Abstract
Exogenously provided glycine betaine functions as an efficient osmoprotectant for Bacillus subtilis in high-osmolarity environments. This gram-positive soil organism is not able to increase the intracellular level of glycine betaine through de novo synthesis in defined medium (A. M. Whatmore, J. A. Chudek, and R. H. Reed, J. Gen. Microbiol. 136:2527-2535, 1990). We found, however, that B. subtilis can synthesize glycine betaine when its biosynthetic precursor, choline, is present in the growth medium. Uptake studies with radiolabelled [methyl-14C]choline demonstrated that choline transport is osmotically controlled and is mediated by a high-affinity uptake system. Choline transport of cells grown in low- and high-osmolarity media showed Michaelis-Menten kinetics with Km values of 3 and 5 microM and maximum rates of transport (Vmax) of 10 and 36 nmol min-1 mg of protein-1, respectively. The choline transporter exhibited considerable substrate specificity, and the results of competition experiments suggest that the fully methylated quaternary ammonium group is a key feature for substrate recognition. Thin-layer chromatography revealed that the radioactivity from exogenously provided [methyl-14C]choline accumulated intracellularly as [methyl-14C]glycine betaine, demonstrating that B. subtilis possesses enzymes for the oxidative conversion of choline into glycine betaine. Exogenously provided choline significantly increased the growth rate of B. subtilis in high-osmolarity media and permitted