Abstract
The association of intracellular viscosity of red blood cells and the dynamic properties of erythrocyte membranes in children suffering from diabetes has been investigated by means of ESR spectroscopy. It has been revealed that the slight decrease in the ratio hw/hs of maleimide bound to membrane protein-SH groups of erythrocytes in diabetes may ensue from the enhanced membrane protein immobilization in the plane of lipid bilayer. These alterations were accompanied by a corresponding increase in the relative rotational correlation time (tau c) of iodoacetamide spin label, thus suggesting that the conformational changes in membrane proteins may occur at both the intrinsic and more exposed thiol groups. The membranes of diabetic red blood cells were more glycosylated than those of relevant controls, and the extent of glycosylation was found to correlate significantly with h + 1/h0 and tau c (r = -0.652, P < 0.01 and r = 0.609, P < 0.01). Further, the conformational alterations in erythrocyte membranes from diabetic subjects were accompanied by a significant increase in the mobility parameter (h + 1/h0) of haemoglobin molecules in diabetic erythrocytes. The latter changes correlated well with the enhanced intracellular viscosity of diabetic red blood cells and the level of glycosylated haemoglobin. We conclude that the alterations in membrane lipid-protein interactions together with the increased glycosylation-derived internal viscosity may consequently imply altered viscoelastic properties of erythrocyte membranes and, underlying the impaired deformability of red blood cells in the diabetic state, contribute to the development of late diabetic sequelae.
Full text
PDF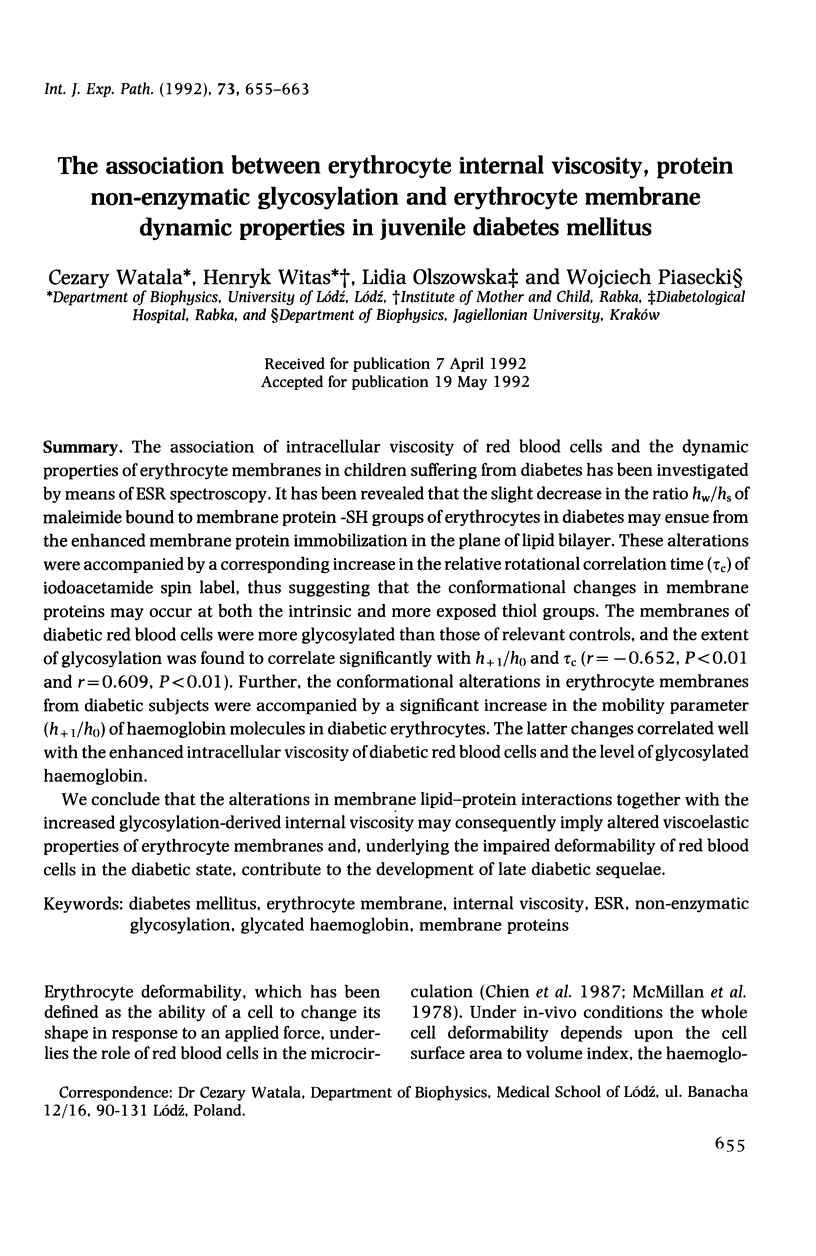
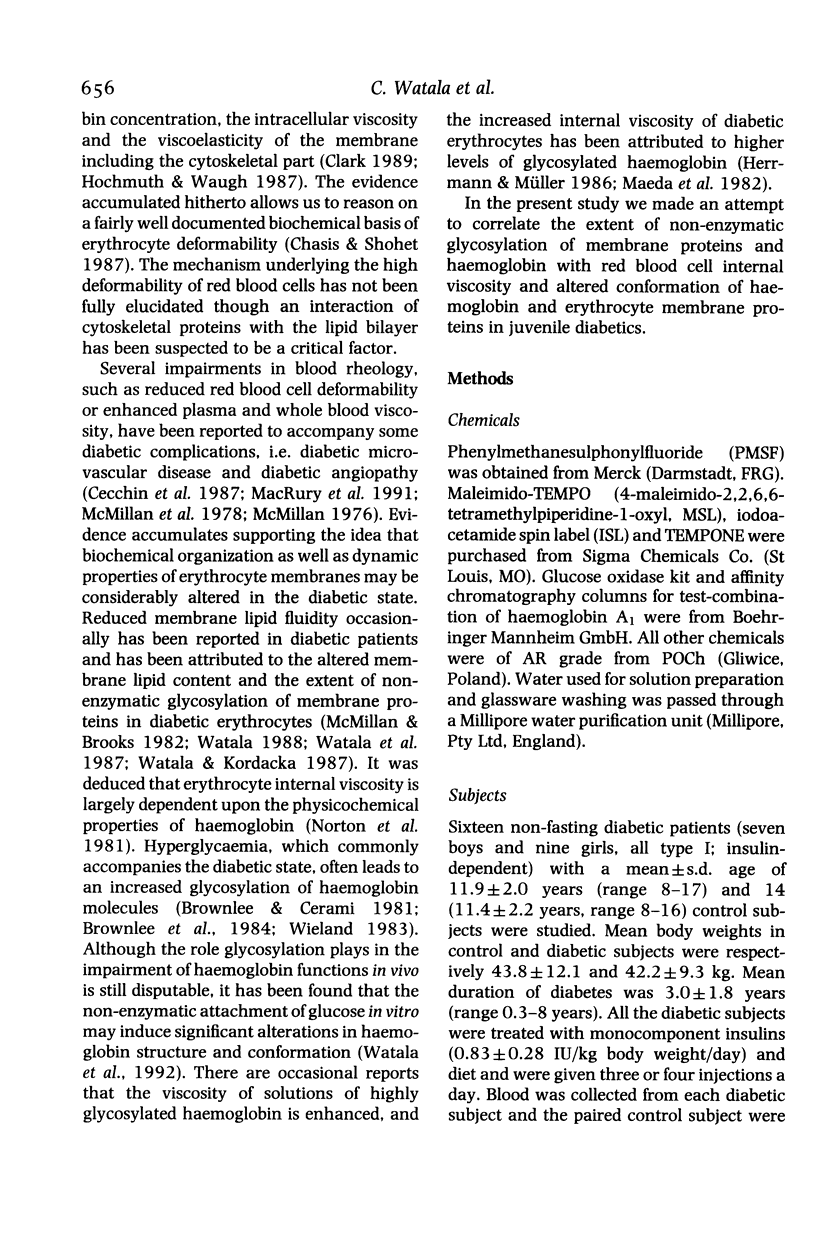
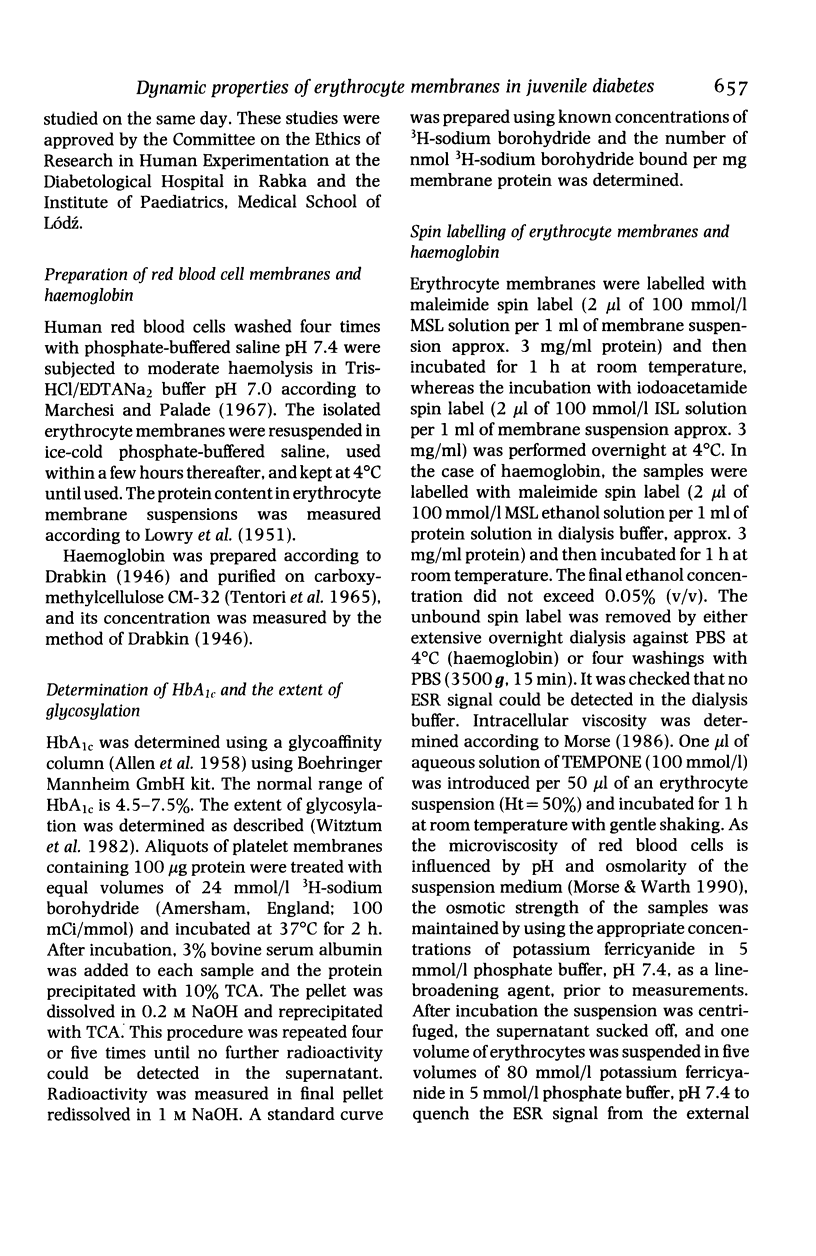
