P. H., Schultz J. E. Genetic basis of starvation survival in nondifferentiating bacteria. Annu Rev Microbiol. 1989;43:293–316. doi: 10.1146/annurev.mi.43.100189.001453. [DOI] [PubMed] [Google Scholar]
- Moyer C. L., Morita R. Y. Effect of growth rate and starvation-survival on the viability and stability of a psychrophilic marine bacterium. Appl Environ Microbiol. 1989 May;55(5):1122–1127. doi: 10.1128/aem.55.5.1122-1127.1989. [DOI] [PMC free article] [PubMed] [Google Scholar]
- Nyström T., Flärdh K., Kjelleberg S. Responses to multiple-nutrient starvation in marine Vibrio sp. strain CCUG 15956. J Bacteriol. 1990 Dec;172(12):7085–7097. doi: 10.1128/jb.172.12.7085-7097.1990. [DOI] [PMC free article] [PubMed] [Google Scholar]
- Roszak D. B., Colwell R. R. Survival strategies of bacteria in the natural environment. Microbiol Rev. 1987 Sep;51(3):365–379. doi: 10.1128/mr.51.3.365-379.1987. [DOI] [PMC free article] [PubMed] [Google Scholar]
- Schmidt T. M., DeLong E. F., Pace N. R. Analysis of a marine picoplankton community by 16S rRNA gene cloning and sequencing. J Bacteriol. 1991 Jul;173(14):4371–4378. doi: 10.1128/jb.173.14.4371-4378.1991. [DOI] [PMC free article] [PubMed] [Google Scholar]
- Smigielski A. J., Wallace B. J., Abrahams S., Marshall K. C. Effects of respiratory activity on starvation survival of marine vibrios. Arch Microbiol. 1990;153(2):175–180. doi: 10.1007/BF00247817. [DOI] [PubMed] [Google Scholar]
- Wyman M., Gregory R. P., Carr N. G. Novel Role for Phycoerythrin in a Marine Cyanobacterium, Synechococcus Strain DC2. Science. 1985 Nov 15;230(4727):818–820. doi: 10.1126/science.230.4727.818. [DOI] [PubMed] [Google Scholar]