its proliferation under conditions that are otherwise strongly inhibitory for its growth. Choline and glycine betaine were not used as sole sources of carbon or nitrogen, consistent with their functional role in the process of adaptation of B. subtilis to high-osmolarity stress.
Full text
PDF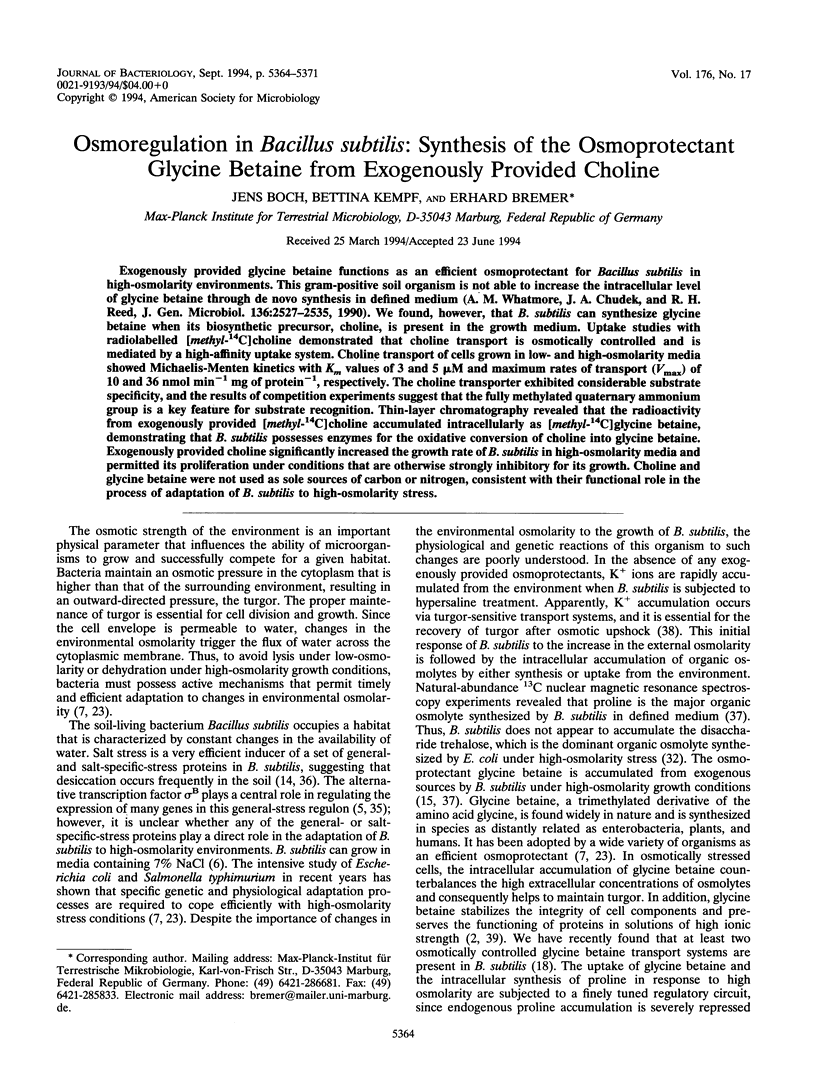
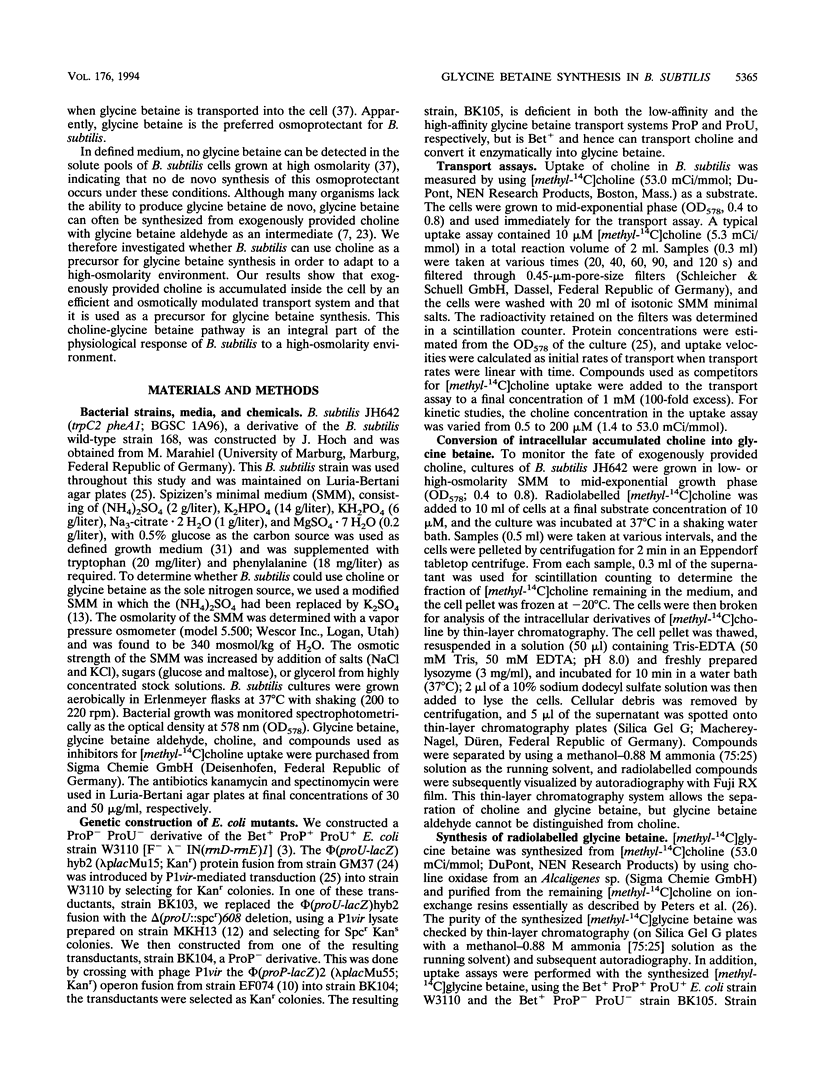