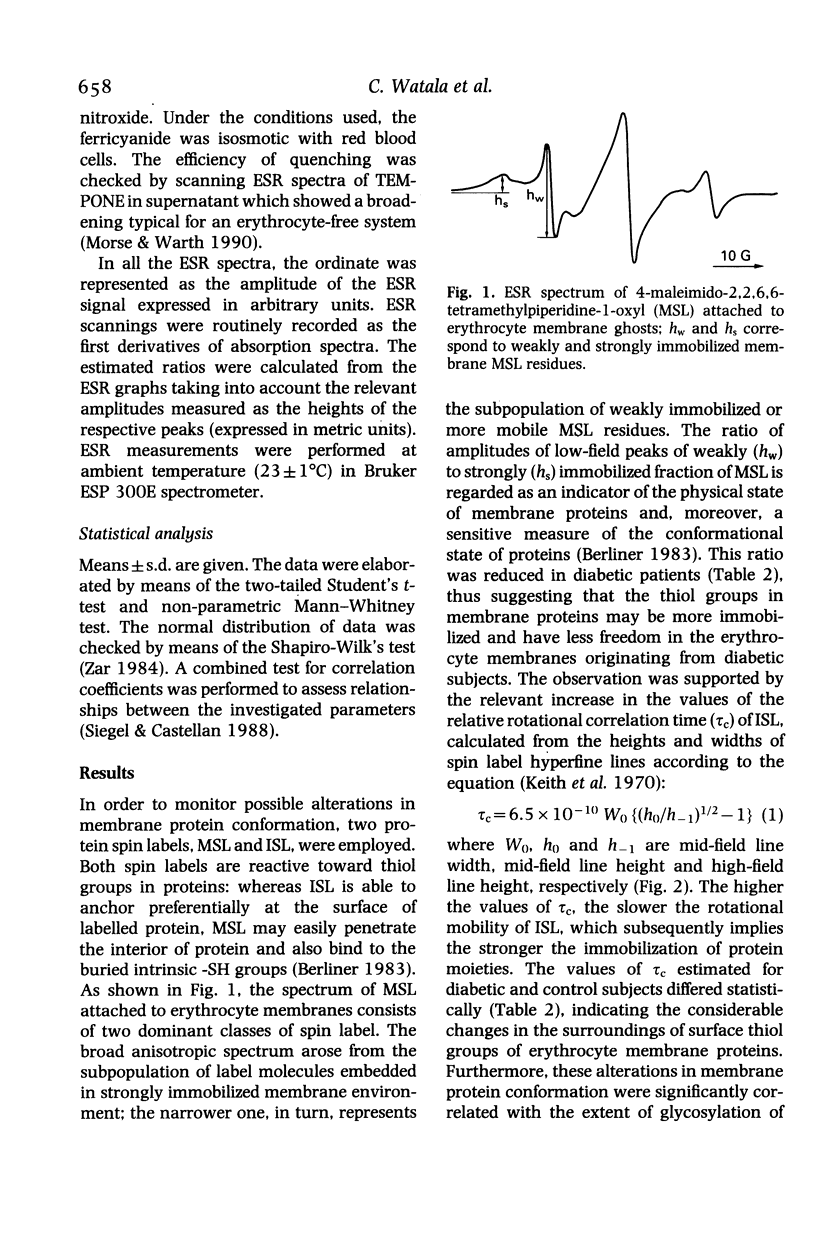
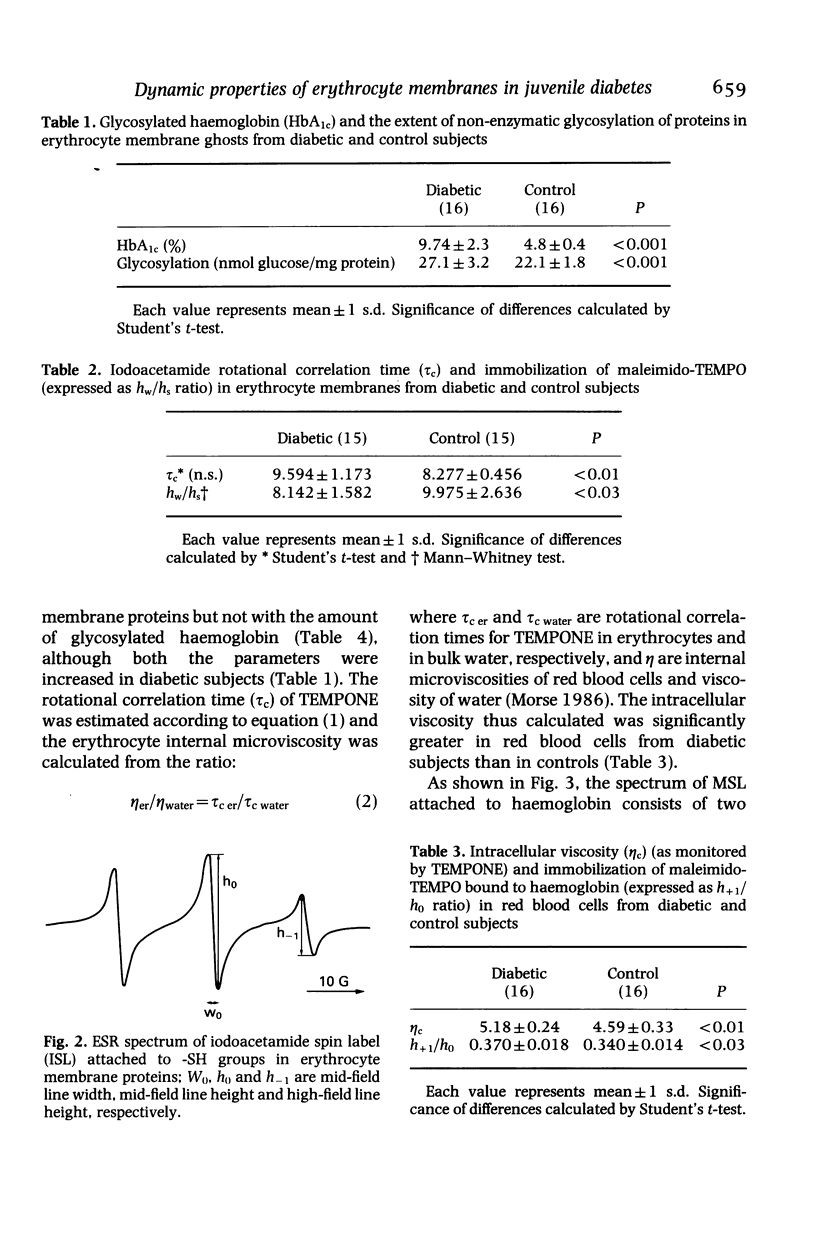
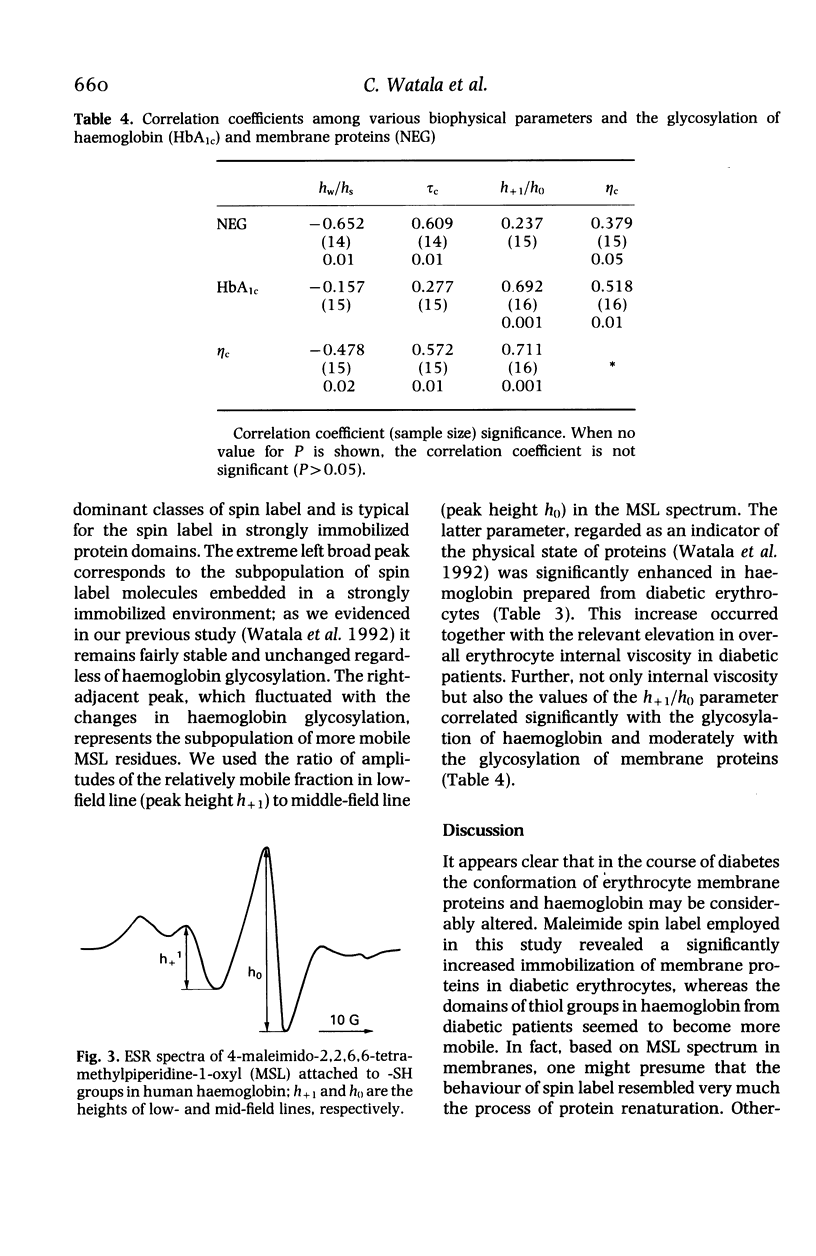
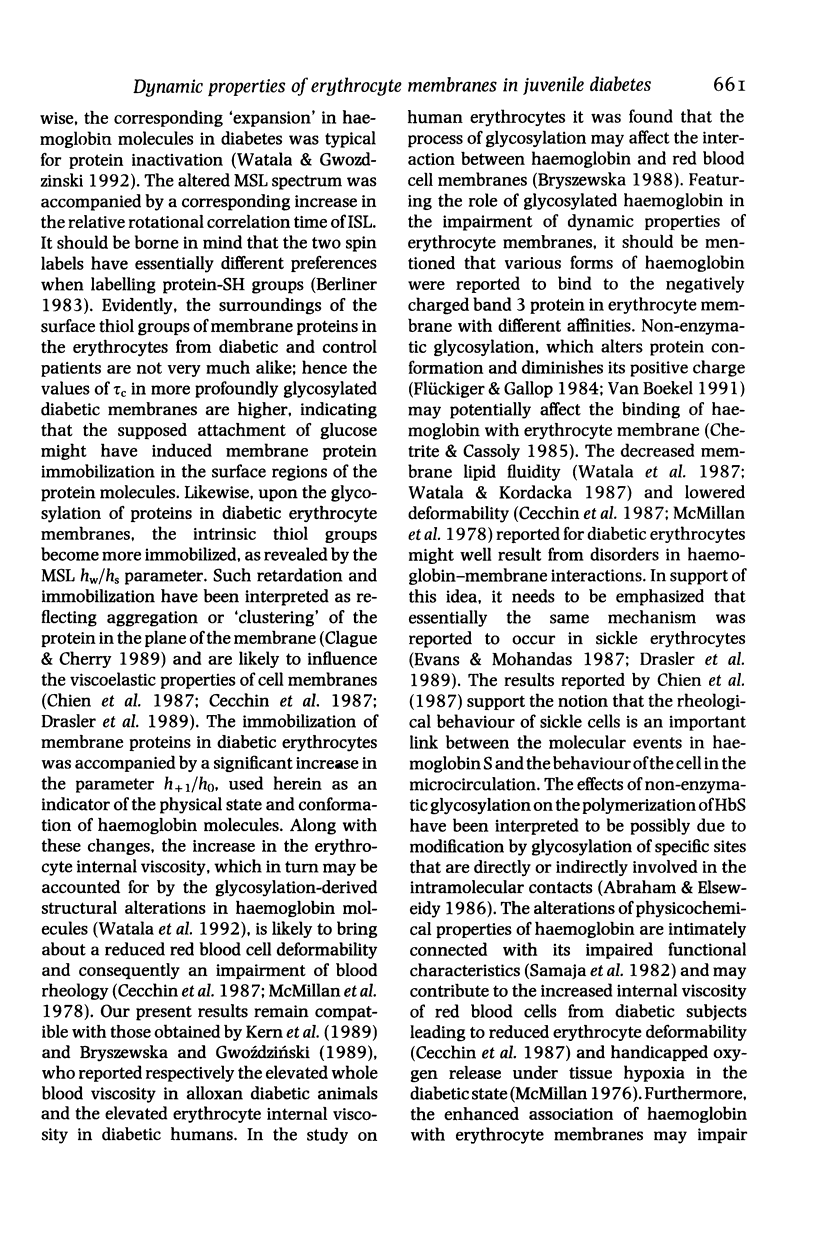
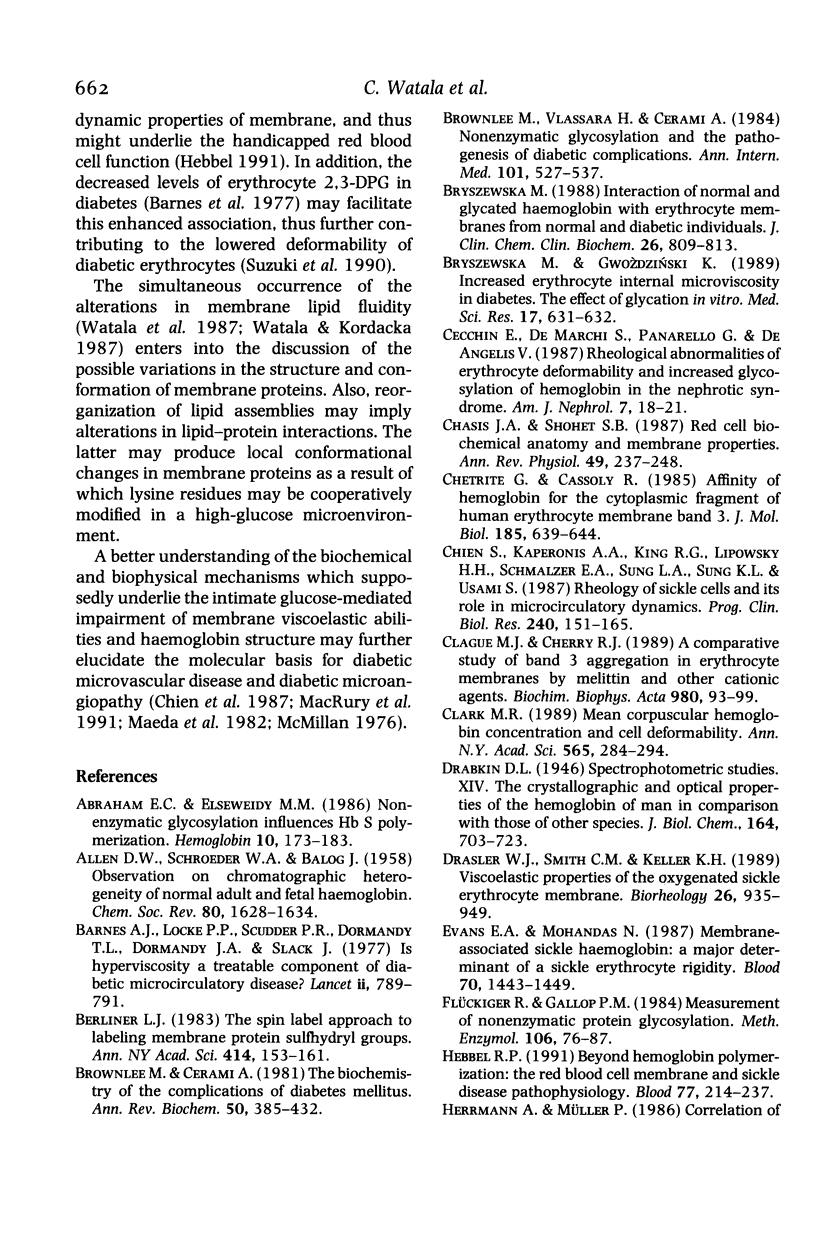
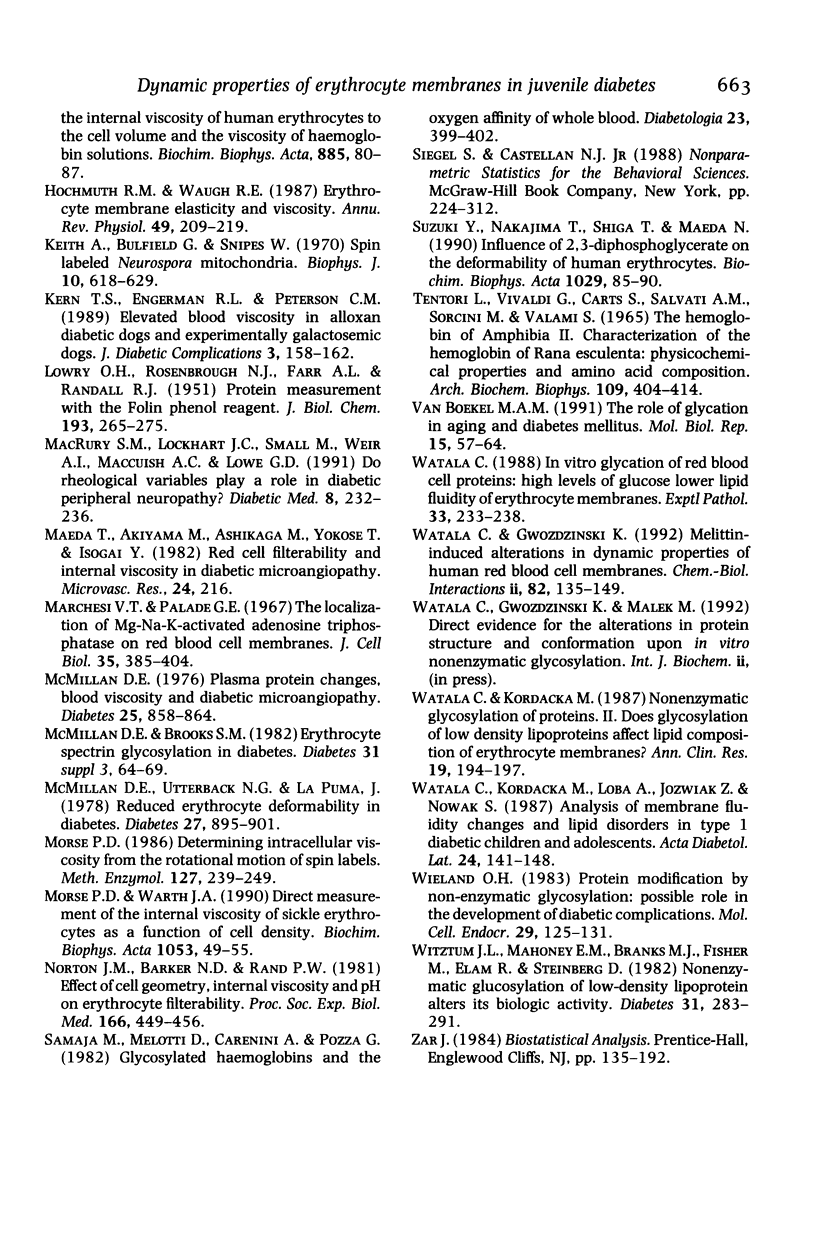
Selected References
These references are in PubMed. This may not be the complete list of references from this article.
