Abstract
The phytotoxin cercosporin, a singlet oxygen-generating photosensitizer, is toxic to plants, mice, and many fungi, yet the fungi that produce it, Cercospora spp., are resistant. We hypothesize that resistance to cercosporin may result from a reducing environment at the cell surface. Twenty tetrazolium dyes differing in redox potential were used as indicators of cell surface redox potential of seven fungal species differing in resistance to cercosporin. Resistant fungi were able to reduce significantly more dyes than were sensitive fungi. A correlation between dye reduction and cercosporin resistance was also observed when resistance levels of Cercospora species were manipulated by growth on different media. The addition of the reducing agents ascorbate, cysteine, and reduced glutathione (GSH) to growth media decreased cercosporin toxicity for sensitive fungi. None of these agents directly reduced cercosporin at the concentrations at which they protected fungi. Spectral and thin-layer chromatographic analyses of cercosporin solutions containing the different reducing agents indicated that GSH, but not cysteine or ascorbate, reacted with cercosporin. Resistant and sensitive fungi did not differ in endogenous levels of cysteine, GSH, or total thiols. On the basis of data from this and other studies, this report presents a model which proposes that cercosporin resistance results from the production of reducing power at the surfaces of resistant cells, leading to transient reduction and detoxification of the cercosporin molecule.
Full text
PDF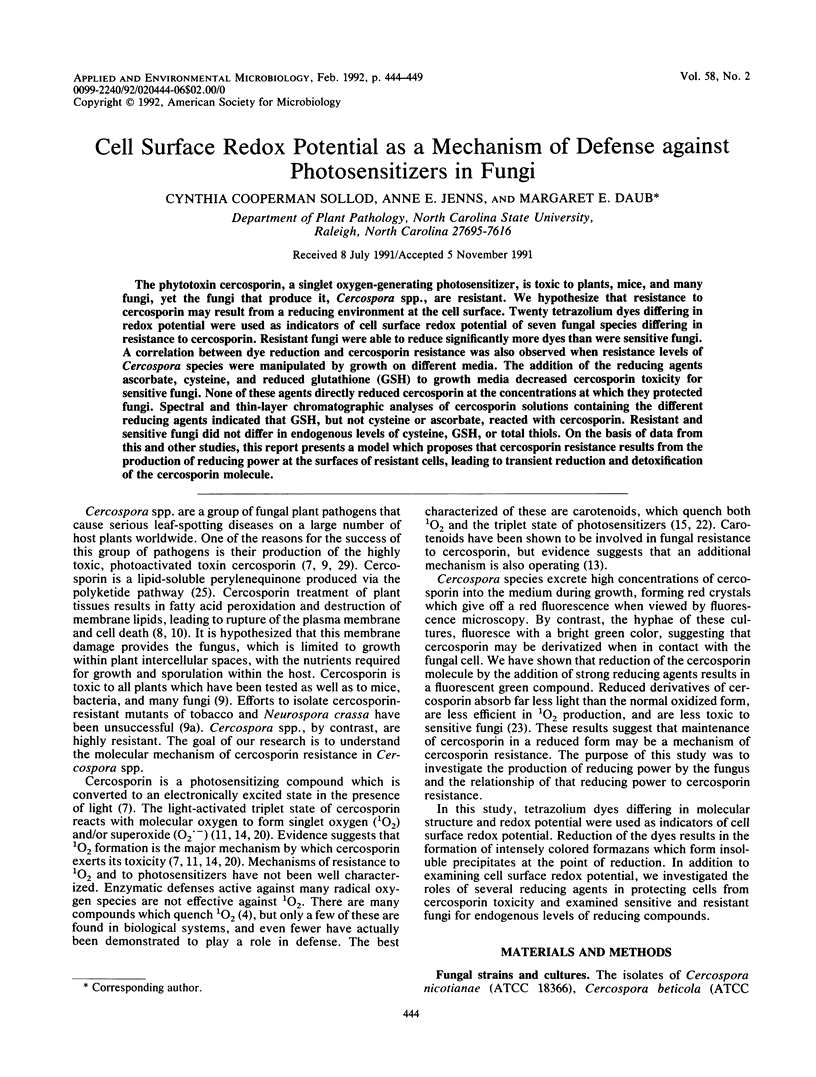
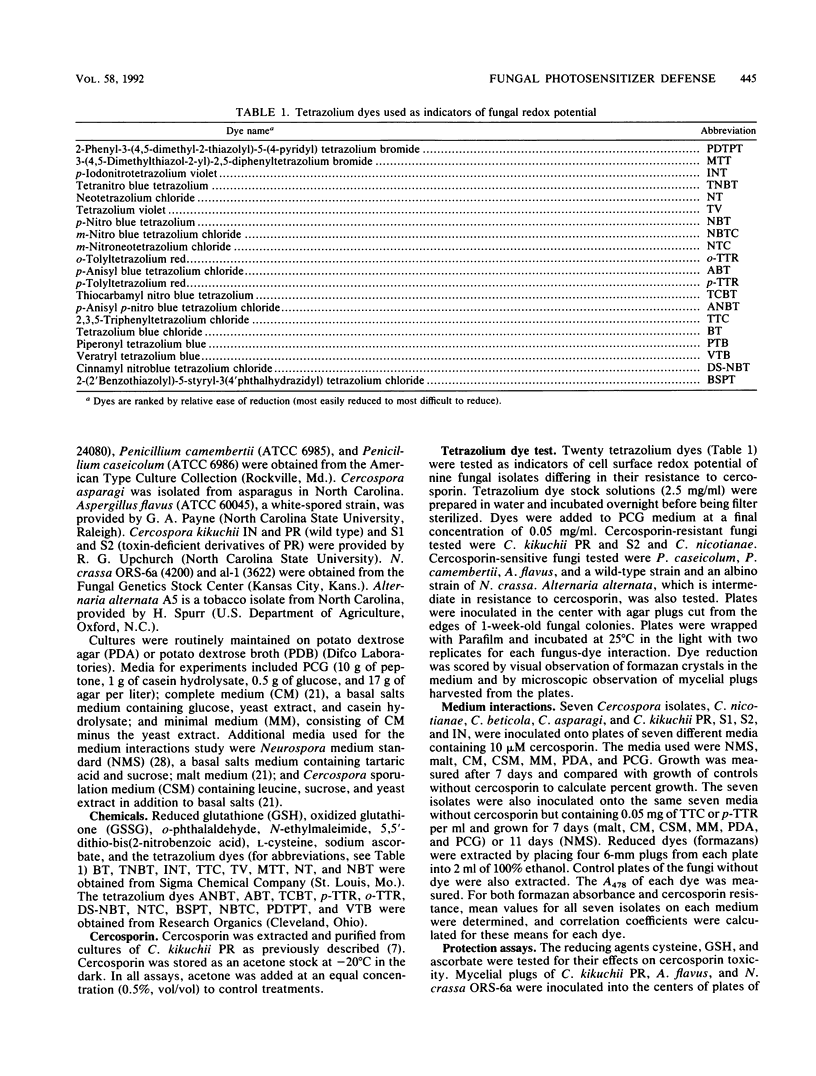
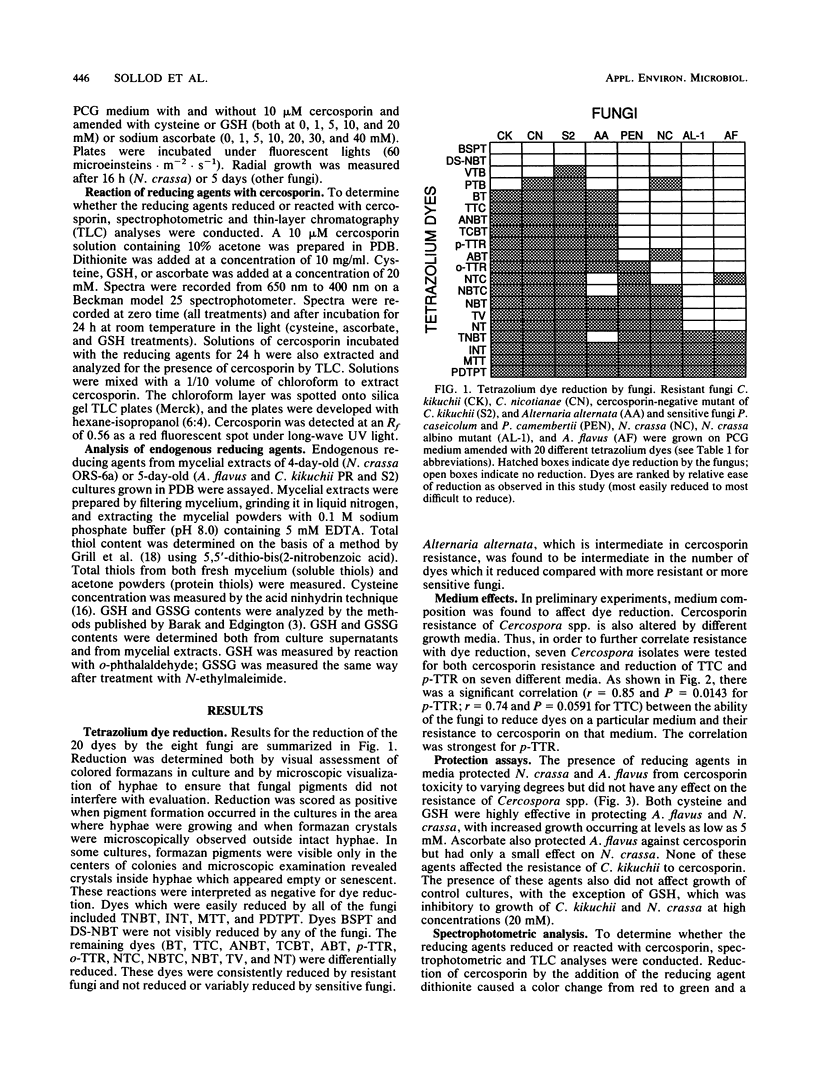
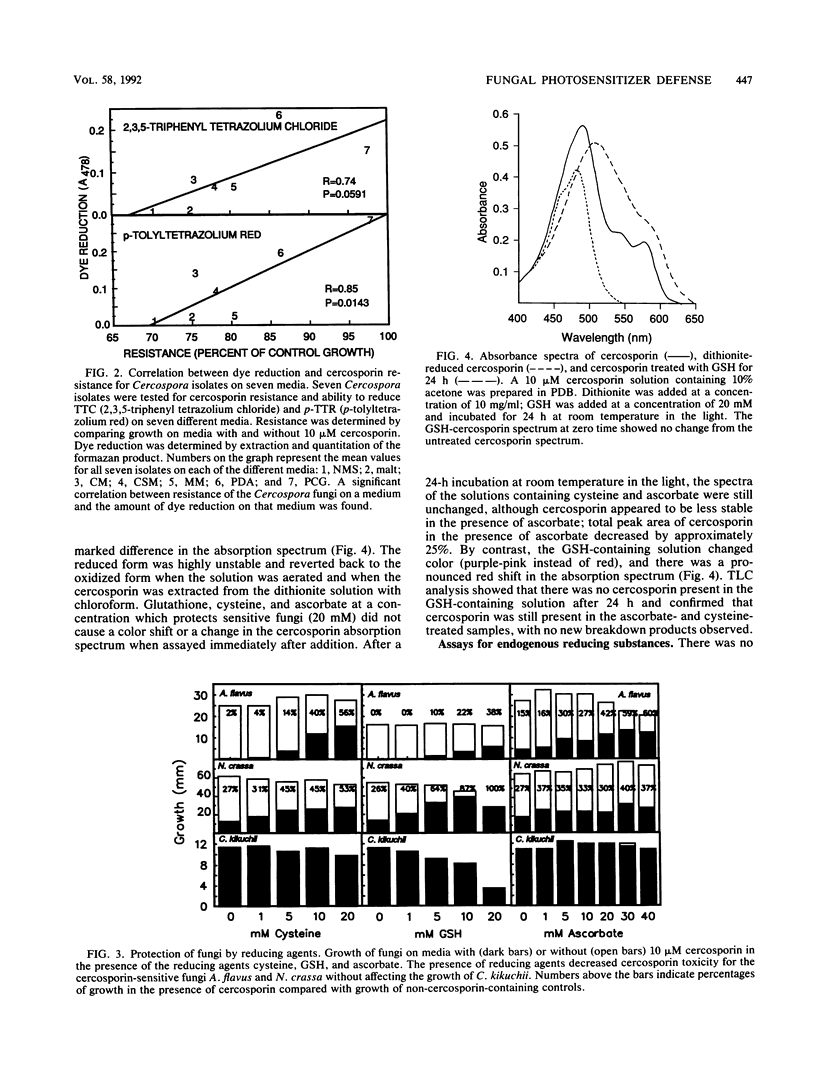
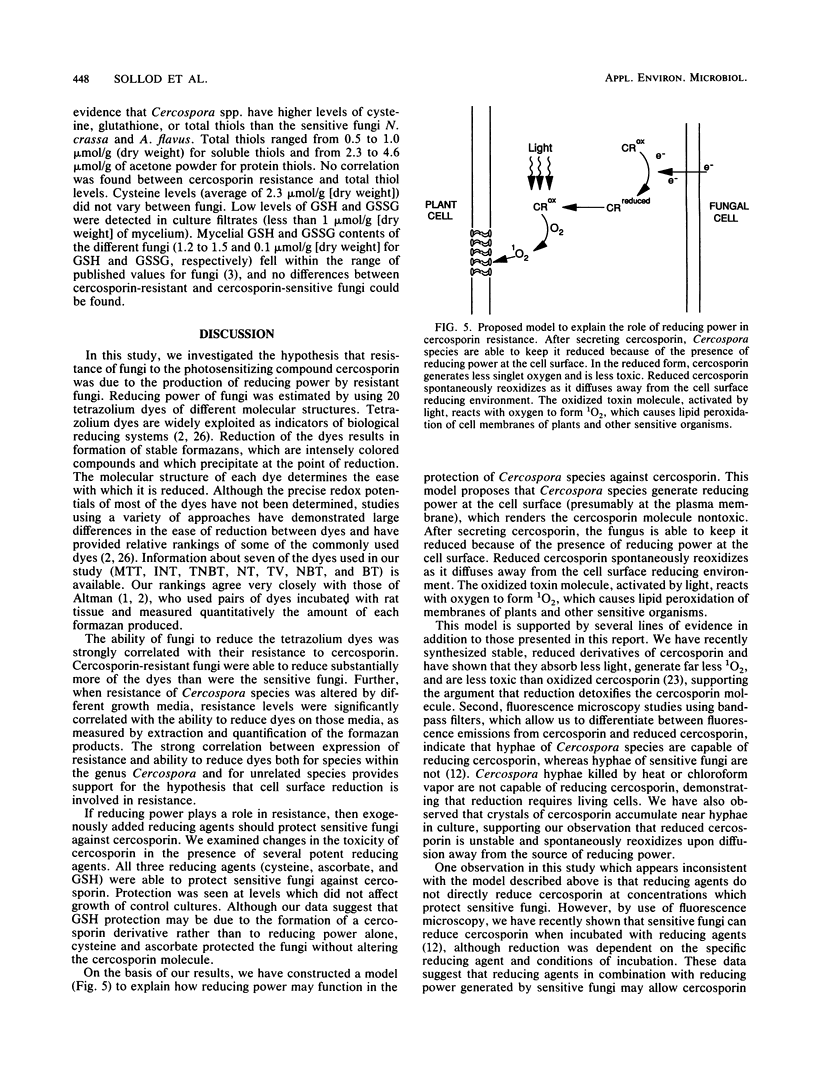
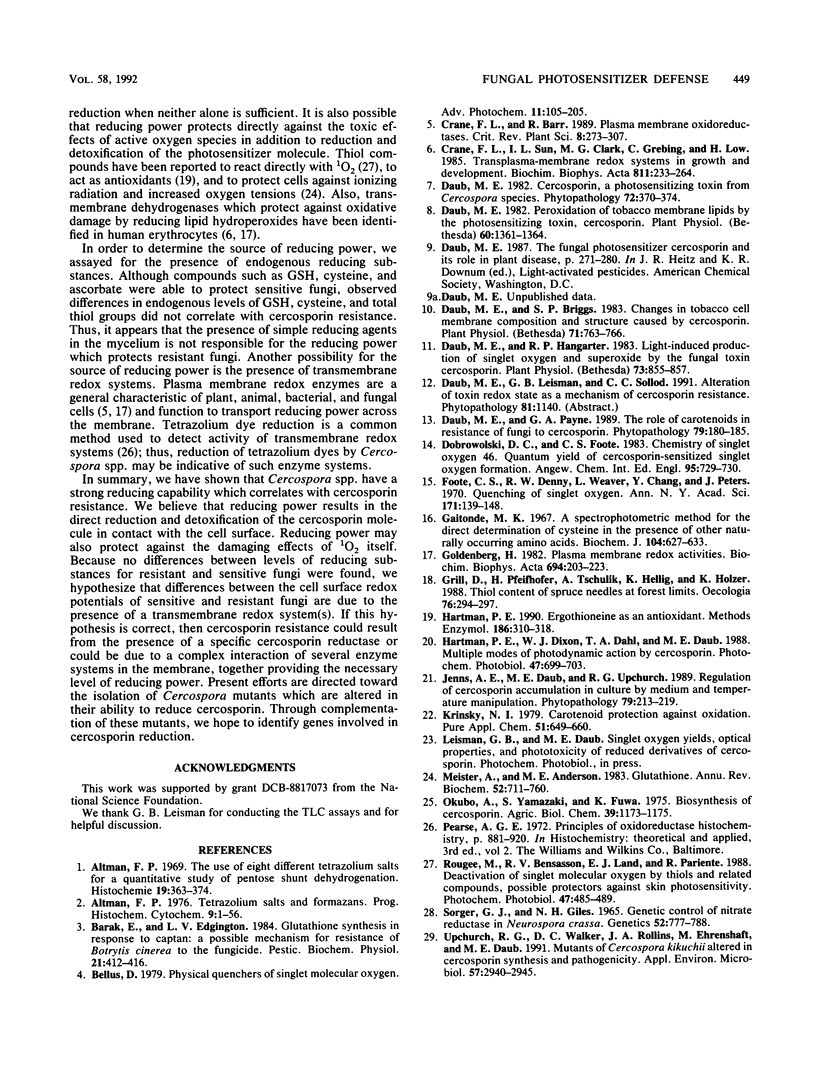
Selected References
These references are in PubMed. This may not be the complete list of references from this article.