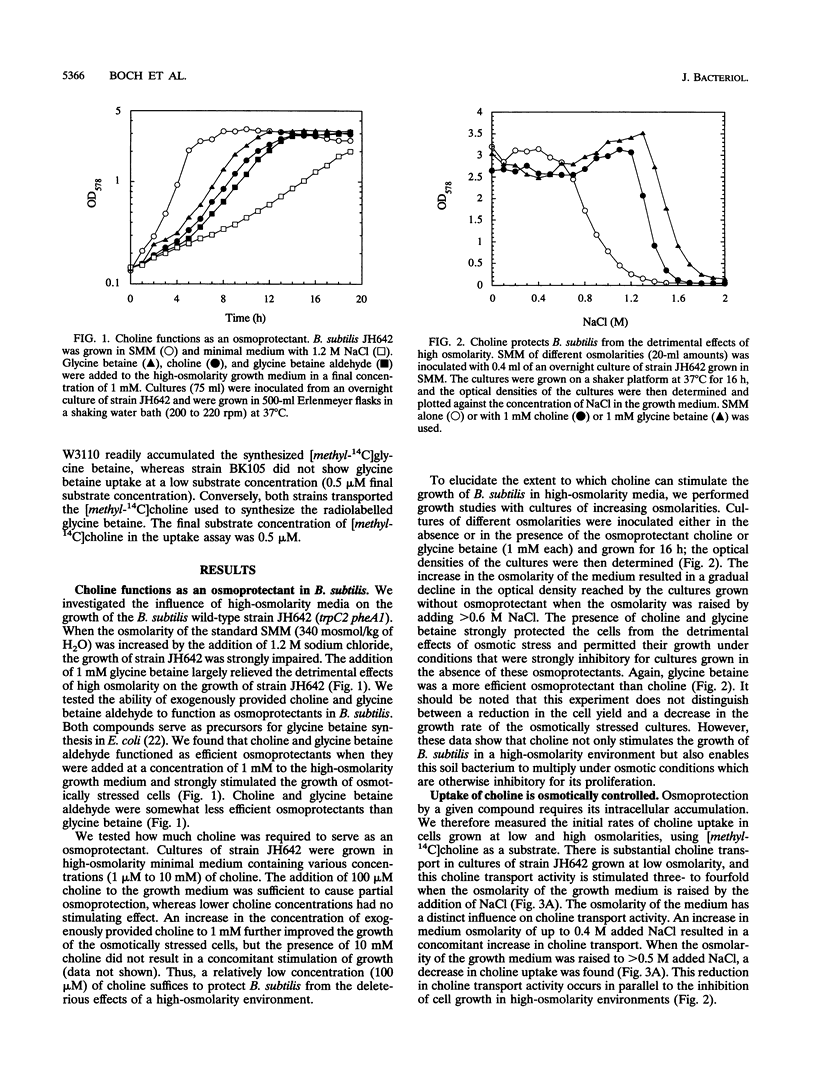
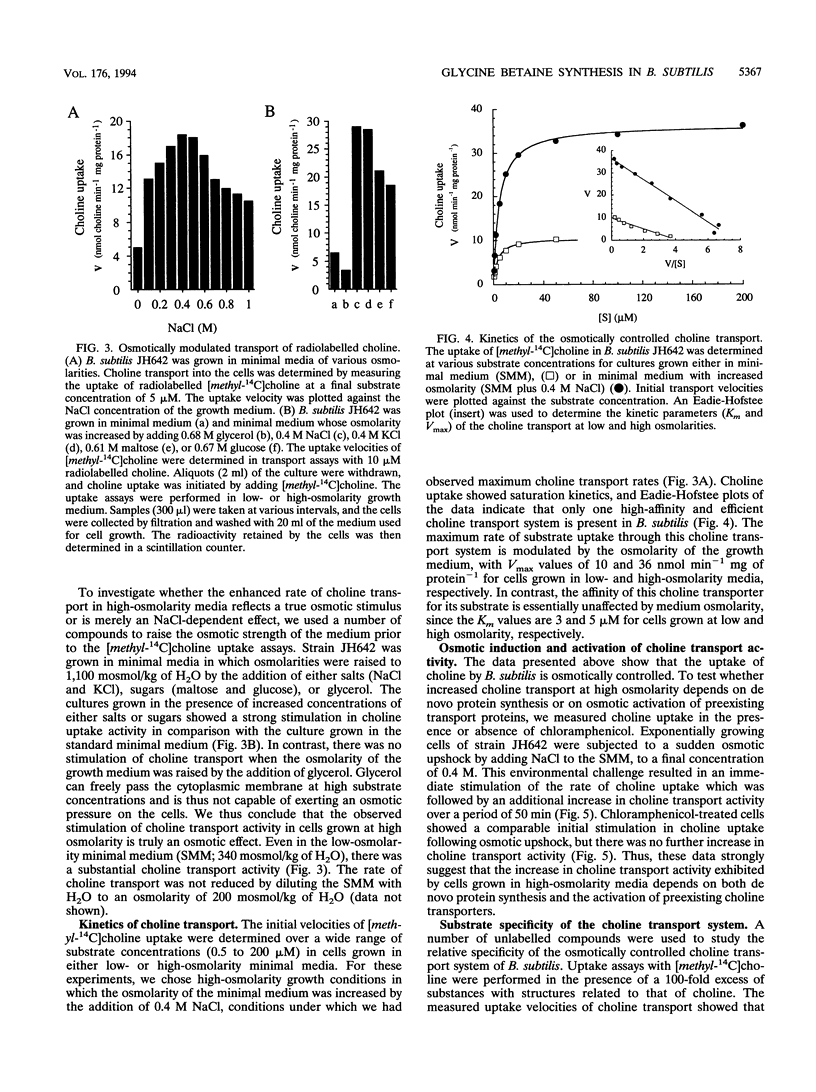
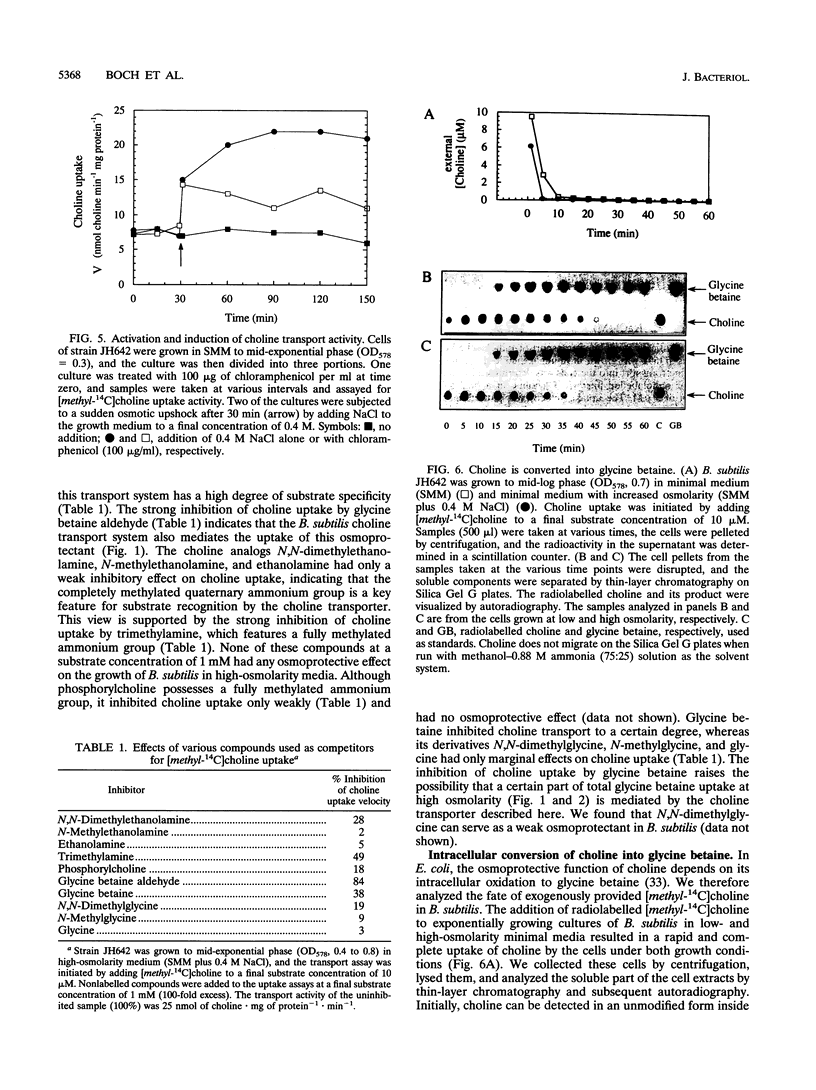
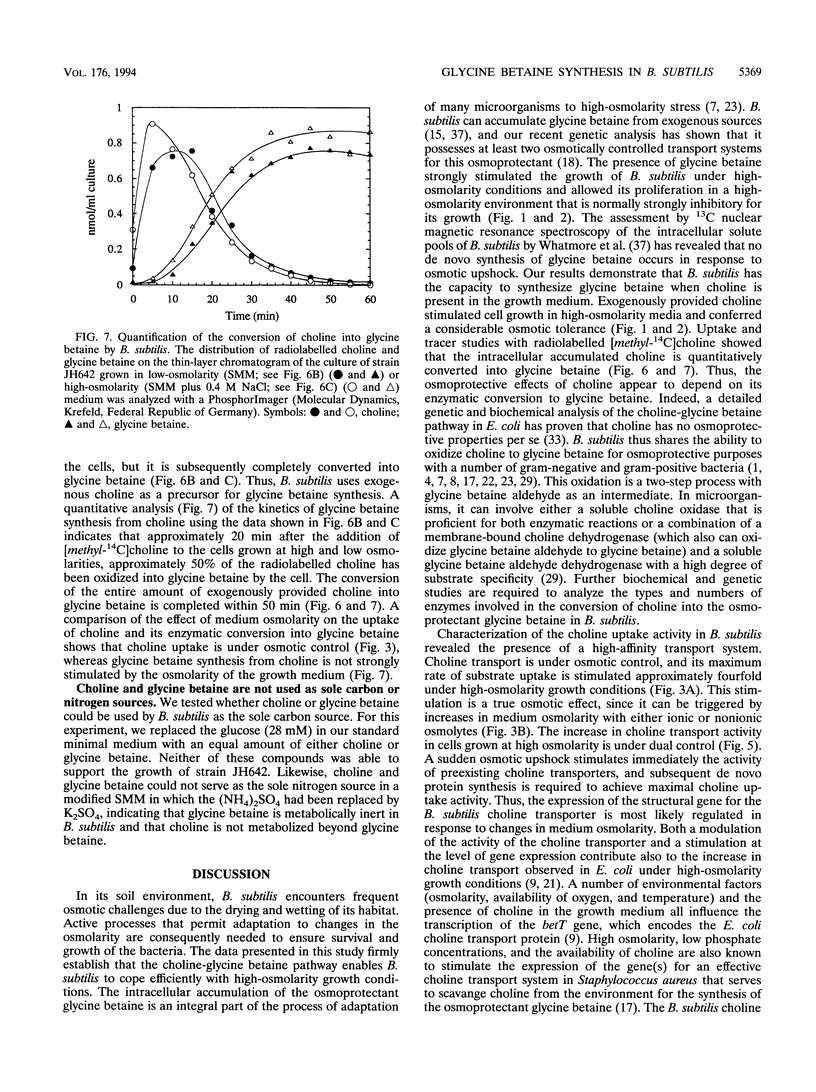
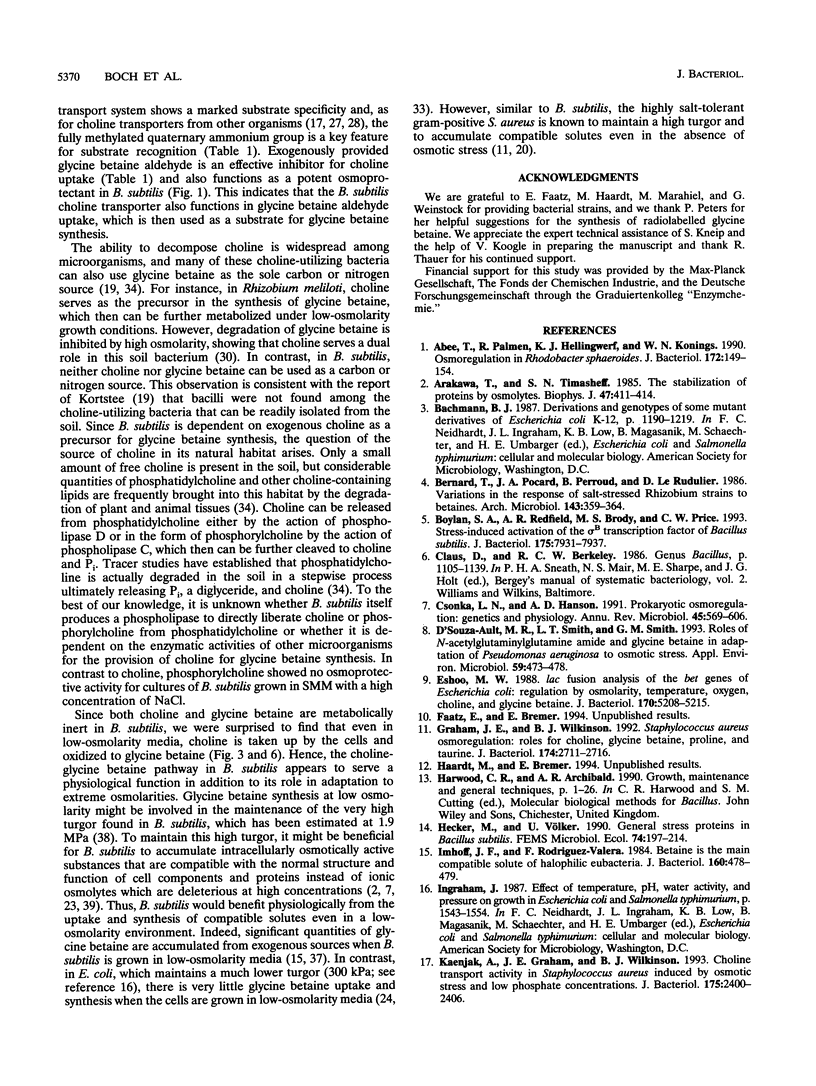
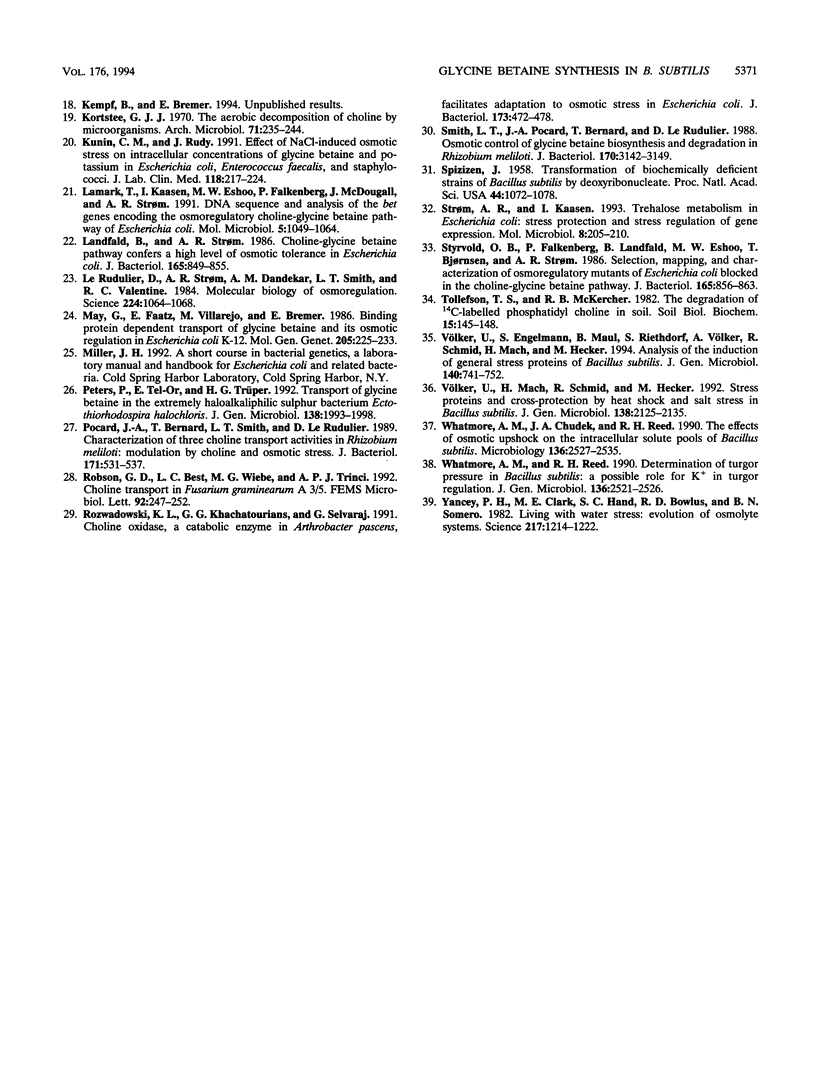
Images in this article
Selected References
These references are in PubMed. This may not be the complete list of references from this article.