- Abraham E. C., Elseweidy M. M. Non-enzymatic glycosylation influences Hb S polymerization. Hemoglobin. 1986;10(2):173–183. doi: 10.3109/03630268609046443. [DOI] [PubMed] [Google Scholar]
- Barnes A. J., Locke P., Scudder P. R., Dormandy T. L., Dormandy J. A., Slack J. Is hyperviscosity a treatable component of diabetic microcirculatory disease? Lancet. 1977 Oct 15;2(8042):789–791. doi: 10.1016/s0140-6736(77)90724-3. [DOI] [PubMed] [Google Scholar]
- Berliner L. J. The spin-label approach to labeling membrane protein sulfhydryl groups. Ann N Y Acad Sci. 1983;414:153–161. doi: 10.1111/j.1749-6632.1983.tb31681.x. [DOI] [PubMed] [Google Scholar]
- Brownlee M., Cerami A. The biochemistry of the complications of diabetes mellitus. Annu Rev Biochem. 1981;50:385–432. doi: 10.1146/annurev.bi.50.070181.002125. [DOI] [PubMed] [Google Scholar]
- Brownlee M., Vlassara H., Cerami A. Nonenzymatic glycosylation and the pathogenesis of diabetic complications. Ann Intern Med. 1984 Oct;101(4):527–537. doi: 10.7326/0003-4819-101-4-527. [DOI] [PubMed] [Google Scholar]
- Bryszewska M. Interaction of normal and glycated human haemoglobin with erythrocyte membranes from normal and diabetic individuals. J Clin Chem Clin Biochem. 1988 Dec;26(12):809–813. doi: 10.1515/cclm.1988.26.12.809. [DOI] [PubMed] [Google Scholar]
- Cecchin E., De Marchi S., Panarello G., De Angelis V. Rheological abnormalities of erythrocyte deformability and increased glycosylation of hemoglobin in the nephrotic syndrome. Am J Nephrol. 1987;7(1):18–21. doi: 10.1159/000167423. [DOI] [PubMed] [Google Scholar]
- Chasis J. A., Shohet S. B. Red cell biochemical anatomy and membrane properties. Annu Rev Physiol. 1987;49:237–248. doi: 10.1146/annurev.ph.49.030187.001321. [DOI] [PubMed] [Google Scholar]
- Chien S., Kaperonis A. A., King R. G., Lipowsky H. H., Schmalzer E. A., Sung L. A., Sung K. L., Usami S. Rheology of sickle cells and its role in microcirculatory dynamics. Prog Clin Biol Res. 1987;240:151–165. [PubMed] [Google Scholar]
- Chétrite G., Cassoly R. Affinity of hemoglobin for the cytoplasmic fragment of human erythrocyte membrane band 3. Equilibrium measurements at physiological pH using matrix-bound proteins: the effects of ionic strength, deoxygenation and of 2,3-diphosphoglycerate. J Mol Biol. 1985 Oct 5;185(3):639–644. doi: 10.1016/0022-2836(85)90076-2. [DOI] [PubMed] [Google Scholar]
- Clague M. J., Cherry R. J. A comparative study of band 3 aggregation in erythrocyte membranes by melittin and other cationic agents. Biochim Biophys Acta. 1989 Mar 27;980(1):93–99. doi: 10.1016/0005-2736(89)90204-6. [DOI] [PubMed] [Google Scholar]
- Clark M. R. Mean corpuscular hemoglobin concentration and cell deformability. Ann N Y Acad Sci. 1989;565:284–294. doi: 10.1111/j.1749-6632.1989.tb24176.x. [DOI] [PubMed] [Google Scholar]
- Drasler W. J., Smith C. M., 2nd, Keller K. H. Viscoelastic properties of the oxygenated sickle erythrocyte membrane. Biorheology. 1989;26(5):935–949. [PubMed] [Google Scholar]
- Evans E. A., Mohandas N. Membrane-associated sickle hemoglobin: a major determinant of sickle erythrocyte rigidity. Blood. 1987 Nov;70(5):1443–1449. [PubMed] [Google Scholar]
- Flückiger R., Gallop P. M. Measurement of nonenzymatic protein glycosylation. Methods Enzymol. 1984;106:77–87. doi: 10.1016/0076-6879(84)06009-2. [DOI] [PubMed] [Google Scholar]
- Hebbel R. P. Beyond hemoglobin polymerization: the red blood cell membrane and sickle disease pathophysiology. Blood. 1991 Jan 15;77(2):214–237. [PubMed] [Google Scholar]
- Hochmuth R. M., Waugh R. E. Erythrocyte membrane elasticity and viscosity. Annu Rev Physiol. 1987;49:209–219. doi: 10.1146/annurev.ph.49.030187.001233. [DOI] [PubMed] [Google Scholar]
- Keith A., Bulfield G., Snipes W. Spin-labeled Neurospora mitochondria. Biophys J. 1970 Jul;10(7):618–629. doi: 10.1016/S0006-3495(70)86324-X. [DOI] [PMC free article] [PubMed] [Google Scholar]