- Altman F. P. Tetrazolium salts and formazans. Prog Histochem Cytochem. 1976;9(3):1–56. doi: 10.1016/s0079-6336(76)80015-0. [DOI] [PubMed] [Google Scholar]
- Altmann F. P. The use of eight different tetrazolium salts for a quantitative study of pentose shunt dehydrogenation. Histochemie. 1969;19(4):363–374. doi: 10.1007/BF00279685. [DOI] [PubMed] [Google Scholar]
- Crane F. L., Sun I. L., Clark M. G., Grebing C., Löw H. Transplasma-membrane redox systems in growth and development. Biochim Biophys Acta. 1985 Aug 1;811(3):233–264. doi: 10.1016/0304-4173(85)90013-8. [DOI] [PubMed] [Google Scholar]
- Daub M. E., Briggs S. P. Changes in tobacco cell membrane composition and structure caused by cercosporin. Plant Physiol. 1983 Apr;71(4):763–766. doi: 10.1104/pp.71.4.763. [DOI] [PMC free article] [PubMed] [Google Scholar]
- Daub M. E., Hangarter R. P. Light-induced production of singlet oxygen and superoxide by the fungal toxin, cercosporin. Plant Physiol. 1983 Nov;73(3):855–857. doi: 10.1104/pp.73.3.855. [DOI] [PMC free article] [PubMed] [Google Scholar]
- Daub M. E. Peroxidation of tobacco membrane lipids by the photosensitizing toxin, cercosporin. Plant Physiol. 1982 Jun;69(6):1361–1364. doi: 10.1104/pp.69.6.1361. [DOI] [PMC free article] [PubMed] [Google Scholar]
- Gaitonde M. K. A spectrophotometric method for the direct determination of cysteine in the presence of other naturally occurring amino acids. Biochem J. 1967 Aug;104(2):627–633. doi: 10.1042/bj1040627. [DOI] [PMC free article] [PubMed] [Google Scholar]
- Goldenberg H. Plasma membrane redox activities. Biochim Biophys Acta. 1982 Oct 20;694(2):203–223. doi: 10.1016/0304-4157(82)90025-9. [DOI] [PubMed] [Google Scholar]
- Hartman P. E., Dixon W. J., Dahl T. A., Daub M. E. Multiple modes of photodynamic action by cercosporin. Photochem Photobiol. 1988 May;47(5):699–703. doi: 10.1111/j.1751-1097.1988.tb02767.x. [DOI] [PubMed] [Google Scholar]
- Hartman P. E. Ergothioneine as antioxidant. Methods Enzymol. 1990;186:310–318. doi: 10.1016/0076-6879(90)86124-e. [DOI] [PubMed] [Google Scholar]
- Meister A., Anderson M. E. Glutathione. Annu Rev Biochem. 1983;52:711–760. doi: 10.1146/annurev.bi.52.070183.003431. [DOI] [PubMed] [Google Scholar]
- Rougee M., Bensasson R. V., Land E. J., Pariente R. Deactivation of singlet molecular oxygen by thiols and related compounds, possible protectors against skin photosensitivity. Photochem Photobiol. 1988 Apr;47(4):485–489. doi: 10.1111/j.1751-1097.1988.tb08835.x. [DOI] [PubMed] [Google Scholar]
- Sorger G. J., Giles N. H. Genetic control of nitrate reductase in Neurospora crassa. Genetics. 1965 Oct;52(4):777–788. doi: 10.1093/genetics/52.4.777. [DOI] [PMC free article] [PubMed] [Google Scholar]
- Upchurch R. G., Walker D. C., Rollins J. A., Ehrenshaft M., Daub M. E. Mutants of Cercospora kikuchii Altered in Cercosporin Synthesis and Pathogenicity. Appl Environ Microbiol. 1991 Oct;57(10):2940–2945. doi: 10.1128/aem.57.10.2940-2945.1991. [DOI] [PMC free article] [PubMed] [Google Scholar]