- Abee T., Palmen R., Hellingwerf K. J., Konings W. N. Osmoregulation in Rhodobacter sphaeroides. J Bacteriol. 1990 Jan;172(1):149–154. doi: 10.1128/jb.172.1.149-154.1990. [DOI] [PMC free article] [PubMed] [Google Scholar]
- Arakawa T., Timasheff S. N. The stabilization of proteins by osmolytes. Biophys J. 1985 Mar;47(3):411–414. doi: 10.1016/S0006-3495(85)83932-1. [DOI] [PMC free article] [PubMed] [Google Scholar]
- Boylan S. A., Redfield A. R., Brody M. S., Price C. W. Stress-induced activation of the sigma B transcription factor of Bacillus subtilis. J Bacteriol. 1993 Dec;175(24):7931–7937. doi: 10.1128/jb.175.24.7931-7937.1993. [DOI] [PMC free article] [PubMed] [Google Scholar]
- Csonka L. N., Hanson A. D. Prokaryotic osmoregulation: genetics and physiology. Annu Rev Microbiol. 1991;45:569–606. doi: 10.1146/annurev.mi.45.100191.003033. [DOI] [PubMed] [Google Scholar]
- D'Souza-Ault M. R., Smith L. T., Smith G. M. Roles of N-acetylglutaminylglutamine amide and glycine betaine in adaptation of Pseudomonas aeruginosa to osmotic stress. Appl Environ Microbiol. 1993 Feb;59(2):473–478. doi: 10.1128/aem.59.2.473-478.1993. [DOI] [PMC free article] [PubMed] [Google Scholar]
- Eshoo M. W. lac fusion analysis of the bet genes of Escherichia coli: regulation by osmolarity, temperature, oxygen, choline, and glycine betaine. J Bacteriol. 1988 Nov;170(11):5208–5215. doi: 10.1128/jb.170.11.5208-5215.1988. [DOI] [PMC free article] [PubMed] [Google Scholar]
- Graham J. E., Wilkinson B. J. Staphylococcus aureus osmoregulation: roles for choline, glycine betaine, proline, and taurine. J Bacteriol. 1992 Apr;174(8):2711–2716. doi: 10.1128/jb.174.8.2711-2716.1992. [DOI] [PMC free article] [PubMed] [Google Scholar]
- Imhoff J. F., Rodriguez-Valera F. Betaine is the main compatible solute of halophilic eubacteria. J Bacteriol. 1984 Oct;160(1):478–479. doi: 10.1128/jb.160.1.478-479.1984. [DOI] [PMC free article] [PubMed] [Google Scholar]
- Kaenjak A., Graham J. E., Wilkinson B. J. Choline transport activity in Staphylococcus aureus induced by osmotic stress and low phosphate concentrations. J Bacteriol. 1993 Apr;175(8):2400–2406. doi: 10.1128/jb.175.8.2400-2406.1993. [DOI] [PMC free article] [PubMed] [Google Scholar]
- Kortstee G. J. The aerobic decomposition of choline by microorganisms. I. The ability of aerobic organisms, particularly coryneform bacteria, to utilize choline as the sole carbon and nitrogen source. Arch Mikrobiol. 1970;71(3):235–244. [PubMed] [Google Scholar]
- Kunin C. M., Rudy J. Effect of NaCl-induced osmotic stress on intracellular concentrations of glycine betaine and potassium in Escherichia coli, Enterococcus faecalis, and staphylococci. J Lab Clin Med. 1991 Sep;118(3):217–224. [PubMed] [Google Scholar]
- Lamark T., Kaasen I., Eshoo M. W., Falkenberg P., McDougall J., Strøm A. R. DNA sequence and analysis of the bet genes encoding the osmoregulatory choline-glycine betaine pathway of Escherichia coli. Mol Microbiol. 1991 May;5(5):1049–1064. doi: 10.1111/j.1365-2958.1991.tb01877.x. [DOI] [PubMed] [Google Scholar]
- Landfald B., Strøm A. R. Choline-glycine betaine pathway confers a high level of osmotic tolerance in Escherichia coli. J Bacteriol. 1986 Mar;165(3):849–855. doi: 10.1128/jb.165.3.849-855.1986. [DOI] [PMC free article] [PubMed] [Google Scholar]
- Le Rudulier D., Strom A. R., Dandekar A. M., Smith L. T., Valentine R. C. Molecular biology of osmoregulation. Science. 1984 Jun 8;224(4653):1064–1068. doi: 10.1126/science.224.4653.1064. [DOI] [PubMed] [Google Scholar]