- Kern T. S., Engerman R. L., Peterson C. M. Elevated blood viscosity in alloxan diabetic dogs and experimentally galactosemic dogs. J Diabet Complications. 1989 Jul-Sep;3(3):158–162. doi: 10.1016/0891-6632(89)90039-1. [DOI] [PubMed] [Google Scholar]
- LOWRY O. H., ROSEBROUGH N. J., FARR A. L., RANDALL R. J. Protein measurement with the Folin phenol reagent. J Biol Chem. 1951 Nov;193(1):265–275. [PubMed] [Google Scholar]
- MacRury S. M., Lockhart J. C., Small M., Weir A. I., MacCuish A. C., Lowe G. D. Do rheological variables play a role in diabetic peripheral neuropathy? Diabet Med. 1991 Apr;8(3):232–236. doi: 10.1111/j.1464-5491.1991.tb01578.x. [DOI] [PubMed] [Google Scholar]
- Marchesi V. T., Palade G. E. The localization of Mg-Na-K-activated adenosine triphosphatase on red cell ghost membranes. J Cell Biol. 1967 Nov;35(2):385–404. doi: 10.1083/jcb.35.2.385. [DOI] [PMC free article] [PubMed] [Google Scholar]
- McMillan D. E. Plasma protein changes, blood viscosity, and diabetic microangiopathy. Diabetes. 1976;25(2 Suppl):858–864. [PubMed] [Google Scholar]
- McMillan D. E., Utterback N. G., La Puma J. Reduced erythrocyte deformability in diabetes. Diabetes. 1978 Sep;27(9):895–901. doi: 10.2337/diab.27.9.895. [DOI] [PubMed] [Google Scholar]
- Morse P. D., 2nd Determining intracellular viscosity from the rotational motion of spin labels. Methods Enzymol. 1986;127:239–249. doi: 10.1016/0076-6879(86)27020-2. [DOI] [PubMed] [Google Scholar]
- Morse P. D., 2nd, Warth J. A. Direct measurement of the internal viscosity of sickle erythrocytes as a function of cell density. Biochim Biophys Acta. 1990 Jun 12;1053(1):49–55. doi: 10.1016/0167-4889(90)90025-9. [DOI] [PubMed] [Google Scholar]
- Norton J. M., Barker N. D., Rand P. W. Effect of cell geometry, internal viscosity, and pH on erythrocyte filterability. Proc Soc Exp Biol Med. 1981 Mar;166(3):449–456. doi: 10.3181/00379727-166-41089. [DOI] [PubMed] [Google Scholar]
- Samaja M., Melotti D., Carenini A., Pozza G. Glycosylated haemoglobins and the oxygen affinity of whole blood. Diabetologia. 1982 Nov;23(5):399–402. doi: 10.1007/BF00260950. [DOI] [PubMed] [Google Scholar]
- Suzuki Y., Nakajima T., Shiga T., Maeda N. Influence of 2,3-diphosphoglycerate on the deformability of human erythrocytes. Biochim Biophys Acta. 1990 Nov 2;1029(1):85–90. doi: 10.1016/0005-2736(90)90439-u. [DOI] [PubMed] [Google Scholar]
- Watała C. In vitro glycation of red blood cell proteins: high levels of glucose lower lipid fluidity of erythrocyte membranes. Exp Pathol. 1988;33(4):233–238. doi: 10.1016/s0232-1513(88)80077-x. [DOI] [PubMed] [Google Scholar]
- Watała C., Kordacka M., Loba A., Jówiak Z., Nowak S. Analysis of membrane fluidity alterations and lipid disorders in type I diabetic children and adolescents. Acta Diabetol Lat. 1987 Apr-Jun;24(2):141–148. doi: 10.1007/BF02742852. [DOI] [PubMed] [Google Scholar]
- Watała C., Kordacka M. Nonenzymatic glycosylation of proteins. II. Does glycosylation of low density lipoproteins affect lipid composition of erythrocyte membranes? Ann Clin Res. 1987;19(3):194–197. [PubMed] [Google Scholar]
- Wieland O. H. Protein modification by non-enzymatic glucosylation: possible role in the development of diabetic complications. Mol Cell Endocrinol. 1983 Feb;29(2):125–131. doi: 10.1016/0303-7207(83)90207-1. [DOI] [PubMed] [Google Scholar]
- Witztum J. L., Mahoney E. M., Branks M. J., Fisher M., Elam R., Steinberg D. Nonenzymatic glucosylation of low-density lipoprotein alters its biologic activity. Diabetes. 1982 Apr;31(4 Pt 1):283–291. doi: 10.2337/diab.31.4.283. [DOI] [PubMed] [Google Scholar]
- van Boekel M. A. The role of glycation in aging and diabetes mellitus. Mol Biol Rep. 1991 May;15(2):57–64. doi: 10.1007/BF00364840. [DOI] [PubMed] [Google Scholar]