- May G., Faatz E., Villarejo M., Bremer E. Binding protein dependent transport of glycine betaine and its osmotic regulation in Escherichia coli K12. Mol Gen Genet. 1986 Nov;205(2):225–233. doi: 10.1007/BF00430432. [DOI] [PubMed] [Google Scholar]
- Pocard J. A., Bernard T., Smith L. T., Le Rudulier D. Characterization of three choline transport activities in Rhizobium meliloti: modulation by choline and osmotic stress. J Bacteriol. 1989 Jan;171(1):531–537. doi: 10.1128/jb.171.1.531-537.1989. [DOI] [PMC free article] [PubMed] [Google Scholar]
- Robson G. D., Best L. C., Wiebe M. G., Trinci A. P. Choline transport in Fusarium graminearum A 3/5. FEMS Microbiol Lett. 1992 May 1;71(3):247–251. doi: 10.1016/0378-1097(92)90717-3. [DOI] [PubMed] [Google Scholar]
- Rozwadowski K. L., Khachatourians G. G., Selvaraj G. Choline oxidase, a catabolic enzyme in Arthrobacter pascens, facilitates adaptation to osmotic stress in Escherichia coli. J Bacteriol. 1991 Jan;173(2):472–478. doi: 10.1128/jb.173.2.472-478.1991. [DOI] [PMC free article] [PubMed] [Google Scholar]
- Smith L. T., Pocard J. A., Bernard T., Le Rudulier D. Osmotic control of glycine betaine biosynthesis and degradation in Rhizobium meliloti. J Bacteriol. 1988 Jul;170(7):3142–3149. doi: 10.1128/jb.170.7.3142-3149.1988. [DOI] [PMC free article] [PubMed] [Google Scholar]
- Spizizen J. TRANSFORMATION OF BIOCHEMICALLY DEFICIENT STRAINS OF BACILLUS SUBTILIS BY DEOXYRIBONUCLEATE. Proc Natl Acad Sci U S A. 1958 Oct 15;44(10):1072–1078. doi: 10.1073/pnas.44.10.1072. [DOI] [PMC free article] [PubMed] [Google Scholar]
- Strøm A. R., Kaasen I. Trehalose metabolism in Escherichia coli: stress protection and stress regulation of gene expression. Mol Microbiol. 1993 Apr;8(2):205–210. doi: 10.1111/j.1365-2958.1993.tb01564.x. [DOI] [PubMed] [Google Scholar]
- Styrvold O. B., Falkenberg P., Landfald B., Eshoo M. W., Bjørnsen T., Strøm A. R. Selection, mapping, and characterization of osmoregulatory mutants of Escherichia coli blocked in the choline-glycine betaine pathway. J Bacteriol. 1986 Mar;165(3):856–863. doi: 10.1128/jb.165.3.856-863.1986. [DOI] [PMC free article] [PubMed] [Google Scholar]
- Völker U., Engelmann S., Maul B., Riethdorf S., Völker A., Schmid R., Mach H., Hecker M. Analysis of the induction of general stress proteins of Bacillus subtilis. Microbiology. 1994 Apr;140(Pt 4):741–752. doi: 10.1099/00221287-140-4-741. [DOI] [PubMed] [Google Scholar]
- Völker U., Mach H., Schmid R., Hecker M. Stress proteins and cross-protection by heat shock and salt stress in Bacillus subtilis. J Gen Microbiol. 1992 Oct;138(10):2125–2135. doi: 10.1099/00221287-138-10-2125. [DOI] [PubMed] [Google Scholar]
- Whatmore A. M., Chudek J. A., Reed R. H. The effects of osmotic upshock on the intracellular solute pools of Bacillus subtilis. J Gen Microbiol. 1990 Dec;136(12):2527–2535. doi: 10.1099/00221287-136-12-2527. [DOI] [PubMed] [Google Scholar]
- Whatmore A. M., Reed R. H. Determination of turgor pressure in Bacillus subtilis: a possible role for K+ in turgor regulation. J Gen Microbiol. 1990 Dec;136(12):2521–2526. doi: 10.1099/00221287-136-12-2521. [DOI] [PubMed] [Google Scholar]
- Yancey P. H., Clark M. E., Hand S. C., Bowlus R. D., Somero G. N. Living with water stress: evolution of osmolyte systems. Science. 1982 Sep 24;217(4566):1214–1222. doi: 10.1126/science.7112124. [DOI] [PubMed] [Google Scholar]